Abstract
Bioassay-guided fractionation of the crude extracts of Moringa oleifera Lam. using in-vitro angiotensin-converting enzyme inhibition assay was employed to purify the two compounds, namely: quercetin-3-O-glucoside (1) and kaempferol-3-O-glucoside (2). Compound 1 has a significant % of inhibition at varying concentrations: 7 ug/ml (56.37 ± 0.0059%), 15 ug/ml (59.16 ± 0.0137%) and 28 ug/ml (75.74 ± 0.0161%). The methanol extract was found to contain 180.15 ug/ml of compound 1 for every 2 mg/ml of methanol extract after standardization. Furthermore, oral administration of M. oleifera crude extracts did not present acute toxicity in mice. Methanolic and ethyl acetate extracts have significantly reduced systolic blood pressure in Nω-nitro-L-arginine methyl ester or L-NAME induced hypertensive mice (90.97 ± 0.7975 mm Hg in methanolic extract treated mice; 87.71 ± 0.9623 mm Hg in ethyl acetate-treated mice versus 89.21 ± 0.8025 mm Hg in control group).
PUBLIC INTEREST STATEMENT
Hypertension is a condition where the blood pressure in the arteries is consistently high. People with hypertension are more likely to have stroke, heart attack and target organ damage. According to the Philippine Society of Hypertension, about 200,000 deaths per year are caused by target organ damage brought about by hypertension.
One way to combat hypertension is by using medication, an example of which is ACE inhibitors. ACE inhibitors work by blocking the action of angiotensin-converting enzyme (ACE), a chemical in our body that helps in producing a hormone called angiotensin-II. Angiotensin II increases our blood pressure by narrowing down our blood vessels. So if we block the action of ACE, less angiotensin-II will be produced, then our blood vessels can relax and our blood pressure will be reduced.
In this study, we prepared samples from ground leaves of Moringa oleifera and identified the compounds that block ACE activity. The plant extracts also lowered the blood pressure of mice.
Competing Interests
The authors declare no competing interests.
1. Introduction
Hypertension or high-blood pressure persists to be a global concern. According to the World Health Organization, the blood pressure of females aged 18 and over was around 22% and males around 24% in 2015. WHO figures show that 1.13 billion people are affected worldwide. Untreated hypertension can lead to stroke, blindness, heart attack, kidney and heart failure (Chobanian et al., Citation2003). Although the most recent drugs have gained success in the therapy of hypertension, the patients definite healing has not yet been attained and current treatments are not free from unwanted side effects (Adhiyaman, Asghar, Oke, White, & Shah, Citation2001; Bridoux et al., Citation1992; Cooper et al., Citation2006; Molinaro et al., Citation2002).
One way of lowering blood pressure is the use of angiotensin converting enzyme (ACE) inhibitors (Cuspidi, Tadic, Grassi, & Mancia, Citation2018). Previous works have been done to look for other potential ACE inhibitors from various plant sources (Adsersen & Adsersen, Citation1997; Duncan, Jäger, & van Staden, Citation1999; Loizzo et al., Citation2007; Somanadhan et al., Citation1999). The ACE-inhibitory and angioprotective effects have been attributed to the presence of flavonoids and terpenoids (Guerrero et al., Citation2002). Bis-benzylisoquinoline alkaloids from a Chinese medicinal herb, Radix stephanae, have shown anti-hypertensive effect due to its vasodilatory action (Kwan & Achike, Citation2002). Several projects were carried out to find other antihypertensive agents from various plant sources by performing studies in normotensive and hypertensive rats (Bello, Calatayud, Beltrán, Primo-Yúfera, & Esplugues, Citation2001; Carmignani et al., Citation2001; El Bardai, Lyoussi, Wibo, & Morel, Citation2001; Ibarrola et al., Citation2000; Kim, Yokozawa, Hattori, Kadota, & Namba, Citation2000). Orthosiphon aristatus (Lamiaceae) is an Indonesian traditional medicinal plant used for the treatment of hypertension and methylripariochromene A was found to be responsible for its pharmacological activity (Matsubara et al., Citation1999). Dried extracts prepared from Hibiscus sabdariffa calyxes showed significant antihypertensive effectiveness in patients with high blood pressure (Herrera-Arellano et al., Citation2007). M. oleifera is an excellent candidate for such an undertaking due to previous works done on this plant (Fahey, Citation2005). There have been several studies on M. oleifera and the scientific basis for its therapeutic use has been reviewed. Thiocarbamate glycosides were previously isolated and identified as hypotensive agents from M. oleifera (Faizi et al., Citation1995). This paper covers the isolation of other bioactive compounds and the evaluation of their potential anti-hypertensive activity.
