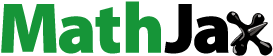
Abstract
The concentration of copper in soil is increasing, which may potentially affect the crop yield. Brassinosteroids are well known to enhance tolerance towards abiotic stress, but role of castasterone in this context is poorly understood. The present study was designed to explore the potential of castasterone to enhance copper tolerance in Brassica juncea plants. Results indicate that copper increased the production of superoxide anion radical and hydrogen peroxide, maximum at 0.75 mM of copper exposure (31.71 and 68.29% at 60 days). This overproduction of reactive oxygen species hampered the photosynthetic pigments and gas exchange parameters. Application of castasterone as seed soaking method significantly activated the enzymatic defense system. Superoxide dismutase, polyphenol oxidase and catalase showed maximum enhancement in the activities. The study further highlighted the modulations of polyphenols in B. juncea with castasterone and copper. Phenolic profiling shows that accumulation of polyphenols increase with the castasterone application under copper stress. Caffeic acid, ellagic acid, catechin and chlorogenic acid were the most prominent polyphenols observed in this study.
Public Interest Statement
In the era of globalization and industrialization, plants are exposed to various abiotic stresses including the heavy metal stress. These stresses adversely affect the growth of plants, thus negatively affects the crop productivity. The crop failure due to heavy metals is very well documented worldwide. Brassica juncea is grown as an oil seed over the globe and accounts for major edible oil production after soy and palm. Being grown over 15% arable land in India, its yield is significantly hampered by numerous biotic and abiotic stresses. Thus, it is imperative to evaluate the response and mechanism of Indian mustard against Cu stress.
Competing Interests
The authors declare no competing interest.
1. Introduction
Copper (Cu) is a vital micronutrient for the plants as it plays role in various metabolic processes like photosynthesis, respiratory electron transport chain etc. It promotes production and formation of seeds, thus affecting quality and quantity of yields (Vamerali, Bandiera, & Mosca, Citation2010). It is also a major constituent of various enzymes like phenolases, ascorbic acid oxidase, polyphenol oxidase, amine oxidase, Cu/Zn superoxide dismutase etc. Cu also plays role in chlorophyll synthesis. However, excessive Cu is harmful for the proper growth and development of plants. The Cu is potentially more toxic than the Mn, Zn and Cd, however it shows more toxicity towards plants than the animals and humans (Dresler, Hanaka, Bednarek, & Maksymiec, Citation2014; Metwali, Gowayed, Al-Maghrabi, & Mosleh, Citation2013). But presence of higher Cu content in growing medium may affect food safety and pose a threat to human health (Kabata-Pendias & Pendias, Citation2001). The toxicity of Cu can be perceived by the decreased yield, reduced germination rate, underdeveloped leaf and roots and changes in ultrastructural and anatomical levels causing production of free radicals prominently reactive oxygen species (ROS). To prevent cells from oxidative damage produced by these free radicals plants have antioxidant mechanism which is comprised of enzymatic antioxidants (catalase, peroxidase, superoxide dismutase etc.) and non-enzymatic antioxidants like ascorbic acid, glutathione etc. (Azooz, Abou-Elhamd, & Al-Fredan, Citation2012; Ivanova, Kholodova, & Kuznetsov, Citation2010). Presence of excessive Cu also leads to reduced photosynthesis. Reduced pigment content and structural damages to the photosynthetic machinery are directly related to the reduced photosynthesis (Kabata-Pendias & Pendias, Citation2001). Various reports are available which shows the inhibitory effect of Cu on photosystem I and II while the PS II is reported to be more sensitive towards Cu toxicity (Mishra & Dubey, Citation2005; Yruela, Citation2009).
Role of various plant growth regulators has been studied widely to observe their mitigating effect towards soil metal toxicity. Out of these plant growth regulators, brassinosteroids, a novel kind of phytohormones showed a significant involvement in developmental and physiological processes along with enhancement in the tolerance against various abiotic stresses (Gruszka, Citation2013; Kaur, Yadav, Thukral, Walia, & Bhardwaj, Citation2016; Li, Chen, Zhou, Xia, & Shi, Citation2013). Castasterone (CS) is among C-28 group of BRs which was first isolated in 1982 by Yakota et al. from the insect galls of chestnut. It showed strong biological activity and plays an important role in growth and development of plants (Kim et al., Citation2004). BRs are being studied for their role in stress management (Clouse, Citation2011; Ye et al., Citation2010) but the role of CS in this direction is yet to be explored.
Brassica juncea (B. juncea) is grown widely in India as an oil seed crop. B. juncea and is known as hyper accumulator of various heavy metals (Kanwar, Poonam, & Bhardwaj, Citation2015; Kanwar, Poonam, Pal, & Bhardwaj, Citation2015). The present study was formulated to establish relationship between Cu induced toxicity and antioxidant system in B. juncea plants.
2. Materials and methods
2.1. Plant material and growth conditions
Certified seeds of B. juncea variety RLC1 were obtained from Punjab Agriculture University (Punjab) India. Surface of seeds was sterilized by washed with 0.5% sodium hypochlorite followed by rinsing with double distilled water. These seeds were soaked in different concentrations of CS (0, 10−11, 10−9 and 10−7 M) for 8 h. The garden soil was prepared by mixing soil and organic manure (3:1) and pots of same size (10 × 12 inches) were filled with 5 kg of soil each pot. The soil was amended with aqueous solution of different concentrations of Cu (0, 0.25, 0.50 and 0.75 mM) in the form of CuSO4·5H2O. The pots were placed according to randomized block design with five replicates each in the Botanical garden of Guru Nanak Dev University, Amritsar, Punjab, India under natural climatic conditions with continuous water supply. Plants were harvested after 60 and 90 days after sowing and used for analysis of various parameters.
