Abstract
The widespread dissemination of bacterial resistance has led to great attention being paid to finding substitutes for traditionally used antibiotics. Plants are rich in various phytochemicals that could be used as antibacterial therapies. Here, we elucidate the phytochemical profile of Euphorbia canariensis ethanol extract (EMEE) and then elucidate the antibacterial potential of ECEE against Pseudomonas aeruginosa clinical isolates. ECEE showed minimum inhibitory concentrations ranging from 128 to 512 µg/mL. The impact of ECEE on the biofilm-forming ability of the tested isolates was elucidated using crystal violet assay and qRT-PCR to study its effect on the gene expression level. ECEE exhibited antibiofilm potential, which resulted in a downregulation of the expression of the biofilm genes (algD, pelF, and pslD) in 39.13% of the tested isolates. The antibacterial potential of ECEE was studied in vivo using a lung infection model in mice. A remarkable improvement was observed in the ECEE-treated group, as revealed by the histological and immunohistochemical studies. Also, ELISA showed a noticeable decrease in the oxidative stress markers (nitric oxide and malondialdehyde). The gene expression of the proinflammatory marker (interleukin-6) was downregulated, while the anti-inflammatory biomarker was upregulated (interleukin-10). Thus, clinical trials should be performed soon to explore the potential antibacterial activity of ECEE, which could help in our battle against resistant pathogenic bacteria.
Introduction
Pseudomonas aeruginosa is a Gram-negative pathogenic bacterium that can cause a wide range of infections that could be mild or life-threatening, like sepsis. It is an opportunistic pathogenic bacterium widely disseminated in hospitals, causing nosocomial infections [Citation1]. It is a highly problematic pathogen, especially in critically ill patients, neutropenic patients, and those who suffer from cystic fibrosis, as well as burn injuries [Citation2].
Respiratory diseases are considered one of the most common causes of death globally. Respiratory infections are an important section in the respiratory diseases that have drawn much attention, especially after the COVID-19 pandemic [Citation3]. Much effort is being developed and applied to control such infections and to lessen their detrimental effects on human health. P. aeruginosa is commonly causing upper and lower respiratory tract infections [Citation4].
Recently, the irrational prescription and use of antimicrobials have led to the spread of serious antibiotic resistance among pathogenic bacteria, particularly P. aeruginosa. This has led to serious consequences of healthcare-related costs and high mortality rates [Citation5]. Therefore, finding and elucidating novel treatments for such resistant and challenging bacterial infections is important.
Plants are a valuable source of many phytochemicals with various pharmacological potentials [Citation6,Citation7]. Euphorbia has more than 2000 species and is a prominent angiosperm genus found worldwide, including in Asia, Africa, and Central and South America. Members of this genus have different morphologies ranging from small annual or perennial herbs to woody shrubs, vines, trees, and even succulent plants, as well as the production of a milky irritating latex. In general, chemical and pharmacological research was conducted on all plants, stems, leaves, latex, roots, and seeds of Euphorbia [Citation8,Citation9].
Different Euphorbia species have been receiving increased attention recently as a source of potential treatment for various ailments in terms of prevention and treatment [Citation10]. The diverse chemical constituents of some species of Euphorbia may be the cause of these treatment capabilities. Among the most researched chemical constituents are the polycyclic diterpenoids obtained from Euphorbia plants as tigline (phorbol esters), ingenane (ingenol esters), jatrophane, and lathyrane skeletons. These diterpenoids have been discovered to be skin irritants, tumour promoters, anticancer agents, and, more recently, agents for overcoming multidrug resistance (anti-MDR). They are physiologically active in a variety of ways. In addition to kaempferol, quercetin, apigenin, luteolin, and their various glycosides are some of the most prevalent flavonoids in the Euphorbia genus [Citation11,Citation12]. E. canariensis was reported to have diterpenes such as 2,3-di epiingol 7,12- diacetate-8-isobutyrate, ingnol-3- angelate- 17-benzoate, ingenol-3- anglate- 17-benz0ate-20-acetate and 3,5,7,8,9,15-hexahydroxyjatropha-6(17), 1 l-dien14-one-5,8-bis(2-methylbutyrate)-7-(2-methylpropionate) [Citation13].
In this study, we aimed to investigate the antimicrobial activity of ECEE in P. aeruginosa clinical isolates after elucidating its secondary metabolites by LC/MS/MS to find novel alternatives to the currently utilized antibiotics that face resistance.