2. Materials and methods
2.1. Purification of crude extracts
Dried M. oleifera leaves were soaked in methanol and the filtrate collected was concentrated in vacuo. Crude methanol extract (ME) was then subjected to exhaustive extraction using hexane and ethyl acetate. Ethyl acetate extracts (EA) were separately purified through open column chromatography using silica and Sephadex LH20. In the normal phase chromatography a linear gradient elution, with hexane as initial solvent up to 100% ethyl acetate, was used. In the size-exclusion chromatography (SEC) 100% methanol was used to elute the fractionated extract. Active fractions were further separated by reverse-phase high performance liquid chromatography (RP-HPLC) (Shimadzu Prominence HPLC) using C18 semi-preparative column (Agilent-Zorbax-5 um, 9.4 mm × 150 mm). Binary solvent system of acetonitrile-water (ACN-H2O) was used as the eluting solvent, a linear gradient from 5% to 95% ACN was applied for 20 min, then it was kept at 95% ACN for another 10 min. The absorbance of the eluates was monitored at 254 nm, 280 nm and 360 nm. Structure elucidation of pure and active compounds was done using Agilent 500MHz NMR spectrometer.
2.2. In-vitro angiotensin-converting enzyme (ACE) inhibition assay
A colorimetric method using a microplate reader (Thermo Fisher Scientific Varioskan Flash Multimode Reader) was optimized in a 96 well quartz microplate with a total volume of 300 uL (Jimsheena & Gowda, Citation2009). This assay was based on the amount of hippuric acid (HA) (Sigma112003) released by the action of ACE (from rabbit lung Sigma A6778) on histidine-histidyl-leucine (HHL) substrate (Sigma H1635). The released HA from the substrate is mixed with pyridine and benzenesulphonyl chloride (BSC) resulting to a yellow coloured complex with λmax at 410 nm.
2.3. Acute toxicity
Clearance from the Institutional Animal Care and Use Committee were obtained prior to the conduct of the experiment. The crude extract of M. oleifera leaves was administered in a single dose by oral gavage (OECD, Citation2001b; Schlede, Genschow, Spielmann, Stropp, & Kayser, Citation2005). Male (n = 3) and female (n = 3) mice were fasted (3–4 h) prior to dosing but they had free access to water. Following the period of fasting, mice were weighed and then extract was administered. After the extract has been administered, food was withheld for a further 1–2 h. A period of 24 h was allowed between the dosing of each animal. All animals were observed for 14 days.
2.4. Mice, study design and treatment
Female ICR strain mice were 6–8 weeks old and weighed 20–35 g. The animals were housed in cages and exposed to 12 h dark/light cycle. They were fed on normal laboratory diet with free access to water and food. Mice were randomly divided in seven groups of five animals each treated as follow: group 1 served as control and received distilled water; group 2 received solvent or vehicle; group 3 was treated with captopril (20 mg/kg/day); group 4 was treated with high-dose methanol extract (0.3 g/kg/day); group 5 was treated with low-dose methanol extract (0.01 g/kg/day); group 6 was treated with high-dose ethyl acetate extract (0.3 g/kg/day) while group 7 was treated with low-dose ethyl acetate extract (0.01 g/kg/day). All the treatments were orally gavage at the corresponding volume of 1 ml/100 g body weight. All the animals received distilled water during the first week before random selection for drug treatment in order to get acquainted to the gavage procedure. Hypertension was induced by Nω-nitro-L-arginine methyl ester (L-NAME) treatment (30 mg/kg/day).
2.5. Non-invasive tail-cuff blood pressure (BP) measurements
Computerized tail-cuff system (Visitech Systems BP-2000 Blood Pressure Analysis System™) was employed to record mice BP measurements (Krege, Hodgin, Hagaman, & Smithies, Citation1995). The following programmable settings were done in order for the device to give reproducible BP measurements: (1) 10 preliminary unrecorded measurements were done to warm up and give a waveform after placing each mice into the device; (2) each session included two sets of 10 measurements, 10 unrecorded sessions and 10 actual recorded session; (3) mice were kept at 37°C for 5 min prior to recording. The animals had undergone seven days of training sessions with the device to become accustomed to the tail-cuff procedure and minimize noise readings. The BP measurement always preceded the animal treatment.