2.2. Oxidative stress studies
2.2.1. Superoxide anion radical assay
The superoxide anion content was determined following the method of Wu et al. (Citation2010) with some modifications. The absorbance was measured at 530 nm and superoxide anion content was calculated from standard curve prepared using NaNO2.
2.2.2. Localization of superoxide anion content
The analysis of superoxide anion content was done on 60 and 90 DAS old plants of B. juncea using the NBT staining method followed by imaging with Nikon digital camera. For imaging of the , the method suggested by Jabs, Dietrich, and Dangl (Citation1996) with slight modifications was used. Leaves of B. juncea were vacuum infiltrated with sodium azide solution for 2 min. Then the leaves were vacuum infiltrated with NBT for 2 min and incubated for 2 h in dark. Leaves were decolorized for imaging using solution of boiling glycerol, acetic acid and ethanol (1:1:3). The imaging of leaves was done using Nikon digital camera.
2.2.3. Hydrogen peroxide estimation
The hydrogen peroxide content was estimated using method proposed by Jana and Choudhuri (Citation1981). H2O2 content was calculated using extinction coefficient 0.28 μmol−1 cm−1.
2.2.3.1. Chlorophyll content
Chlorophylls were extracted by homogenizing plant samples in 80% acetone and incubated for 24 h. The supernatant was collected and absorbance was measured at 643 and 663 nm. Total chlorophyll, chlorophyll a and chlorophyll b were calculated using equations given by Arnon (Citation1949).
2.2.3.2. Total carotenoids content
Carotenoids were extracted by homogenizing plant samples in 80% acetone and incubated for 24 h. The supernatant was collected after centrifugation and absorbance of supernatant was measured at 480 and 510 nm. Total carotenoids content was calculated using the equation provided by Maclachlan and Zalik (Citation1963).
2.2.3.3. Anthocyanins content
Fresh plant tissue was homogenized in acidified methanol and incubated for 24 h. The homogenate obtained was centrifuged and supernatant was used to record absorbance at 530 and 657 nm. Total anthocyanin content was calculated according to Mancinelli (Citation1984).
2.2.3.4. Gas exchange parameters
Various gas exchange parameters like transpiration rate, internal CO2 concentration, stomatal conductance and net photosynthetic rate was measured at selected stages of plants using portable infra-red gas analyzer (IRGA LI-COR-6400XT, Lincoln, NE, USA). Second fully expanded leaf of plant was selected in each concentration. The relative humidity, CO2 concentration, air temperature and photosynthetic photon flux density were maintained at 85%, 600 μmol−1, 25°C and 800 μmol mol−2s−1 respectively. The measurements were taken during 10–12 h under clear sun light.
2.2.3.5. Protein content
Protein content was estimated using the method given by Bradford (Citation1976). Total soluble protein content was calculated from the graph plotted using standard solution of bovine serum albumin (BSA).
2.2.3.6. Catalase (CAT, EC 1.11.1.6)
CAT activity was measured using the method provided by Aebi (Citation1984). Reaction mixture contained phosphate buffer (50 mM, pH 7.0), H2O2 (15 mM) and 100 μl plant extract. The decrease in absorbance was measured at 240 nm and catalase activity was calculated from the equation using extinction coefficient 39.4 mM−1 cm−1.
2.2.3.7. Guaiacol peroxidase (POD, EC 1.11.1.7)
POD activity was measured following the method of Sànchez (1995). Reaction mixture prepared contained 50 mM phosphate buffer (50 mM, pH 7.0), H2O2 (12.3 mM), guaiacol solution (20 mM) and 100 μl plants extract. The increase in absorbance was measured at 436 nm and enzyme activity was determined using extinction coefficient 26.6 mM−1 cm−1.
2.2.3.8. Ascorbate peroxidase (APOX, EC 1.11.1.11)
APOX activity was estimated according to the method provided by Nakano and Asada (Citation1981). Reaction mixture was prepared containing phosphate buffer (50 mM, pH 7.0), H2O2 (1.0 mM), ascorbate (0.5 mM) and 100 μl enzyme extract. The decrease in absorbance was measured at 290 nm. The extinction coefficient 2.8 mM−1 cm−1 was used to calculate activity of APOX.
2.2.3.9. Superoxide dismutase (SOD, EC 1.15.1.1)
SOD activity was measured using the method given by Kono (Citation1978). Reaction mixture contained sodium carbonate buffer (50 mM, pH 10.2), EDTA (0.1 mM), hydroxylamine hydrochloride (1 mM), NBT (24 μM), Triton X-100 (0.03%) and 100 μl enzyme extract. The increase in absorbance was measured at 560 nm for 2 min. The amount of enzyme required to cause 50% of NBT reduction is defined as one unit of enzyme.
2.2.3.10. Polyphenol oxidase (PPO, EC 1.10.3.1)
PPO was measured by using the method provided by Kumar and Khan (Citation1982). Reaction mixture containing 1 ml of phosphate buffer (100 mM, pH 6.0), 0.5 ml of 100 mM catechol and 0.25 ml enzyme extract was incubated for 2 min. Reaction was stopped by adding 1 ml of 1.25 M H2SO4. The increase in absorbance was recorded at 495 nm.
2.2.3.11. Quantitative estimation of sugars
Total soluble sugar content was analyzed using Anthrone method. Total soluble sugar content was calculated from the standard curve prepared using the standard solution of glucose.
2.2.3.12. Quantitative estimation of phenols
Total phenols content was estimated using the method of Singleton and Rossi (Citation1965). Total phenolic content was calculated from standard curve prepared by using gallic acid as standard.
