Abstract
The hot and cold extracted virgin coconut oil (VCO) has been subjected to the various quality parameters. There was a no significant difference in iodine value, saponification value, refractive index, fatty acid profile, specific gravity, and moisture content of hot extracted virgin coconut oil (HEVCO), cold extracted virgin coconut oil (CEVCO), and copra oil (CCO) samples. The phenolic components of the HEVCO, CEVCO, and CCO were found to be 650.35 ± 25.11 μg/g, 401.23 ± 20.11 μg/g, and 182.82 ± 15.24 μg/g, respectively. The antioxidant activity ranged from 80 to 87% in HEVCO, 65 to 70% in CEVCO, and 35 to 45% in CCO. The results showed that VCO obtained from hot extraction process contained more total polyphenol, antioxidant activity, tocopherol, phytosterol, monoglycerides, and diglyceride content in comparison to CEVCO and CCO samples.
Public Interest Statement
To give a light that coconut oil extracted from natural means (without application of chemicals) contains more bioactive compounds than the commercial available coconut oil (refined coconut oil). This study was proved by quantitative and qualitative analysis of bioactive components.
Competing interests
The authors declare no competing interest.
1. Introduction
Virgin coconut oil (VCO) (oil which is obtained from fresh, mature kernel of the coconut by mechanical or natural means, with or without the use of heat and devoid of chemical refining process, i.e. refined, bleached, deodorized) is one of the emerging products in Indian oil market and is gaining lot of importance among consumers due to its medicinal and other valuable properties which is only next to virgin olive oil (Villarino, Dy, & Lizada, Citation2007). Due to increase in public awareness of health, it is expected in near future that VCO will gain a significant importance and consumption growth in the market. The demand for this oil continues to rise, which can be attributed to its superior flavor, and potential health benefits. The consumption of VCO lowered the lipid components compared to copra oil (CO) by reducing total cholesterol, triglycerides (TG), phospholipids, low density lipoprotein (LDL), and very LDL levels and increase the high-density lipoprotein in serum and tissues (Nevin & Rajamohan, Citation2004).
Animal experiments suggested that, rats fed with diets containing these coconut oils for 45 days exhibits increased levels of antioxidant enzymes (Nevin & Rajamohan, Citation2006). Among the coconut oil producing countries, India is placed third after Philippines and Indonesia (FAO, Citation2004). The USA, Canada, Europe, Asia, Australia, and South Africa are importing VCO from South Pacific countries (Muralidharan & Jayashree, Citation2011). Coconut oil has distinct yellow color, perceptible aroma, while VCO is almost colorless and has an acid flavor (Dayrit et al., Citation2007; Villarino et al., Citation2007). As per Codex Alimentarius (Citation2006) and Asian Pacific Coconut Community (APCC, Citation2006), it can be concluded that VCO has same physicochemical properties like iodine value, saponification value, moisture, and other parameters almost similar to normal coconut oil. The fatty acid compositions of both the types of oil are almost similar (Marina, Che Man, Nazimah, & Amin, Citation2009a). However, some researchers reported higher lauric acid in VCO than normal coconut oil, but this difference is not considerable, because it may vary with location, variety of crop (Laureles et al., Citation2000), age of nuts (Balleza & Sierra, Citation1976) and time of harvesting (Carandang, Citation2008). To differentiate the VCO from refined coconut oil (RCO) many methods have been used, among them P31 NMR was found to be more reliable and also it has been found that, 1-monoglycerides was higher in VCO (0.027%) than RCO (0.019%) and total sterols were more in VCO (0.096%) compared to RCO (0.032%), while diglyceride was lower in VCO (1.55%) than RCO (4.10%) (Dayrit, Buenafe, Chainani, & de Vera, Citation2008).
Still due to the lack of rapid method availability and simplicity there is needed to establish the demarcation between VCO and CCO in terms of bioactive components. The present study has been designed to compare the bioactive components in VCO extracted by cold and hot process with normal available CCO.