2.6. Data analysis
Data were represented as means ± SEM. Statistical data significance at the level of p < 0.05 was determined using one-way Analysis of Variance (ANOVA) followed by the Tukey’s method using GraphPad-Prism software.
3. Results and discussion
3.1. Bioassay-guided isolation of antihypertensive compounds
Preliminary results have shown that EA extract exhibited the highest ACE inhibitory activity at 64.23 ± 0.0562% while positive control captopril at 87.57 ± 0.0153%. This led to further purification of EA in order to identify the compound/s responsible for its bioactivity. EA was purified using normal phase vacuum liquid chromatography (NP-VLC) and size exclusion chromatography (SEC). The resulting NP-VLC fractions were tested for their bioactivity. As shown in Figure , ME-F6 and ME-F13 fractions exhibited relatively high percent inhibition (58.23 ± 0.0202% and 53.00 ± 0.0205%, respectively). On the other hand, ME-F11 displayed the significantly highest %inhibition (95.85 ± 0.0181%) at 12ug/ml concentration, even higher than Captopril that represents the positive control (57.67 ± 0.0051%).
Figure 1. ACE inhibitory activity of EA extract purified by NP-VLC; Captopril was used as the positive control. The results were expressed as % of ACE inhibition with respect to the control. Each value was the mean of three independent determinations.
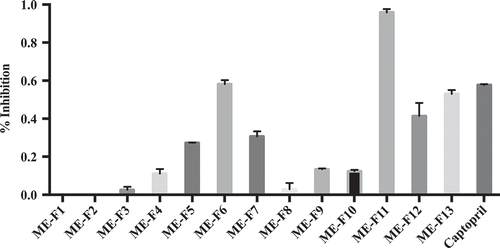
Two SEC pooled fractions were revealed in ME-F11, namely: ME-F1101 (10 mg) and ME-F1102 (3 mg) and this result was confirmed by HPLC profile. In fact, also in this case, two significant peaks were with retention time at 20:5” and 21:5, respectively. To further check the purity of the fractioned extracts from ME-F11, HPLC profiles of each were obtained and both produced a single distinct peak. The results show that the purified fractions (ME-F1101 and ME-F1102) can be considered pure and therefore it was interesting to proceed with their structure elucidation by NMR and with bioactivity evaluation.
Fraction ME-F1101 was then subjected to ACE inhibitory assay (Figure ) to determine its bioactivity at three varying concentrations: 7, 15 and 28 ug/mL. The purified fraction ME-F1101 remained active at the lowest concentration of 7 ug/mL (56.37 ± 0.0059%) and exhibited the highest % inhibition at 28 ug/mL (75.74 ± 0.0161%). The decrease in ACE inhibition activity that we have observed between the extracts can be attributed to synergistic effect loss. The bioactivity of ME-F1102 was not determined due to its low yield.
3.2. Structure elucidation of bioactive compounds
Bioactivity-guided fractionation of M. oleifera leaves ethyl acetate extract afforded an active fraction, ME-F11. This was further purified using a Sephadex column and gave two pure compounds: ME-F1101 [1] and ME-F1102 [2]. Compounds 1 and 2 were obtained as yellow and dark-yellow powders respectively. Based on spectral data, these compounds were elucidated as two known flavonoid glycosides.
NMR spectral data measured in CD3OD are tabulated in Tables and . Compound 1 was determined as quercetin-3-O-glucoside, C21H20O12. NMR signals of 1 were found to be the same with that of the literature (Kazuma, Noda, & Suzuki, Citation2003). The molecular formula of 2 was established as C21H20O11 ([M-H]+ = 449.1089) by ESI-MS. It is suspected to be kaempferol-3-O-glucoside since its 1HNMR signals were similar with that of the literature (Kazuma et al., Citation2003). The structures are shown in Figure .
