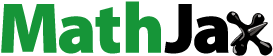
ABSTRACT
The open source 30 m Copernicus DEM (COP-DEM) has been used to derive the geo-morphometric and topo-hydrological characteristics of Betwa River Basin. Drainage network with 7th order stream and mostly dendritic pattern has been delineated using the Strahler’s method. The drainage density, stream frequency, form factor, length of overland flow and circulatory ratio of the basin were computed as 0.38 Km/Km2, 0.08 Km−2, 0.23, 1.31, and 0.20, respectively. These outcomes suggest less available surface flow, lesser relative relief, poor drainage network, and nearly elongated shape of the basin. The mean bifurcation ratio for entire basin was calculated as 4.0 that signifies gradient dependent drainage network of the basin. The ruggedness number was computed as 0.24, which reflects the geology dominated (granite–gneiss) rocky and undulated topography of the basin. The dissection index ranges from 0.06 to 0.3, which implies less degree of dissection and mature stage of the basin. The topographic wetness index (TWI), stream power index (SPI), terrain ruggedness index (TRI), and sediment transport index (STI) ranges from 3.13 to 26.35; 0 to 6; 0 to 30; and 0 to 121.4, respectively. It implies that the lower basin is less susceptible towards erosion as compared to upper basin.
1. Introduction
The river basins of semi-arid and arid regions, where surface water is scarce and in some places completely unavailable, are facing more severe challenges as compare to other regions of the world (Dubey et al., Citation2020; Sreedevi et al., Citation2013). Over exploitation of water resources in semi-arid and arid basins aggravate the water availability in the basins and alter the river flows and sediment load which in turn affects the morphology of that basin (Desai et al., Citation2021; Singh et al., Citation2021; Sreedevi et al., Citation2013). The relationships of different hydro-meteorological phenomena with the physiographic features of the drainage basins have been extensively studied by researchers (Bryndal et al., Citation2020; Kanhaiya et al., Citation2018, Citation2019; Magesh et al., Citation2013; Obeidat et al., Citation2021; Singh, Kanhaiya, et al., Citation2019; Rai et al., Citation2017; Singh, Kanhaiya et al., Citation2019). Quantification of geo-morphometric parameters such as basin area, perimeter, length, stream order, bifurcation ratio, stream length, stream frequency, drainage density, elongation ratio, circularity ratio, form factor ratio, texture ratio etc. of any basin gives detail about the geological, physiological, and hydrological dynamics of a basin (Shreve, Citation1966; Sreedevi et al., Citation2013; Singh & Kanhaiya, Citation2015; Prakash et al., Citation2017; Kumar et al.,; Singh, Kanhaiya et al., Citation2019; Kanhaiya et al., Citation2019; Kumar & Singh, Citation2021a; Kumar et al., Citation2021b). The hydro-morphological aspects of basins have also been investigated widely by assessing the topo-hydrological parameters viz. Topographic Wetness Index (TWI); Stream Power Index (SPI); Terrain Roughness Index (TRI) and Sediment Transport Index (STI) etc. (Alilou et al., Citation2019). Both, the geo-morphometric and topo-hydrological analysis is essential to unearth the geological, physiological and hydrological characteristics of river basins and help in river basin management (Alilou et al., Citation2019). It also helps in identification of structural and tectonic events associated with the evolutionary processes of a basin (Resmi et al., Citation2019). Nowadays, digital elevation models (DEM; Burrough et al., Citation2015; Lakshmi & Yarrakula, Citation2018; Polidori & El Hage, Citation2020) in conjunction with geospatial techniques (Smith & Clark, Citation2005) are being widely used by the researchers for various scientific analysis including studies pertinent to the topography, geomorphology, hydrology, tectonic activities, morphometric analysis, and so on (Dubey et al., Citation2015; Banerjee et al., Citation2017; Singh, Kanhaiya et al., Citation2019; Kumar et al., Citation2021b; Jothimani et al., Citation2021). Details of open source and commercial DEMs are outlined in .
Table 1. Details of open source and commercial DEMs.
Majority of the recent DEM based studies have been performed using Shuttle Radar Topography Mission (SRTM) DEMs (Guth, Citation2011; Singh et al., Citation2020; Patel et al., Citation2022), DEM generated from topographic contours (TOPO) DEMs (Chopra et al., Citation2005; Prasannakumar et al., Citation2013), Cartosat DEMs (Pandule, Citation2019; Sharma & Mahajan, Citation2020; Yadav et al., Citation2014 Yadav et al., Citation2018 Yadav et al., Citation2020) and Advanced Spaceborne Thermal Emission and Reflection Radiometer (ASTER) DEMs (Farhan, Citation2017; Sujatha et al., Citation2015), Advanced Land Observation System (ALOS) DEMs (Caglar et al., Citation2018; Tesema, Citation2021). Recently, an improved DEM data product namely Copernicus DEM GLO-30 has been released by the European Space Agency (ESA) for the academic, scienCitation2017tific research and civil applications. As per ESA, improvements such as flattening of water bodies with assured hydrological consistency and editing of other man made special features and implausible structures gives Copernicus DEM GLO-30 an edge over other DEMs.