2. Materials and methods
2.1. Extraction of VCO
VCO can be extracted directly from the fresh coconut meat or from coconut milk because it contains high amount of polyphenol, tocopherol, phytosterol, polyphenols, monoglycerides, and radical scavenging activity. It involves two major steps: production of coconut milk and extraction of oil. Extraction of oil can be done by two ways, cold and hot extraction process.
2.2. Preparation of coconut milk
The production of coconut milk involves selection of 100 nut, dehusking (49.99 kg), splitting (41.97 kg), deshelling (25.32 kg), washing, three times pulverization/gratting (25.86 kg), and milk extraction (18L). Fully matured 10–11 months old coconut nuts were selected for VCO production. As an indicator of maturity of the nut, the husk will be yellowish to brown in color and makes a sloshing sound when shaken. By a special type of tool the shell was removed and two halves kernel was scoop out by knife. The testa of coconut kernel removed by testa remover machine. The coconut kernel free from testa was fed to the mechanical grating machine (contain rotating blades). Coconut milk was extracted from the grated coconut meat using manually operated hydraulic coconut milk press. The coconut milk obtained from the first extraction was collected separately and residue was utilized for second and third extraction. Pool the first, second, and third milk extracts by stirring vigorously for few minutes.
2.3. Preparation cold extracted VCO
In cold extraction method, coconut milk was allowed to stand for 20–24 h. Under favorable conditions (35–40°C, 75% relative humidity), the oil separated from the water and the protein. The airborne lactic acid bacteria, which has the capability to break the protein bonds, act on the coconut milk mixture causing the VCO separation. By proper operating conditions (temperature & relative humidity maintenance) and sanitary precautions (hygiene condition) were strictly followed, four distinct layers could be visible in the container after settling for 20–24 h. The bottom layer was made up of gummy sediment. The next layer was the watery, fermented skim milk that is no longer fit for human consumption. The next layer was the separated oil for recovery as VCO. The top layer was floating fermented curd. The fermented curd also contained a considerable amount of trapped oil. By carefully separating the distinct layers, the oil can be separated. The separated oil contained some adhering particles of fermented curd and it need filtration. Oil was filtered through sterilized filter paper placed in big funnel.
2.4. Preparation of hot extracted VCO
Coconut milk is an emulsion of oil and water that is stabilized by protein. To recover the oil from coconut milk, the protein bond had to be broken by heat in double-walled boiler known as VCO cooker under slow heating to coagulate the protein and release the oil. Separate the Class A oil from the protenacious residue (kalkam) by straining the mixture through a muslin cloth. The remaining kalkam can be further slow heated to remove more oil (Class B). The iodine value, saponification value, specific gravity, refractive index, moisture content, and fatty acid composition were determined by AOCS (Citation1990). The quantitative bioactive components like tocopherol (Edisbury, Gillow, & Taylor, Citation1954), phytosterol (Sabir, Hayat, & Gardezi, Citation2003), polyphenols (Singleton, Orthofer, & Lamuela-Raventós, Citation1999), monoglycerides (Hartman & Esteves, Citation1976), and radical scavenging activity (Karioti, Hadjipavlou-Litina, Mensah, Fleischer, & Skaltsa, Citation2004) were analyzed in hot extracted virgin coconut oil (HEVCO), cold extracted virgin coconut oil (CEVCO), and CCO.
3. Results and discussion
3.1. Difference in iodine value, saponification value, specific gravity, refractive index, and moisture content
Iodine value, saponification value, specific gravity refractive index, and moisture content are summarized in Table which showed no significant change in CEVCO, HEVCO, and CCO. The iodine value refers to the percentage by weight of molecular iodine, I2, absorbed by an oil or fat and is a standard procedure for determining the amount of unsaturation in an oil sample. In the present study, the iodine value obtained for the VCO samples in a range of 7.0–8.6 g I2/100g oil which is in agreement with APCC standard, but it cannot differentiate all the three types of coconut oil. The similar results were applicable for saponification value, specific gravity, refractive index and match the results obtained from VCO samples of Malaysia and Indonesia (Marina et al., Citation2009a). The saponification value is a measure of the average molecular weight of all the fatty acids present. The higher the saponification value, the shorter the fatty acids on the glycerol backbone. Fatty acid profile of the VCO as well as CCO samples showed little difference and this could be the reason for similar saponification values, specific gravity, refractive index, and moisture content of CEVCO, HEVCO, and CCO (Marina et al., Citation2009a).
