ABSTRACT
Until recently folate receptor alpha (FRα) has only been considered as a folate transporter. However, a novel role of FRα as a transcription factor was reported by our lab. More recently our lab showed a novel pleiotropic role of FRα: (a) direct transcriptional activation of Oct4, Sox2, and Klf4 genes; and (b) repression of biogenesis of miRNAs that target these genes or their effector molecules. These observations beg a question: “Can a simple molecule such as folate be used to manipulate the production and/or differentiation of endogenous neural stem cells (NSCs), which may hold promise for future therapies?” Conditions such as spinal cord injury, motor neuron diseases, Alzheimer's disease and multiple sclerosis may benefit from increasing stem cell pool and promoting specific pathways of differentiation. On the flip-side, these NSCs may also contribute to some CNS tumors therefore promoting differentiation could prove more beneficial. FRα may hold promises for both since it has the potential to remodel chromatin in a context dependent manner. In this commentary we discuss our previous data and new questions arising in the context of the new role for FRα.
Introduction
FA is a biologically inactive water-soluble B vitamin that is transformed to bioactive forms in vivo. Naturally occurring folates exist in many chemical forms; folates are found in food, as well as in metabolically active forms in the human body. Folic acid is the major synthetic form found in fortified foods and vitamin supplements. Folate is a generic term referring to both natural folates in food and folic acid; the synthetic form used in supplements and fortified food. Folate is critical in the metabolism of nucleic acid precursors and several amino acids, as well as in methylation reactions. Severe deficiency in either folate or vitamin B12 can lead to megaloblastic anemia, which causes fatigue, weakness, and shortness of breath and thus leave the individual at risk of developing irreversible brain damage. Folate status is influenced by the presence of genetic variations in folate metabolism, particularly those found in the 5, 10-methylenetetrahydrofolate reductase. Inadequate folate status during early pregnancy increases the risk of congenital anomalies, such as neural tube defects and cardiovascular disease. Additionally, folate is essential for brain development. Low folate status and/or high homocysteine concentrations are associated with cognitive dysfunction in aging.
Folate receptor alpha (FRα) is cysteine-rich cell-surface glycoprotein that binds folate with high affinity to mediate cellular uptake of folate. FRα expression is restricted to few epithelial tissues.Citation1 Although expressed at very low levels in most tissues, FRα is expressed at high levels in numerous cancers to meet the high folate demand of rapidly dividing cells under low folate conditions.Citation1-4 Although folate is required for rapidly dividing cancer cells, the role of FRα behaving like a transcription factor and activating oncogenic genes point out to the fact that FRα have other undiscovered functions.Citation5,6 which aid stem cell proliferation and possibly tumorigenesis.
Role of FRα in maintaining stem cell pool and subsequent neurogenesis
OCT4
Stem cell maintenance and regulation of differentiation is a key aspect to neuronal generation during both embryonic development and in adulthood. An improved understanding of the stem cell microenvironment to be targeted by exogenous stimulators such as folate is critical for our ability to predict responses of endogenous cells to drugs or biologicals. Our recent work has demonstrated folate's ability, via the receptor protein folate receptor alpha (FRα), to regulate expression of genes such as Hes1, Oct4, Sox2, Klf4, and TRIM71, which all work to maintain the stem cell pool.Citation5,6
KDM6B
Earlier weCitation7 showed that folate downregulates the miRNAs (miR-138, miR-148a, miR-185, miR-339-5p) which target H3K27 demethylase KDM6B levels, indirectly upregulating KDM6B and decreasing the methylation on H3K27. KDM6B was associated with Hes1 promoter during cell proliferative stages (E10.5) and Neurog2 promoters at the start of neurogenesis (E12.5) during murine embryonic development. Park and colleaguesCitation8 showed that KDM6B activation is required for post-natal and adult neurogenesis. Our recent work shows that folate receptor FRα is responsible for downregulating miRNAs by interacting with Drosha-Pasha miRNA processing complex. If that is the case it is plausible that FRα could activate KDM6B indirectly by down-regulating the miRNAs which target KDM6B. Whether FRα directly interacts with the cis-responsive elements of KDM6B and causes its activation remains to be seen.
NOTCH
Along with the activity of the genes mentioned above, the Notch signaling pathway represents another actor in the dynamic between stem cell maintenance and differentiation. Notch signaling is mediated through cell-to-cell interactions, with the Notch receptor of one cell interacting with Notch ligands on an adjacent cell.Citation9,10 Pathway activation ultimately culminates in the release of the Notch intracellular domain (NICD), which then translocates to the nucleus to induce expression of Hes1 and related genes for stem cell maintenance.Citation9-11 Interestingly, here too it has been suggested that folic acid influences the stem cell regulation pathway, as folic acid supplementation increases Notch1 mRNA and protein expression in rat embryonic neural stem cells.Citation12,13 Additionally, we have shown that FRα also binds to the cis-regulatory elements of Hes1 geneCitation5 which is also regulated by NICD. So Hes1 regulation by folate is by 2 pathways: one by increasing Notch levels and another is by cis-acting FRα on its promoter.