Results
Phytochemical profile of ECEE
A total of 25 components were tentatively determined in ECEE using LC-ESI-MS/MS in a positive mode. The main substances were several types of flavonoids and their glycosidic derivatives, alkaloid derivatives, organic, fatty and carboxylic acids. The comprehensive profile is listed in .
Table 1. Phytochemical profiling of ECEE by LC-MS/MS analysis (positive mode ESI).
In vitro
Antibacterial potential
ECEE revealed antibacterial action against the tested isolates by agar well diffusion method. The minimum inhibitory concentration (MIC) values detected by broth microdilution assay revealed that ECEE had MIC values of 128 to 512 µg/mL (Table S1).
Antibiofilm action
ECEE decreased the number of biofilm-forming isolates (strong plus moderate forming isolates) from 69.57% to 30.43% ().
Table 2. Impact of ECEE on the biofilm formation of P. aeruginosa isolates.
Owing to the detected antibiofilm action at the phenotypic level, qRT-PCR was employed as a molecular method to elucidate the potential effect of ECEE on the expression of the genes encoding biofilm formation in P. aeruginosa as revealed in . Interestingly, ECEE resulted in downregulating the genes encoding biofilm formation in 39.13% of the tested isolates.
In vivo study
Lung bacterial load of the different groups
As shown in , ECEE significantly diminished (p < 0.05) the number of colony forming unit per gram (CFU/g) lung tissues. The ECEE-treated group revealed a non-significant (p > 0.05) change in relation to the ciprofloxacin-treated group.
Histology and immunohistochemistry
The impact of ECEE on the histological features of the lung tissues is shown in and after staining with haematoxylin and eosin (H&E). Also, the nuclear factor-kappa B (NF-κB) and caspase-3 immunostained lung sections are shown in .
Figure 3. Histological features of the lung tissues stained with H&E of A) Group I with normal-sized alveoli separated by fibrous septa (blue arrows), normal-sized bronchioles (black arrows), and normal-sized blood vessels (red arrow) (×100). B) Group II with dilated destructed bronchioles (black arrows), surrounded by marked alveolar fibrosis (blue arrows), marked inflammation (green arrows), and vascular congestion (red arrow) (×100). C) Group III with normal-sized bronchioles (black arrows), surrounded by normal-sized alveoli (blue arrows) with focal alveolar and vascular congestion (red arrows) (×100). D) Group IV with dilated bronchiole (black arrow), surrounded by normal-sized alveoli (blue arrows) with focal alveolar congestion (red arrow) (×100).
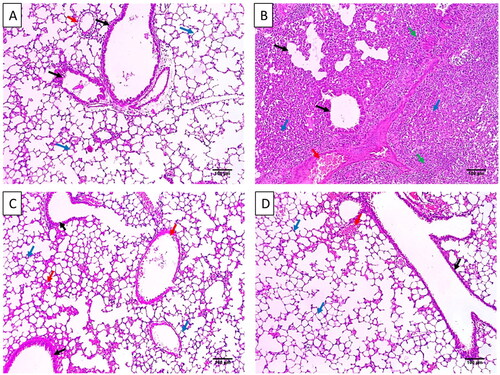
Figure 4. Masson’s trichrome staining of A) Group I with bronchioles and vessels surrounded by minimal blue stained collagen (red arrows) (×100). B) Group II with dilated bronchioles surrounded by marked fibrosis (red arrows) (×100). C) Group III with minimal fibrosis (red arrows) (×100). D) Group IV with mild fibrosis (red arrows) (×100).
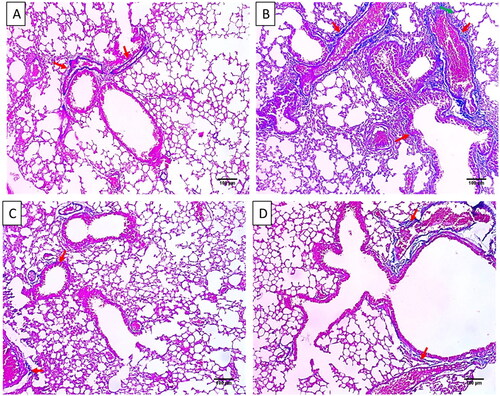
Figure 5. Immunostained lung sections of A) Group I exhibited a negative expression of caspase-3 (0.12%) (×100). B) Group II exhibited a strong positive caspase-3 expression (87.24%) (×100). C) Group III exhibited a weak positive caspase-3 expression (17.14%) (×100). D) Group IV exhibited a weak positive caspase-3 expression (18.16%). E) Group I presented a negative expression of NF-kB (2.63%) (×100). F) Group II exhibited a strong positive NF-kB expression (82.17%) (×100). G) Group III exhibiting a weak positive NF-kB expression (16.25%) (×100). H) Group IV exhibited a weak positive NF-kB expression (18.06%) (×100).