Table 1. Color, refractive Index, saponification value (mg KOH/g oil), iodine value (gI2/100g oil), specific gravity, and moisture (%) of HEVCO, CEVCO, and CCO
3.2. Changes in fatty acid profile of VCO samples
The fatty acid profile of VCO is presented in Table . The fatty acid composition was as expected and comparable to the fatty composition of coconut oil according to APCC. Lauric acid was the most dominant fatty acid and ranged from 48 to 51%. In this study changes in the fatty acid composition has not been observed in CEVCO, HEVCO, and commercial coconut oil (CCO). This could be due to same geographical origin and ecological conditions. This finding is in the agreement with Banzon and Resurreccion (Citation1979) in which no changes were observed in the fatty acid distribution in samples of coconut oil obtained by heating, fermentation, freeze thawing, and solvent extraction. The similar results were also reported by Marina et al. (Citation2009a) in various VCO samples obtained from Malaysia and Indonesia.
Table 2. Fatty acid composition (%) of HEVCO, CEVCO, and CCO
3.3. Changes in polyphenol content and antioxidant activity in VCO samples
The 30 batches of VCO samples (prepared in different lot of coconut) have been analyzed for various quality parameters. The value obtained for various bioactive compounds present in CEVCO, HEVCO, and CCO tabulated in Table . The phenollic component of the HEVCO, CEVCO, and CCO were found to be 650.35 ± 25.11 μg/g, 401.23 ± 20.11 μg/g, and 182.82 ± 15.24 μg/g, respectively. The results indicate that polyphenols were higher in HEVCO than CEVCO and CCO. Higher temperatures favor the incorporation of more phenolic substances which may be the reason for higher phenolic substances in HEVCO. It has already been reported that due to application of temperature (100°C) during extraction leads to evaporation of water from coconut milk emulsion and incorporation of phenolic substances (Marina, Che Man, Nazimah, & Amin, Citation2009b; Seneviratne & Sudarshana Dissanayake, Citation2008). In the CEVCO the phenollic substances are not properly incorporated due to the mild temperature conditions. However, in the hot extraction method the temperature of the coconut milk emulsion reaches above 100°C.
Table 3. Tocopherol (μg/g), polyphenols (μg/g), antioxidant activity (%), monoglycerides (%), and phytosterol (μg/g) content in HEVCO, CEVCO, and CCO
The concentration of the phenolic substances increases when the water in the emulsion evaporates during the hot extraction process. The results were in agreement with the study reported by Seneviratne and Sudarshana Dissanayake (Citation2008) and Marina et al. (Citation2009b) in coconut oil extracted under cold and hot conditions. In order to compare the composition of phenolic substances HPLC experiments were carried out for the phenolic extracts of HEVCO, CEVCO, and CCO.The chromatograms of the phenolic extracts of CEVCO, HEVCO, and CCO are given in Figure . The concentrations of gallic acid, ferullic, epicatechin, catechin, chlorogenic, caffeic acid, homovallin, vanillic acid, rutin, p-coumaric acid, quercetin, and sinapic were higher in HEVCO than CEVCO (Table ). In CEVCO and CCO, chlorogenic, epicatechin, caffeic, homovallin, rutin, p-coumaric, and quercetin were not detected. In general, less phenolic acids were detected in CCO in comparison to CEVCO and HEVCO because some phenolic compounds were lost or degraded during the refining process. Similar, trends were also reported by Seneviratne and Sudarshana Dissanayake (Citation2008) in CEVCO as HEVCO samples. According to Caponio, Gomes, and Pasqualone (Citation2001), cultivar and ecological location affected the distribution of phenolic compounds. However, in the present study VCO was obtained from the same source. Thus, the loss of some phenolic compounds could be the different extraction methods or due to formation of insoluble complexes with other substances such as protein during extraction (Dia, Garcia, Mabesa, & Tecson, Citation2005). Earlier, Marina et al. (Citation2009b) reported that protocatechuic acid, vanillic acid, caffeic acid, syringic acid, ferulic acid, and p-coumaric acid detected in coconut oil by fermented and chilling technique. Table shows the antioxidant activity of VCO samples. The antioxidant activity ranged from 80 to 87% in HEVCO, 65 to 70% in CEVCO, and 35 to 45% in CCO. The highest antioxidant activity was observed in HEVCO in comparison to CEVCO and CCO. This difference in antioxidant activity of VCO samples may be due to the presence of phenolic components as a result of difference in processing methods. The results were comparable with the study reported by Dia et al. (Citation2005) and Marina et al. (Citation2009b) in different VCO samples.