In accordance with the recent progresses and researches as detailed above the present study aims to extract geo-morphometric and topo-hydrological characteristics of BRB using Copernicus DEM GLO-30 and geospatial techniques as an maiden attempt. It is a maiden attempt to explore the geo-morphometric characteristics together with topo-hydrological aspects of BRB using fine resolution (30 meter) Copernicus DEM GLO-30. Being located in the semi arid to humid subtropical region of Central India, BRB has also experienced considerable change over the years (Singh et al., Citation2022 Kumar et al., Citation2017; Singh & Sharma, Citation2022). It covers major portion of India’s water scarce Bundelkhand region within its diverse geological, hydrological, and physiographic setups. Recently, Government of India has announced interlinking of Ken River with Betwa River to bring the “surplus” waters of Ken river basin into water “deficit” Betwa river basin, under the national river interlinking project namely Ken-Betwa Link Project (KBLP; Avtar et al., Citation2010). Hence, this study will be a reference work in future for analyzing and monitoring the landform changes in the basin after completion of the proposed river interlinking project (KBLP). Further the study will signify the suitability and potentials of Copernicus DEM GLO-30 as an alternative DEM for studying topo-hydrological and geo-morphometric characteristics of drainage basins. This study will also prove to be vital for the better management, planning, decision making, and conservation of the basin as well as for future strategies to be implemented in the BRB.
2. Study area
The Betwa River Basin (BRB), a sub-basin of the Yamuna River Basin, is situated between longitudes 23°5′ to 25°55′E and latitudes 77°15′ to 79°45′N in Central India (). The strike ridges of Vindhyan hills and Malwa plateau along the south and southwest margin of Betwa River Basin separates it from Narmada valley. The ravines of Chambal-Sindh and basin area of Ken River reside to the west and east margins of the BRB respectively. Betwa River, a major cratonic tributary of Yamuna River, is main stream of the BRB. Betwa River rises at an elevation of about 576 m above mean sea level (a.m.s.l.) from Jhiri perennial spring at the foot of Dhondi Dant hills (Vindhyan Range) near Barkhera village in Raisen district of Madhya Pradesh, India. It flows in SW to NE direction for 590 Km to join river Yamuna at the village Merapur Daria (about 106 m a.m.s.l.) in Hamirpur district of Uttar Pradesh, India. The major sub tributaries that join the Betwa River in the BRB are Kaliasot, Halali, Sagar, and Bina in upstream, Narain, Orr, and Jamni in midstream, and Dhasan, and Birma in downstream. It drains a total catchment area of about 43,346.11 km2 between the two states viz. Madhya Pradesh (68.84% of the total area) and Uttar Pradesh (32.16% of the total area). The Basin has an average population density of 295.9 people per kilometer with a total population of 20.20 million people (Ahlawat, Citation2010; Chandramouli, Citation2011). Majority of the population living in the basin is agrarian, rural poor, economically deprived, and prone to nature induced distresses like frequent drought (Pandey et al., Citation2008; Singh & Sharma, Citation2022) and disastrous floods (Avtar et al., Citation2010).The basin receives average annual rainfall of about 918 mm, mostly (80%) from south-west monsoon system (Chaube et al., Citation2011). More rainfall occurs in the upper and middle portion of the basin as compare to the lower portion of the basin (Singhai et al., Citation2019). Basin is also characterized by average minimum and maximum temperatures of 06.7°C (mild winter) and 44.2°C (hot summer) respectively (Suryavanshi et al., Citation2014). The National Bureau of Soil Survey and Land Use Planning (NBSSLUP), Nagpur, India, has identified major soil types in the basin as clay, silty clay, clay loam and sandy loam soil (Suryavanshi et al., Citation2017). The basin is pervaded by extremely jagged, rocky and undulating surface topography, having dispersed elongated hills, steep forest-covered hills, scattered bushes, flat plateaus, and parallel linear ridges with land slopes varying from 0 degree to 57 degree.