Notch has been relatively well characterized for neurogenesis in development. In recent years, evidence has come forth which suggests Notch's role in stem cell regulation within the adult brain. In the adult brain neural stem cells are located in the subventricular zone of the lateral ventricle and the subgranular zone of the hippocampal dentate gyrus.Citation11 Protein expression and mRNA studies show that Notch1 and its activators are associated with cells in the subventricular zone and the dentate gyrus, indicating that notch signaling components are expressed in these regions.Citation14 In neural stem cells of the adult mouse hippocampus, it has been found that the Notch signaling pathway is highly active,Citation15 and interfering with activity of Notch1 or the transcription regulator it affects results in depletion of the adult neural stem cell pool and increased early neuronal differentiation.Citation11 A well-regulated Notch signaling system is needed for maintaining a healthy neural stem cell population in the adult brain. This signaling system also has a role in certain diseased states that may afflict the brain. For example, in the subventricular zone of ischemic rat brains one finds enhanced activity of Notch1 and its downstream transcriptional targets; this helps expand the neural stem cell pool in response to ischemia-induced damage.Citation14 On the other hand, abnormal Notch activity is implicated in the growth of the stem cell pool that results in brain cancers such as glioblastoma multiforme, anaplastic astrocytoma, medulloblastoma, and high-grade astrocytomas.Citation10 Ultimately, as in embryogenesis, there appears to be a balancing act when it comes to the neural stem cell population in adults. It will be important to know whether FRα is expressed in subventricular zone, dentate gyrus or in the hippocampus and how it affects the stem cell pool and neurogenesis in these neurogenic niches.
Role of FRα in folate delivery to the brain
With all said and done, it's important that the brain receives the right amount of folate. If the level delivered is more than optimum, there is a high probability of tumorigenesis and if less than optimum, then the stem cell pool is compromised. There is a different pathway for folate delivery especially in brain parenchyma. Grapp and colleaguesCitation16 very elegantly demonstrated that choroid plexus via transcytosis and exosome shuttling deliver folate in the brain parenchyma. According to them, 5-methyl tetra hydro folate (5-MTHF)-FRα complex is internalized by receptor-mediated endocytosis, translocated into GPI-anchored protein-enriched early endosomal compartments (GEECs) and further transferred to multi-vesicular bodies (MVBs). MVBs are late endosomal compartments localized in the endocytic route. The intra-luminal vesicles (ILVs) of MVBs containing FRα are generated by inward budding of the limiting membrane. These ILVs are released as exosomes into the cerebrospinal fluid (CSF) after fusion of the MVB with the apical cell membrane. FRα-containing exosomes circulate in the CSF, cross the ependymal cell layer and are distributed in the brain parenchyma. FRα-positive exosomes might initially be taken up by astrocytes and from these further delivered to neurons. Thus FRα is not only important for high affinity folate uptake via receptor mediated endocytosis, but also to activate genes when it behaves as a transcription factor. Its recent role is to transport folate in brain parenchyma via transcytosis and exosome shuttling ().
Figure 1. Cerebral folate transport by FRα. 5MTHF or folate binds to FRα and is taken up at the basolateral membrane of the human choroid plexus. The 5MTHF-or FA-FRα complex is internalized by receptor-mediated endocytosis. The endocytic vesicles are translocated into GPI-anchored protein-enriched early endosomal compartments (GEECs) and further transferred to MVBs. ILVs of MVBs containing FRα are generated by inward budding of the limiting membrane. These are then released as exosomes into the CSF after fusion of the MVB with the apical cell membrane. FRα-containing exosomes circulate in the CSF, cross the ependymal cell layer and are distributed in the brain parenchyma. FRα-positive exosomes then are taken up by astrocytes and neurons.
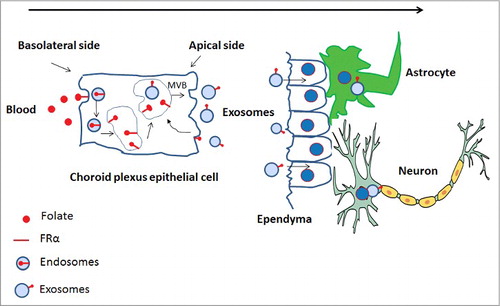
Conclusions and perspectives
A summary of the different fates of FRα in and out of the cell is described in . FRα binds to folate and undergoes endocytosis. Folate is released and the FRα is set free to act like a transcription factor, or is recycled. Another route that has been recently described is the translocation of FRα+Folate into GPI-anchored protein-enriched early endosomal compartments (GEECs) which is further transferred to multi-vesicular bodies (MVBs). MVBs are late endosomal compartments localized in the endocytic route. The intra-luminal vesicles (ILVs) of MVBs containing FRα are generated by inward budding of the limiting membrane. These ILVs are released as exosomes into the cerebrospinal fluid (CSF) after fusion of the MVB with the apical cell membrane. FRα-containing exosomes circulate in the CSF, cross the ependymal cell layer and are distributed in the brain parenchyma. FRα-positive exosomes might initially be taken up by astrocytes and from these further delivered to neurons.