Table 1. Summary of 13C and 1H NMR data of 1
Table 2. 1HNMR data of isolated compounds
3.3. Standardization of ME-F1101 against crude extract
The compound marker (ME-F1101) amount in the crude extract preparation was determined using RP-HPLC. Twenty milligrams of the methanol extract in 10 mL of solvent and 1 mg of the isolated compound used as a reference standard in 1 mL of solvent were analysed separately using gradient (5%-95% ACN) and isocratic (95% ACN) elution. The chromatogram of M. oleifera methanol extract showed a significant peak at 19.52 min (ME-F1101) that was determined by comparing it against the chromatogram of the reference standard, ME-F1101. Calibration standards were prepared at concentrations of 500, 250, 125 and 62.5 μg/mL. A graph of area versus concentration was plotted linear with a correlation coefficient (R2) of 0.9973. Results showed that the relative amount of ME-F1101 to 2 mg/ml of methanol extract was 180.15 ug/ml.
3.4. Determination of LD50
Crude extract of M. oleifera showed no toxicity up to the dosage level of 2000 mg/kg. At 2000 mg/kg, mice were observed to be dull and weak at the onset of administration but recovered fully within 24 h. Results of mice tests show that doses of 5000 and 8000 mg/kg cause 33% and 67% of deaths, respectively. Therefore, estimated LD50 of crude methanol M. oleifera extract is 8000 mg/kg <LD50 < 5000 mg/kg. Substances that have LD50 higher than 5000 mg/kg by oral route can be considered practically non-toxic (Kennedy, Ferenz, & Burgess, Citation1986), therefore, ME administered orally does not possess acute toxicity. It is a great candidate for testing in L-NAME induced hypertension model in mice.
3.5. Blood pressure lowering effect of M. oleifera extracts
The oral administration of L-NAME (30 mg/kg/day) to mice increased the systolic BP from 90.35 ± 2.3411 mm Hg to 108.64 ± 3.1723 mm Hg with BP levelling from the 10th to 25th day of treatment (Figure ). It has induced a rapid increase in BP that is significantly different from that of the control group and continued until the end of the treatment. Administration ofL-NAMEorally produced an increase in blood pressure due to the chronic inhibition of NO synthase, one of the major endogenous relaxing factors, and persistent activation of rennin–angiotensin system (Monassier, Combe, & El Fertak, Citation2006; Nguelefack et al., Citation2009).
Figure 4. Systolic blood pressure (mm Hg) of mice treated with water (control, 1 ml/0.1kg/day, n = 5) or treated with L-NAME (30 mg/kg/day, n = 5). After the hypertension was established, a group of mice was treated with captopril (30 mg/kg/day, n = 5) as positive control group. Data are represented as means ± SEM. *Significantly different from the control group.
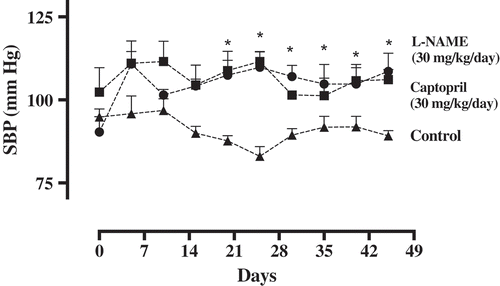
Three weeks after oral administration of L-NAME to mice, five of these animals were randomly selected and treated with ME or EA extracts (0.3 g/kg/day) during 25 days. In the animals receiving ME, BP gradually decreased from 102.35 ± 1.5509 mm Hg to 90.97 ± 0.7975 mm Hg (Figure )). Furthermore, BP values (95.65 ± 0.2849 mm Hg versus 108.83 ± 3.5537 mm Hg in L-NAME-treated mice) were significantly lower with respect to the L-NAME-treated mice already at the second week of treatment. Addition of ME (0.3 g/kg/day) to the mice treatment group resulted to a significant and persistent reduction in BP that is comparable with the control group at the end of the treatment period (90.97 ± 0.7975 mm Hg versus 89.21 ± 0.8025 mm Hg in control group).
Figure 5. Systolic blood pressure (mm Hg) of mice treated with water (control, 1 ml/0.1 kg/day, n = 5) or treated with L-NAME (30 mg/kg/day, n = 5). After the hypertension was established, a group of mice was treated with ME (a) or EA (b) of M. oleifera (300 mg/kg/day, n = 5). Data are represented as means ± SEM. *Significantly different from L-NAME-treated mice.