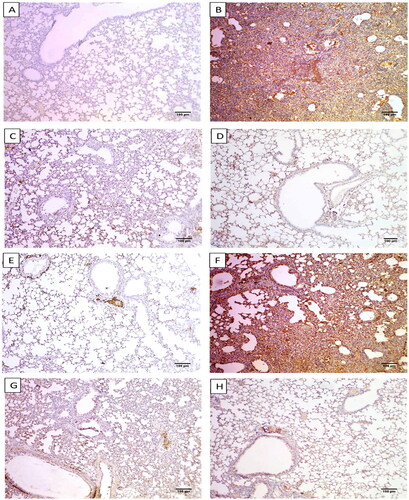
Colorimetric assay and qRT-PCR
Owing to the effect of bacterial infection on increasing oxidative stress and inflammation, we used colorimetric methods and qRT-PCR to detect the markers of such effects. The impact of ECEE on the oxidative stress markers, including nitric oxide (NO) and malondialdehyde (MDA), was studied in the lung tissues, as shown in . Also, using qRT-PCR, the impact of ECEE on the inflammatory markers, interleukins, IL-6 and IL-10 was elucidated in .
Discussion
Antibacterial activity of the medicinal plant extracts was widely elucidated owing to the spread of antibiotic resistance among the pathogenic bacteria [Citation14]. Here, the antibacterial activity of ECEE was revealed in vitro using the agar well diffusion method, and its MIC values ranged from 128 to 512 µg/mL. Many reports of promising antibacterial activity in different plant extracts are attributed to their many bioactive compounds [Citation15–17].
Numerous biological properties of Euphorbia plant species include antibacterial, antifungal, antiviral, antiplasmodial, antioxidant, anticancer, larvicidal, and molluscicidal effects. Euphorbia species also showed cytotoxic effects on numerous cell lines, including the human lung fibroblast cell line (MRC-5) [Citation18]
Euphorbia canariensis has 25 bioactive metabolites found using the positive ionisation mode of LC-ESI-MS/MS. The identified metabolites belong to several phytochemical classes, such as flavonoids, alkaloid derivatives, and organic, fatty and carboxylic acids, which aligns with the earlier literature on different Euphorbia species [Citation10,Citation18].
Biofilm formation is an important virulence factor of P. aeruginosa, enabling these pathogenic bacteria to resist antibiotics [Citation1]. Thus, finding antibiofilm agents is highly needed in combating bacterial resistance. Crystal violet assay was performed to assess the biofilm-forming ability of P. aeruginosa isolates and to reveal the impact of ECEE on biofilm. Also, qRT-PCR was utilised as a molecular method to investigate the potential effect of ECEE on the biofilm formation genes in the tested isolates. Interestingly, ECEE decreased the number of the biofilm-forming isolates (strong plus moderate forming isolates) from 69.57% to 30.43%. ECEE also resulted in a downregulation of the genes encoding biofilm formation (algD, pelF and pslD) in 39.13% of the tested isolates. These genes are involved in synthesising the exopolysaccharides that constitute a major component of the biofilm in which the bacterial cells are embedded [Citation19].
The lung infection model in mice was used to elucidate the potential activity of ECEE on P. aeruginosa in vivo. ECEE significantly diminished (p < 0.05) the number of CFU/g lung tissues. The lung is a common organ usually infected by P. aeruginosa bacteria, and serious consequences occur after this infection. Thus, the lung infection model was selected in the current study.
Inflammation is a protective reaction that occurs by the host’s body as a response to tissue damage and infections. In the inflammatory process of lung infection, events usually occur, like vasodilation, destructed bronchioles, marked alveolar fibrosis, and vascular congestion [Citation20,Citation21]. Remarkably, these findings were diminished by treatment with ECEE, as revealed by the histopathological studies.
