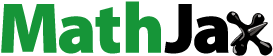
Abstract
Ethiopia is the fifth-largest coffee producer in the world. The country has made continuous efforts to enhance the production, productivity and quality of its coffee. Yet, comprehensive data on these issues are scant. This paper aims to document available information on the economic importance, production, productivity, quality and chemical contents of Ethiopian coffee, and to identify developmental and/or research gaps on its productivity and quality. Coffee now accounts for ca. 25–30% of the country’s total foreign currency earnings and the amount of foreign currency earnings from coffee increases over the years with a varying rate. Production and cultivation areas of Ethiopian coffee also increase over the past 60 years, but the changes in its productivity and quality are minor. Also, the share of the top grade (Grade 1 and 2) coffees in Ethiopia has remained lower over time, and the quality and chemical composition of Ethiopian coffee vary with growing region and locality. Compared to others, coffees from Eastern (Harar) and Southern regions are better in overall quality, and coffee from Northwestern region is higher in chlorogenic acid and sucrose contents, whereas those from Harar and Southwestern regions are lower in caffeine and chlorogenic acid contents, respectively. However, Harar coffee is higher in fatty acid content than other region coffees. Overall, the paper shows (1) the economic importance, production, productivity, quality and chemical contents, (2) information gaps on productivity, and quality and chemical profiles, and (3) the existence of a large room for productivity and quality improvements of Ethiopian coffee.
PUBLIC INTEREST STATEMENT
Ethiopia has suitable environments for coffee production and it produces different coffee types in different coffee growing regions. Coffee in Ethiopia is the most important source of foreign currency earnings for the country and cash incomes for the coffee producing farmers. It is often said that the Ethiopian coffee is low in production, productivity and quality, and varied in quality with growing region/area. However, well-organized data on these issues are not available. Hence, evaluating available information on production, productivity, quality and chemical composition of coffee in Ethiopia is important to identify suitable interventions for quality coffee production in the country. This enhances the foreign currency earnings and livelihoods of small-holder coffee farmers in the country. The paper shows the trends in production, productivity and quality of coffee over time, differences between coffee origins in quality and quality precursors, and developmental and research gaps for quality coffee production in Ethiopia.
1. Introduction
Coffee is the second most traded commodity in the world market after oil, accounting for an estimated export worth of 36.3 billion USD in 2021 (Workman, Citation2022), and it is the main source of foreign currency earnings for over 80 tropical countries including Ethiopia (Gole, Citation2015). The coffee industry provides a job opportunity and a livelihood for over 100 million people and 25 million farmers worldwide, respectively (A. P. Davis, Citation2011; Gole, Citation2015). Coffee is also important for industries involved in its roasting, mixing and packaging (ICO, Citation2014), and around 40% of the world’s population consumes coffee (Alonso-Salces et al., Citation2009).
Ethiopia, being the origin and the center of genetic diversity of arabica coffee (Coffea arabica L.), is the largest producer, exporter and consumer of coffee in Africa and the fifth-largest producer in the world. Ethiopia produces only arabica coffee and it is the third-largest arabica coffee producer in the world, next to Brazil and Colombia. This coffee is the backbone of the Ethiopian economy, accounting for 25–30% and 10% of the total foreign currency earnings and the government revenues of the country, respectively (MoARD Ministry of Agriculture and Rural Development, Citation2007; Tefera et al., Citation2014); and providing employment for 25% of the country’s population (Gole, Citation2015). Coffee also plays an important role in the sociocultural life of many people in Ethiopia. For example, the coffee drinking ceremony after certain sociocultural events such as marriage arrangement and dispute settling is often considered as approval for these events.
Coffee in Ethiopia is produced under four different production systems, namely forest, semi-forest, garden and plantation coffees, which vary in their level of management from little or none in the forest coffee to intensive in the plantation coffee, and in four coffee-growing regions, namely Eastern or Harar, Southern, Southwestern and Northwestern regions, which vary in their growing environment, coffee production system or both. Forest coffee is a naturally regenerated and unmanaged (little managed) coffee grown under natural forests. It is mainly present in the Southwestern region, which is wetter than the other coffee regions.Worku et al. (Citation2019) has more details on the four coffee-growing regions. Semi-forest coffee is a dominant production system in the Southwestern region. It is either an intensively managed forest coffee (e.g., clearing of plants competing with coffee, reducing the large canopy trees to open up the canopy, protecting and thinning of naturally regenerated coffee seedlings and planting of coffee seedlings in open areas) or a traditionally managed planted coffee in natural forests. The latter is sometimes called a semi-forest plantation coffee or a semi-plantation coffee. Garden coffee, which is mainly present in the Eastern, Southern and Northwestern coffee regions, is a coffee production system that is commonly found around homesteads and consists of other crops (e.g., enset, banana, fruit trees, root crops, vegetables, spices, maize, chat or khat, etc.) with no or few shade trees (Gole, Citation2015). Plantation coffee (mainly found in the Southwestern region) is a coffee production system wherein improved varieties and agronomic practices, such as seed preparation, nursery management, plant spacing, mulching, weeding, fertilizer and herbicide application and pruning, are usually used (Gole, Citation2015). These practices are rarely used in other production systems. Coffee in Ethiopia is processed by dry and wet methods. However, majority of Ethiopian coffee (> 90%) is produced by smallholder farmers under semi-forest and garden systems in three major coffee-growing regions (i.e., Harar, Southern and Southwestern regions) and processed by the dry method (Boot, Citation2011; Worku, Citation2019). Ethiopian Commodity Exchange (ECX) evaluates and grades the quality of coffee in Ethiopia. Currently, ECX has nine coffee quality inspection centers (cupping labs) distributed across the three major coffee regions. Most of these cupping labs and their cuppers are Q certified, and each cupping lab has 3–5 professional cuppers (Worku et al., Citation2016). ECX also mediates the auctions of the exported coffee.
Production and cultivation areas of Ethiopian coffee increased during the past 60 years. However, its productivity and quality have not changed considerably in spite of the several interventions in the coffee sector of the country for many years to boost both productivity and quality of coffee (Ayele et al., Citation2021; Worku, Citation2019). Moreover, some recent studies (e.g.,Moat, Williams, Baena, Wilkinson, Demissew, et al., Citation2017; Moat, Williams, Baena, Wilkinson, Gole, et al., Citation2017) indicated the impact of climate change on coffee production in Ethiopia in addition to the commonly known production constraints of coffee in the country, such as traditional cultivation practices and diseases. Yet, the impact varies with coffee growing region; for example, it is higher in the Eastern than in other coffee regions. Studies also indicated the influences of (1) coffee production system and growing region on production and productivity of coffee (Gole, Citation2015;Wiersum et al., Citation2008; Worku, Citation2019) and (2) the growing region (Mehari et al., Citation2016a, Citation2016b; Worku et al., Citation2023a), the growing condition (e.g., elevation, soil, shade and production system) (Getachew et al., Citation2022; Tolessa et al., Citation2017; Worku et al., Citation2018, Citation2022), the harvest period (Tolessa et al., Citation2017) and the postharvest processing of coffee (Tolessa, Citation2017; Worku et al., Citation2018, Citation2023b) on quality and chemical composition of coffee in Ethiopia. The objectives of this review paper are as follows: (1) to present the physiognomies of Ethiopian coffee with special emphasis on its economic importance, production and productivity, quality and chemical composition, and (2) to identify developmental and/or research gaps that need a great focus to improve its productivity and quality.
2. Economic importance
Agriculture is the largest sector in the Ethiopian economy, accounting for over 40% of GDP, 80% of exports and 70% of the raw materials required for agro-processing industries. It also employs over 80% of the country’s labor force and provides basic needs and income to more than 90% of the poor (A. Gebreselassie et al., Citation2010; Alemu et al., Citation2003; Diao et al., Citation2010; Tefera & Bickford, Citation2021). The coffee industry is the major one in the agricultural sector of Ethiopia in its contribution to the national economy and exports. It accounts for about 5% of GDP, 10% of total agricultural production, 30% of total agricultural export, 10% of the total government revenue and 25–30% of the total export earnings of the country, and it has generated about 821.14 million USD in the 2019/2020 cropping year (MoARD Ministry of Agriculture and Rural Development, Citation2007; Girma, Citation2011; Tefera & Bickford, Citation2021; Tefera et al., Citation2014). However, its share of total export earnings was historically more than 60%, but has gradually declined over the years as a result of increased exports of other commodities, such as gold, flowers, chat (khat), textiles and leather products (Tefera et al., Citation2014). Chat (Catha edulis (Vahl) Forssk. ex Endl.) (a bushy plant with a mild addictive stimulant property) currently generates a higher income for the farmers compared to coffee, particularly in the Eastern (Harar) region of Ethiopia and the total volume of chat grown during the last 5 years (2015–2020) has increased by around 220% (Tefera & Bickford, Citation2021). In value terms, however, the earnings from coffee export show an upward trend with some downward interruptions mainly due to a substantial decline in international coffee prices (e.g., it increased from 344.3 million USD in 2005/2006 cropping year to about 821.14 million USD in 2019/2020 cropping year) (Tefera & Bickford, Citation2021; Tefera & Francom, Citation2016; Tefera et al., Citation2014). In addition, studies have shown a significant positive relationship between coffee exports and economic growth in Ethiopia (Gizaw et al., Citation2022; Yifru, Citation2015).
Coffee is not only the major source of foreign currency for Ethiopia but also supports the whole economic growth of the nation, and is the source of income for millions of coffee-growing farmers, coffee-trading farmers, cooperatives and unions, financial institutions and transportation enterprises (Gebreselassie et al., Citation2010). In Ethiopia, coffee is produced by more than 4 million farming households (Wiersum et al., Citation2008) and accounts for about 70% of the total value of the output sold and up to 95% of cash income of smallholder coffee farmers (Gebreselassie & Lud, Citation2007). Furthermore, a number of people are currently engaged in small roadside coffee stands that emerged and flourished in and around major towns of the country during the past few years. These shops serve coffee in traditional fashion and have become popular among a segment of consumers who are growing increasingly frustrated by the escalating coffee prices and the deteriorating quality of coffee being served at upper-end cafes (Tefera et al., Citation2014).
3. Production and productivity
Ethiopia produces and exports only arabica coffee (Figure ), which is widely believed to have originated in the Southwestern highlands of the country where it still grows as understory or “wild” coffee in the natural forests (Gole et al., Citation2002). About 90% of Ethiopia’s coffee is produced by smallholder farmers on less than 2 ha of land, while the remaining 5–10% is grown on modern commercial farms (Gole, Citation2015; Petit, Citation2007; Tefera et al., Citation2014). Ethiopian coffee production is mainly characterized by traditional farm management system, limited use of fertilizers and pesticides coupled with manual coffee cultivation and drying systems; hence, nearly 95% of the country’s production is arguably claimed as organic. However, only a fraction of the total production is certified as organic because certification costs are relatively high and the returns for organic coffee are somewhat minimal (Tefera & Francom, Citation2015). Ethiopian coffee is processed by two common processing methods: the dry (“natural” sun-drying) and wet methods. Currently, about 70–80% of the total coffee produced in Ethiopia is the dry-processed (unwashed) coffee and the remaining 20–30% is the wet-processed (washed) coffee (Tefera & Bickford, Citation2021). The country’s export also consists of 70–80% and 20–30% unwashed and washed coffee, respectively (Boot, Citation2011). Unwashed coffee commands a lower price in many markets, including the US, where consumers prefer “cleaner” washed coffee. However, some countries, such as Japan, specifically require unwashed coffee for its more natural and richer taste (Tefera & Bickford, Citation2021).
Figure 1. Total production (black line), domestic consumption (blue line) and export (red line) of Ethiopian coffee from 1990/1991 to 2017/2018 cropping years; author’s computation based on data from ICO database, http://www.ico.org/new_historical.asp.

An accurate estimate of coffee production in Ethiopia is difficult because a high fraction of the crop is consumed on-farm or locally (Petit, Citation2007). It is also difficult to generalize the productivity of Ethiopian coffee primarily due to lack of data and secondly due to its high variation with coffee production system and growing region of the country. Despite these limitations, International Coffee Organization (ICO) and United States Department of Agriculture (USDA) estimates show that the country’s production trend over the past 60 years is generally upward despite some downward interruptions, reaching around 6.5 million (60 kg) bags in the 2016/2017 cropping year (FAS, & Francom, Citation2017; ICO, Citation2014, Citation2018). Coffee export also shows a similar trend of growth with that of production. Production grew by an average annual growth rate of 2.6% during the period from 1963/1964 to 2012/2013 and export by 6.2% during the period from 1990/1991 to 2011/2012 (ICO, Citation2014), but both showed a stagnant growth during the period from 2011/2012 to 2016/2017 (Figure ). During this period, production has hovered around 6.5 million bags and export around 3.3 million bags (FAS, & Francom, M. G, Citation2017; ICO, Citation2018; Tefera & Francom, Citation2016). Yet, both production and export have been growing steadily over the past 3 years and their respective estimated amount reached to 7.6 and 4.2 million 60 kg bags (456,000 and 250,000 t) in 2020/21 (Tefera & Bickford, Citation2021). Such increase in production is mainly attributed to coffee cultivation area expansion and coffee management intensification. The coffee area, for example, has substantially expanded from 250,000 ha in 1990/1991 to 540,000 ha in 2019/2020 (FAO, Citation2015; Tefera & Bickford, Citation2021). The findings of Ayele et al. (Citation2021) also show an increasing trend in harvested area and production, but a cyclical decreasing trend in productivity over the past 27 years (1993–2019) despite the several interventions that have been made in the sector to improve coffee productivity (Figure ). During this period, the harvested area and production increased by 8.1% and 6.7%, respectively, but productivity reduced by about 0.5%. Productivity was, however, more stable than harvested area and production, and the effect of harvested area on production differentials across cropping years was substantial and positive, while that of productivity and productivity-harvested area interaction was negative. These changes in the production and productivity of Ethiopian coffee over the 1993–2019 periods are also much lower compared to the top four coffee producing countries in the world (Table ). Moreover, the productivity of Ethiopian coffee, which is estimated to be 700–800 kg ha−1, is considered to be lower compared to other countries, such as Brazil, Vietnam and Colombia (Ayele et al., Citation2021; Tefera & Bickford, Citation2021). For example, it is nearly half of that of Brazil and Colombia, the two largest producers of arabica coffee in the world (). However, Ethiopia, accounting for about 4.5% of global coffee production, is the largest coffee producer in Africa and the fifth in the world, next to Brazil (32.7%), Vietnam (18.6%), Colombia (9.5%) and Indonesia (7.8%) (ICO, Citation2016; Tefera & Francom, Citation2016). Ethiopia is also the third-largest arabica coffee producer in the world next to Brazil and Colombia. The production and productivity of the five top coffee producing countries in the world is presented in .