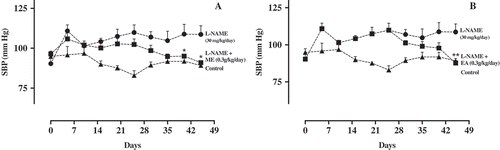
Since ethyl acetate extract has showed significant inhibition of ACE activity in-vitro, its ability to lower BP in L-NAME model of hypertension was also investigated. The systolic blood pressure in EA-treated mice tends to decrease but it only showed significant inhibitory effect only at the end of treatment (87.71 ± 0.9623 mm Hg versus 89.21 ± 0.8025 mm Hg in control group). Although it only decreased BP at the last day of the treatment period, EA (0.3 g/kg/day) has greatly diminished the hypertensive effects of L-NAME with BP values lower than that of the control group (Figure )). The results obtained with ME and EA treatments demonstrated the BP lowering effect of M. oleifera crude extracts in L-NAME induced hypertensive mice.
Figure 6. Systolic blood pressure (mm Hg) of mice treated with water (control, 1 ml/0.1 kg/day, n = 5) or treated with L-NAME (30 mg/kg/day, n = 5). Dose dependent studies of (a) ME-treated mice and (b) EA-treated mice. Data are represented as means ± SEM.
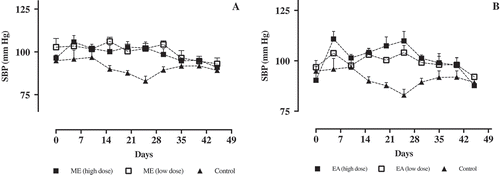
Dose dependent response test has also been done by comparing the effects of high (0.3 g/kg/day) and low (0.01 g/kg/day) doses of crude extracts in systolic BP of mice (Figure ). However, results revealed that there was no significant difference in BP measurements in both dosage treatments of crude extracts. It can be concluded that crude extracts of M. oleifera remained potent in lowering BP even at 0.01 g/kg/day dose. Minimum inhibitory concentration should be, therefore, established to further determine the efficacy of standardized crude extracts of M. oleifera.
4. Summary and conclusion
At present, there are no published reports regarding standardized herbal products based on M. oleifera. The results established the anti-hypertensive activity of M. oleifera using the ACE inhibitory assay and revealed the concentration of the activity in the ethyl acetate portions. Two flavonoid glycosides were reported to have significant %inhibition both comparable with the positive control captopril. The results obtained in vivo indicated that mice treated with crude methanol and ethyl acetate extracts of M. oleifera significantly decrease the systolic blood pressure of L-NAME-induced hypertensive mice. In either case, the effect was established after 4 weeks of treatment and there was no significant difference in BP values between high (0.3 g/kg/day) and low (0.01 g/kg/day) doses. In conclusion, the study shows that M. oleifera extracts are effective in the inhibition of two major pathways in hypertension study that are correlated to ACE inhibition and to NO synthesis. The results of this study support that M. oleifera extracts can be developed as a potential anti-hypertensive health product.
Acknowledgements
We wish to thank the Department of Science and Technology through the Philippine Council for Health Research and Development for funding this project.
This work was supported by the Department of Science and Technology through the Philippine Council for Health Research and Development.
Disclosure Statement
The authors report no declarations of interest.
Additional information
Funding
Notes on contributors
Christine L. Chichioco Hernandez
Christine L. Chichioco Hernandez is an associate professor of the Institute of Chemistry, University of the Philippines Diliman. She is also the principal investigator of the Bioorganic and Natural Products Laboratory of the same institution. Her group looks for biologically active compounds from plant extracts (anti-mitotic, anti-inflammatory, anti-mutagenic, anti-ulcer, anti-pyrexia, hypolipidemic and anti-malarial); identification of enzymatic inhibitors from plant extracts with potential therapeutic applications (acetylcholinesterase, angiotensin-converting enzyme, lipase, lipoxygenase, xanthine oxidase and HMG-CoA reductase) and identification of drug targets.
References
- Adhiyaman, V., Asghar, M., Oke, A., White, A. D., & Shah, I. U. (2001). Nephrotoxicity in the Elderly Due to Co-Prescription of Angiotensin Converting Enzyme Inhibitors and Nonsteroidal Anti-Inflammatory Drugs. Journal of the Royal Society of Medicine, 94, 512–514. doi:10.1177/014107680109401005
- Adsersen, A., & Adsersen, H. (1997). Plants from Réunion Island with alleged antihypertensive and diuretic effects—An experimental and ethnobotanical evaluation. Journal of Ethnopharmacology, 58, 189–206. doi:10.1016/S0378-8741(97)00100-1
- Bello, R., Calatayud, S., Beltrán, B., Primo-Yúfera, E., & Esplugues, J. (2001). Cardiovascular effects of the methanol and dichloromethanol extracts from Mentha suaveolens Ehrh. Phytotherapy Research, 15, 447–448.