NF-κB are inducible transcription factors regulating different inflammatory genes [Citation22]. It has been established that apoptosis is involved in different infections as it is a fundamental pathway in the interaction between the host and the pathogenic bacteria [Citation23]. Though apoptosis could eliminate the compromised and infected cells, excessive induction of apoptosis could lead to harmful effects. It could disturb the normal tissues that protect against infection, like epithelial barriers or remove the circulating immune cells [Citation24]. Here, by immunohistochemistry studies, ECEE exhibited a decrease in the NF-κB and caspase-3 (as a marker for apoptosis) immune cells.
Oxidative stress occurs if there is an imbalance between the reactive oxygen species (ROS) level and the ability of the cell to get rid of them. Oxidative stress occurs in bacterial infections, which could result in organ damage [Citation25]. Here, ECEE was found to substantially decrease the levels of NO and MDA, which are oxidative stress biomarkers.
IL-6 is a proinflammatory cytokine that potentiates bacterial infections’ inflammatory process [Citation26]. On the other hand, IL-10 has a potent anti-inflammatory activity that could limit the host immune response towards pathogens and lessen the potential tissue damage that usually accompanies bacterial infections [Citation27]. Here, ECEE resulted in a remarkable reduction in the gene expression of IL-6 and upregulation of IL-10.
Materials and methods
Plant collection and analysis
Euphorbia canariensis aerial parts were gathered from a local garden, Gharbia Governorate, in April 2022. The plant was recognised by Dr. Esrraa Amar, Faculty of Science, Tanta University. A voucher sample (PG-A-EC-W-21) was deposited at the Herbarium of Plant Ecology, Tanta University. Plant preparation is listed in supporting data.
Extract of E. canariensis aerial parts was analysed by adopting procedures previously reported by Attallah et al. [Citation28] using positive ion mode.
In vitro antibacterial activity
Twenty-three P. aeruginosa isolates were utilised in the current study to reveal the antibacterial potential of ECEE. The bacterial isolates were obtained from the Department of Microbiology and Immunology culture collection, Faculty of Pharmacy, Tanta University.
Using agar well diffusion technique, the antibacterial action of ECEE was elucidated by making wells in the Muller-Hinton agar. ECEE (2000 µg/mL) was poured into one of these wells, and the other wells contained a positive control (ciprofloxacin) and a negative control (dimethyl sulfoxide), as previously reported [Citation29]. The Minimum inhibitory concentration values were determined using the broth microdilution method in microtitration plates [Citation30] using Muller–Hinton broth. The
In vitro antibiofilm activity
Crystal violet assay
This test was utilized to elucidate the biofilm-forming ability of the tested isolates and the effect of ECEE on this capability, as previously explained [Citation31]. Using 96 well microtitration plates, P. aeruginosa isolates were grouped into four categories according to the measured optical density values at 490 nm (strong, moderate, weak, and none-biofilm forming) [Citation32].
Relative gene expression of the biofilm genes
The expression of the genes that encode the production of the biofilm exopolysaccharides (algD, pelF and pslD) [Citation19] was studied before and after treatment with ECEE using qRT-PCR after extraction of the total RNA by Purelink™ RNA Mini Kit (Thermo Scientific, Waltham, USA). Then, cDNA was formed using a power™ cDNA synthesis kit (iNtRON Biotechnology, Korea), and qRT-PCR was carried out by Rotor-Gene Q 5plex (Qiagen, Germany) by SYBR green (Thermo Scientific, Waltham, USA) using oprL as a housekeeping gene [Citation33,Citation34]. The utilized primers are in Table S1. The fold change was calculated using 2 -ΔΔCT [Citation35].
In vivo antibacterial activity
Experimental procedures
The utilized mice in this experiment were obtained from the animal house of the faculty of veterinary medicine, Cairo University, Egypt. All standard procedures for handling laboratory animals were followed according to ARRIVE guidelines. The experiment protocol was approved by the research ethical committee of the faculty of pharmacy, Tanta University, Egypt (TP/RE/5/23 p-0024).
The antibacterial efficiency of ECEE was revealed in vivo by a lung infection model using P. aeruginosa suspension (107 CFU/mL). Briefly, after anaesthetising the animals, 25 µL of the bacterial suspension was put in each mouse nares of all groups except for group I, which served as the negative or the normal control. The other three groups were infected, and group II (positive control) didn’t receive any treatment. Group III received ciprofloxacin as a standard treatment, and Group IV received ECEE (100 mg/kg). All the administered treatments were taken orally after four hours, one day, and two days [Citation36]. After three days, the mice were anaesthetised and euthanized. Lung tissues were obtained for histological examination, immunohistochemical, colorimetric, and qRT-PCR studies. The bacterial load was determined and expressed as the number of CFU/g lung tissues.