Figure 2. Trends in harvested area, production and productivity of coffee in Ethiopia for the periods of 1993–2019; Pre-AGP (Pre-Agricultural Growth Program) and AGP (Agricultural Growth Program); adapted fromAyele et al. (Citation2021).

Table 1. Compound growth rate (CGR) of coffee production and productivity of the five major coffee producing countries in the world for the 1993–2019 period; adapted from Ayele et al. (Citation2021)
Low coffee production and productivity in Ethiopia is mainly due to the use of traditional farm management system, limited use of farm inputs (e.g., improved seeds, fertilizers and pesticides), harvesting and postharvest processing problems, inadequate extension services and increased farmland competition from chat cultivation (Tefera & Bickford, Citation2021; Tefera et al., Citation2014). Despite these limitations, Ethiopia has a good potential to increase its coffee production and productivity as it is endowed with suitable elevations and climates in the coffee-growing belt of the country (Tefera et al., Citation2014). However, some studies (e.g.,Moat, Williams, Baena, Wilkinson, Gole, et al., Citation2017) show that climate change affects this potential and may lead to decreased suitability of some regions to produce coffee. Particularly, climate change (e.g., erratic rainfall and drought occurrence) and expansion of chat cultivation impact coffee production in the Harar region more than that in other coffee-growing regions (Kandari et al., Citation2014; Moat, Williams, Baena, Wilkinson, Gole, et al., Citation2017; Woldu et al., Citation2015).
About 50–55% of Ethiopia’s total production is consumed domestically (Tefera & Bickford, Citation2021) and domestic consumption increased at a similar rate as that of export (Figure ). This is because coffee plays a vital role not only in economical but also in cultural and social lives of many Ethiopians. For many people in Ethiopia, coffee drinking is part of their lifestyle and an everyday habit. However, the coffee consumed in the local market is mainly the coffee that failed to meet the ECX export quality standards or was rejected by ECX; but still the local price is usually higher than the export price (Tefera & Bickford, Citation2021).
4. Quality
There are several coffee quality measures in the Ethiopian coffee market, including certification, geographical origin, processing method and quality grade (Minten et al., Citation2014). Certification and traceability have become major new requirements in the global food trade (Swinnen, Citation2007). Such certification schemes are often implemented to add value to a product (Jena et al., Citation2012). International buyers and consumers are often willing to pay extra for a certified product. Following this, the share of certified coffee in Ethiopia is increasing, but is clearly lower than that in other countries. For example, data from the Ministry of Trade show that the share of this coffee over the period 2006/2007 to 2012/2013 was only 2% (Minten et al., Citation2014).
Geographic origin is another important quality indicator of coffee, as it is strongly related to cup taste. As a result, some coffee types that can be differed in their quality attributes are identified in Ethiopia based on their geographic origins. The major ones include Harar, Sidamo, Yirgacheffe, Jimma, Limmu and Wellega (Lekemt) coffees (Minten et al., Citation2014). Harar coffee is produced in the Eastern (Harar) coffee region, Sidamo coffee and Yirgacheffe coffee are produced in Sidamo and Gedeo areas of the Southern coffee region, respectively, and Jimma coffee, Limmu coffee and Wellega coffee are produced in Jimma, Limmu and Wellega areas of the Southwestern coffee region, respectively. Kufa (Citation2012) associated coffee tastes and regions as follows: “mocha” (chocolate) for Harar coffee, spicy for Sidamo coffee, floral for Yirgacheffe coffee, winy for Jimma and Limmu coffees, and fruity for Wellega (Lekemt) coffee. As per Boot (Citation2011), high-quality Harar coffee is notable for its fruity flavor and creamy body; Sidamo coffee for its very wide variety of flavors; Yirgacheffe coffee for its fruity flavor, bright acidity and silky mouth feel; Limmu coffee for its mild acidity; and Wellega (Lekemt) coffee for its perfume-like aftertaste. Recent studies (Montagnon, Citation2020; Worku et al., Citation2023a) also show that the quality of Ethiopian coffee varies with growing region and locality. In Worku et al. (Citation2023a), higher physical, acidity and overall quality scores are observed for Harar and Yirgacheffe coffees than for Southwestern coffees. Yet, the export shares of the premium quality Harar and Yirgacheffe coffees are relatively small although they are higher in terms of value because of their relatively higher prices (Minten et al., Citation2014). This generally shows that it is hard to generalize the flavor of Ethiopian coffee as each coffee-growing region in Ethiopia is home to unique flavors of coffee.
A quality grade of coffee is the main quality indicator used as a yardstick to determine the price of coffee. Based on the scores of green bean physical and cup qualities (0–100), the coffee lots in Ethiopia can be classified into six different preliminary quality grades (i.e., Grade 1 ≥ 85, Grade 2 = 75–84, Grade 3 = 63–74, Grade 4 = 47–62, Grade 5 = 31–46 and under grade (U.G.) ≤ 30 scores) and three specialty quality grades (i.e., Specialty 1 (Q1) (≥85), specialty 2 (Q2) (80–< 85) and commercial (Grade 3) (71–< 80) (ECX Ethiopia Commodity Exchange, Citation2011). Preliminary quality assessment includes four physical quality attributes of green beans (i.e., primary defects, secondary defects, odor and color), four cup quality attributes (i.e., acidity, body, cup cleanness and flavor) and total preliminary quality (the sum of physical and cup qualities) (ECX Ethiopia Commodity Exchange, Citation2011). The primary defects include full black, sour, fungus attacked and insect damaged beans as well as the presence of foreign matters, and the secondary defects include partial black, broken, insect damaged, faded and coated beans (Worku et al., Citation2023a). Specialty quality assessment includes 10 cup quality attributes (i.e., aroma, body, acidity, flavor, cup cleanness, balance, sweetness, uniformity, aftertaste and overall cup preference) and total specialty quality (the sum of the 10 cup quality attributes). It is done for those coffee lots obtaining Grade 1 or Grade 2 in the preliminary quality assessment (ECX Ethiopia Commodity Exchange, Citation2011).
Minten et al. (Citation2014) report a slight increase in quality from the 2006/2007 to the 2012/2013 cropping years for both washed and unwashed coffees. For the unwashed bean market segment, the share of Grade 4 has been increasing slightly over time, while the share of the worse quality (Grade 5) has been decreasing slightly (Figure ). In the 2006/2007 cropping year, for example, 24% of unwashed coffee was Grade 4, but this increased to 31% in 2012/2013. However, there are few changes over time in the quality grade for washed coffee and a small decline in the U.G. washed coffee (Figure ); so, the share of washed coffee in total exports has not changed significantly over time, and remained close to 30% (Minten et al., Citation2014). Most importantly, the share of top-quality coffees (e.g., Grade 1 washed coffee and Grade 3 unwashed coffee) is negligible and remains unchanged over time (Figure ). Nure (Citation2008) also indicate that out of coffee sent to the coffee quality inspection and auction center from 2003/2004 to 2006/2007, more than 60% of unwashed coffee was classified into Grade 3 and about 80% of washed coffee into Grade 2 and Grade 3. Our recent study also shows an average Grades of 2 and 3 for unwashed coffee samples from Harar and Southern regions and Southwestern region, respectively (Worku et al., Citation2023a). Considering a lower average share of washed coffee in total productions and exports (not more than 30%) (Boot, Citation2011; Minten et al., Citation2014; Tefera & Bickford, Citation2021), the above information in general can indicate a lower share of top-grade coffees in Ethiopia (Figure ) and the presence of a large room for the improvement of the Ethiopian coffee quality.
Figure 3. Evolution of quality grades (Grade 1–5 and U.G. (under grade)) for the washed (left) and unwashed (right) coffee market segment from 2006 to 2013 at six-month interval; original copy from Minten et al. (Citation2014).

5. Chemical composition
Green coffee beans are composed of volatile and nonvolatile fractions of compounds, some of the former ones being responsible for the aroma and the latter for the basic taste sensations of sourness, bitterness and astringency (Buffo & Cardelli-Freire, Citation2004; Farah, Citation2012). About 100 different volatile (aroma) compounds have been identified in green coffee. The most abundant classes of aroma compounds are alcohols, esters, hydrocarbons and aldehydes. Ketones, pyrazines, furans and sulfur compounds are also important volatile compounds identified in green coffee (Farah, Citation2012; Toci & Farah, Citation2008). During roasting, a number of aroma compounds are also formed from nonvolatile compounds (carbohydrates, proteins, peptides, chlorogenic acids, trigonelline, lipids) through different chemical reactions (Maillard reactions, Strecker degradation, caramelization, oxidation) (Grosch, Citation2001). As a result, more than 950 aroma compounds have been identified in roasted coffee (Farah, Citation2012). The classes of aroma compounds typically found in roasted coffee are hydrocarbons, alcohols, aldehydes, ketones, acids and anhydrides, esters, lactones, phenols, furans and pyrans, thiophenes, pyrroles, oxazoles, thiazoles, pyridines, pyrazines, amines, and various sulfur and nitrogen compounds (Farah, Citation2012; Grosch, Citation2001). However, the actual number of indispensable coffee aroma compounds could be as small as nine (Yeretzian et al., Citation2019). The nonvolatile fraction of coffee beans is primarily composed of caffeine, trigonelline, chlorogenic acids, organic acids, carbohydrates, fibers, proteins, free amino acids, lipids and minerals (Farah, Citation2012). Of these compounds, caffeine, trigonelline, chlorogenic acids, soluble fiber and diterpenes from the lipid fraction are important contributors to the beverage flavor after roasting. Carbohydrates are precursors for the Maillard reaction and caramelization, which are vital for the development of color and aroma of the coffee brew. They also contribute to the acidity and body of the brew after coffee roasting. Sucrose, the most abundant carbohydrates (soluble sugars) present in coffee beans, is important for coffee flavor. Proteins, peptides and free amino acids are also vital for coffee flavor since they are needed for the Maillard reaction and serve as precursors for the formation of aroma compounds, such as furans, pyridines, pyrazines, pyrrols, aldehydes and melanoidins (Farah, Citation2012). Likewise, trigonelline contributes to the flavor of the beverage by acting as the precursor for different classes of aroma compounds, including furans, pyrazines, pyrroles and pyridines, formed during coffee roasting (Mehari et al., Citation2016b).
The complete chemical profiles of Ethiopian coffee have not been studied so far. However, some pioneer studies (e.g.,Habte et al., Citation2016; Mehari et al., Citation2016c, Citation2016a, Citation2016b,Citation2019, Citation2020; Worku et al., Citation2023a) report the contents of some chemicals (caffeine, theobromine, theophylline, trigonelline, chlorogenic acids, sucrose, fatty acids, flavonoids and total polyphenols) and minerals in green beans of different geographical origins. In green coffee beans of Ethiopia, the theobromine content ranges from 0.0048% to 0.0094% dw (Mehari et al., Citation2016b) and that of the total soluble polyphenols, cell wall-bound polyphenols and flavonoids ranges from 21.8 to 43.6 mg GAE (gallic acid equivalents) g−1, 8.6–15.3 mg GAE g−1 and 3.3–6.2 mg CE ((+)-catechin equivalents) g−1, respectively (Mehari et al., Citation2020). The cell wall-bound polyphenols and flavonoids represent 21.0–32.5% and 10–17% of the total polyphenols and soluble polyphenols contents of green coffee beans, respectively (Mehari et al., Citation2020). However, theophylline and theobromine, which have stimulatory effects on the central nervous system and cause diuresis, peripheral vasoconstriction, smooth muscle relaxation and myocardial stimulation, but to a lesser degree than caffeine, are not found in all region coffees and Harar coffee, respectively (Mehari et al., Citation2016b). Much lower amounts of total soluble and cell wall-bound polyphenols are also found in Harar coffee than in the other region coffees (Mehari et al., Citation2020). These findings imply that the absence or presence of theobromine and/or the lower or higher amounts of polyphenols can be used as a chemical marker for ascertaining whether the coffee originates from Harar or not.
This section focuses and discusses in detail on the main and the most studied aroma and flavor precursors and bioactive compounds, such as caffeine, trigonelline, chlorogenic acids, sucrose, fatty acids and proteins, and minerals in green coffee beans of Ethiopia.
5.1. Caffeine content
Caffeine (1,3,7-trimethylxanthine) is a purine alkaloid that occurs naturally in coffee beans (Higdon & Frei, Citation2006). It possesses bitter characteristics (Farah, Citation2012) and contributes to the strength, body and bitterness of the brewed-coffee (Buffo & Cardelli-Freire, Citation2004); however, it is responsible for no more than 10% of the perceived bitterness of the coffee beverage (Farah, Citation2012) and there is no strong association between its levels and good cup quality (Farah et al., Citation2006). Caffeine is heat stable and as a result its content is not affected by roasting (Farah et al., Citation2006;Oestreich-Janzen, Citation2010).