2.2.3.13. Qualitative estimation of phenols
Qualitative determination of phenolic compounds was done using UHPLC (ultra-high performance liquid chromatography). The extracts were prepared by homogenizing plant samples in methanol. The extracts were allowed to incubate for 24 h at 4°C. Then the homogenate was centrifuged at 13,000 rpm for 10 min at 4°C. The supernatant was collected and filtered through micro filters. About 10 μL sample was injected in the UHPLC (Shimadzu Nexera system, USA) coupled with photodiode array detector. The sample was run under conditions 25°C temperature with flow rate of 1 ml/min at 280 nm and C18 column (150 mm × 4.6 mm) with pore size of 5 μM. Two solvents, 0.01% acetic acid in water as solvent A and methanol as solvent B were used. Analysis of peaks was done using software provided with system. Standards of various polyphenols (gallic acid, epicatechin, caffeic acid, coumaric acid, ellagic acid, quercetin and kaempferol) were run to obtain calibration curves. Each compound was detected based on combination of retention time and spectral similarity.
2.2.3.14. Total flavonoids content
Flavonoids content were estimated using the method of Zhishen, Mengcheng, and Jianming (Citation1999). Rutin was used to draw standard curve for the calculation of flavonoid content.
2.2.3.15. Statistical analysis
The data was subjected to two-way analysis of variance (ANOVA) for scrutinizing the effect of Cu and CS on various biochemical parameters and expressed as the mean ± standard error of three replicates. Tukey’s HSD post hoc test (p ≤ 0.05) was applied for the comparisons against control values.
3. Results
3.1. Superoxide anion content
The treatment of Cu (0.25, 0.50 and 0.75 mM) enhanced the superoxide anion content in plants of B. juncea (Table ). In 60 days old plants, maximum superoxide anion content was observed in plants grown in 0.75 mM of Cu concentration. The treatment of seeds with CS reduced the superoxide anion content. The superoxide anion content was reduced by 11.32% under 0.25 mM Cu with treatment in 10−7 M of CS at 60 days stage.
Table 1. Effect of Cu and castasterone on ROS indicators (superoxide anion and hydrogen peroxide content), photosynthetic pigments (chlorophyll a, b, total chlorophyll and carotenoids content) and anthocyanin content in 60 days old B. juncea plants
3.2. H2O2 content
The data presented in Table show that H2O2 content increased with the increasing Cu concentration. Maximum H2O2 content was observed in the plants grown in soil treated with 0.75 mM concentration of metal (68.29% increase) as compared to control plants. The application of CS as seed soaking lowered the H2O2 content in metal treated plants. In lower concentration of metal treated plants (0.25 and 0.50 mM), 10−7 M CS has completely neutralized the toxicity while in 0.75 mM metal treated plants 10−11 M CS was more effective in neutralization of H2O2 content.
3.3. Superoxide anion radical staining
NBT staining for superoxide anion content has supported the quantitative results. The leaves of 60 days old plants were stained with NBT for visualization of production of superoxide anion radical. The production of superoxide anion radical was increased. It was also enhanced with Cu toxicity as compared to control. It has been observed that in plants grown under Cu stress (0.50 mM), the production of superoxide anion radical was maximum which is shown by increase in the intensity of blue color produced as compared to control untreated plants (Figure ). The CS treatment did not induce production of superoxide anion radical. The application of CS as seed soaking method (10−7 M) with Cu (0.50 mM) decreased the accumulation of superoxide anion content in leaves, which leads to decrease in the blue formagen produced in leaves of B. juncea plants (Figure ).
3.4. Photosynthetic pigments
Application of Cu (0.25, 0.50 or 0.75 mM) through soil amendment significantly decreased chlorophyll a, b and total chlorophyll content. In 60 days old plants more decrease in chlorophyll a was noticed than chlorophyll b. Maximum decline (27.99, 21.39 and 28.16% in chlorophyll a, b and total chlorophyll content respectively over control plants) in plants was observed in highest Cu concentration (Table ). The pre-sowing treatment of seeds with CS induced the production of chlorophylls under various metal toxic concentrations. Maximum increase in chlorophyll a, b and total chlorophyll content (18.84, 15.3 and 17.46% respectively) was recorded in 10−7 M CS treated seeds grown under 0.50 mM Cu amended soil. Increase in the carotenoids content was recorded under Cu stress. The maximum increase by 48.93% was recorded in plants grown under 0.25 mM Cu toxicity. With increasing metal concentration, decrease in carotenoids was observed. The CS application as pre-sowing seed treatment has partially improved the carotenoids content. In case of 60 days old plants, 10−7 M CS was effective on all the three metal concentrations. The maximum increase in carotenoids content (13.23%) was recorded in plants grown in 0.50 mM of Cu toxicity and treated with 10−7 M CS. Table clearly shows that anthocyanin content was significantly increased both under metal toxicity as well on application of CS. The Cu toxicity has enhanced the pigment content in dose treatment manner in which increasing dose of metal increased pigment content. However, plants grown from seeds treated with various concentrations of CS (10−11, 10−9 and 10−7 M) further increased the anthocyanin content (Table ). Maximum increase (91.78%) was observed in plants grown from 10−7 M CS seed treatment and raised under 0.75 mM Cu toxicity.
3.5. Gas exchange parameters
The increasing concentration of Cu (0.25, 0.50 and 0.75 mM) in soils leads to the decrease in the photosynthetic parameters like intercellular CO2 concentration, photosynthetic rate, transpiration rate and stomatal conductance in the plants (Figure ). Maximum decrease in intercellular CO2 concentration values (22.82%) was observed in 0.75 mM Cu treated B. juncea plants in 60 days old plants. Similarly, net photosynthetic rate (26.19%), stomatal conductance (40.10%) and transpiration rate (30.08%) were lowest in 0.75 mM Cu treated plants. Seed soaking treatment with different concentrations of CS enhanced the photosynthetic parameters as compared to control in 60 days old B. juncea plants. Maximum increase in intercellular CO2 concentration (19.64%) was recorded in 0.75 mM of Cu toxicity and plants grown from 10−7 M CS treatment.