Histology and immunohistochemistry
H&E and Masson’s trichrome staining were used to stain the lung tissues of the tested groups as previously reported [Citation37,Citation38]. A digital camera captured photos. Using NF-kB and caspase-3 monoclonal antibodies, the lung tissues were stained for immunohistochemical studies, and the percentage of the positive immune reactive cells was quantified as previously reported [Citation39].
Colorimetric assay
The NO levels and MDA levels were identified in the lung tissues at 540 nm using Biodiagnostic colorimetric kits (Egypt).
qRT-PCR for inflammatory markers
The inflammatory markers’ expression level, IL-6 and IL-10, was elucidated using qRT-PCR, as previously explained by using the primers listed in Table S2 [Citation28,Citation40].
Statistical analysis
Using Prism version 8 (USA), ANOVA test was applied to investigate the significance at p < 0.05. The tests were carried out three times and revealed as mean ± standard deviation (SD).
Conclusions
The results of this study displayed that ECEE has antibacterial activity towards P. aeruginosa isolates in vitro using the agar dilution method and broth microdilution method. It also exhibited a potential antibiofilm action by crystal violet and qRT-PCR. In addition, the in vivo finding revealed that ECEE decreased the bacterial load in the lung and improved the lung histological as well as the immunohistochemical features. Also, there was a remarkable decrease in oxidative stress and inflammatory markers. So, future studies need to be performed to elucidate the potential antimicrobial activity of ECEE against other pathogenic microbes.
Informed consent statement
Not applicable.
Institutional review board statement
All standard procedures of handling of laboratory animals were followed and the experiment protocol was approved by the research ethical committee of faculty of pharmacy, Tanta University, Egypt (TP/RE/5/23 p-0024).
Author’s contribution
BA: conceptualisation, data curation, methodology, and formal analysis, EE: conceptualisation, data curation, formal analysis, methodology, writing original draft, and editing. TAE: methodology, writing original draft and revision and editing, AS: methodology, writing original draft and revision and editing, MEA: investigation, methodology, and writing original draft, KNA: data curation, methodology, writing original draft, and formal analysis, WAN: conceptualisation, data curation, formal analysis, methodology, writing original draft, and editing. All authors have read and agreed to the published version of the manuscript.
Supplemental Material
Download MS Word (19.1 KB)Acknowledgments
This work was funded by the Deanship of Scientific Research at Princess Nourah bint Abdulrahman University, Riyadh, Saudi Arabia, through the Research Groups Program Grant no. (RGP-1443-0044). The authors, therefore, gratefully acknowledge the DSR technical and financial support.
Disclosure statement
No potential conflict of interest was reported by the author(s).
Data availability statement
All data that support the finding displayed in our study are included in the manuscript. Any further data is available upon request.
Additional information
Funding
References
- Qin S, Xiao W, Zhou C, et al. Pseudomonas aeruginosa: pathogenesis, virulence factors, antibiotic resistance, interaction with host, technology advances and emerging therapeutics. Signal Transduct Target Ther. 2022;7(1):199. doi: 10.1038/s41392-022-01056-1.
- Tuon FF, Dantas LR, Suss PH, et al. Pathogenesis of the Pseudomonas aeruginosa biofilm: a review. Pathogens. 2022;11(3):300. doi: 10.3390/pathogens11030300.
- Murray CJ, Ikuta KS, Sharara F, et al. Global burden of bacterial antimicrobial resistance in 2019: a systematic analysis. The Lancet. 2022;399(10325):629–655. doi: 10.1016/S0140-6736(21)02724-0.
- Kunz Coyne AJ, El Ghali A, Holger D, et al. Therapeutic strategies for emerging multidrug-resistant Pseudomonas aeruginosa. Infect Dis Ther. 2022;11(2):661–682. doi: 10.1007/s40121-022-00591-2.
- Serwecińska L. Antimicrobials and antibiotic-resistant bacteria: a risk to the environment and to public health. Water. 2020;12(12):3313. doi: 10.3390/w12123313.
- Aziz E, Batool R, Akhtar W, et al. Rosemary species: a review of phytochemicals, bioactivities and industrial applications. S Afr J Bot. 2022;151:3–18. doi: 10.1016/j.sajb.2021.09.026.