Caffeine stimulates the central nervous system as an adenosine-receptor antagonist and exhibits antioxidant and antibacterial activities (Antonio et al., Citation2010; Lee, Citation2000). Currently, caffeine is the world’s most famous behaviorally active drug and consumed chiefly from coffee (Davis et al., Citation2010). However, its effects on health are controversial. For example, caffeine intake has been associated with high blood cholesterol, coronary diseases and cancer, but may lower the risk of Parkinson’s disease, suicide and hepatic cirrhosis (Farah, Citation2012; Higdon & Frei, Citation2006). Low to moderate caffeine intake is generally linked to increased energy availability, alertness and concentration, learning capacity, exercise performance, and perhaps less fatigue and better mood, but high doses can produce some negative effects; e.g., anxiety, tachycardia, insomnia, in some sensitive individuals during 2–6 h after coffee intake (Farah, Citation2012; Jiang et al., Citation2014; Ogita et al., Citation2003). Acute caffeine intake has harmful effects on glucose tolerance, glucose disposal and insulin sensitivity in lean, obese and type 2 diabetic individuals (Higdon & Frei, Citation2006; Shearer et al., Citation2007) and increases the urinary excretion of some minerals, such as Ca (Ribeiro-Alves et al., Citation2003). However, after long-term consumption, most of these acute effects tend to disappear owing to metabolic adaptations in the body (Demirbag et al., Citation2006). Moreover, caffeine has exhibited antibacterial effect against cariogenic microorganisms (Antonio et al., Citation2010). It also acts as a natural pesticide for coffee plant (Ashihara & Crozier, Citation2001).
Caffeine is found in coffee beans in small quantity, ranging from ca. 9.0–13.0 g kg−1 dw in Coffea arabica and ca. 15.0–25.0 g kg−1 dw in Coffea canephora (Worku, Citation2019); hence, its concentration in C. arabica is about half of that found in C. canephora. As indicated in Table , the average caffeine contents reported for Ethiopian coffee from four coffee regions are comparable to those reported for arabica coffees by Farah (Citation2012). The four regional coffees and the seven coffee types (i.e., Harar, Sidamo, Yirgacheffe, Jimma, Kaffa, Wellega and Gojam coffees) have different levels of caffeine. For example, caffeine content was significantly higher in Gojam coffee (a coffee from Northwestern region) than in the other coffee types. Harar coffee was found to contain lower levels of caffeine; however, the difference is significant only when compared to that of Jimma, Wellega and Gojam coffees (Mehari et al., Citation2016b). Worku et al. (Citation2023a) also report lower caffeine content for Harar and Southern (Yirgacheffe) coffees (average 12.0 mg g−1 dw) than for Southwestern, Western (Wellega) and Northwestern coffees (average 13.2, 14.6 and 14.5 mg g−1 dw, respectively). Caffeine content in green beans of Ethiopia also varies with the genotype (variety) (Girma et al., Citation2020; Dessalegn et al., Citation2007; Mengistu et al., Citation2020; Weldemichael et al., Citation2020; Wondimkun et al., Citation2022), growing environment (e.g., elevation and shade) and postharvest processing method of coffee (Tolessa et al., Citation2017; Tolessa, Citation2017; Worku et al., Citation2018, Citation2023b). These studies, however, report different effects of elevation, shade and postharvest processing on caffeine. Studies conducted elsewhere on the effects of elevation and shade on caffeine content of coffee beans also report contradictory results (Avelino et al., Citation2007; Vaast et al., Citation2005). This can be explained by site specificity or growing condition (e.g., micro-climate, season), agronomic practice or postharvest processing dependency of caffeine content of coffee beans.
Table 2. Chemical composition of green C. arabica beans growing in four coffee regions of Ethiopia; author’s computation based on own data and data from Farah (Citation2012), Habte et al. (Citation2016), Mehari et al. (Citation2016b, Citation2016a, Citation2016c), Tolessa et al. (Citation2017), Tolessa (Citation2017) and Mehari et al. (Citation2019)
5.2. Trigonelline content
Trigonelline (N-methylpyridinium-3-carboxylate) is an alkaloid biologically derived from enzymatic methylation of nicotinic acid (Farah, Citation2012). It is the second most abundant alkaloid in coffee beans next to caffeine (Cheng et al., Citation2016). Yet, unlike caffeine, C. arabica contains higher levels of trigonelline than C. canephora with 0.6–2.0 and 0.6–0.7 g kg−1 dw, respectively (Worku, Citation2019). Trigonelline contributes to the bitterness of the brew and is an aroma precursor that contributes to the desirable flavor products formed during coffee roasting, including pyrazine, furans, alkyl-pyridines and pyrroles (Clifford, Citation1985; Dart & Nursten, Citation1985; De Maria et al., Citation1996; Farah, Citation2012). Therefore, high trigonelline levels in coffee beans are strongly correlated with good quality (Farah et al., Citation2006).
Depending on the roasting temperature (e.g., at 220°C for 20 min.), trigonelline rapidly decomposes during roasting (Cheng et al., Citation2016) and the roasting process causes a significant decrease in trigonelline content (Farah et al., Citation2006). Trigonelline de-methylation during roasting produces a water-soluble B-complex vitamin, nicotinic acid also known as niacin, which is bioavailable in coffee beverages compared with other natural sources in bound forms (Trugo, Citation2003). According toTeply and Prier (Citation1957), coffee consumption of 3.5 standard (250 ml) cups per day accounts for one-third of the minimum dietary nicotinic acid requirement for an adult, showing its high potential to fulfill our dietary nicotinic acid requirement. Regarding the bioactivity potential of trigonelline, it can inhibit the invasiveness of cancer cells (Hirakawa et al., Citation2005) and regenerate dendrites and axons, suggesting that it may improve memory (Tohda et al., Citation2005). Trigonelline has a therapeutic potential for diabetes and central nervous system disease, and antioxidant and antimicrobial activities (Zhou et al., Citation2012, Citation2013). It has also been considered as a novel phytoestrogen (Allred et al., Citation2009).
The average trigonelline contents in Ethiopian green coffee beans from four coffee regions (Table ) are within the mid-concentration range indicated for green arabica coffee beans (Farah, Citation2012). Different levels of trigonelline have also been found for the four regional coffees and the eight coffee types (i.e., Harar, Sidamo, Yirgacheffe, Jimma, Kaffa, Wellega and Gojam coffees). Kaffa coffee (a coffee that is produced in Kaffa area of Southwestern region), for example, was found to have a significantly lower content of trigonelline than the other coffee types, with the exception of Jimma and Wellega coffees (Mehari et al., Citation2016b). As reported by different studies elsewhere in the world (Duarte et al., Citation2010; Odeny et al., Citation2014; Vaast et al., Citation2005, Citation2006), trigonelline content of Ethiopian coffee, such as caffeine content, can also vary with coffee genotype (variety), growing environments (e.g., elevation, shade, soil or climate) or postharvest processing method. However, to our knowledge, except some studies (e.g.,Dessalegn et al., Citation2007; Mengistu et al., Citation2020; Wondimkun et al., Citation2022) that report the effects of genotype and postharvest method, studies on the effects of these factors on trigonelline content in Ethiopian coffee are limited.
5.3. Chlorogenic acids content
Chlorogenic acids (CGA) comprise a major class of phenolic compounds, which are derived primarily from esterification of trans-cinnamic acids (e.g., caffeic, ferulic and p-coumaric acids) with quinic acid. The main subclasses of CGA in green coffee beans are caffeoylquinic acids (CQA), dicaffeoylquinic acids (diCQA), feruloylquinic acids (FQA), p-coumaroylquinic acids (pCoQA) and caffeoyl-feruloylquinic acids (CFQA). Apart from CFQA, which contains six major isomers, each of these subclasses consists of at least three major positional isomers (i.e., 3-, 4- and 5-CQA; 3,4, 3,5 and 4,5diCQA; 3-, 4- and 5-FQA; and 3-, 4- and 5-pCoQA) (Farah, Citation2012; Scholz et al., Citation2018). Among these classes, CQA account for ca. 80% of the total CGA content and 5-CQA in particular accounts for almost 60% of the total CGA, whereas pCoQA and CFQA are less abundant (Farah & Donangelo, Citation2006; Farah et al., Citation2008; Farah, Citation2012). The content of CGA in C. arabica is generally 1.5–2 times lower than that in C. canephora (Worku, Citation2019).
CGA are one of the major chemical constituents of green coffee beans, which determine cup quality, mainly the brew’s astringency, bitterness and acidity (Farah, Citation2012). Particularly, 3,4diCQA levels in coffee beans are strongly correlated with high quality. On the other hand, high amounts of CGA in green coffee beans, particularly CQA (predominantly 5-CQA), FQA and their oxidation products are associated with poor cup quality and off-flavor (Farah et al., Citation2006). Nevertheless, the impact of the CGA isomers on the formation of the quality of the beverage can be dependent on combination with the concentration of other compounds, as proteins, lipids or sucrose to CGA isomers (Scholz et al., Citation2018). CGA are also precursors of phenols and cathecols that may confer unpleasant sensory notes that are formed during roasting (Trugo, Citation2003). Lower levels of CGA also appear to explain the superiority of C. arabica in beverage quality when compared with C. canephora. The large difference in CGA contents of these two species was considered as one of the factors responsible for flavor difference between these coffees (Farah & Donangelo, Citation2006). Moreover, studies have demonstrated several health benefits of CGA; e.g., reduction of risk factors for type 2 diabetes and cardiovascular diseases (Farah, Citation2012; Godos et al., Citation2014) and mitigating oxidative and inflammatory stresses (Liang & Kitts, Citation2016). These beneficial properties of CGA attribute to their potent antioxidant activities (Bhattacherjee & Datta, Citation2015; Shearer et al., Citation2007). Many other studies have shown that CGA and their metabolites have antimutagenic, hypoglycemic, hepatoprotective, immunostimulatory and antiviral activities (Farah & Donangelo, Citation2006; Farah, Citation2012). For the coffee plant itself, CGA are significant plant metabolites that are associated with the protection of plant cells against stress; for example, oxidative stress, UV irradiation and pathogen infection (Cheng et al., Citation2016).
CGA are thermally unstable; hence, roasting of green coffee beans degrades CGA, which results in up to about 61%, 93% and 97% losses of CGA for light, dark and very dark roasting, respectively (Farah et al., Citation2006; Trugo & Macrae, Citation1984). Although most of CGA are lost by roasting, a sharp increase in total antioxidant activity was reported in the coffee beverage that suggests that the breakdown products of CGA are antioxidants in humans (Upadhyay & Mohan Rao, Citation2013). In addition, during roasting of coffee beans, volatile phenols (e.g., guaiacol and 4-vinylguaiacol) from CGA degradation and chlorogenic lactones that may be formed from CGA and quinic acid contribute to increased aroma and bitterness of the coffee brew, respectively (Sunarharum et al., Citation2014).
The average CGA contents across regional green coffee beans in Ethiopia (Table ) are within the concentration range indicated for green arabica coffee beans in Farah (Citation2012). The concentrations of CGA of green coffee beans from four coffee regions of Ethiopia are given in . According to Mehari et al. (Citation2016a), the four regional coffees have been found to contain statistically similar levels of total CGA. The mean total CGA contents across the regional green coffee beans range from 60 to 64 mg g−1 dw. However, the amounts of the various individual CGAs (except 5-pCoQA) have been found to differ significantly among the regions (). Joet et al. (Citation2010) also found similar results for green arabica coffee beans from different locations in Reunion Island. However, a recent study in Ethiopia (Worku et al., Citation2023a) shows a higher total CGA content for Harar coffee than for the other region coffees (). This study also reports slightly higher 3,5diCQA, 4,5diCQA, FQA and CFQA contents for Harar coffee, but slightly lower 4-CQA, 3,5diCQA, 4,5diCQA and CFQA contents for Western (Wellega) coffee than the other region coffees. Mehari et al. (Citation2016a) also report significantly higher amounts of 3,4diCQA, FQA and 4,5diCQA for Harar coffee than Southwestern coffee, Southwestern and Northwestern coffees and other region coffees, respectively. On the other hand, they report higher amounts of 3-CQA and 4-CQA for Northwestern coffee, and higher amounts of 5-CQA and 3,5diCQA and lower levels of 4,5diCQA for Southwestern coffee than the other region coffees. However, both studies report lower amounts of 5-CQA for Harar coffee than the other region coffees. The higher 3,4diCQA content and the lower 5-CQA content in Harar coffee may contribute towards the much appreciated cup quality characteristics of this coffee. As indicated above, the presence of high concentrations of 3,4diCQA in green coffee beans is strongly associated with higher beverage quality, but that of 5-CQA with lower beverage quality (Farah et al., Citation2006). Among the determined CGA in both studies, 5-CQA is the most abundant in the green coffee beans, followed by 3,5diCQA and 4-CQA (). The concentration of 5-CQA in Ethiopian green coffee beans constitutes 46–59% of the total CGA, which is within the range of literature values indicated for the same coffee of the other countries (Duarte et al., Citation2010; Farah & Donangelo, Citation2006).
Table 3. The concentrations of chlorogenic acids (CGA) in green C. arabica beans from the four coffee regions of Ethiopia; CQA = caffeoylquinic acid; FQA = feruloylquinic acid; pcoqa = p-coumaroylquinic acid; CFQA = caffeoyl-feruloylquinic acids
Studies also show different contents of CGA in Ethiopian coffee for different growing localities (sub-regions) or coffee types (e.g., Sidamo, Yirgacheffe, Jimma, Kaffa and Wellega coffees) (Mehari et al., Citation2016a;Worku et al., Citation2023a), growing environments (e.g., elevation and shade), postharvest processing methods (Tolessa et al., Citation2017;Tolessa, Citation2017;Wondimkun et al., Citation2022;Worku et al., Citation2018,Citation2023b) and varieties or genotypes (Dessalegn et al., Citation2007;Mengistu et al., Citation2020;Weldemichael et al., Citation2020;Wondimkun et al., Citation2022). It can also be varied with agronomic practices; e.g., plant density and planting pattern, as observed in Londrina, Brazil (Scholz et al., Citation2018). However, the effects of agronomic practices and coffee production systems (which vary in management intensity) on contents of CGA and other chemicals in Ethiopian coffee have not been studied so far.