3.6. Activities of antioxidative enzymes
Cu toxicity significantly enhanced the activities of antioxidative enzymes in 60 days old B. juncea plants. Activities of all the enzymes CAT, POD, SOD, APX, and PPO were increased with the increasing concentration of Cu as compared to control plants. Maximum activity of CAT was observed in 0.75 mM Cu treated plants (108.46%). Similarly, activities of POD (110.05%), SOD (121.92%), APOX (39.92%) and PPO (86.27%) were recorded maximum in 0.75 mM Cu treated B. juncea plants. The application of CS as pre-sowing seed treatment led to the enhancement in the activities of antioxidative enzymes (Table ).
Table 2. Effect of Cu and castasterone on specific activities of enzymes (CAT, POD, SOD, APOX, DHAR and PPO) in 60 days old B. juncea plants
3.7. Protein content
The plants grown in Cu amended soil possessed significantly lowered protein content than their respective controls plants (Figure ). In 60 days old B. juncea plants, the protein content was reduced under metal toxicity and lowest protein content (17.77% less compared to control plants) was recorded in 0.75 mM of Cu. The soaking of seeds in various levels of CS (10−11, 10−9 and 10−7 M) has improved the toxic effects of Cu and stabilized the protein content. Maximum improvement was noted in 10−7 M CS under various concentrations of metal (0.25, 0.50 and 0.75 mM of Cu) over their control alone metal treated plants.
3.8. Osmoprotectants
Osmoprotectants like sugar, total phenols and flavonoids content increased with Cu toxicity as well with CS treatment in the present study. In 60 days old plants, total sugar content increased with metal content maximum being at the 0.75 mM of Cu. 10−7 M CS treatment was found to be most effective in increasing total sugar content with various metal treatments. It has approx. increased the sugar content by 15.66% under 0.25 mM of Cu treatments. Maximum increase in phenolic content in the leaves of 60 days old plant was observed in the plants grown from seeds soaked in 10−7 M CS along with various levels of metal. Approximately 11.64% increase was observed in 10−7 M CS raised under 0.25 mM of Cu at 60 days stage. Maximum increase in flavonoids content at 60 days old plants was observed in combination of 10−7 M of CS with 0.50 mM of Cu with respect to its only metal treated control.
3.9. Phenolic profiling
Phenolic content of 60 days old plants showed increase in the total polyphenol content under Cu stress. The concentration of caffeic acid was recorded highest under stress. Umbelliferone, rutin and ellagic acid was also recorded in the B. juncea plants under Cu stress. With the application of CS under Cu stress, the concentration of ellagic acid and catechin increased significantly (Table ). The caffeic acid was recorded in highest concentration.
Table 3. Effect of Cu and castasterone on contents of various polyphenols (μg g−1) in 60 days old B. juncea plants
4. Discussion
Metal toxicity leads to physiological and cellular alterations, which finally causes distortion of plant metabolism (Hossain, Piyatida, da Silva, & Fujita, Citation2012). In the present study, it is clearly evident that ROS production viz. superoxide anion radical and hydrogen peroxide significantly increased in 60 and 90 old plants of B. juncea under higher concentrations of Cu. The Cu being a redox active metal it produces ROS directly through participating in Fenton reactions. The overproduction of ROS cause oxidative burst, damage biomolecules like membrane lipids, proteins, enzymes and nucleic acids and affect the detoxification processes. It has been found in the present study that application of CS reduced the ROS level in Cu treated B. juncea seedlings. Ramakrishna and Rao (Citation2012) observed that increased ROS levels were lowered by application of brassinosteroids in radish seedlings under Zn stress by application of brassinosteroids. The reduced levels of free radicals are associated with the up-regulation of antioxidative defense system including both enzymatic and non-enzymatic antioxidants with the help of BRs.
Decrease in the photosynthetic pigments is one of the most evident effects produced by metal toxicity. Present result showed that Cu stress significantly reduced the concentrations of chlorophylls while CS increased the pigment levels. Cu is known for disturbing the photosynthetic apparatus by altering the structure of chloroplast and composition of thylakoid membrane (Quartacci, Pinzino, Sgherri, Dalla Vecchia, & Navari‐Izzo, Citation2000). At toxic levels, Cu replaces Mg2+ by inhibiting synthesis of aminolevulinic acid (precursor of chlorophylls) and protochlorophyllide reductase (enzyme to catalyze the reductive formation of chlorophyllide). Feigl et al. (Citation2015) has also reported decrease in pigment concentration in B. juncea and B. napus under Cu stress. Kanoun-Boulé, Vicente, Nabais, Prasad, and Freitas (Citation2009) has reported decrease in chlorophyll content under Cu stress in duckweeds. They also revealed that chl a and carotenoids content were more sensitive to Cu toxicity than chl b. However, it has been observed in present study that carotenoids and anthocyanin content increased with Cu treatment as well with CS treatment. It has been reported that anthocyanin and carotenoids have role as antioxidant. These directly quench the intermediatory ROS and protect chlorophyll (Bajguz & Hayat, Citation2009). Similar results of increase in carotenoids content with EBL treatment are reported by Bali, Poonam, Kohli, Kaur, and Bhardwaj (Citation2016) under Cu stress in B. juncea plant. Our finding is confirmed by report of Baek et al. (Citation2012) who observed increase in anthocyanin content in Arabidopsis thaliana plants under Cu treatment. It has been perceived that anthocyanin content has role as antioxidant and enhances the protection against metal stresses (Neill & Gould, Citation2003). Under Cu stress, the photosynthetic efficiency was observed to decrease. Modifications in photosynthetic machinery under Cu stress by modifying the pigment and thylakoid protein composition results in decrease in gas exchange parameters (Azmat & Riaz, Citation2012; Fariduddin, Khalil, Mir, Yusuf, & Ahmad, Citation2013). The improvement in photosynthesis mediated by BRs might be due to enhanced activity and synthesis of enzymes of chlorophyll synthesis and those associated to photosynthesis (Xia et al., Citation2009). BRs enhance Rubisco activity and protect PS II under various environmental stresses and have positive effect on various gas exchange parameters (Wu, Ding, Zhu, Yang, & Zha, Citation2012). BRs also improve water uptake and relative water content causing enhanced photosynthetic rate (Ali, Hayat, & Ahmad, Citation2005). It has been suggested that BRs transcript for various photosynthetic genes, activates Calvin-Benson cycle thus leading to improvement in the photosynthetic capacity (Jiang et al., Citation2012). Earlier studies also validated that application of BRs shield photosynthesis and related characteristics (Ali et al., Citation2008; Fariduddin et al., Citation2013; Poonam et al., Citation2014; Poonam, Bhardwaj, & Sirhindi, Citation2015).