- Bridoux, F., Hazzan, M., Pallot, J. L., Fleury, D., Lemaitre, V., Kleinknecht, D., & Vanhille, P. (1992). Acute renal failure after the use of angiotensin-converting-enzyme inhibitors in patients without renal artery stenosis. Nephrology Dialysis Transplantation, 7, 100.
- Carmignani, M., Volpe, A. R., Botta, B., Espinal, R., De Bonnevaux, S. C., De Luca, C., … Delle Monache, G. (2001). Novel hypotensive agents from Verbesina c aracasana. 8. Synthesis and pharmacology of (3, 4-Dimethoxycinnamoyl)-N-agmatine and Synthetic Analogues1. Journal of Medicinal Chemistry, 44, 2950–2958.
- Chobanian, A. V., Bakris, G. L., Black, H. R., Cushman, W. C., Green, L. A., Isso, J. R., Jr, … Roccella, E. J. (2003). Seventh Report of the Joint National Committee on Prevention, Detection, Evaluation, and Treatment of High Blood Pressure. Hypertension, 42, 1206. doi:10.1161/01.HYP.0000107251.49515.c2
- Cooper, W. O., Hernandez-Diaz, S., Arbogast, P. G., Dudle, J. A., Dyer, S., Gideon, P. S., … Ray, W. A. (2006). Major Congenital Malformations after First-Trimester Exposure to ACE Inhibitors. New England Journal of Medicine, 354, 2443–2451. doi:10.1056/NEJMoa055202
- Cuspidi, C., Tadic, M., Grassi, G., & Mancia, G. (2018). Treatment of hypertension: The ESH/ESC guidelines recommendations. Pharmacological Research, 128, 315–321. doi:10.1016/j.phrs.2017.10.003
- Duncan, A. C., Jäger, A. K., & van Staden, J. (1999). Screening of Zulu medicinal plants for angiotensin converting enzyme (ACE) inhibitors. Journal of Ethnopharmacology, 68, 63–70. doi:10.1016/S0378-8741(99)00097-5
- El Bardai, S., Lyoussi, B., Wibo, M., & Morel, N. (2001). Pharmacological evidence of hypotensive activity of Marrubium vulgare and Foeniculum vulgare in spontaneously hypertensive rat. Clinical and Experimental Hypertension, 23, 329–343.
- Fahey, J. (2005). Moringa oleifera: A review of the medical evidence for its nutritional, therapeutic, and prophylactic properties. Part 1. Trees for Life Journal, 1, 5.
- Faizi, S., Siddiqui, B. S., Saleem, R., Siddiqui, S., Aftab, K., & Gilani, A. H. (1995). Fully acetylated carbamate and hypotensive thiocarbamate glycosides from Moringa oleifera. Phytochemistry, 38, 957–963. doi:10.1016/0031-9422(94)00729-D
- Guerrero, M. F., Puebla, P., Carrón, R., Martín, M. L., Arteaga, L., & Román, L. S. (2002). Assessment of the antihypertensive and vasodilator effects of ethanolic extracts of some Colombian medicinal plants. Journal of Ethnopharmacology, 80, 37–42. doi:10.1016/S0378-8741(01)00420-2
- Herrera-Arellano, A., Miranda-Sanchez, J., Avila-Castro, P., Herrera-Alvarez, S., Jimenez-Ferrer, J. E., Zamilpa, A., … Tortoriello, J. (2007). Clinical effects produced by a standardized herbal medicinal product of Hibiscus sabdariffa on patients with hypertension. A randomized, double-blind, lisinopril-controlled clinical trial. Planta medica, 73, 6. doi:10.1055/s-2006-957065
- Ibarrola, D. A., Hellión-Lbarrola, M. C., Montalbetti, Y., Heinichen, O., Alvarenga, N., Figueredo, A., & Ferro, E. A. (2000). Isolation of hypotensive compounds from Solanum sisymbriifolium Lam. Journal of Ethnopharmacology, 70, 301–307. doi:10.1016/S0378-8741(00)00191-4