Table 4. Concentration of phenolic acids (μg/g) in HEVCO, CEVCO, and CCO
3.4. Changes in tocopherols in VCO samples
Very low amount of the tocopherol obtained in all CCO samples in comparison to VCO samples. Amoung VCO samples CEVCO contained more tocopherol than HEVCO. There was no literature available for the amount of tocopherol in VCO samples, whereas few literatures is available on the tocopherol content in RCO, i.e. 7 μg/g (Lambertsen, Myklestad, & Brækan, Citation1962). The reason may be that tocopherol is actually found in the coconut testa (the thin brown layer that clings to the white coconut meat) and since coconut testa was removed in the production of VCO, it was logical that the VCO samples contain only trace amount in tocopherol (Marina et al., Citation2009a).
3.5. Changes in phytosterol, monoglycerides, and diglyceride content in VCO samples
Monoglycerides exists in certain oils mainly as a result of enzymatic splitting of triglycerides. In the present study, the monoglycerides in VCO samples were more than the CCO samples. Among VCO samples, CEVCO contained more monoglycerides than HEVCO and CCO (Table ). Plant oils are concentrated source of phytosterol which compete with the absorption of cholesterol in the body and thus has the ability to lower down the body cholesterol. In the present study, both the VCO samples contained high phytosterol content in comparison to CCO. Our findings from this study supports previous work by Sabir et al. (Citation2003), i.e. 0.08 mg/g and Okpuzor et al. (Citation2009) which showed that phytosterol content 1.64 mg/g in coconut oil.
3.6. Quantitative determination of the diglycerides and sterol amount in HEVCO, CEVCO, and CO samples
3.6.1. Optimization of phosphitylation reaction
The reagent 2-cholro-4,4,5,5 tetramethyldioxa phospholane (2-CITMDOP) has been shown to react quickly and quantitatively with hydroxyl functional groups (Figure .). The derivatives of 2-CITMDOP generate simple phosphorus-31 NMR spectrum due to the absence of protons within three bonds of the phosphorus atom of the reagent moiety. The alcohols containing one chiral center (viz. pinacolyl alcohol) generate a single NMR signal due to formation of enantiomeric products. Chemical shifts and 3JP-H values are very characteristic and the signals are well-dispersed and appear at values > 130 ppm. Therefore, they do not interfere with the analysis of other organophosphorus compounds. Presence of four methyl groups of the derivatives significantly reduces spin–lattice relaxation times of the phosphorus nuclei. Therefore, 2-CITMDOP was selected from the various reagents reported in the literature for the phosphitylation of the hydroxyl functions. The study was based on the derivatization of the labile hydrogens of hydroxyl groups of the DG with 2-CITMDOP according to the reaction shown in Figures and . The 2-CITMDOP reacts rapidly and quatitatively under mild conditions with hydroxyl groups. It should be noted that reagent 2-CITMDOP also reacts quantitavely with other functional groups such as carboxylic and aldehydic hydrogens according to the reaction.