To overcome the oxidative damage caused by the excessive production of ROS, plants have antioxidant defense system. In settlement to ROS production, enhanced activities of antioxidative enzymes SOD, POD, CAT, APOX and PPO were observed with exposure to Cu stress which shows self-protection response by plants against Cu stress (Table ). SOD acts as first line of defense to scavenge ROS and catalyzes superoxide anion to H2O2 and O2 (Alscher, Erturk, & Heath, Citation2002). CAT and peroxidases breakdown H2O2 to H2O and O2. These results are supported by Ramakrishna and Rao (Citation2015) who reported increased SOD, POD, CAT, APOX and GPOX activities under Zn metal stress in radish seedlings. Enhanced enzymatic activity along with PPO in Jatropha curcas under Cd, Cr and Hg has been recorded by Devi Chinmayee et al. (Citation2014). The application of CS as seed soaking method further improved the activities of enzymes, which provide extra power to neutralize the ROS (Table ). These results are supported by Ramakrishna and Rao (Citation2012) who observed enhanced SOD, POD and CAT activities in Raphanus under Zn stress with EBL application. Similar results, enhanced activities of enzymes with EBL were obtained in B. juncea and Cucumis sativus under Ni and Cu stress (Ali et al., Citation2008; Fariduddin et al., Citation2013). The activity of PPO was further enhanced with the application of CS. These results are supported by Sharma, Hundal, Sharma, and Bhardwaj (Citation2014) who observed enhancement in the PPO activity under metal stress and 28-HBL application in radish plants. These results points that BRs induced protection are related with the efficient scavenging of ROS and thus lowering of stress.
Plants enhance the biosynthesis and accumulation of osmolytes like sugars, total phenols and flavonoids to regulate the plant responses towards metal stress (Sharma & Dietz, Citation2006). In the present investigation sugars, total phenol and flavonoids content was increased in the leaves of B. juncea plants under Cu treatment. However exogenous application of CS as seed soaking method further enhanced these osmolytes (Figure ). These results are in accordance to Xi et al. (Citation2013), who found that epibrassinolide application enhance these osmolytes in grapewine and increase resistance to chilling stress. It has been reported that sugars acts as osmolytes and provide protection to cells from metal toxicity (Jha & Dubey, Citation2005; Li et al., Citation2013). These sugars not only help as osmolytes, participate in stabilization of cellular membranes and maintenance of turgor but also act as signaling molecules (Wu, Srivastava, & Zou, Citation2013).
Compounds with phenol group are one of these secondary metabolites with known importance in stress management. Polyphenols are also involved in free radical scavenging due to their chemical structure and show better expression than vitamin E and C. Hydroxyl and carboxyl group of phenols help them to bind with heavy metals like Fe and cu (Jung et al., Citation2003). Accumulation of phenolic content under Cu stress might be due to induced synthesis of shikimate dehydrogenase (Dı́az, Bernal, Pomar, & Merino, Citation2001).
Flavonoids have metal chelating properties suggesting their role in oxidative stress conditions where transition metals ions like iron and Cu are involved. Flavonoids have higher reducing capacity for Cu ions than Fe ions (Mira et al., Citation2002). Flavonoids containing multiple hydroxyl substitutions displayed higher antiperoxyl radical activities (Cao, Sofic, & Prior, Citation1997). Flavonoids locate and neutralize free radicals before they harm the cells, thus act as ROS scavengers under hostile environmental conditions. Increase in total flavonoids content was observed by Mamat, Chong, Samad, Chai, and Manan (Citation2015) in Orthosiphon stamineus under Cu stress. Similar increase in flavonoids content under Cd stress in B. juncea plant is reported by Kapoor, Kaur, and Bhardwaj (Citation2014). The increase in total flavonoids content suggests its role in ROS scavenging. Cu and CS treatment further enhanced the flavonoids content in the B. juncea plants. These results are in accordance to the results obtained by Ahammed et al. (Citation2013). They found significant enhancement in the flavonoids content with the application of 24-EBL to tomato under phenanthrene. These results show that BRs regulated biosynthesis and accumulation of osmolytes help in maintaining cell functionality, stabilizing cellular membranes and also detoxification of ROS to enhance tolerance of B. juncea to Cu toxicity
5. Conclusion
It is established from the present study that presence of Cu in soil affects the photosynthetic pigments, gas exchange parameters and modulates the pool of ROS. The application of CS as seed soaking method has direct effect on the stress protective responses of B. juncea plants. It enhanced the enzymatic antioxidative defense system and levels of various metabolites, leading to the improved photosynthetic pigments and parameters. The findings lay ground to study the molecular mechanism of interactions between CS and stress indicators in managing the stress produced by Cu in B. juncea plants.
Funding
This work was supported by University Grants Commission.
Acknowledgements
Authors are thankful to University Grants Commission for providing financial assistance.