5.4. Sucrose content
Sucrose is one of the most abundant carbohydrates present in green coffee beans. It accounts for more than 90% of the total low-molecular-weight carbohydrates present in green coffee beans and represents a source of energy that is easily mobilized during germination (Farah et al., Citation2006). Sucrose is a precursor for the Maillard reaction (after inversion) and caramelization, which are important for the development of the color and aroma of the coffee brew (Farah, Citation2012; Flament, Citation2002). It also contributes to the acidity of the brew after coffee roasting (Farah, Citation2012). In the Maillard reaction, sucrose reacts with proteins, amino acids and peptides (Farah, Citation2012; Flament, Citation2002). Sucrose accounts for up to 9% of C. arabica dry weight and approximately half of it in C. canephora (Worku, Citation2019). Hence, higher sucrose content can be one of the reasons for the superior aroma and overall flavor of C. arabica compared to C. canephora. Scholz et al. (Citation2018) also reported the dependency of beverage sensory attributes on the concentrations of sucrose, lipids and proteins in combination to CQA isomers in coffee beans. However, as opposed to what are reported by Worku et al. (Citation2023b) and Scholz et al. (Citation2018), Farah et al. (Citation2006) show no correlation between sucrose content and cup quality. As an aroma precursor, sucrose degrades rapidly during roasting and forms volatile (aroma) and non-volatile compounds, such as furans, pyrazine, aliphatic acids and hydroxymethyl furfural through Maillard reaction (Cheng et al., Citation2016). As a consequence of Maillard and caramelization reactions, sucrose content considerably decreases during the roasting process; for example, an average loss of 98% was reported for the dark roasted beans (Farah et al., Citation2006).
The mean sucrose contents in Ethiopian green coffee beans from four coffee regions (Table ) are lower than the concentration range indicated for green arabica coffee beans in the world (Farah, Citation2012;Table ). However, the mean value of sucrose content (65.0 g kg−1 dw) in Southwestern coffee, excluding Wellega, which has been obtained by calculating the results of the three different studies (Worku, Citation2019) is higher than these values (Table ), but within the lower range of values indicated for green arabica coffee beans by Farah (Citation2012) or in the world (Table ). As per the results of a recent study (Worku et al., Citation2023a), the mean sucrose content of Harar coffee is slightly higher than that of the other region coffees (Table ). Sucrose content in green coffee beans of Ethiopia also varies according to genotype (Dessalegn et al., Citation2007), growing environments (e.g., elevation and shade) and postharvest processing methods used to produce green beans (Tolessa, Citation2017;Worku et al., Citation2018). It can also be varied with agronomic practices, such as plant density, as observed in Londrina, Brazil, by Scholz et al. (Citation2018).
5.5. Fatty acids content
Fatty acids (FA) in coffee beans are found primarily in combined forms; most are esterified with glycerol in the triacylglycerol fraction, some 20% esterified with diterpenes and a small proportion in sterol esters. Triacylglycerols, esters of diterpene alcohols and FA, esters of sterols and FA, and free FA constitute 75.2%, 18.5%, 3.2% and 1% of the total lipid fraction of green coffee beans, respectively (Farah, Citation2012; Speer & Kölling-Speer, Citation2006). The remaining lipid fractions include unesterified sterols (2.2%) and tocopherols (0.05%). Lipids are the major components of coffee beans, and the total lipid content in C. arabica beans (ca. 15.5–18.2 g 100 g−1 dw) is nearly two times that of C. canephora beans (ca. 7.2–10.8 g 100 g−1 dw) (Farah, Citation2012). Most lipids are located in the endosperm of green coffee seeds, but the coffee wax that accounts for 0.2–0.3% of the coffee seed’s weight is located in the outer layer (Speer & Kölling-Speer, Citation2006).
There are more than 32 FA in coffee beans (Badmos, Citation2020) and most of them are unsaturated (USFA), accounting for 51.7–54.3% of the total FA of green arabica coffee beans and consisting of both monounsaturated FA (MUSFA) and polyunsaturated FA (PUSFA) (Joet et al., Citation2010;Zhu et al., Citation2021). Oleic acid (C18:1), linoleic acid (C18:2), linolenic acid (C18:3) and gondoic acid (C20:1) are the main USFA found in coffee beans. Linoleic acid (18:2), oleic acid (18:1) and linolenic acid (18:3) account for about 43–54%, 7–14% and 1–2.6% of the triacylglycerol fraction, respectively, and about 46%, 11% and 1% of the free FA fraction, respectively (Farah, Citation2012). The saturated FA (SFA) content of green arabica coffee beans ranges from 45.5% to 48.9% of the total FA (Dong et al., Citation2021; Joet et al., Citation2010) and this is much higher than those of red pepper and Echium seed oils (Dong et al., Citation2021). Myristic acid (C14:0), palmitic acid (C16:0), margaric acid (C17:0), stearic acid (C18:0), arachidic acid (C20:0), behenic acid (C22:0) and lignoceric acid (C24:0) are the major SFA found in coffee beans. The order of the three groups of FA based on their concentrations in green arabica coffee beans is shown as PUSFA > SFA > MUSFA (Dong et al., Citation2021; Zhu et al., Citation2021). Considering both USFA and SFA, linoleic acid (18:2) and palmitic acid (C16:0) are the major FA found in green arabica coffee beans, ranging from 40.7% to 43.8% and 36.6–38.7%, respectively, followed by oleic acid (C18:1) (8.5–9.9%) and stearic acid (C18:0) (6.7–7.1%). The two major FA account for about 77.2–82.0% of the total lipid fraction of green arabica coffee beans (Dong et al., Citation2021).
Lipids in coffee beans serve as carriers of flavors and fat-soluble vitamins and contribute significantly to organoleptic quality, such as texture and mouthfeel, of the beverage (Romano et al., Citation2014). Coffee oil also prevents volatilization and loss of flavor during the roasting process (Wagemaker et al., Citation2011) by dissolving volatile components and gases generated by roasting and protecting them from heat degradation (Koshima et al., Citation2020). FA keep coffee fresh and avoid the staleness caused by hydrolysis and oxidation of triacylglycerols (Farah, Citation2012). Moreover, according to Figueiredo et al. (Citation2015), SFA, such as arachidic, stearic and palmitic acids, are potential discriminators of the quality of specialty coffees, indicating better sensory quality, whereas USFA, such as elaidic, oleic, linoleic and linolenic acids, may be related to coffees with less intense acidity, fragrance, body and flavor. PUSFA also negatively impact coffee quality because the double bonds are easily broken down, promoting the formation of undesired odor compounds (Cong et al., Citation2020). Similarly, short-chain FA (acetic, propanoic, butanoic; pentanoic, hexadecanoic, heptadecanoic and ocadecanoic acids) in green coffee beans may give the coffee beverage an unpleasant odor and taste. However, as these FA are found in low concentrations both in green and roasted coffee beans, they do not influence the aroma or the odor of the drink (Pinheiro et al., Citation2021).
Fatty acids in coffee beans are not only important for cup quality but also for health and formulating food and cosmetic products. Cafestol and kahweol, which are the primary diterpene components in arabica coffee beans and mainly esters of various FA, are receiving a significant attention due to their demonstrated emollient properties, ability to increase serum cholesterol level and block solar radiation, and potential anticarcinogenic and hepatoprotective properties (Cavin et al., Citation2002; Farah, Citation2012). Coffee oil, being rich in USFA with a high sun protection factor value is also suited for formulating high-quality cosmetic products that are able to promote moisture retention and protect the skin against UVB radiation (Wagemaker et al., Citation2011). Besides coffee oil could be used in food formulations since it has a unique flavor and nutritional properties, such as high oxidant activity (Calligaris et al., Citation2009; Dong et al., Citation2021).
The lipid fraction, including triacylglycerols and sterols, is relatively heat stable. But, diterpenes and tocopherols are more sensitive to heat; for example, depending on the degree of roasting, tocopherols may be reduced by 83–99% (Speer & Kölling-Speer, Citation2006). The FA composition of coffee oil is also not significantly affected by heat during roasting and hot water extraction (Calligaris et al., Citation2009; Koshima et al., Citation2020). This indicates that coffee roasting and extraction conditions for beverage processing are not important factors for coffee FA composition and that coffee residue is a resource rich in coffee oil. This oil can be utilized for food flavoring in low-quality coffee, confectioneries and dairy products (Koshima et al., Citation2020). The effective use of coffee oil in the residue could provide economic and environmental benefits.
Although most coffee lipids are heat stable, they are much affected by storage. As per Speer and Kölling-Speer (Citation2006), the FA contents in green coffee beans increase during storage due to increase in the activity of fat-splitting enzymes (e.g., lipase) and all the different FA esters hydrolyze to the same degree. For example, the contents of free FA of a fresh Brazilian coffee prepared both in a wet and dry manner, was 1 g kg−1, while that of the 10-year-old coffee was 30 g kg−1. However, according to Moon et al. (Citation2022), the changes in the FA contents of green coffee beans during storage depend on FA and storage conditions, such as storage temperature and moisture. For example, during accelerated storage [i.e., a storage with higher temperature (30–50 ◦C) and relative humidity (50%)], the amount of USFA decreased, while that of SFA increased. Hexanal and some other aldehydes derived from the oxidation of PUSFA, such as linoleic acid, show exponential increases in coffees stored under oxidizing atmospheres. Hexanal can give a rancid taste to coffee and it is suggested as a marker compound for coffee storage (Makri et al., Citation2011). Changes in coffee FA contents during storage are strongly affected by bean moisture, storage moisture and temperature, or storage duration. This is high for high moisture and temperature conditions, and longer storage time (Moon et al., Citation2022; Speer & Kölling-Speer, Citation2006). Higher temperatures and longer storage time lead to a higher degree of lipid oxidation and quality change of green coffee beans. Due to the oxidation reaction during accelerated storage, longer storage caused a reduction in stearic acid (C18:0), oleic acid (C18:1) and linoleic acid (C18:2), with an increase in palmitic acid (C16:0) and arachidic acid (C20:0) (Moon et al., Citation2022).
The FA compositions of coffee beans also vary with geographical origin (Bertrand et al., Citation2008; Mehari et al., Citation2019), growing environment (e.g., temperature and elevation) (Figueiredo et al., Citation2015; Joet et al., Citation2010; Tsegay et al., Citation2020; Villarreal et al., Citation2009), genetic traits (Bertrand et al., Citation2008; Figueiredo et al., Citation2015; Villarreal et al., Citation2009) and coffee management practices, such as postharvest processing (Joet et al., Citation2010). Mehari et al. (Citation2019) report a variation between the coffee origins and 21 different FA (12 saturated and 9 unsaturated FA) in all of the green bean samples of arabica coffee (n = 100) collected from four coffee regions of Ethiopia. In this study, the total FA content varies from 83 to 204 g kg−1 dw across the regions, and linoleic acid and palmitic acid are the most abundant FA, accounted for 48–57% and 26–33%, respectively, of the total FA content (Table ). These two FA together comprise 79–85% of the total FA. Comprising 77.4–88.4% of the total FA, linoleic acid and palmitic acid are also the major FA in green coffee beans from the Southern and Southwestern regions (Tsegay et al., Citation2020). The other major FA reported in both studies are oleic acid and stearic acid, representing 6–10% and 4–7%, respectively (Mehari et al., Citation2019), and 6.2–7.5% and 8.2–10.5%, respectively, (Tsegay et al., Citation2020) of the total FA. The maximum values of linoleic and palmitic acids reported in both studies (85% and 88.4%) are slightly higher than those indicated for green arabica coffee beans in Speer and Kölling-Speer (Citation2006) (78–82%) and Dong et al. (Citation2021) (77.2–82.0%). The levels of linoleic acid and palmitic acid in Speer and Kölling-Speer (Citation2006) range from 52% to 54% and 26% to 28% of the total FA, respectively, and in Dong et al. (Citation2021) range from 40.7% to 43.8% and 36.6% to 38.7% of the total FA, respectively. However, unlike in these and other studies (e.g.,Joet et al., Citation2010), the levels (37.0–42.0%, average 51.6 mg g−1) of linoleic acid in Tsegay et al. (Citation2020) are lower than those of palmitic acid (40.4–46.4%, average 55.5 mg g−1). In Joet et al. (Citation2010), the major FA in green coffee beans is linoleic acid (43.6–44.0%), followed by palmitic acid (35.4–35.6%), oleic acid (7.2–7.4%), stearic acid (7.0–7.1%), arachidic acid (2.4–2.4%) and linolenic acid (1.9–1.9%). This difference could be related to the different extraction methods used by the different studies; the relative percentages of FA in the coffee beans are slightly different by the different methods (Dong et al., Citation2021). Dong et al. (Citation2021) found the lowest content of linoleic acid and the highest content of palmitic acid in the ultrasonic/microwave-assisted extraction method.
Table 4. The concentrations of fatty acids in green C. arabica beans from four coffee regions of Ethiopia; adapted from Mehari et al. (Citation2019)
The findings of Mehari et al. (Citation2019) also show a significant variation between coffees in the four coffee regions of Ethiopia. The FA concentrations of green coffee beans from four coffee regions of Ethiopia are given in . According to this study, coffee beans from the Harar region contain much higher levels of total FA and oleic acid than those from the other regions. Furthermore, the levels of oleic acid in each Harar coffee sample (13–19 g kg−1) are considerably higher than those in each sample from the other regions (7–12 g kg−1) (). This indicates that analysis of oleic acid content can be used to separate Harar coffee from the other region coffees. The levels of oleic acid in Northwestern coffee are significantly higher than those in Southern and Southwestern coffees. In addition, levels of hypogeic acid are much higher in Northwestern coffee than those in the other region coffees. However, the levels of stearic acid in Southwestern and Northwestern coffees are much lower than those in Harar coffee. The stearic acid contents in Southwestern coffee are also much lower than those in Southern coffee. Regarding the remaining FA, Harar coffee generally contained higher contents than the other region coffees (Table ).