3.6.2. Quantification of 1-monoglycerides, 1,2-diglycerides, 1,3-diglycerides, and sterols in CEVCO, HEVCO, and CO
The plant seed oils contain triglycerides which account for the majority of the weight of the oil, while the minor constituents include monoglycerides (MGs), diglycerides (DGs), and sterols. The presence of these minor constituents may vary depending on the processing, storage, and age of the oil (Dayrit et al., Citation2008). Diglycerides (DGs), minor constituent of VCO, found in the form of 1,2-diglycerides (1,2-DGs) and 1,3-diglycerides (1,3-DGs). Generally, 1,2-DGs arise from the incomplete biosynthesis of triglycerides or by enzymatic hydrolysis (lipolysis) of TGs, while 1, 3-DGs form by isomerization of 1,2-DGs during extraction process. It may provide an idea to discriminate the quality of oils prepared by different processing methods; so, it is considered as the best index to check the quality of oils (Hitchcock & Nichols, Citation1971; Pérez-Camino, Moreda, & Cert, Citation2001). The present investigation has been designed for the qualitative and quantitative differentiation among CCO, CEVCO, and HEVCO by 31P NMR spectroscopy on the basis of their chemical shifts and coupling constants using coconut oil blank matrix. Using the NMR experimental conditions, phospholane derivatives of 1-monoglycerides, 1,2-diglycerides, 1,3-diglycerides, and sterols were synthesized individually with 2-CITMDOP for the experiments.
The quantitative analysis of all the compounds was performed by primary ratio method and system stability for the quantitative analysis was also checked by determining specific detector response factors of the phosphitylated derivatives of 1-monoglycerides, 1,2-diglycerides, 1,3-diglycerides, and sterols at different concentrations. The four-point calibration curves of 1-monoglycerides (0.49–3.89 mg/ml), 1, 2-diglycerides (0.47–3.75 mg/ml), 1,3-diglycerides (0.67–5.35 mg/ml), and sterols (0.75–6.03 mg/ml) were plotted against benzoic acid as internal standard from freshly phosphitylated solution. The calibration curves were found to have excellent linearity with regression coefficient (R2) ≥ 0.99. Straight calibration curves representing a direct linear relationship between analytical response and standard concentrations were obtained (Figure ). A typical 31P NMR spectrum of 1-monoglycerides, 1,2-diglycerides, 1,3-diglycerides, and sterols standard shown in Figure , while Figures depicted spectrum of CEVCO, HEVCO, and CCO. The peak at δ 136.348 belongs to the internal standard (benzoic acid), while at δ 175.00 related to 2-CITMDOP which ensure complete phosphitylization. 31P chemical shifts of the phosphorylated derivatives of 1-monoglycerides, 1,2-diglycerides, 1,3-diglycerides, and sterols were 148.82; 147.34, 149.274, 147.578, and 145.765 mg/ml, respectively. The results are in agreement with the study reported by Dayrit et al. (Citation2008) in VCO. The excellent resolution between the 31P chemical shifts of the mono and diglycerides permits a reliable quantification of these compounds in CEVCO, HEVCO, and CCO. The quantitative 31P NMR data of 1-monoglycerides, 1,2-diglycerides, 1,3-diglycerides, and sterols in CEVCO, HEVCO, and CCO are tabulated in Table . The results revealed that 1, 2 DG content was more in CEVCO, HEVCO, than CCO. The sterol content was found to be higher in HEVCO than CEVCO and CCO. The obtained value was less in comparison to the data reported by Vigli, Philippidis, Spyros, and Dais (Citation2003) and Dayrit et al. (Citation2008) in coconut oil. This may due to the varietal and ecological changes in coconut.
Figure 4. Reaction of hydroxyl groups of DG with 2-chloro4,4,5,5-tetramethyl-1,3-dioxa-2-phospholane (2-CITMDOP) & Structures of different phosphitylated mono and diesters of glycerol.
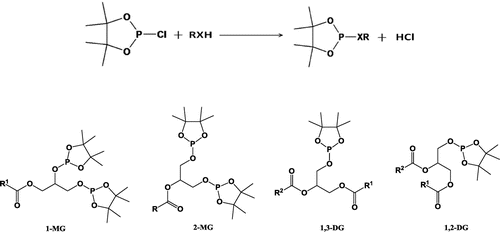
Figure 5. A typical 31P NMR spectrum of standard 1-monoglycerides (1-MG), 1, 2-diglycerides (1, 2-DGs), 1, 3-diglycerides (1, 3-DGs), and sterols.