Additional information
Notes on contributors
Poonam Yadav
Poonam Yadav is a PhD student in Department of Botanical and Environmental Sciences, Guru Nanak Dev University, Amritsar, Punjab, India. Her research area is to dissect the mechanism of abiotic stress tolerance in plants through engineered metabolites and biochemical traits in the presence and absence of phytohormones. Currently she is working as senior research fellow under University Grants Commission, Government of India. She has also worked in a project funded by Council of Scientific and Industrial Research, India. She has more than 15 publications of international repute including research paper, reviews and book chapters.
References
- Aebi, H. (1984). Catalase in Vitro. Methods Enzymology, 105, 121–126.10.1016/S0076-6879(84)05016-3
- Ahammed, G. J., Choudhary, S. P., Chen, S., Xia, X., Shi, K., Zhou, Y., & Yu, J. (2013). Role of brassinosteroids in alleviation of phenanthrene-cadmium co-contamination-induced photosynthetic inhibition and oxidative stress in tomato. Journal of Experimental Botany, 64, 199–213.10.1093/jxb/ers323
- Ali, B., Hasan, S. A., Hayat, S., Hayat, Q., Yadav, S., Fariduddin, Q., & Ahmad, A. (2008). A role for brassinosteroids in the amelioration of aluminium stress through antioxidant system in mung bean (Vigna radiata L. Wilczek). Environmental and Experimental Botany, 62, 153–159.10.1016/j.envexpbot.2007.07.014
- Ali, B., Hayat, S., & Ahmad, A. (2005). Response of germinating seeds of Cicerarietinum to 28-homobrassinolide and/or potassium. General and Applied Plant Physiology, 3, 55–63.
- Alscher, R. G., Erturk, N., & Heath, L. S. (2002). Role of superoxide dismutases (SODs) in controlling oxidative stress in plants. Journal of Experimental Botany, 53, 1331–1341.10.1093/jexbot/53.372.1331
- Arnon, D. I. (1949). Copper enzymes in isolated chloroplasts. Photophenoloxidase in Beta vulgaris. Plant Physiology, 24, 1–15.10.1104/pp.24.1.1
- Azmat, R., & Riaz, S. (2012). The inhibition of polymerization of glucose in carbohydrate under Cu stress in Vigna radiata. Pakistan Journal of Botany, 44, 95–98.
- Azooz, M. M., Abou-Elhamd, M. F., & Al-Fredan, M. A. (2012). Biphasic effect of copper on growth, proline, lipid peroxidation and antioxidant enzyme activities of wheat (Triticum aestivum cv. Hasaawi) at early growing stage. Australian Journal of Crop Science, 6, 688–694.
- Baek, S., Han, T., Ahn, S.-K., Kang, H., Cho, M. R., Lee, S.-C., & Im, K.-H. (2012). Effects of heavy metals on plant growths and pigment contents in Arabidopsis thaliana. The Plant Pathology Journal, 28, 446–452.10.5423/PPJ.NT.01.2012.0006
- Bajguz, A., & Hayat, S. (2009). Effects of brassinosteroids on the plant responses to environmental stresses. Plant Physiology and Biochemistry, 47, 1–8.10.1016/j.plaphy.2008.10.002
- Bali, S., Poonam, Kohli, S.K., Kaur, H., & Bhardwaj, R. (2016). Improvement in photosynthetic efficiency of Brassica juncea under copper stress by plant steroid hormone. Journal of Chemical and Pharmaceutical Research, 8, 464–470.
- Bradford, M. M. (1976). A rapid and sensitive method for the quantitation of microgram quantities of protein utilizing the principle of protein-dye binding. Analytical Biochemistry, 72, 248–254.10.1016/0003-2697(76)90527-3
- Cao, G., Sofic, E., & Prior, R. L. (1997). Antioxidant and prooxidantbehavior of flavonoids: Structure-activity relationships. Free Radical Biology and Medicine, 22, 749–760.10.1016/S0891-5849(96)00351-6
- Clouse, S. D. (2011). Brassinosteroid signal transduction: From receptor kinase activation to transcriptional networks regulating plant development. The Plant Cell, 23, 1219–1230.10.1105/tpc.111.084475
- Devi Chinmayee, M., Anu, M., Mahesh, B., Mary, S., Mini, I., & Swapna, T. S. (2014). A comparative study of heavy metal accumulation and antioxidant responses in Jatropha curcas. IOSR Journal of Environmental Science, Toxicology and Food Technology, 8, 58–67.
- Dı́az, J., Bernal, A., Pomar, F., & Merino, F. (2001). Induction of shikimate dehydrogenase and peroxidase in pepper (Capsicum annuum L.) seedlings in response to copper stress and its relation to lignification. Plant Science, 161, 179–188.10.1016/S0168-9452(01)00410-1
- Dresler, S., Hanaka, A., Bednarek, W., & Maksymiec, W. (2014). Accumulation of low-molecular-weight organic acids in roots and leaf segments of Zea mays plants treated with cadmium and copper. Acta Physiologiae Plantarum, 36, 1565–1575. doi:10.1007/s11738-014-1532-x
- Fariduddin, Q., Khalil, R. R. A. E., Mir, B. A., Yusuf, M., & Ahmad, A. (2013). 24-Epibrassinolide regulates photosynthesis, antioxidant enzyme activities and proline content of Cucumis sativus under salt and/or copper stress. Environmental Monitoring and Assessment, 185, 7845–7856.10.1007/s10661-013-3139-x
- Feigl, G., Kumar, D., Lehotai, N., Pető, A., Molnár, Á., & Rácz, É. (2015). Comparing the effects of excess copper in the leaves of Brassica juncea (L. Czern) and Brassica napus (L.) seedlings: Growth inhibition, oxidative stress and photosynthetic damage. Acta Biologica Hungarica, 66, 205–221.10.1556/018.66.2015.2.7
- Gruszka, D. (2013). The brassinosteroid signaling pathway—New key players and interconnections with other signaling networks crucial for plant development and stress tolerance. International Journal of Molecular Sciences, 14, 8740–8774.10.3390/ijms14058740
- Hossain, M. A., Piyatida, P., da Silva, J. A. T., & Fujita, M. (2012). Molecular mechanism of heavy metal toxicity and tolerance in plants: Central role of glutathione in detoxification of reactive oxygen species and methylglyoxal and in heavy metal chelation. Journal of Botany, 2012, 37.