- Adnan M, Rasul A, Hussain G, et al. Ginkgetin: a natural biflavone with versatile pharmacological activities. Food Chem Toxicol. 2020;145:111642. doi: 10.1016/j.fct.2020.111642.
- Zhao H, Sun L, Kong C, et al. Phytochemical and pharmacological review of diterpenoids from the genus euphorbia linn (2012–2021). J Ethnopharmacol. 2022;298:115574. doi: 10.1016/j.jep.2022.115574.
- Xu Y, Tang P, Zhu M, et al. Diterpenoids from the genus euphorbia: structure and biological activity (2013–2019). Phytochemistry. 2021;190:112846. doi: 10.1016/j.phytochem.2021.112846.
- Jassbi AR. Chemistry and biological activity of secondary metabolites in euphorbia from Iran. Phytochemistry. 2006;67(18):1977–1984. doi: 10.1016/j.phytochem.2006.06.030.
- Chamkhi I, Hnini M, Aurag J. Conventional medicinal uses, phytoconstituents, and biological activities of euphorbia officinarum L.: a systematic review. Adv Pharmacol Pharm Sci. 2022;2022:9971085–9971089. doi: 10.1155/2022/9971085.
- Amtaghri S, Akdad M, Slaoui M, et al. Traditional uses, pharmacological, and phytochemical studies of euphorbia: a review. Curr Top Med Chem. 2022;22(19):1553–1570. doi: 10.2174/1568026622666220713143436.
- Miranda FJ, Alabadí JA, Ortí M, et al. Comparative analysis of the vascular actions of diterpenes isolated from euphorbia canariensis. J Pharm Pharmacol. 1998;50(2):237–241. doi: 10.1111/j.2042-7158.1998.tb06182.x.
- Vaou N, Stavropoulou E, Voidarou C, et al. Towards advances in medicinal plant antimicrobial activity: a review study on challenges and future perspectives. Microorganisms. 2021;9(10):2041. doi: 10.3390/microorganisms9102041.
- Gonelimali FD, Lin J, Miao W, et al. Antimicrobial properties and mechanism of action of some plant extracts against food pathogens and spoilage microorganisms. Front Microbiol. 2018;9:1639. doi: 10.3389/fmicb.2018.01639.
- Mostafa AA, Al-Askar AA, Almaary KS, et al. Antimicrobial activity of some plant extracts against bacterial strains causing food poisoning diseases. Saudi J Biol Sci. 2018;25(2):361–366. doi: 10.1016/j.sjbs.2017.02.004.
- Hemeg HA, Moussa IM, Ibrahim S, et al. Antimicrobial effect of different herbal plant extracts against different microbial population. Saudi J Biol Sci. 2020;27(12):3221–3227. doi: 10.1016/j.sjbs.2020.08.015.
- El-Hawary SS, Mohammed R, Tawfike AF, et al. Cytotoxic activity and metabolic profiling of fifteen euphorbia species. Metabolites. 2020;11(1):15. doi: 10.3390/metabo11010015.
- Banar M, Emaneini M, Satarzadeh M, et al. Evaluation of mannosidase and trypsin enzymes effects on biofilm production of Pseudomonas aeruginosa isolated from burn wound infections. PLoS One. 2016;11(10):e0164622. doi: 10.1371/journal.pone.0164622.
- Ansar W, Ghosh S. Inflammation and inflammatory diseases, markers, and mediators: role of CRP in some inflammatory diseases. Biology of C Reactive Protein in Health and Disease. 2016;20:67–107.
- Ning Q, Wu D, Wang X, et al. The mechanism underlying extrapulmonary complications of the coronavirus disease 2019 and its therapeutic implication. Signal Transduct Target Ther. 2022;7(1):57. doi: 10.1038/s41392-022-00907-1.
- Bhatt D, Ghosh S. Regulation of the NF-κB-mediated transcription of inflammatory genes. Front Immunol. 2014;5:71. doi: 10.3389/fimmu.2014.00071.
- Jorgensen I, Rayamajhi M, Miao EA. Programmed cell death as a defence against infection. Nat Rev Immunol. 2017;17(3):151–164. doi: 10.1038/nri.2016.147.
- Yu X, He S. The interplay between human herpes simplex virus infection and the apoptosis and necroptosis cell death pathways. Virol J. 2016;13(1):77. doi: 10.1186/s12985-016-0528-0.