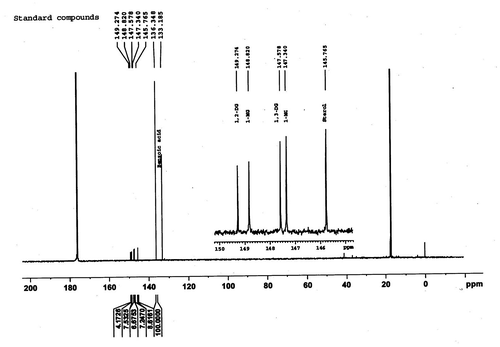
Figure 6. A typical 31P NMR spectrum of HEVCO showing 1-monoglycerides (1-MG), 1, 2-diglycerides (1, 2-DGs), 1, 3-diglycerides (1, 3-DGs), and sterols.
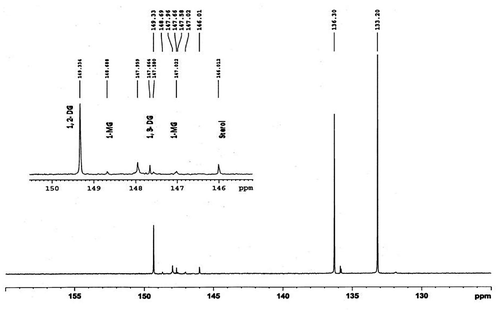
Figure 7. A typical 31P NMR spectrum of CEVCO showing 1-monoglycerides (1-MG), 1, 2-diglycerides (1, 2-DGs), 1, 3-diglycerides (1, 3-DGS), and sterols.
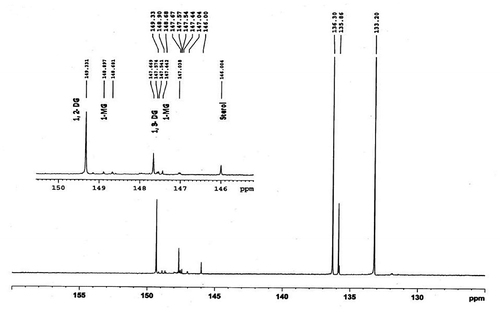
Figure 8. A typical 31P NMR spectrum of CCO showing 1-monoglycerides (1-MG, 1, 2-diglycerides (1, 2-DGs), 1, 3-diglycerides (1, 3-DGS), and sterols.
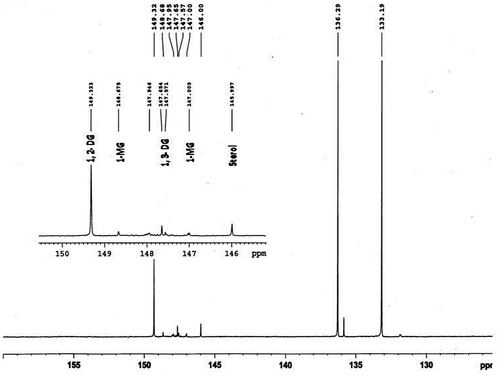
Table 5. Concentration of 1-monoglycerides (mg/ml), 1, 2-diglycerides (mg/ml), 1, 3-diglycerides (mg/ml), and sterols (mg/ml) in HEVCO, CEVCO, and CCO by 31P NMR
4. Conclusion
Among the two VCO (hot and cold extraction process) and CCO samples, there was a no significant difference in iodine value, saponification value, refractive index, fatty acid profile, specific gravity, and moisture content. Further total polyphenol, antioxidant activity, tocopherol, phytosterol, monoglycerides, and diglyceride content in both HEVCO and CEVCO samples are significantly higher than CCO samples. In comparison to both the oils HEVCO found to be better in terms of polyphenol, phytosterol, monoglycerides, and diglyceride. The higher amount of polyphenol, phytosterol, monoglycerides, and diglyceride content gives a significant medicinal value in terms of diabetes and hyperlipidemia. Therefore, VCO has a wide future scope as functional oil. It can be incorporated in various products for fortification.