- Ivanova, E. M., Kholodova, V. P., & Kuznetsov, V. l. V. (2010). Biological effects of high copper and zinc concentrations and their interaction in rapeseed plants. Russian Journal of Plant Physiology, 57, 806–814.10.1134/S1021443710060099
- Jabs, T., Dietrich, R. A., & Dangl, J. L. (1996). Initiation of runaway cell death in an arabidopsis mutant by extracellular superoxide. Science, 273, 1853–1856.10.1126/science.273.5283.1853
- Jana, S., & Choudhuri, M. (1981). Glycolate metabolism of three submerged aquatic angiosperms during aging. Aquatic Botany, 12, 345–354.
- Jha, A. B., & Dubey, R. S. (2005). Effect of arsenic on behaviour of enzymes of sugar metabolism in germinating rice seeds. Acta Physiologiae Plantarum, 27, 341–347.10.1007/s11738-005-0010-x
- Jiang, Y. P., Cheng, F., Zhou, Y. H., Xia, X. J., Shi, K., & Yu, J. Q. (2012). Interactive effects of CO2 enrichment and brassinosteroid on CO2 assimilation and photosynthetic electron transport in Cucumis sativus. Environmental and Experimental Botany, 75, 98–106.10.1016/j.envexpbot.2011.09.002
- Jung, C., Maeder, V., Funk, F., Frey, B., Sticher, H., & Frossard, E. (2003). Release of phenols from Lupinusalbus L. roots exposed to Cu and their possible role in Cu detoxification. Plant Soil, 252, 301–312.10.1023/A:1024775803759
- Kabata-Pendias, A., & Pendias, H. (2001). Trace elements in soils and plants (3rd ed.). Boca Raton, FL: CRC Press.
- Kanoun-Boulé, M., Vicente, J. A. F., Nabais, C., Prasad, M. N. V., & Freitas, H. (2009). Ecophysiological tolerance of duckweeds exposed to copper. Aquatic Toxicology, 91, 1–9.10.1016/j.aquatox.2008.09.009
- Kanwar, M. K., Poonam, & Bhardwaj, R. (2015). Arsenic induced modulation of antioxidative defense system and brassinosteroids in Brassica juncea L. Ecotoxicology and Environmental Safety, 115, 119–125.10.1016/j.ecoenv.2015.02.016
- Kanwar, M. K., Poonam, Pal, S., & Bhardwaj, R. (2015). Involvement of asada-halliwell pathway during phytoremediation of chromium (VI) in Brassica juncea L. plants. International Journal of Phytoremediation, 17, 1237–1243.10.1080/15226514.2015.1058326
- Kapoor, D., Kaur, S., & Bhardwaj, R. (2014). Physiological and biochemical changes in Brassica juncea plants under Cd-induced stress. BioMed Research International, 2014, 13.
- Kaur, R., Yadav, P., Thukral, A. K., Walia, A., & Bhardwaj, R. (2016). Co-application of 6-ketone type brassinosteroid and metal chelator alleviates cadmium toxicity in B. juncea L. Environmental Science and Pollution Research, 1–16. doi:10.1007/s11356-016-7864-x
- Kim, T.-W., Chang, S. C., Lee, J. S., Hwang, B., Takatsuto, S., Yokota, T., & Kim, S. K. (2004). Cytochrome P450-catalyzed brassinosteroid pathway activation through synthesis of castasterone and brassinolide in Phaseolus vulgaris. Phytochemistry, 65, 679–689.10.1016/j.phytochem.2004.01.007
- Kono, Y. (1978). Generation of superoxide radical during autoxidation of hydroxylamine and an assay for superoxide dismutase. Archives of Biochemistry and Biophysics, 186, 189–195.10.1016/0003-9861(78)90479-4
- Kumar, K. B., & Khan, P. A. (1982). Peroxidase & polyphenol oxidase in excised ragi (Eleusine corocana cv. PR 202) leaves during senescence. Indian Journal of Experimental Biology, 20, 412–416.
- Li, P., Chen, L., Zhou, Y., Xia, X., & Shi, K. (2013). Brassinosteroids-induced systemic stress tolerance was associated with increased transcripts of several defence-related genes in the phloem in Cucumis sativus. PLoS One, 8, e66582.10.1371/journal.pone.0066582
- Maclachlan, S., & Zalik, S. (1963). Plastid structure, chlorophyll concentration, and free amino acid composition of a chlorophyll mutant of barley. Canadian Journal of Botany, 41, 1053–1062.10.1139/b63-088
- Mamat, D. D., Chong, C.-S., Samad, A. A., Chai, T.-T., & Manan, F. A. (2015). Effects of Copper on Total Phenolics, Flavonoids and Mitochondrial Properties of Orthosiphon stamineus Callus Culture. International Journal of Agriculture and Biology, 17, 1243–1248.10.17957/IJAB
- Mancinelli, A. L. (1984). Photoregulation of anthocyanin synthesis. Plant Physiology, 75, 447–453.10.1104/pp.75.2.447
- Metwali, E. M. R., Gowayed, S. M. H., Al-Maghrabi, O. A., & Mosleh, Y. Y. (2013). Evaluation of toxic effect of copper and cadmium on growth, physiological traits and protein profile of wheat (Triticum aestivium L.), maize (Zea mays L.) and shorghum (Sorghum bicolor L.). World Applied Sciences Journal, 21, 301–314.