Figure 2. A summary of the different fates of FRα in (endosomes) and out of the cell (exosomes). (1) One of the pathways of folate transport is by way of endosomes in which folate binds FRα and the folate is used for 1 carbon metabolism; (2) Some FRα is recycled back to plasma membrane and (3) some translocated to the nucleus where it acts as a (i) transcription factor, (ii) negative regulator of miRNA biogenesis and (iii) chromatin remodeler; (4) FRα also undergoes exocytosis in the form of exosomes and is taken up by astrocytes and neurons.
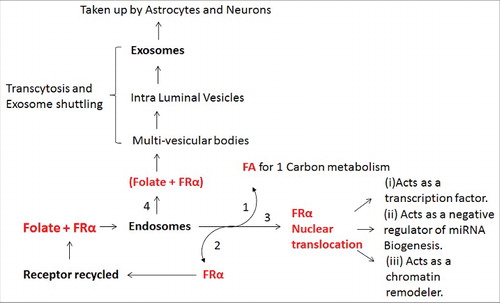
Mutations in FRα protein or autoantibodies against FRα, impairs proper high affinity folate transport inside the choroid plexus cell, causing cerebral folate deficiency (CFD). Folinic acid or 5-MTHF supplementation is suggested for treatment of CFD. Cellular metabolism of 5-MTHF depends on the route of folate entry into the cell. 5-MTHF taken up via a non-FRα –mediated process is rapidly metabolized to folylpolyglutamates, whereas 5-MTHF that accumulates via FRα remains non-metabolized, supporting the hypothesis that FRα may be part of a pathway for transcellular movement of the vitamin. Additional function of FRα as a potential transcriptional regulator of genes underscores the importance of FRα as not just a high affinity folate carrier but as a regulator of genes involved in autism spectrum disorder and cerebral folate deficiency. FRα, with high tumor specificity and overexpression in a broad range of cancers, has attracted considerable attention as a target for these various immunotherapeutic and FA conjugated nano-therapeutic modalities. Novel methods and efforts to stimulate active immunity against FRα-expressing cancer include the use of folate-localized molecules to enhance cancer immunogenicity, genetically modified autologous T cells, and techniques to raise FRα-specific immunity via viral vector, as well as multiple vaccine strategies to include modified whole tumor cells, DNA, dendritic cell and peptide vaccines.Citation17 Active immunotherapy, with the potential to not only attack tumors but also to generate long-lasting protection has the potential to add a new important therapeutic approach to the already multimodal treatment of cancer. Next major advances will see the active use of FRα dependent exosome-mediated folate or folate-drug conjugates delivery into the brain parenchyma as a mode of cerebral drug targeting, which has been prevented because of the impenetrable blood brain barrier. Selective targeting of FRa-expressing exosomes to the brain parenchyma not only substantiate the biological significance of this transport shuttle but also opens up new avenues for therapeutic approaches. By designing their protein expression, exosomes may serve as organ-specific delivery vehicle for therapeutic agents. Targeted manipulation of the choroid plexus or direct application of FRα-positive exosome like vesicles into the CSF may be a novel strategy to deliver biological active substances into the brain.Citation16
Disclosure of potential conflicts of interest
No potential conflicts of interest were disclosed.
Funding
This work was supported by the State of Illinois Excellence in Academic Medicine award a Grant from the Spastic Paralysis Research Foundation of Illinois-Eastern Iowa District of Kiwanis; the Spina Bifida Association and SMCRI Pilot Grant award; Eleanor Clarke Chair in Developmental Neurobiology (CSM); Francis Chou from Chou Mutual Funds for providing financial support for research in Neural Tube Defects.