- Jimsheena, V. K., & Gowda, L. R. (2009). Colorimetric, High-Throughput Assay for Screening Angiotensin I-Converting Enzyme Inhibitors. Analytical Chemistry, 81, 9388–9394. doi:10.1021/ac901775h
- Kazuma, K., Noda, N., & Suzuki, M. (2003). Malonylated flavonol glycosides from the petals of Clitoria ternatea. Phytochemistry, 62, 229–237. doi:10.1016/S0031-9422(02)00486-7
- Kennedy, G. L., Ferenz, R. L., & Burgess, B. A. (1986). Estimation of acute oral toxicity in rates by determination of the approximate lethal dose rather than the LD50. Journal of Applied Toxicology, 6, 145–148. doi:10.1002/(ISSN)1099-1263
- Kim, D., Yokozawa, T., Hattori, M., Kadota, S., & Namba, T. (2000). Effects of aqueous extracts of Apocynum venetum leaves on spontaneously hypertensive, renal hypertensive and NaCl-fed-hypertensive rats. Journal of Ethnopharmacology, 72, 53–59. doi:10.1016/S0378-8741(00)00197-5
- Krege, J. H., Hodgin, J. B., Hagaman, J. R., & Smithies, O. (1995). A Noninvasive Computerized Tail-Cuff System for Measuring Blood Pressure in Mice. Hypertension, 25, 1111–1115. doi:10.1161/01.HYP.25.5.1111
- Kwan, C., & Achike, F. (2002). Tetrandrine and related bis-benzylisoquinoline alkaloids from medicinal herbs: Cardiovascular effects and mechanisms of action. Acta Pharmacologica Sinica, 23, 1057.
- Loizzo, M. R., Said, A., Tundis, R., Rashed, K., Statti, G. A., Hufner, A., & Menichini, F. (2007). Inhibition of angiotensin converting enzyme (ACE) by flavonoids isolated fromAilanthus excelsa (Roxb) (Simaroubaceae). Phytotherapy Research, 21, 32–36. doi:10.1002/(ISSN)1099-1573
- Matsubara, T., Bohgaki, T., Watarai, M., Suzuki, H., Ohashi, K., & Shibuya, H. (1999). Antihypertensive Actions of Methylripariochromene A from Orthosiphon aristatus, an Indonesian Traditional Medicinal Plant. Biological and Pharmaceutical Bulletin, 22, 1083–1088. doi:10.1248/bpb.22.1083
- Molinaro, G., Cugno, M., Perez, M., Lepage, Y., Gervais, N., Agostoni, A., & Adam, A. (2002). Journal of Pharmacology and Experimental Therapeutics, 303, 232. doi:10.1124/jpet.102.038067
- Monassier, L., Combe, R., & El Fertak, L. (2006). Mouse models of hypertension. Drug Discovery Today: Disease Models, 3, 273–281. doi:10.1016/j.ddmod.2006.10.008
- Nguelefack, T. B., Mekhfi, H., Dongmo, A. B., Dimo, T., Watcho, P., Zoheir, J., … Ziyyat, A. (2009). Hypertensive effects of oral administration of the aqueous extract of Solanum torvum fruits in L-NAME treated rats: Evidence from in vivo and in vitro studies. Journal of Ethnopharmacology, 124, 592–10. doi:10.1016/j.jep.2009.05.030
- OECD. (2001b). Guideline for the testing of chemicals: Acute oral toxicity—Fixed Dose Procedure (TG 420), adopted: 17.07.92; revised method adopted: 17th December 2001. Paris: Author.
- Schlede, E., Genschow, E., Spielmann, H., Stropp, G., & Kayser, D. (2005). Oral acute toxic class method: A successful alternative to the oral LD50 test. Regulatory Toxicology and Pharmacology, 42, 15–23. doi:10.1016/j.yrtph.2004.12.006
- Somanadhan, B., Varughese, G., Palpu, P., Sreedharan, R., Gudiksen, L., Smitt, U. W., & Nyman, U. (1999). An ethnopharmacological survey for potential angiotensin converting enzyme inhibitors from Indian medicinal plants. Journal of Ethnopharmacology, 65, 103–112. doi:10.1016/S0378-8741(98)00201-3