- Mira, L., Fernandez, M. T., Santos, M., Rocha, R., Florencio, M. H., & Jennings, K. R. (2002). Interactions of flavonoids with iron and copper ions: A mechanism for their antioxidant activity. Free Radical Research, 36, 1199–1208.10.1080/1071576021000016463
- Mishra, S., & Dubey, R. S. (2005). Heavy metal toxicity induced alterations in photosynthetic metabolism in plants. Handbook of Photosynthesis, 2, 845–863.
- Nakano, Y., & Asada, K. (1981). Hydrogen peroxide is scavenged by ascorbate specific peroxidase in spinach chloroplasts. Plant Cell Physiology, 22, 867–880.
- Neill, S. O., & Gould, K. S. (2003). Anthocyanins in leaves: Light attenuators or antioxidants? Functional Plant Biology, 30, 865–873.10.1071/FP03118
- Poonam, Kaur, R., Bali, S., Singh, R., Pati, P.K., & Bhardwaj, R. (2014). Treatment of 24-EBL to B. juncea plants under Cu-metal stress lowers oxidative burst by activity of antioxidant enzymes. Journal of Stress Physiology and Biochemistry, 10, 315–327.
- Poonam, R. K., Bhardwaj, R., & Sirhindi, G. (2015). Catsaterone regulated polyphenolic metabolism and photosynthetic system in Brassica juncea plants under copper stress. Journal of Pharmacognosy and Phytochemistry, 4, 282–289.
- Quartacci, M. F., Pinzino, C., Sgherri, C. L., Dalla Vecchia, F., & Navari‐Izzo, F. (2000). Growth in excess copper induces changes in the lipid composition and fluidity of PSII-enriched membranes in wheat. Physiologia Plantarum, 108, 87–93.10.1034/j.1399-3054.2000.108001087.x
- Ramakrishna, B., & Rao, S. S. R. (2012). 24-Epibrassinolide alleviated zinc-induced oxidative stress in radish (Raphanus sativus L.) seedlings by enhancing antioxidative system. Plant Growth Regulation, 68, 249–259.10.1007/s10725-012-9713-3
- Ramakrishna, B., & Rao, S. R. R. (2015). Foliar application of brassinosteroids alleviates adverse effects of zinc toxicity in radish (Raphanussativus L.) plants. Protoplasma, 252, 665–677.10.1007/s00709-014-0714-0
- Sharma, N., Hundal, G. S., Sharma, I., & Bhardwaj, R. (2014). 28-Homobrassinolide alters protein content and activities of glutathione-s-transferase and polyphenol oxidase in Raphanus sativus L. plants under heavy metal stress. Toxicology International, 21, 44–50.
- Sharma, S. S., & Dietz, K. J. (2006). The significance of amino acids and amino acid-derived molecules in plant responses and adaptation to heavy metal stress. Journal of Experimental Botany, 57, 711–726.10.1093/jxb/erj073
- Singleton, V. L., & Rossi, J. A. (1965). Colorimetry of total phenolics with phosphomolybdic phosphotungstic acid reagents. American Journal of Enology and Viticulture, 16, 144–158.
- Vamerali, T., Bandiera, M., & Mosca, G. (2010). Field crops for phytoremediation of metal-contaminated land. A review. Environmental Chemistry Letters, 8, 1–17.10.1007/s10311-009-0268-0
- Wu, Q.-S., Srivastava, A. K., & Zou, Y.-N. (2013). AMF-induced tolerance to drought stress in citrus: A review. Scientia Horticulturae, 164, 77–87.10.1016/j.scienta.2013.09.010
- Wu, X. X., Ding, H. D., Zhu, Z. W., Yang, S. J., & Zha, D. S. (2012). Effects of 24-epibrassinolide on photosynthesis of eggplant (Solanum melongena L.) seedlings under salt stress. African Journal of Biotechnology, 11, 8665–8671.
- Wu, X., Yang, Z. Y., Li, Y., Hogerkorp, C. M., Schief, W. R., Seaman, M. S., … Longo, N. S. (2010). Rational design of envelope identifies broadly neutralizing human monoclonal antibodies to HIV-1. Science, 329, 856–861. 10.1126/science.1187659
- Xi, Z. M., Zhang, Z. W., Huo, S. S., Luan, L. Y., Gao, X., Ma, L. N., & Fang, Y. L. (2013). Regulating the secondary metabolism in grape berry using exogenous 24-epibrassinolide for enhanced phenolics content and antioxidant capacity. Food Chemistry, 141, 3056–3065.10.1016/j.foodchem.2013.05.137
- Xia, X. J., Zhang, Y., Wu, J. X., Wang, J. T., Zhou, Y. H., Shi, K., … Yu, J. Q. (2009). Brassinosteroids promote metabolism of pesticides in cucumber. Journal of Agricultural and Food Chemistry, 57, 8406–8413.10.1021/jf901915a
- Ye, Q., Zhu, W., Li, L., Zhang, S., Yin, Y., Ma, H., & Wang, X. (2010). Brassinosteroids control male fertility by regulating the expression of key genes involved in Arabidopsis anther and pollen development. Proceedings of the National Academy of Sciences, 107, 6100–6105.10.1073/pnas.0912333107
- Yruela, I. (2009). Copper in plants: Acquisition, transport and interactions. Functional Plant Biology, 36, 409–430.10.1071/FP08288
- Zhishen, J., Mengcheng, T., & Jianming, W. (1999). The determination of flavonoid contents in mulberry and their scavenging effects on superoxide radicals. Food Chemistry, 64, 555–559.10.1016/S0308-8146(98)00102-2