The FA contents in Ethiopian coffee also vary with growing locality (sub-region), or coffee type (Mehari et al., Citation2019; Shiferaw, Citation2018), elevation (Tsegay et al., Citation2020) or genotype (Weldemichael et al., Citation2020). For example, the contents of oleic acid (average 17.4 g kg−1) and total FA (average 179 g kg−1) in Harar A coffee from the East were significantly higher than in Harar B coffee (average 13.3 g kg−1) and in the other coffee types (average 104–154 g kg−1), respectively. Moreover, the hypogeic acid content (average 0.49 g kg−1) of Finoteselam coffee from the Northwest was much higher than that of the other coffee types (average 0.11–0.28 g kg−1) (Mehari et al., Citation2019). Similarly, the levels of the total FA in West Gojam coffee from the Northwest (average 102.74 mg g−1) and Bure coffee from West Gojam (average 123.54 mg g−1) were much higher than those in East Gojam and Awi coffees (average 84.56 and 90.55 mg g−1, respectively) and in the coffees of other localities in the same region (78.45 − 104.88 mg g−1), respectively (Shiferaw, Citation2018). According to Tsegay et al. (Citation2020), the FA contents in green coffee beans from the Southern and Southwestern regions decrease with increasing elevations of the coffee growing areas. However, the reduction was higher for the contents of palmitic acid (R = −0.574) and linoleic acid (R = −0.506) than those of stearic acid (R = −0.430) and oleic acid (R = −0.291). Considering a negative relationship between elevation and temperature, the negative relationship between elevation and the contents of stearic acid and oleic acid reported in Tsegay et al. (Citation2020) agrees with a positive relationship between temperature and the contents of these FA, but disagrees with a negative relationship between temperature and the contents of linoleic acid and linolenic acid reported in Villarreal et al. (Citation2009). As said by these authors, high temperatures increase acyl chain elongation and low temperatures increase acyl desaturation.
5.6. Protein content
Proteins are one of the main components in coffee beans that are important for cup quality. A wide range of protein contents in coffee beans, ranging from 8.5% to 18.1% dw, has been reported in the literature (Belitz et al., Citation2009; Kitzberger et al., Citation2016; Mazzafera et al., Citation2019; Scholz et al., Citation2016; Zhu et al., Citation2021) and no significant difference seems to exist between C. arabica and C. canephora beans (Mazzafera et al., Citation2019; Zhu et al., Citation2021). However, coffee is not a good nutritional source of protein because it lacks essential amino acids (Farah, Citation2012). One-third of the total protein content of coffee beans is thought to bind to arabinogalactans (one of the polysaccharides consists of 30% of the total polysaccharides) in the cell wall, while the largest fraction of proteins remains in the cytoplasm (Mazzafera et al., Citation2019). The contents of free amino acids in coffee beans, ranging from 0.15% to 2.5% dw, are much lower than that of proteins (Mazzafera et al., Citation2019). According to Murkovic and Derler (Citation2006), alanine and asparagine are the major amino acids both in C. arabica and C. canephora beans, followed by phenylalanine, glutamine and serine. But, as per Shimizu and Mazzafera (Citation2000), asparagine, glutamic acid, aspartic acid, alanine and lysine in descending order are the major free amino acids in green arabica coffee beans, followed by serine and glutamine. In general, C. canephora beans have higher levels of all free amino acids than C. arabica beans, except for glutamate (Farah, Citation2012), proline, histidine, glutamine and serine (Murkovic & Derler, Citation2006). Despite that, free amino acids are the single most important group of compounds in coffee beans related with the development of coffee flavor (Mazzafera et al., Citation2019).
Ludwig et al. (Citation1995) fractionated the soluble proteins of coffee seeds in to albumin (15%) and globulin (85%) type proteins, and the amino acid profile of the water-soluble proteins (albumin fraction) is similar to that of the total proteins. An 11S-globulin seed storage protein is the most abundant protein in coffee beans, accounting for approximately 45% of the total soluble proteins in coffee endosperm (Mazzafera et al., Citation2019). However, in a 2D electrophoresis, Rogers et al. (Citation1999) also found the 11S protein (α- and β-subunits or isoforms) in the embryo. This protein has a storage function and is degraded during germination; consequently, its level declines during germination (Mazzafera et al., Citation2019). Glutamate/glutamine and aspartate/asparagine are the main amino acids of the 11S storage protein and the total soluble protein fraction of coffee beans. However, sulfur-containing amino acids, such as cysteine and methionine, are presented in low amounts in the 11S storage and total proteins of coffee beans (Mazzafera et al., Citation2019). According to Rogers et al. (Citation1999), cysteine and methionine represent only 1.1% and 0.2%, respectively, of the 20 amino acids coded by csp1. However, it is indicated that these amino acids are important for taste and aroma development of coffee beverage during coffee roasting (Mazzafera et al., Citation2019).
Over the past 50 years, several reports on the protein and/or amino acid content and profile of coffee beans have consistently confirmed the importance of these compounds for coffee flavor; however, despite the improvement of the techniques and scientific approaches used in these studies, all of these studies failed to clearly identify their relative importance (proportion and concentration) (Mazzafera et al., Citation2019). Amino acids, peptides and proteins play an important role in coffee aroma production because they interact with sugars during roasting by the Maillard reaction (Farah, Citation2012). During the roasting process, proteins can also react with galactomannans (the most abundant polysaccharides of green coffee beans) through Millard reaction, and these reactions not only contribute to coffee aroma but also to beverage viscosity (Bastian et al., Citation2021). In addition, the enzyme polyphenol oxidase oxidizes chlorogenic acids to quinones, which in turn react with proteins, affecting the final bean quality and consequently the beverage (Campos et al., Citation2022; Mazzafera et al., Citation2019). However, there is limited information regarding bioactivity of proteins and peptides in coffee beans. Farah (Citation2012), for example, listed several bioactive components of coffee, including caffeine, trigonelline, chlorogenic acids, terpenes, fibers, lipids, minerals, carbohydrates and other compounds but proteins and peptides were not included. However, some recent studies (e.g.,Ribeiro et al., Citation2021; Samsalee & Sothornvit, Citation2021; Valdés et al., Citation2020) reported the high anti-hypertensive and antioxidant potentials of protein extracts and bioactive peptides from green coffee beans and spent coffee grounds. It is also indicated that besides, chlorogenic acid and caffeine, tryptophan (an amino acid that is present in green and roasted coffee beans) is one of the major bioactive compounds in coffee (Bastian et al., Citation2021). Tryptophan has various biological effects on the human body, including altering mood, cognition, sleep, aggressiveness, vomiting and other physiological processes (Young, Citation2013). Mood, the immune system, cardiovascular disease and other conditions could be affected by drinking coffee due to the tryptophan content (Samsalee & Sothornvit, Citation2021). Therefore, green coffee beans are highly recommended as a source of free and protein-bound tryptophan (Martins & Glória, Citation2010). Yet, Comai et al. (Citation2007) reported that tryptophan is a minor amino acid in the human diet. Moreover, tryptophan is a minor amino acid in coffee beans (Murkovic & Derler, Citation2006).
The coffee protein content is modified during coffee roasting and brewing. Coffee roasting before brewing significantly alters the protein profile and under the temperatures used to roast coffee not only proteins are destroyed, entirely or partially, but peptides are released (Mazzafera et al., Citation2019). Bhattarai et al. (Citation2022) reported the slightly but significantly higher protein levels in green coffee than in roasted coffee and in roasted coffee than in spent coffee. Some other studies (Ballesteros et al., Citation2014; Franca et al., Citation2005) also reported similar results. This indicates that some proteins are lost from the green coffee beans during roasting and from the roasted coffee beans into the coffee beverage during brewing. However, higher amount of protein exist in spent coffee (15.1–17.4 g 100 g−1 dw), which can be used for protein concentrate production (Bhattarai et al., Citation2022). The protein fraction in coffee beans is also modified during postharvest treatment prior to roasting (Bastian et al., Citation2021), but it seems not during storage (Mazzafera et al., Citation2019). For example, a germination process occurs during wet processing, which increases the quantity of amino acids, while the dry process induces an increase in non-protein amino acid γ-aminobutyric acid (Bytof et al., Citation2005; Selmar et al., Citation2005). Campos et al. (Citation2022) also reported that recirculation of water during wet processing leads to modification of amino acids. The increase in the quantity of amino acids (the higher contents of free amino acids, such as glutamate, aspartate and alanine) in wet processing is related to the protein breakdown that creates substrates for the germination process (Bytof et al., Citation2005; Semir et al., 20008). Conversely, it is shown that although the proteolytic activity, which can be triggered due to the 11S protein degradation during germination (Shimizu & Mazzafera, Citation2000), is stable for months in stored coffee beans, either the total content of protein amino acids or the concentration of most individual amino acids, except a decrease in glutamine, is not significantly changed during storage (Selmar et al., Citation2008). However, several factors prior to post-harvest processing and storage, including genetic traits, maturity of the beans at harvest, agricultural practices (pruning, fertilization), growing environments (soil, elvation, shade and climate) and origin significantly affect the protein content of green coffee beans (Bastian et al., Citation2021;Scholz et al., Citation2016).
Concerning the protein content of Ethiopian coffee, to my best knowledge, it is not studied so far and as a result, there is not enough information. However, some studies that compared the chemical composition of coffee beans from different geographical origins reported certain protein values for Ethiopian coffee. Zhu et al. (Citation2021) is one of such studies that compared chemical composition of green arabica coffee beans from Brazil (n = 7), Colombia (n = 7), Ethiopia (n = 5), Guatemala (n = 5), Honduras (n = 7), Indonesia (n = 5), Kenya (n = 6) and China (n = 8). The protein content of Ethiopian coffee reported in this study ranges from 14.01 to 14.13 g 100 g−1 dw, with an average value of 14.06 g 100 g−1 dw. It was lower than the values of Guatemalan, Chinese and Brazilian coffees (average 14.53–15.27 g 100 g−1 dw) and higher than that of Kenyan coffee (average 13.34 g 100 g−1). But, there was no obvious difference between the protein content of Ethiopian coffee and those of Colombian, Indonesian and Honduran coffees (average 13.60–13.85 g 100 g−1 dw). The protein content that ranges from 12.33 to 16.78 g 100 g−1 dw with an average value of 14.29 g 100 g−1 dw is also reported by Scholz et al. (Citation2016) for wild Ethiopian arabica coffee accessions (n = 18) that are originated from east (n = 5) and west (n = 13) of the Rift Valley in Ethiopia and maintained in Londrina, Brazil. Accessions were also varied in their protein, caffeine, CGA, sucrose, lipids, cafestol and kahweol contents. The results of this study and other studies (Mehari et al., Citation2016c, Citation2016a, Citation2016b, Citation2016c, Citation2019) indicate that the protein content of Ethiopian coffee can also vary withgenotype (variety) and growing region or locality of coffee.
5.7. Mineral content
Minerals (elements) are about 5% of the total weight of coffee beans (Pohl et al., Citation2013), which appears to vary slightly between C. arabica (30–42 g kg−1) and C. canephora (44–45 g kg−1) (). Over 40 different elements, namely Ag, Al, As, B, Ba, Be, Bi, Br, Ca, Cd, Ce, Co, Cr, Cs, Cu, Eu, Fe, Ga, Ge, Hg, Hf, Ho, In, Ir, K, La, Li, Mg, Mn, Mo, Na, Nb, Nd, Ni, P, Pb, Rb, S, Sc, Se, Si, Sn, Sr, Tl, U, V, Y, Zn and Zr, can be found in green coffee beans (Habte et al., Citation2016; Mehari et al., Citation2016c; Pohl et al., Citation2013). According to their concentration, these elements can be categorized into three groups: major elements or macronutrients (Al, Ca, Fe, K, Mg, Na, P, S), minor elements or micronutrients (B, Cu, Mn, Ni, Rb, Sr, Zn) and trace elements (the remaining ones) (Habte et al., Citation2016). Among the major elements, K (12.1–21.4 g kg−1), P (1.41–2.20 g kg−1) and Mg (0.14–2.09 g kg−1) are the most abundant elements, followed by Ca (0.79–1.87 g kg−1), S (0.113–0.335 g kg−1), Na (0.0024–0.118 g kg−1) and Fe (0.024–0.108 g kg−1) (Habte et al., Citation2016; Mehari et al., Citation2016c; Pohl et al., Citation2013). K and P account for around 40% and 4% of the mineral composition of coffee beans, respectively (Farah, Citation2012). The order of minor elements based on their mean concentrations in green coffee beans is shown as Mn > Cu > Sr > Zn > Rb > Ni > B (Habte et al., Citation2016). Such trace elements as Ag, Bi, Eu, Ge, Hf, Ho, In, Ir, Tl and U are often present at very low levels or may not be detected in green coffee beans (Habte et al., Citation2016; Pohl et al., Citation2013). For example, Habte et al. (Citation2016) did not detect Hg in Ethiopian coffee. Hg is toxic to humans.
The presence of major elements, i.e., K, P, Mg, S, Ca and Na, in coffee is essential because these elements may expose a certain nutritional and dietary value, especially when considering a high worldwide consumption of coffee (Pohl et al., Citation2013). For example, a 240 ml cup of brewed-coffee is reported to provide 116 mg of K and 7 mg of Mg, which could contribute 1–2 and 1–5% of the recommended dietary allowance for K (4700 mg day−1) and Mg (420 mg day−1) in adult persons, respectively (Higdon & Frei, Citation2006). Coffee can also be regarded as a substantial source of some minor elements, i.e., Cr, Cu, Fe, Mn and Zn that are essential for metabolic processes in humans (Grembecka et al., Citation2007; Habte et al., Citation2016; Oliveira et al., Citation2012; Dos Santos & De Oliveira, Citation2001). Essential trace elements such as Co, Cr, Mo, Ni, Se and V are essential nutrients, which act as cofactors during metabolism and other biological processes (Habte et al., Citation2016). However, it seems that the coffee consumption contributes to a small degree to recommended dietary intakes for nutritionally and physiologically important elements (Ca, Cu, Cr, Fe, K, Mg, Mn, Ni, Sr and Zn) that can be covered from one to several percentages (Pohl et al., Citation2013).