- Sharifi-Rad M, Anil Kumar NV, Zucca P, et al. Lifestyle, oxidative stress, and antioxidants: back and forth in the pathophysiology of chronic diseases. Front Physiol. 2020;11:694. doi: 10.3389/fphys.2020.00694.
- Kany S, Vollrath JT, Relja B. Cytokines in inflammatory disease. Int J Mol Sci. 2019;20(23):6008. doi: 10.3390/ijms20236008.
- Ouyang W, O’Garra A. IL-10 family cytokines IL-10 and IL-22: from basic science to clinical translation. Immunity. 2019;50(4):871–891. doi: 10.1016/j.immuni.2019.03.020.
- Attallah NG, El-Sherbeni SA, El-Kadem AH, et al. Elucidation of the metabolite profile of yucca gigantea and assessment of its cytotoxic, antimicrobial, and anti-inflammatory activities. Molecules. 2022;27(4):1329. doi: 10.3390/molecules27041329.
- Alotaibi B, El-Masry TA, Elekhnawy E, et al. Aqueous core epigallocatechin gallate PLGA nanocapsules: characterization, antibacterial activity against uropathogens, and in vivo reno-protective effect in cisplatin induced nephrotoxicity. Drug Deliv. 2022;29(1):1848–1862. doi: 10.1080/10717544.2022.2083725.
- Elekhnawy E, Sonbol F, Abdelaziz A, et al. An investigation of the impact of triclosan adaptation on Proteus mirabilis clinical isolates from an egyptian university hospital. Braz J Microbiol. 2021;52(2):927–937. doi: 10.1007/s42770-021-00485-4.
- Attallah NG, Negm WA, Elekhnawy E, et al. Antibacterial activity of boswellia sacra flueck. Oleoresin extract against Porphyromonas gingivalis periodontal pathogen. Antibiotics. 2021;10(7):859. doi: 10.3390/antibiotics10070859.
- Attallah NG, Al-Fakhrany OM, Elekhnawy E, et al. Anti-biofilm and antibacterial activities of cycas media R. Br secondary metabolites: in silico, in vitro, and in vivo approaches. Antibiotics. 2022;11(8):993. doi: 10.3390/antibiotics11080993.
- Abdel Bar FM, Alossaimi MA, Elekhnawy E, et al. Anti-Quorum sensing and anti-biofilm activity of pelargonium × hortorum root extract against Pseudomonas aeruginosa: combinatorial effect of catechin and gallic acid. Molecules. 2022;27(22):7841. doi: 10.3390/molecules27227841.
- Alomair BM, Al-Kuraishy HM, Al-Buhadily AK, et al. Is sitagliptin effective for SARS-CoV-2 infection: false or true prophecy? Inflammopharmacology. 2022;30(6):2411–2415. doi: 10.1007/s10787-022-01078-9.
- Livak KJ, Schmittgen TD. Analysis of relative gene expression data using real-time quantitative PCR and the 2− ΔΔCT method. Methods. 2001;25(4):402–408. doi: 10.1006/meth.2001.1262.
- Binsuwaidan R, Sultan AA, Negm WA, et al. Bilosomes as nanoplatform for oral delivery and modulated in vivo antimicrobial activity of lycopene. Pharmaceuticals. 2022;15(9):1043. doi: 10.3390/ph15091043.
- Alherz FA, Negm WA, Elekhnawy E, et al. Silver nanoparticles prepared using encephalartos laurentianus De wild leaf extract have inhibitory activity against Candida albicans clinical isolates. JoF. 2022;8(10):1005. doi: 10.3390/jof8101005.
- Al-Kuraishy HM, Al-Gareeb AI, Albogami SM, et al. Potential therapeutic benefits of metformin alone and in combination with sitagliptin in the management of type 2 diabetes patients with COVID-19. Pharmaceuticals. 2022;15(11):1361. doi: 10.3390/ph15111361.
- Wang M, Weng X, Chen H, et al. Resveratrol inhibits TNF-α-induced inflammation to protect against renal ischemia/reperfusion injury in diabetic rats. Acta Cir Bras. 2020;35(5):219. doi: 10.1590/s0102-865020200050000006.
- Batiha GE-S, Al-Gareeb AI, Elekhnawy E, et al. Potential role of lipoxin in the management of COVID-19: a narrative review. Inflammopharmacology. 2022;30(6):1993–2001. doi: 10.1007/s10787-022-01070-3.