Abbreviations | ||
HEVCO | = | hot extracted virgin coconut oil |
CEVCO | = | cold extracted virgin coconut oil |
CCO | = | copra oil |
Acknowledgments
Authors express their gratitude to the National Agricultural Innovation Project (NAIP) and Director, Central Plantation Crops Research Institute (CPCRI), Kasargod, Kerala for providing the VCO which is required for the study.
Additional information
Funding
Notes on contributors
Yashi Srivastava
Yashi Srivastava has completed doctorate from Defence Food Research Laboratory, Ministry of Defence, Mysore, India. She has many high-quality peer-reviewed publications in National and International journals. Yashi Srivastava got Best Researcher Award 2012, Technology group Award in 2012 from Coconut Development Board & Ministry of Defence, respectively, for providing various new product development technologies from the byproducts to small-scale industry. She has also authored many research articles, book chapters, short communication, and review articles. She got fellowship from the renowned government scientific bodies like APEDA and ICAR. She is a member of Association of Food Science and Technology Institution (AFSTi), India. Her candidature is having capacity of editor-in-chief, associate editor, member editor.
References
- AOCS. (1990). Official methods of analysis (15th ed.). Gaithersburg, MD: Association of official Analytical Chemists. Retrieved from http://www.eoma.aoac.org/
- Asia Pacific Coconut Community. (2006). Retrieved November 2, 2006, from http://www.apccsec.org/standard.htm
- Balleza, C. F., & Sierra, Z. N. (1976). Proximate analysis of the coconut endosperm in progressive stages of development. Philippines Journal of Crop Science, 1, 37–44.
- Banzon, J. A., & Resurreccion, A. P. (1979). Fatty acid distribution in coconut oil obtained by four processing methods and secured from four Philippine types of coconut. Philippines Journal of Coconut Studies, 4, 1–8.
- Caponio, F., Gomes, T., & Pasqualone, A. (2001). Phenolic compounds in virgin olive oils: Influence of the degree of olive ripeness on organoleptic characteristics and shelf life. European Food Research Technology, 212, 329–333.10.1007/s002170000268
- Carandang, E. V. (2008). Health benefits of virgin coconut oil. Retrieved from www.pcrdf.org/artimages%5CVCO.doc
- Codex Alimentarius. (2006). Codex standard for named vegetable oils. Retrieved from http://www.codexalimentarius,net/web/indexen.jsp
- Dayrit, F., Buenafe, O. E., Chainani, E., de Vera, I. M., Dimzon, I. K., Gonzales, E., & Santos, J. E. (2007). Standards for essential composition and quality factors of commercial virgin coconut oil and its differentiation from RBD coconut oil and copra oil. Philippines Journal Science, 136, 121–131.
- Dayrit, F. M., Buenafe, O. E. M., Chainani, E. T., & de Vera, I. M. S. (2008). Analysis of monoglycerides, diglycerides, sterols, and free fatty acids in coconut (Cocos nucifera L.) oil by 31P NMR spectroscopy. Journal of Agricultural and Food Chemistry, 56, 5765–5769.10.1021/jf8005432
- Dia, V. P., Garcia, V. V., Mabesa, R. C., & Tecson, M. E. M. (2005). Comparative physicochemical characteristics of virgin coconut oil produced by different methods. Philippines Agriculture Science, 88, 462–475.
- Edisbury, J. R., Gillow,J., & Taylor,R. J. (1954). The determination of total tocopherol. The Analyst, 79, 617–623.10.1039/an9547900617
- Food and Agriculture Organization of United Nations (FAO). (2004). Economic and Social Department: The Statistical Division. Retrieved from FAOSTAT data base: faostat.fao.org
- Hartman, L., & Esteves, W. (1976). Determination of monoglycerides in crude oils and fats. Journal of the American Oil Chemists’ Society, 53, 584–585.10.1007/BF02586314
- Hitchcock, C., & Nichols, B. W. (1971). The lipid and fatty acid composition of specific tissues. In C. Hitchcock & B. W. Nichols (Eds.), Plant lipid biochemistry (Experimental Botany Monographs, 387 p.). New York, NY: Academic Press.