Some studies (Habte et al., Citation2016; Mehari et al., Citation2016c; Worku et al., Citation2019) analyzed elements in Ethiopian coffee. As can be seen in, the content of the elements reported by these studies for Ethiopian green coffee and coffee from each coffee region is generally lower than the values indicated in Farah (Citation2012), but it is within the range values (14.5–28.1 g kg−1) indicated in Pohl et al. (Citation2013). As per Mehari et al. (Citation2016c), the contents of various elements of Ethiopian coffee are generally within the range reported for green arabica coffee beans from other countries; e.g., Brazil, Colombia and Hawaii. An exception is the amount of Mg reported by Bertrand et al. (Citation2008) for green beans from Colombia, which is approximately one-tenth of that found in Ethiopian coffee (Habte et al., Citation2016; Mehari et al., Citation2016c). These studies also reported the presence of significant differences between coffee regions and coffee types of Ethiopia in their mean contents of elements. Coffee beans from the southern region contained higher amounts of Cu, Fe, Mg, Mn, P, Si and S than beans from the southwestern and eastern regions (Mehari et al., Citation2016c). There is, however, inconsistency between the two studies regarding differences between coffee types. A significant difference between the coffee regions in Ba, Ca, Cr, Cu, Mn, Rb and Sr contents is also reported by Worku et al. (Citation2019). According to this study, southwestern coffee has higher (1) Rb and Sr contents compared to those coffees from the other three regions, (2) Ca and Mn contents compared to Southern and Northwestern coffees, and (3) Ba content compared to Harar and Northwestern coffees. Harar coffee also has a higher Ca and Cu contents than those of the Southern and Northwestern regions, and all other regions, respectively, but it has a lower Cr content than those of all other regions. Rb content decreased consistently from the Southwestern to Southern to Northwestern and to the Harar regions. Yet, Southern and Northwestern coffees do not significantly differ in their contents of elements, except Rb. This means that the Rb content may be used to separate Ethiopian green coffee beans based on their geographical origins or growing regions.
As reported in various studies elsewhere (Anderson & Smith, Citation2002; Bertrand et al., Citation2008;Gonzalvez et al., Citation2009; Dos Santos et al., Citation2010; Szefer, Citation2007), the content of elements in green coffee beans in Ethiopia can also vary with variety, growing environment (e.g., soil and climate) and agricultural practice. Principally, the contents of various elements in coffee have a strong correlation with the growing environment, which is closely linked with the soil characteristics (e.g., lime content, organic matter content, pH and drainage status of the soil) and climatic conditions; e.g., precipitation (Anderson & Smith, Citation2002; Bertrand et al., Citation2008; Krivan et al., Citation1993; Mehari et al., Citation2016c). However, in Ethiopia, studies on the effects of these factors on contents of elements in coffee beans are scant. This calls for future studies on mineral profiling of coffee beans grown in different growing environments and production systems (agricultural practices) in major coffee growing regions of the country.
6. Conclusion and future line of work
Through exploration of available data, this review work shows the current status and the trends of changes over time as well as information and development gaps in production, productivity and quality of Ethiopian coffee for the first time. This kind of information is vital to apply the appropriate interventions that can improve the productivity and quality of coffee in the country.
Cultivation area, production, productivity and quality of coffee in Ethiopia show different patterns over time. Over the last 60 years, coffee production, export and cultivation area in Ethiopia show a growing trend. But, the changes in productivity and quality are negligible. Besides, production (482561 t), productivity (ca. 0.7–0.8 t ha−1) and the change in production (ca. 2.6–6.7%) of Ethiopian coffee are lower compared to those of the top coffee producing countries in the world; e.g., Brazil (ca. 3009402 t, 1.7 t ha−1, 5.3%) and Vietnam (ca. 1683971 t, 2.7 t ha−1, 54.9%). As Ethiopia is expected to continue having suitable conditions for coffee production (e.g., climate, soil and genetic resource), but less usage of improved farming practices compared to these countries, using modern production practices can sizably improve the production and productivity of Ethiopian coffee. The coffee production improvement project in Ethiopia also needs to target more on productivity improvement than expanding plantations since the latter can lead to deforestation and competition for land. Moreover, field studies on productivity over the years and coffee growing conditions, such as growing region, management level/production system, shade level, elevation range and climate are required so as to determine the actual productivity and identify the appropriate interventions for each production situation in the country. The existing information on productivity, which is obtained from estimated total production and cultivation area, may not reflect the actual productivity. Moreover, the impact of climate change and expansion of chat plantation on coffee production and productivity needs a solution in order to sustain coffee production in Ethiopia.
Available information on quality and chemical composition shows that (1) the share of the top-grade (Grades 1 and 2) coffees in Ethiopia has remained lower over time, (2) some Ethiopian coffee types (e.g., Harar, Sidamo, Yirgacheffe, Limmu and Lekemt coffee) are thought to be associated with certain flavor lexicons (e.g., “Mocha” or chocolate, spice, floral, winy and fruity), and (3) quality and chemical composition of Ethiopian coffee vary with coffee origin. However, the flavor of each coffee type is not well studied and documented. Similarly, except minerals and some quality precursors (caffeine, trigonelline, CGA, sucrose and fatty acids), data on the chemical profile of Ethiopian coffee and factors contributing to variations between coffees of different geographical origins in quality and chemical composition are absent. This indicates the presence of large rooms for the quality improvement of Ethiopian coffee, which can be achieved by improving coffee-growing conditions (e.g., soils and micro-climates) and using improved agricultural practices (e.g., pruning and fertilization) and storage conditions of coffee and calls for studies on quality and chemical profiling and mapping of Ethiopian coffee, and factors contributing for variations between coffees of different origins in chemical composition and quality, e.g., genotype, soil, climate and agricultural practice.
Availability of data and materials
The author would like to confirm that there are no raw data to be available.
Acknowledgments
I acknowledge Prof. Tessema Astatkie for his support in editing the draft manuscript.
Disclosure statement
No potential conflict of interest was reported by the author.
Additional information
Funding
Notes on contributors

Mohammed Worku
Mohammed Worku (PhD) is an associate professor of Agronomy at Jimma University, College of Agriculture and Veterinary Medicine, Jimma, Ethiopia. His key research activities and interests include determinants of productivity, quality and chemical composition of coffee; identification of geographical origin of agro-products; crop ecology and cropping systems and agro-ecosystem services; and stress physiology of crops.
References
- Alemu, Z. G., Oosthuizen, K., & Van Schalkwyk, H. D. (2003). Contribution of agriculture in the Ethiopian economy: A time-varying parameter approach. Agrekon, 42(1), 29–27. https://doi.org/10.1080/03031853.2003.9523608
- Allred, K. F., Yackley, K. M., Vanamala, J., & Allred, C. D. (2009). Trigonelline is a Novel Phytoestrogen in Coffee Beans. The Journal of Nutrition, 139(10), 1833–1838. https://doi.org/10.3945/jn.109.108001
- Alonso-Salces, R. M., Serra, F., Reniero, F., & Héberger, K. (2009). Botanical and geographical characterization of green coffee (Coffea arabica and Coffea canephora): Chemometric evaluation of phenolic and methylxanthine contents. Journal of Agricultural and Food Chemistry, 57(10), 4224–4235. https://doi.org/10.1021/jf8037117
- Anderson, K. A., & Smith, B. W. (2002). Chemical profiling to differentiate geographic growing origins of coffee. Journal of Agricultural and Food Chemistry, 50(7), 2068–2075. https://doi.org/10.1021/jf011056v
- Antonio, A. G., Moraes, R. S., Perrone, D., Maia, L. C., Santos, K. R. N., Iório, N. L. P., & Farah, A. (2010). Species, roasting degree and decaffeination influence the antibacterial activity of coffee against Streptococcus mutans. Food Chemistry, 118(3), 782–788. https://doi.org/10.1016/j.foodchem.2009.05.063
- Ashihara, H., & Crozier, A. (2001). Caffeine: A well known but little mentioned compound in plant science. Trends in Plant Science, 6(9), 407–413. https://doi.org/10.1016/S1360-1385(01)02055-6
- Avelino, J., Barboza, B., Davrieux, F., & Guyot, B. (2007). Shade effects on sensory and chemical characteristics of coffee from very high altitude plantations in Costa Rica. In: 2nd International Symposium on Multi-strata Agroforestry Systems with Perennial Crops: Making ecosystem services count for farmers, consumers, and the environment, 17–21 September 2007, Turrialba, Costa Rica.
- Ayele, A., Worku, M., & Bekele, Y. (2021). Trend, instability and decomposition analysis of coffee production in Ethiopia (1993–2019). Heliyon, 7(9), e08022. https://doi.org/10.1016/j.heliyon.2021.e08022
- Badmos, S. O. (2020). Comparison of chlorogenic acid and lipid profiles in green and roasted coffee beans. PhD Thesis, Jacobs University,
- Ballesteros, L. F., Teixeira, J. A., & Mussatto, S. I. (2014). Chemical, functional, and structural properties of spent coffee grounds and coffee silverskin. Food Bioprocess Technology, 7(12), 3493–3503. https://doi.org/10.1007/s11947-014-1349-z
- Bastian, F., Hutabarat, O. S., Dirpan, A., Nainu, F., Harapan, H., Emran, T. B., & Simal-Gandara, J. (2021). From plantation to cup: Changes in bioactive compounds during coffee processing. Foods, 10(11), 2827. https://doi.org/10.3390/foods10112827
- Belitz, H. -D., Grosch, W., & Schieberle, P. (2009). Coffee, tea, cocoa. In H.-D. Belitz, W. Grosch, & P. Schieberle (Eds.), Food chemistry, 4th ed (pp. 938–951). Springer-Verlag.
- Bertrand, B., Villarreal, D., Laffargue, A., Posada, H., Lashermes, P., & Dussert, S. (2008). Comparison of the effectiveness of fatty acids, chlorogenic acids, and elements for the chemometric discrimination of coffee (Coffea arabica L.) varieties and growing origins. Journal of Agricultural and Food Chemistry, 56(6), 2273–2280. https://doi.org/10.1021/jf073314f
- Bhattacherjee, A., & Datta, A. (2015). Mechanism of antiglycating properties of syringic and chlorogenic acids in vitro glycation system. Food Research International, 77, 540–548. https://doi.org/10.1016/j.foodres.2015.08.025
- Bhattarai, R. R., Al-Ali, H., & Johnson, S. K. (2022). Extraction, isolation and nutritional quality of coffee protein. Foods, 11(20), 3244. https://doi.org/10.3390/foods11203244
- Boot, W. J. (2011). Ethiopian coffee buying manual: Practical guidelines for the purchasing and importing of Ethiopian specialty coffee beans. USAID/Ethiopia’s Agribusiness and Trade Expansion. Fintrac Inc.
- Buffo, R. A., & Cardelli-Freire, C. (2004). Coffee flavour: An overview. Flavour and Fragrance Journal, 19(2), 99–104. https://doi.org/10.1002/ffj.1325
- Bytof, G., Knopp, S. -E., Schieberle, P., Teutsch, I., & Selmar, D. (2005). Influence of processing on the generation of γ-aminobutyric acid in green coffee beans. European Food Research and Technology, 220(3–4), 245–250. https://doi.org/10.1007/s00217-004-1033-z
- Calligaris, S., Munari, M., Arrighetti, G., & Barba, L. (2009). Insights into the physicochemical properties of coffee oil. European Journal of Lipid Science and Technology, 111(12), 1270–1277. https://doi.org/10.1002/ejlt.200900042
- Campos, G. A. F., Kruizenga, J. G. K. T., Sagu, S. T., Schwarz, S., Homann, T., Taubert, A., & Rawel, H. M. (2022). Effect of the post-harvest processing on protein modification in green coffee beans by phenolic compounds. Foods, 11(2), 159. https://doi.org/10.3390/foods11020159
- Cavin, C., Holzhaeuser, D., Scharf, G., Constable, A., Huber, W. W., & Schilter, B. (2002). Cafestol and kahweol, two coffee specific diterpenes with anticarcinogenic activity. Food and Chemistry Toxicology, 40(8), 1155–1163.
- Cheng, B., Furtado, A., Smyth, H. E., & Henry, R. J. (2016). Influence of genotype and environment on coffee quality. Trends in Food Science & Technology, 57(Part A), 20–30.
- Clifford, M. N. (1985). Chemical and physical aspects of green coffee and coffee products. In M. N. Clifford & K. C. Willson (Eds.), Coffee botany, biochemistry, and production of beans and beverage (pp. 305–374). Croom Helm.
- Comai, S., Bertazzo, A., Bailoni, L., Zancato, M., Costa, C. V. L., & Allegri, G. (2007). The content of proteic and nonproteic (free and protein-bound) tryptophan in quinoa and cereal flours. Food Chemistry, 100(4), 1350–1355.
- Cong, S., Dong, W., Zhao, J., Hu, R., Long, Y., & Chi, X. (2020). Characterization of the lipid oxidation process of robusta green coffee beans and shelf life prediction during accelerated storage. Molecules, 25(5), 1157.
- Dart, S. K., & Nursten, H. E. (1985). Volatile components. In R. J. Clarke & R. Macrae (Eds.), Coffee (pp. 223–265). Springer.
- Davis, A. P. (2011). Psilanthus mannii, the type species of Psilanthus, transferred to Coffea. Nordic Journal of Botany, 29(4), 471–472.
- Davis, P. G., Schmidt, B., Roberts, R. S., Doyle, L. W., Asztalos, E., & Haslam, R. (2010). Caffeine for apnea of prematurity trial: Benefits may vary in subgroups. The Journal of Pediatrics, 156(3), 382–387.
- De Maria, C., Trugo, L., Neto, F. A., Moreira, R., & Alviano, C. (1996). Composition of green coffee water-soluble fractions and identification of volatiles formed during roasting. Food Chemistry, 55(3), 203–207.