References
- Kelemen LE. The role of folate receptor α in cancer development, progression and treatment: cause, consequence or innocent bystander? Int J Cancer 2006; 119:243-250; PMID:16453285; https://doi.org/10.1002/ijc.21712
- Kane MA, Elwood PC, Portillo RM, Antony AC, Najfeld V, Finley A, Waxman S, Kolhouse JF. Influence on immunoreactive folate-binding proteins of extracellular folate concentration in cultured human cells. J Clin Invest 1988; 81:1398-1406; PMID:3366900; https://doi.org/10.1172/JCI113469
- Matsue H, Rothberg KG, Takashima A, Kamen BA, Anderson RG, Lacey SW. Folate receptor allows cells to grow in low concentrations of 5-methyltetrahydrofolate. Proc Natl Acad Sci USA 1992;89:6006-6009; PMID:1631087
- Zhao R, Diop-Bove N, Visentin M, Goldman ID. Mechanisms of membrane transport of folates into cells and across epithelia. Annu Rev Nutr 2011; 31:177-201; PMID:21568705; https://doi.org/10.1146/annurev-nutr-072610-145133
- Boshnjaku V, Shim KW, Tsurubuchi T, Ichi S, Szany EV, Xi G, Mania-Farnell B, McLone DG, Tomita T, Mayanil CS. Nuclear localization of folate receptor alpha: a new role as a transcription factor. Sci Rep 2012; 2:980; PMID:23243496; https://doi.org/10.1038/srep00980
- Mohanty V, Shah A, Allender E, Siddiqui MR, Monick S, Ichi S, Mania-Farnell B, G McLone D, Tomita T, Mayanil CS. Folate Receptor Alpha Upregulates Oct4, Sox2 and Klf4 and Downregulates miR-138 and miR-let-7 in Cranial Neural Crest Cells. Stem Cells 2016; PMID:27300003; https://doi.org/10.1002/stem.2421
- Ichi S, Costa FF, Bischof JM, Nakazaki H, Shen YW, Boshnjaku V, Sharma S, Mania-Farnell B, McLone DG, Tomita T, et al. Folic acid remodels chromatin on Hes1 and Neurog2 promoters during caudal neural tube development. J Biol Chem 2010; 285(47):36922-32; PMID:20833714; https://doi.org/10.1074/jbc.M110.126714
- Park DH, Hong SJ, Salinas RD, Liu SJ, Sun SW, Sgualdino J, Testa G, Matzuk MM, Iwamori N, Lim DA. Activation of neuronal gene expression by the JMJD3 demethylase is required for postnatal and adult brain neurogenesis. Cell Rep 2014; 8 (5):1290-9; PMID:25176653; https://doi.org/10.1016/j.celrep.2014.07.060
- Pierfelice T, Alberi L, Gaiano N. Notch in the vertebrate nervous system: an old dog with new tricks. Neuron 69(5):840-55; PMID:21382546; https://doi.org/10.1016/j.neuron.2011.02.031
- Lasky JL, Wu H. Notch signalling, brain development and human disease. Pediatr Res 2005; 57(5 Pt 2):104R-109R; PMID:15817497; https://doi.org/10.1203/01.PDR.0000159632.70510.3D
- Imayoshi I, Kageyama R. The role of Notch signalling in adult neurogenesis. Mol Neurobiol 2011; 44(1):7-12; PMID:21541768; https://doi.org/10.1007/s12035-011-8186-0
- Liu H, Huang GW, Zhang XM, Ren DL, X Wilson J. Folic Acid supplementation stimulates notch signalling and cell proliferation in embryonic neural stem cells. J Clin Biochem Nutr 2010; 47(2):174-80; PMID:20838574; https://doi.org/10.3164/jcbn.10-47
- Zhang X, Liu H, Cong G, Tian Z, Ren D, Wilson JX, Huang G. Effects of folate on notch signalling and cell proliferation in neural stem cells of neonatal rats in vitro. J Nutr Sci Vitaminol (Tokyo) 2008; 54(5):353-6; PMID:19001765; https://doi.org/10.3177/jnsv.54.353
- Xiao MJ, Han Z, Shao B, Jin K. Notch signaling and neurogenesis in normal and stroke brain. Int J Physiol Pathophysiol Pharmacol 2009; 1(2):192-202; PMID:20428478
- Ehm O, Göritz C, Covic M, Schäffner I, Schwarz TJ, Karaca E, Kempkes B, Kremmer E, Pfrieger FW, Espinosa L, et al. RBPJkappa-dependent signaling is essential for long-term maintenance of neural stem cells in the adult hippocampus. J Neurosci 2010; 30(41):13794-807; PMID:20943920; https://doi.org/10.1523/JNEUROSCI.1567-10.2010
- Grapp M, Wrede A, Schweizer M, Hüwel S, Galla HJ, Snaidero N, Simons M, Bückers J, Low PS, Urlaub H, et al. Choroid plexus transcytosis and exosome shuttling deliver folate into brain parenchyma. Nat Commun 2013; 4:2123; PMID:23828504; https://doi.org/10.1038/ncomms3123
- Clifton GT, Sears AK, Clive KS, Holmes JP, Mittendorf EA, Ioannides CG, Ponniah S, Peoples GE. Folate receptor α: a storied past and promising future in immunotherapy. Hum Vaccin 2011; 7:183-190; PMID:21321484; https://doi.org/10.4161/hv.7.2.13784