- Karioti, A., Hadjipavlou-Litina, D., Mensah, M. L., Fleischer, T. C., & Skaltsa, H. (2004). Composition and antioxidant activity of the essential oils of Xylopia aethiopica (Dun) A. Rich. (Annonaceae) leaves, stem bark, root bark, and fresh and dried fruits, growing in Ghana. Journal of Agricultural and Food Chemistry, 52, 8094–8098.
- Lambertsen, G., Myklestad, H., & Brækan, O. R. (1962). Tocopherols in nuts. Journal of the Science of Food and Agriculture, 13, 617–620.10.1002/(ISSN)1097-0010
- Laureles, L. R., Rodriguez, M. A. A., Caraos, C. E., Reano, G. A., Santos, L. A. C., & Mendoza, E. M. T. (2000). Storage lipid variability in promising coconut cultivars and hybrids: Fatty acids and triacylglycerol composition. Philippines Journal of Crop Science, 25, 42–54.
- Marina, A. M., Che Man, Y. B., Nazimah, S. A. H., & Amin, I. (2009a). Chemical properties of virgin coconut oil. Journal of the American Oil Chemists’ Society, 86, 301–307.10.1007/s11746-009-1351-1
- Marina, A. M., Che Man, Y. B., Nazimah, S. A. H., & Amin, I. (2009b). Antioxidant capacity and phenolic acids of virgin coconut oil. International Journal of Food Sciences and Nutrition, 60, 114–123.10.1080/09637480802549127
- Muralidharan, K., & Jayashree, A. (2011). Value addition, product diversification and by-product utilization in coconut. Indian Coconut Journal, 7, 4–10.
- Nevin, K. G., & Rajamohan, T. (2004). Beneficial effects of virgin coconut oil on lipid parameters and in vitro LDL oxidation. Clinical Biochemistry, 37, 830–835.10.1016/j.clinbiochem.2004.04.010
- Nevin, K. G., & Rajamohan, T. (2006). Virgin coconut oil supplemented diet increases the antioxidant status in rats. Food Chemistry, 99, 260–266.10.1016/j.foodchem.2005.06.056
- Okpuzor, J., Okochi, V. I., Ogbunugafor, H. A., Ogbonnia, S., Fagbayi, T., & Obidiegwu, C. (2009). Estimation of cholesterol level in different brands of vegetable oils. Pakistan Journal of Nutrition, 8, 57–62.10.3923/pjn.2009.57.62
- Pérez-Camino, M. C., Moreda, W., & Cert, A. (2001). Effects of olive fruit quality and oil storage practices on the diacylglycerol content of virgin olive oils. Journal of Agricultural and Food Chemistry, 49, 699–704.10.1021/jf001064w
- Sabir, S. M., Hayat, I. H., & Gardezi, S. D. A. (2003). Estimation of sterols in edible fats and oils. Pakistan Journal of Nutrition, 2, 178–181.
- Seneviratne, K. N., & Sudarshana Dissanayake, D. M. (2008). Variation of phenolic content in coconut oil extracted by two conventional methods. International Journal of Food Science and Technology, 43, 597–602.10.1111/ifs.2008.43.issue-4
- Singleton, V. L., Orthofer, R., & Lamuela-Raventós, R. M. (1999). Analysis of total phenols and other oxidation substrates and antioxidants by means of Folin–Ciocalteau reagent. Methods Enzymology, 299, 152–178.10.1016/S0076-6879(99)99017-1
- Vigli, G., Philippidis, A., Spyros, A., & Dais, P. (2003). Classification of edible oils by employing 31P and 1H NMR spectroscopy in combination with multivariate statistical analysis. A proposal for the detection of seed oil adulteration in virgin olive oils. Journal of Agricultural and Food Chemistry, 51, 5715–5722.10.1021/jf030100z
- Villarino, B. J., Dy, L. M., & Lizada, Ma. Concepćion C. (2007). Descriptive sensory evaluation of virgin coconut oil and refined bleached and deodorized coconut oil. LWT-Food Science and Technology, 40, 193–199.10.1016/j.lwt.2005.11.007