- Demirbag, D., Ozdemir, F., & Ture, M. (2006). Effects of coffee consumption and smoking habit on bone mineral density. Rheumatology International, 26(6), 530–535.
- Dessalegn, Y., Labuschagne, M. T., Osthoff, G., & Herselman, L. (2007). Variation for green bean caffeine, chlorogenic acid, sucrose and trigonelline contents among Ethiopian arabica coffee accessions. SINET: Ethiopian Journal of Science, 30(1), 77–82.
- Diao, X., Taffesse, A. S., Yu, B., & Pratt, A. N. (2010). Economic importance of agriculture for sustainable development and poverty reduction: The case study of Ethiopia. Paper presented at Global Forum on Policies for Agricultural Development, Poverty Reduction and Food Security, 29–30 November 2010,
- Dong, W., Chen, Q., Wei, C., Hu, R., Long, Y., Zong, Y., & Chu, Z. (2021). Comparison of the effect of extraction methods on the quality of green coffee oil from arabica coffee beans: Lipid yield, fatty acid composition, bioactive components, and antioxidant activity. Ultrasonics Sonochemistry, 74, 105578.
- Dos Santos, É. J., & De Oliveira, E. (2001). Determination of mineral nutrients and toxic elements in Brazilian soluble coffee by ICP-AES. Journal of Food Composition and Analysis, 14(5), 523–531.
- Dos Santos, J. S., Dos Santos, M. L. P., & Conti, M. M. (2010). Comparative study of metal contents in Brazilian coffees cultivated by conventional and organic agriculture applying principal component analysis. Journal of the Brazilian Chemical Society, 21(8), 1468–1476.
- Duarte, G. S., Pereira, A. A., & Farah, A. (2010). Chlorogenic acids and other relevant compounds in Brazilian coffees processed by semi-dry and wet post-harvesting methods. Food Chemistry, 118(3), 851–855.
- ECX (Ethiopia Commodity Exchange). (2011). ECX quality operation manual. Addis Ababa.
- FAO. (2015). Statistical pocketbook: Coffee 2015. ( Accessed 03.02.2017). http://www.fao.org/3/a-i4985e.pdf
- Farah, A. (2012). Coffee constituents. In Y.-F. Chu (Ed.), Coffee: Emerging health effects and disease prevention, 1st ed (pp. 281–304). IFT Press.
- Farah, A., & Donangelo, C. M. (2006). Phenolic compounds in coffee. Brazilian Journal of Plant Physiology, 18(1), 23–36.
- Farah, A., Monteiro, M. C., Calado, V., Franca, A. S., & Trugo, L. C. (2006). Correlation between cup quality and chemical attributes of Brazilian coffee. Food Chemistry, 98(2), 373–380.
- Farah, A., Monteiro, M., Donangelo, C. M., & Lafay, S. (2008). Chlorogenic acids from green coffee extract are highly bioavailable in humans. The Journal of Nutrition, 138(12), 2309–2315.
- FAS, & Francom, M. G. (2017). Coffee annual report, Global Agricultural Information Network (GAIN) Report Number: ET1710, 19 May. 2017.
- Figueiredo, L. P., Borém, F. M., Ribeiro, F. C., Giomo, G. S., Taveira, J. H. S., & Malta, M. R. (2015). Fatty acid profiles and parameters of quality of specialty coffees produced in different Brazilian regions. African Journal of Agricultural Research, 10(35), 3484–3493.
- Flament, I. (2002). Coffee flavour chemistry. John Willey and Sons.
- Franca, A. S., Mendonça, J. C. F., & Oliveira, S. D. (2005). Composition of green and roasted coffees of different cup qualities. Lwt-Food Science and Technology, 38(7), 709–715.
- Gebreselassie, A., Bekele, T., Kalweit, D., & Grech, W. (2010). A review of Ethiopian agriculture: Roles, policy and small-scale farming systems. KOPIN (Koperazzjoni Internazzjonali), and Ethiopian Nongovernmental Organization Emmanuel Development Association (EDA), Addis Ababa.
- Gebreselassie, S., & Lud, E. (2007). Agricultural commercialization in coffee growing areas of Ethiopia. Ethiopian Journal of Economics, 15(1), 89–118.
- Getachew, M., Tolassa, K., De Frenne, P., Verheyen, K., Tack, A. J. M., & Hylander, K. (2022). The relationship between elevation, soil temperatures, soil chemical characteristics, and green coffee bean quality and biochemistry in Southwest Ethiopia. Agronomy for Sustainable Development, 42(4), 61.
- Girma, N. K. (2011). Marketing information operation in Ethiopia with special reference to the Ethiopia Commodity Exchange (ECX) coffee trading. MSc Thesis, Swedish University of Agricultural Sciences,
- Girma, B., Gure, A., & Wedajo, F. (2020). Influence of altitude on caffeine, 5-caffeoylquinic acid, and nicotinic acid contents of arabica coffee varieties. Journal of Chemistry, 2020(1), 3904761.
- Gizaw, N., Abafita, J., & Merra, T. M. (2022). Impact of coffee exports on economic growth in Ethiopia: An empirical investigation. Cogent Economics and Finance, 10(1), 2041260.
- Godos, J., Pluchinotta, F. R., Marventano, S., Buscemi, S., Volti, G. L., Galvano, F., & Grosso, G. (2014). Coffee components and cardiovascular risk: Beneficial and detrimental effects. International Journal Food Sciences and Nutrition, 65(8), 925–936.
- Gole, T. W. (2015). Coffee: Ethiopia’s gift to the world. In: The traditional production systems as living examples of crop domestication, and sustainable production and an assessment of different certification schemes. Environment and Coffee Forest Forum (ECFF), Addis Ababa.
- Gole, T. W., Denich, M., Teketey, D., & Vlek, P. L. G. (2002). Human impact on the Coffea arabica genepool in Ethiopia and the need for its in situ conservation. In J. M. M. Engels, V. Ramanatha Rao, A. H. D. Brown, & M. T. Jackson (Eds.), Managing plant genetic diversity (pp. 237–247). IPGRI.
- Gonzalvez, A., Armenta, S., & De La Guardia, M. (2009). Trace-element composition and stable-isotope ratio for discrimination of foods with protected designation of origin. Trends in Analytical Chemistry, 28(11), 1295–1311.
- Grembecka, M., Malinowska, E., & Szefer, P. (2007). Differentiation of market coffee and its infusions in view of their mineral composition. The Science of the Total Environment, 383(1), 59–69.
- Grosch, W. (2001). Coffee: Recent developments. In R. J. Clarke & O. Z. Vitzthum (Eds.), Chemistry III: Volatile compounds (pp. 68–89). Blackwell Science.
- Habte, G., Hwang, I. M., Kim, J. S., Hong, J. H., Hong, Y. S., & Choi, J. Y. (2016). Elemental profiling and geographical differentiation of Ethiopian coffee samples through inductively coupled plasma-optical emission spectroscopy (ICP-OES), ICP-mass spectrometry (ICP-MS) and direct mercury analyzer (DMA). Food Chemistry, 212, 512–520.
- Higdon, J. V., & Frei, B. (2006). Coffee and health: A review of recent human research. Critical Reviews in Food Science and Nutrition, 46(2), 101–123.
- Hirakawa, N., Okauchi, R., Miura, Y., & Yagasaki, K. (2005). Anti-invasive activity of niacin and trigonelline against cancer cells. Bioscience, Biotechnology, and Biochemistry, 69(3), 653–658.
- ICO. (2014). World coffee trade (1963–2013): A review of the markets, challenges and opportunities facing the sector, International Coffee Council 112th Session (pp. 1–27), 3–7 March. 2014,
- ICO. (2016). Trade statistics tables. ( Accessed 14.11.2017). http://www.ico.org/trade_statistics.asp
- ICO. (2018). Historical data on the global coffee trade. ( Accessed 09.06.2018). http://www.ico.org/new_historical.asp?section=Statistics
- Jena, P. R., Chichaibelu, B. B., Stellmacher, T., & Grote, U. (2012). The impact of coffee certification on small-scale producers’ livelihoods: A case study from the Jimma Zone, Ethiopia. Agricultural Economics, 43(4), 429–440.
- Jiang, L., Ding, Y., Jiang, F., Li, L., & Mo, F. (2014). Electrodeposited nitrogen-doped graphene/carbon nanotubes nanocomposite as enhancer for simultaneous and sensitive voltammetric determination of caffeine and vanillin. Analytica chimica acta, 833, 22–28.
- Joet, T., Laffargue, A., Descroix, F., Doulbeau, S., Bertrand, B., & de Kochko, A. (2010). Influence of environmental factors, wet processing and their interactions on the biochemical composition of green arabica coffee beans. Food Chemistry, 118(3), 693–701.
- Kandari, L. S., Yadav, H. R., Thakur, A. K., & Kandari, T. (2014). Chat (Catha edulis): A socio economic crop in Harar region, Eastern Ethiopia. SpringerPlus, 3(1), 579.
- Kitzberger, C. S. G., Scholz, M. B. S., Pereira, L. F. P., da Silva, J. B. G. D., & Benassi, M. T. (2016). Profile of the diterpenes, lipid and protein content of different coffee cultivars of three consecutive harvests. AIMS Agriculture and Food, 1(3), 254–264.
- Koshima, Y., Kitamura, Y., Islam, M. Z., & Kokawa, M. (2020). Quantitative and qualitative evaluation of fatty acids in coffee oil and coffee residue. Food Science and Technology Research, 26(4), 545–552.
- Krivan, V., Barth, P., & Morales, A. F. (1993). Multielement analysis of green coffee and its possible use for the determination of origin. Microchimica Acta, 110(4–6), 217–236.
- Kufa, T. (2012). Recent coffee research development in Ethiopia. Paper presented at the Ethiopian Coffee Export Conference: Strengthening the legacy of our coffee, 8–9 November 2012, Addis Ababa, Ethiopia.
- Lee, C. (2000). Antioxidant ability of caffeine and its metabolites based on the study of oxygen radical absorbing capacity and inhibition of LDL peroxidation. Clinica Chimica Acta, 295(1–2), 141–154.
- Liang, N., & Kitts, D. D. (2016). Role of chlorogenic acids in controlling oxidative and inflammatory stress conditions. Nutrients, 8(1), 16.
- Ludwig, E., Raczek, N. N., & Kurrock, T. (1995). Contribution to composition and reactivity of coffee protein. In: Proceedings of the 16th International Conference of ASIC (pp.359–365), Kyoto, Japan.
- Makri, E., Tsimogiannis, D., Dermesonluoglu, E. K., & Taoukisa, P. S. (2011). Modeling of Greek coffee aroma loss during storage at different temperatures and water activities. Procedia Food Science, 1, 1111–1117.
- Martins, A. C. C., & Glória, M. B. A. (2010). Changes on the levels of serotonin precursors—tryptophan and 5-hydroxytryptophan—during roasting of arabica and robusta coffee. Food Chemistry, 100, 529–533.
- Mazzafera, P., Schimpl, F., & Kiyota, E. (2019). Proteins of coffee beans: Recent advances. In A. Farah (Ed.), Coffee: Production, quality and chemistry (pp. 431–444). The Royal Society of Chemistry.
- Mehari, B., Chandravanshi, B. S., Redi-Abshiro, M., Combrinck, S., McCrindle, R., & Atlabachew, M. (2020). Polyphenol contents of green coffee beans from different regions of Ethiopia. International Journal of Food Properties, 24(1), 17–27.
- Mehari, B., Redi-Abshiro, M., Chandravanshi, B. S., Combrinck, S., Atlabachew, M., & McCrindle, R. (2016a). Profiling of phenolic compounds using UPLC-MS for determining the geographical origin of green coffee beans from Ethiopia. Journal of Food Composition and Analysis, 45(6), 16–25.
- Mehari, B., Redi-Abshiro, M., Chandravanshi, B. S., Combrinck, S., Atlabachew, M., & McCrindle, R. (2016b). Simultaneous determination of alkaloids in green coffee beans from Ethiopia: Chemometric evaluation of geographical origin. Food Analysis Methods, 9, 1627–1637.
- Mehari, B., Redi-Abshiro, M., Chandravanshi, B. S., Combrinck, S., & McCrindle, R. (2016c). Characterization of the cultivation region of Ethiopian coffee by elemental analysis. Analytical Letters, 49(15), 2474–2489.
- Mehari, B., Redi-Abshiro, M., Chandravanshi, B. S., Combrinck, S., McCrindle, R., & Atlabachew, M. (2019). GC-MS profiling of fatty acids in green coffee (Coffea arabica L.) beans and chemometric modeling for tracing geographical origins from Ethiopia. Journal the Science of Food and Agriculture, 99(8), 3811–3823.
- Mengistu, M. W., Workie, M. A., & Mohammed, A. S. (2020). Biochemical compounds of arabica coffee (Coffea arabica L.) varieties grown in Northwestern highlands of Ethiopia. Cogent Food & Agriculture, 6(1), 1741319.
- Minten, B., Tamru, S., Kuma, T., & Nyarko, Y. (2014). Structure and performance of Ethiopia’s coffee export sector. Ethiopia Strategy Support Program working paper 66, International Food Policy Research Institute (IFPRI).
- MoARD (Ministry of Agriculture and Rural Development). (2007). Agriculture marketing sector, coffee: The gift of Ethiopia to the world.
- Moat, J., Williams, J., Baena, S., Wilkinson, T., Demissew, S., & Challa, Z. K. (2017). Coffee farming and climate change in Ethiopia: Impacts, forecasts, resilience and opportunities. –summary. The Strategic Climate Institutions Programme (SCIP). Royal Botanic Gardens.
- Moat, J., Williams, J., Baena, S., Wilkinson, T., Gole, T. W., & Challa, Z. K. (2017). Resilience potential of the Ethiopian coffee sector under climate change. Nature Plants, 3(7), 1708.
- Montagnon, C. (2020). Genetic diversity analysis of 158 C. arabica samples of the Cup of Excellent 2020 in Ethiopia, RD2 Vision.
- Moon, S. A., Wongsakul, S., Kitazawa, H., & Saengrayap, R. (2022). Lipid oxidation changes of arabica green coffee beans during accelerated storage with different packaging types. Foods, 11(19), 3040.
- Murkovic, M., & Derler, K. (2006). Analysis of amino acids and carbohydrates in green coffee. Journal of Biochemical and Biophysical Methods, 69(1–2), 25–32.
- Nure, D. (2008). Mapping quality profiles of Ethiopian coffee by origin. In: G. Adugna, B. Bellachew, T. Shimber, E. Taye, & T. Kufa (Eds.), Proceedings of a National Workshop on Four Decades of Coffee Research and Development in Ethiopia (pp. 317–334), 14–17 August 2007, Addis Ababa, Ethiopia.
- Odeny, D., Chemining’wa, G., & Shibairo, S. (2014). Beverage quality and biochemical components of shaded coffee. Paper presented at the 25th International Conference of ASIC, 8–13 September 2014, Armenia - Quindío, Colombia.
- Oestreich-Janzen, S. (2010). Chemistry of coffee. ( Accessed 28.12.2017). https://booksite.elsevier.com/brochures/conap2/PDFs/Vol3_Chemistry_of_Coffee_lr.pdf
- Ogita, S., Uefugi, H., Yamaguchi, Y., Koizumi, N., & Sano, H. (2003). Producing decaffeinated coffee plants. Nature, 423, 823.
- Oliveira, M., Casal, S., Morais, S., Alves, C., Dias, F., & Ramos, S. (2012). Intra- and interspecific mineral composition variability of commercial coffees and coffee substitutes: Contribution to mineral intake. Food Chemistry, 130(3), 702–709.
- Petit, N. (2007). Ethiopia’s coffee sector: A bitter or better future? Journal of Agrarian Change, 7(2), 225–263.
- Pinheiro, P. F., Pinheiro, C. A., Osório, V. M., & Pereira, L. L. (2021). Chemical constituents of coffee. In L. L. Pereira & T. R. Moreira (Eds.), Quality determinants in coffee production (pp. 209–254). Food Engineering Series, Springer.
- Pohl, P., Stelmach, E., Welna, M., & Szymczycha-Madeja, A. (2013). Determination of the elemental composition of coffee using instrumental methods. Food Analytical Methods, 6(2), 598–613.
- Ribeiro-Alves, M., Trugo, L. C., & Donangelo, C. (2003). Use of oral contraceptives blunts the calciuric effect of caffeine in young adult women. The Journal of Nutrition, 133(2), 393–398.
- Ribeiro, E., Rocha, T. D. S., & Prudencio, S. H. (2021). Potential of green and roasted coffee beans and spent coffee grounds to provide bioactive peptides. Food Chemistry, 348 , 129061.
- Rogers, W., Bézard, G., Deshayes, A., Meyer, I., Pétiard, V., & Marraccini, P. (1999). Biochemical and molecular characterization and expression of the 11S-type storage protein from Coffea arabica endosperm. Plant Physiology and Biochemistry, 37(4), 261–272.
- Romano, R., Santini, A., Grottaglie, L. L., Manzo, N., Visconti, A., & Ritieni, A. (2014). Identification markers based on fatty acid composition to differentiate between roasted arabica and Canephora (robusta) coffee varieties in mixtures. Journal of Food Composition and Analysis, 35(1), 1–9.
- Samsalee, N., & Sothornvit, R. (2021). Physicochemical, functional properties and antioxidant activity of protein extract from spent coffee grounds using ultrasonic-assisted extraction. AIMS Agriculture and Food, 6(3), 864–878.
- Scholz, M. B. S., Kitzberger, C. S. G., Durand, N., & Rakocevic, M. (2018). From the field to coffee cup: Impact of planting design on chlorogenic acid isomers and other compounds in coffee beans and sensory attributes of coffee beverage. European Food Research and Technology, 244, 1793–1802.
- Scholz, M. B. S., Kitzberger, C. S. G., Pagiatto, N. F., Pereira, L. F. P., Davrieux, F., & Pot, D. (2016). Chemical composition in wild Ethiopian arabica coffee accessions. Euphytica, 209(2), 429–438.
- Selmar, D., Bytof, G., & Knopp, S. -E. (2008). The storage of green coffee (Coffea arabica): Decrease of viability and changes of potential aroma precursors. Annals of Botany, 101(1), 31–38.
- Selmar, D., Bytof, G., Knopp, S. -E., Bradbury, A., & Wilkens, J. (2005). Biochemical insights into coffee processing: Quality and nature of green coffees are interconnected with an active seed metabolism. In: Proceedings of the 20th International Conference of ASIC (pp. 111–119), 11–15 October 2004, Bangalore, India.
- Shearer, J., Sellars, E., Farah, A., Graham, T. E., & Wasserman, D. H. (2007). Effects of chronic coffee consumption on glucose kinetics in the conscious rat. Canadian Journal of Physiology and Pharmacology, 85(8), 823–830.
- Shiferaw, T. (2018). Profiling the fatty acid constituents in green coffee beans from some selected areas of Amhara region. MSc Thesis, Bahir Dar University,
- Shimizu, M. M., & Mazzafera, P. (2000). Compositional changes of proteins and amino acids in germinating coffee seeds. Brazilian Archives of Biology and Technology, 439(3), 1–7.
- Speer, K., & Kölling-Speer, I. (2006). The lipid fraction of the coffee bean. Brazilian Journal of Plant Physiology, 18(1), 201–216.
- Sunarharum, W. B., Williams, D. J., & Smyth, H. E. (2014). Complexity of coffee flavor: A compositional and sensory perspective. Food Research International, 62, 315–325.
- Swinnen, J. (2007). Global supply chains, standards and the poor. CABI Publishing.
- Szefer, P. (2007). Chemometric techniques in analytical evaluation of food quality. In P. Szefer & J. Nriagu (Eds.), Mineral components in foods (pp. 69–121). CRC Press—Taylor & Francis.
- Tefera, A., & Bickford, R. (2021). Coffee annual report, Global Agricultural Information Network (GAIN), report number: ET2021-0013, 14 May 2021.
- Tefera, A., & Francom, M. G. (2015). Coffee annual report, Global Agricultural Information Network (GAIN), report number: ET1514, 6 May 2015.
- Tefera, A., & Francom, M. G. (2016). Coffee annual report, Global Agricultural Information Network (GAIN), report number: ET1615, 13 June 2016.
- Tefera, A., Tefera, T., & Gray, Q. (2014). Coffee annual report. Global Agricultural Information Network (GAIN), report number: ET1402, 13 May 2014.
- Teply, L., & Prier, R. (1957). Nutrients in coffee, nutritional evaluation of coffee including niacin bioassay. Journal of Agricultural and Food Chemistry, 5(5), 375–377.
- Toci, A. T., & Farah, A. (2008). Volatile compounds as potential defective coffee seeds’ markers. Food Chemistry, 108(3), 1133–1141.
- Tohda, C., Kuboyama, T., & Komatsu, K. (2005). Search for natural products related to regeneration of the neuronal network. Neurosignals, 14(1–2), 34–45.
- Tolessa, K. (2017). Biophysical control of coffee quality: The case of Southwestern Ethiopia. PhD Thesis, Ghent University,
- Tolessa, K., D’heer, J., Duchateau, L., & Boeckx, P. (2017). Influence of growing altitude, shade and harvest period on quality and biochemical composition of Ethiopian specialty coffee. Journal of the Science of Food and Agriculture, 97(9), 2849–2857.
- Trugo, L. C. (2003). Analysis of coffee products. In B. Caballero, L. C. Trugo, & P. Finglas (Eds.), Encyclopedia of food sciences and nutrition, 2nd ed (pp. 1498–1506). Academic Press.
- Trugo, L. C., & Macrae, R. (1984). A study of the effect of roasting on the chlorogenic acid composition of coffee using HPLC. Food Chemistry, 15(3), 219–227.
- Tsegay, G., RediAbshiro, M., Chandravanshi, B. S., Ele, E., Mohammed, A. M., & Mamo, H. (2020). Effect of altitude of coffee plants on the composition of fatty acids of green coffee beans. BMC Chemistry, 14(1), 36.
- Upadhyay, R., & Mohan Rao, L. J. (2013). An outlook on chlorogenic acids occurrence, chemistry, technology, and biological activities. Critical Reviews in Food Science and Nutrition, 53(9), 968–984.
- Vaast, P., Bertrand, B., Perriot, J. -J., Guyot, B., & Genard, M. (2006). Fruit thinning and shade improve bean characteristics and beverage quality of coffee (Coffea arabica L.) under optimal conditions. Journal of the Science of Food and Agriculture, 86(2), 197–204.
- Vaast, P., Cilas, C., Perriot, J. -J., Davrieux, F., Guyot, B., & Bolaño, M. (2005). Mapping of coffee quality in Nicaragua according to regions, ecological conditions and farm management. In: Proceedings of the 20th International Conference of ASIC (pp. 842–850), 11–15 October 2004, Bangalore, India.
- Valdés, A., Castro-Puyana, M., & Marina, M. L. (2020). Isolation of proteins from spent coffee grounds. Polyphenol removal and peptide identification in the protein hydrolysates by RP-HPLC-ESI-Q-TOF. Food Research International, 137, 109368.
- Villarreal, D., Laffargue, A., Posada, H., Bertrand, B., Lashermes, P., & Dussert, S. (2009). Genotypic and environmental effects on coffee (Coffea arabica L.) bean fatty acid profile: Impact on variety and origin chemometric determination. Journal of Agricultural and Food Chemistry, 57(23), 11321–11327.
- Wagemaker, T. A. L., Carvalhoa, C. R. L., Maiaa, N. B., Baggio, S. R., & Filho, O. G. (2011). Sun protection factor, content and composition of lipid fraction of green coffee beans. Industrial Crops and Products, 33(2), 469–473.
- Weldemichael, G., Alamerew, S., Tulu, L., & Berecha, G. (2020). Genetic variability of Ethiopian coffee (Coffea arabica L.) accessions collected from East Wollega Zone for bean biochemical constituents. Ethiopian Journal of Agricultural Sciences, 30(3), 77–97.
- Wiersum, K. F., Gole, T. W., Gatzweiler, F., Volkmann, J., Bognetteau, E., & Wirtu, O. (2008). Certification of wild coffee in Ethiopia: Experiences and challenges. Forests, Trees and Livelihoods, 18(1), 9–21.
- Woldu, Z., Belew, D., & Benti, T. (2015). The coffee-khat interface in Eastern Ethiopia: A controversial land use and livelihood change scenario. Journal of Agricultural Science and Technology B, 5(3), 149–169.
- Wondimkun, Y. W., Emire, S. A., Teferra, T. F., Stoecker, B., & Esho, T. B. (2022). Influence of genotype and processing on bioactive compounds of Ethiopian specialty arabica coffee. International Journal of Food Properties, 25(1), 2574–2588.
- Workman, D. (2022). Coffee Exports by Country. ( Accessed 26.7.2022). https://www.worldstopexports.com/coffee-exports-country/
- Worku, M. (2019), Quality control, quality determinants and indication of geographic origin of Ethiopian coffee. PhD Thesis, Ghent University,
- Worku, M., Astatkie, T., & Boeckx, P. (2022). Effect of growing conditions and postharvest processing on arabica coffee bean physical quality features and defects. Heliyon, 8(1–3), e09201.
- Worku, M., Astatkie, T., & Boeckx, P. (2023a). Quality and biochemical composition of Ethiopian coffee varied with growing region and locality. Journal of Food Composition and Analysis, 115(4), 105015.
- Worku, M., Astatkie, T., & Boeckx, P. (2023b). Shade and postharvest processing effects on arabica coffee quality and biochemical composition in lowland and midland coffee-growing areas of Southwestern Ethiopia. Journal of Food Composition and Analysis, 115(4), 105027.
- Worku, M., de Meulenaer, B., Duchateau, L., & Boeckx, P. (2018). Effect of altitude on biochemical composition and quality of green arabica coffee beans can be affected by shade and postharvest processing method. Food Research International, 105, 278–285.
- Worku, M., Duchateau, L., & Boeckx, P. (2016). Reproducibility of coffee quality cupping scores delivered by cupping centers in Ethiopia. Journal of Sensory Studies, 31(5), 423–429.
- Worku, M., Upadhayay, H. R., Latruwe, K., Taylor, A., Blake, W., & Vanhaecke, F. (2019). Differentiating the geographical origin of Ethiopian coffee using XRF-and ICP-based multi-element and stable isotope profiling. Food Chemistry, 290, 295–307.
- Yeretzian, C., Opitz, S., Smrke, S., & Wellinger, M. (2019). Coffee volatile and aroma compounds – from the green bean to the cup. In A. Farah (Ed.), Coffee: Production, quality and chemistry (pp. 726–770). The Royal Society of Chemistry.
- Yifru, T. (2015). Impact of agricultural exports on economic growth in Ethiopia: The case of coffee, oilseed and pulses, MSc Thesis, Egerton University,
- Young, S. N. (2013). The effect of raising and lowering tryptophan levels on human mood and social behaviour. Philosophical Translations of the Royal Society B Biological Sciences, 368(1615), 20110375.
- Zhou, J., Chan, L., & Zhou, S. (2012). Trigonelline: A plant alkaloid with therapeutic potential for diabetes and central nervous system disease. Current Medicinal Chemistry, 19(21), 3523–3531.
- Zhou, J., Zhou, S., & Zeng, S. (2013). Experimental diabetes treated with trigonelline: Effect on β cell and pancreatic oxidative parameters. Fundamental & Clinical Pharmacology, 27(3), 279–287.
- Zhu, M., Long, Y., Ma, Y., Chen, Y., Yu, Q., & Xie, J. (2021). Comparison of chemical and fatty acid composition of green coffee bean (Coffea arabica L.) from different geographical origins. LWT - Food Science and Technology, 140(5), 110802.