3. Geology
Geologically, the basin is underlain with the litho-assemblages of various age groups from Archaean to Quaternary period. The geological map of the basin () is based on the geological data of BRB obtained from the Bhukosh (https://bhukosh.gsi.gov.in/Bhukosh/Public) gateway of Geological Survey of India (GSI). The Deccan trap (66 million years), the Vindhyan Supergroup (1.4–0.9 billion years), the Bijawar group (2.6–2.4 billion years) and Bundelkhand complex (older than 2.6 billion years) are the major group of rocks with high uncertain ages that collectively underlain the Betwa River basin (Venkatesh & Anshumali, Citation2019). The basaltic rocks of Deccan trap extends over 42% area basically in upper portion of the basin; Bundelkhand massif covers 41% area basically in upper and middle parts of the basin; Alluvial plain envelopes 9% area in lower regions of the basin; Vindhyan Supergroup occupies about 6% basin area and Bijawar Group is extended over 2% area of the BRB. Vindhyan Supergroup in BRB includes rocks of Bhander group, Kaimur group, Rewa group, and Semri group.
Betwa river and its tributaries in the basin drain basically through the terrains comprises of coarse, medium and fine grained granite, porphyritic granite, basalt, sandstone, shale, silt, and clay with kankar, red quartizofelpathic, sand and grey micaceous sand, alluvium, laterite, limestone, dolomite, dolerite, amphibolites, migmatite, calc-silicate rocks, granitoids, granite gneisses, syenites, tonalite–trondhjemite–granodiorite (TTG), basaltic metasediments, quartz veins, and other terrigenous sediments and rocks (Malviya et al., Citation2006; Pati et al., Citation2007). Major portion of the basin is underlined by granite followed by basalt, sandstone, and alluvium respectively. These terrains have relatively low primary porosity and permeability except alluvium; hence does not permit sufficient groundwater recharge that produce ground water scarcity in different parts of the basin. There are small patches of alluvium or highly fractured/ jointed rocks which stores and provides water for drinking and irrigation purposes.
4. Materials and methods
In the present study, an integrated approach of using Topographical maps and Digital Elevation Model (DEM) for quantifying different drainage dimensions have been employed. Copernicus DEM with spatial resolution 30 m () and Survey of India’s (SOI) toposheets with scale of 1:50,000 have been used in a geo-processing framework to build database, extraction of drainage parameters and generation of thematic maps. , Shows details of the data sets used in present study. The steps and procedures opted for this study has been shown in .
Table 2. Formulae and methods applied for computing geo-morphometric parameters of Betwa River Basin.
Table 3. Major specifications of Copernicus DEM.
Table 4. Details of SOI toposheets and DEM used in the study.
Geocoding of SOI toposheets have been done by geometrically rectifying and geo-referencing each of them using ground control points (GCPs) and projection system Universal Transverse Mercator (UTM), Zone 43, datum World Geodetic System (WGS) 1984 in Arc GIS software. The Drainage network of the basin has been derived by defining threshold values during each the steps viz. pits or sinks fill, flow direction, and accumulation, stream order extraction, and drainage network delineation. Appropriate threshold values determine the level of pairing between actual ground based drainage network and drainage network extracted from DEM (Arulbalaji & Padmalal, Citation2020). A larger threshold value will provide sparse drainage network and vice versa (Anish et al., Citation2021). The sinks have been filled to eliminate drainage network discontinuity by the higher values of surrounding elevations. Eight-direction flow model has been used on the filled DEM for deriving flow direction from each pixel (Fairfield & Leymarie, Citation1991). Further, flow direction was used for generating drainage system by taking the pixels having a threshold value of more than 100 into consideration using a trial-and-error approach (Mark, Citation1983). The boundary polygon of Betwa River basin was extracted from Copernicus DEM and SOI toposheets using pour point (Magesh et al., Citation2013). The cross verification of Copernicus DEM based drainage system was performed using a mosaiced map derived from the geocoded toposheets of SOI. The stream network present in toposheet has been validated with the extracted stream network from Copernicus DEM by visually as well as using Coefficient of Line Correspondence (CLC) analysis method (Stanislawski et al., Citation2015). CLC automatically differentiates matching and non matching linear features from two separate drainage networks. The CLC value ranges from 0 to 1 where zero denotes no match between features and 1 represents perfect match between features. The expression of CLC is as follows (EquationEquation. 1)(1)
(1) :
Where, Lm denotes the sum of the length of the matching lines and La represents the sum of the length of all lines in both datasets.
Only matching stream networks of both datasets were taken for the study. The unmatched extracted stream networks were reprocessed again and again by altering the threshold values until the acceptable result obtained (Harsha et al., Citation2020). The assessment of geo-morphometric and topo-hydrological parameters of the BRB have been quantified using well established formulae and methods mentioned in 1.
5. Results and discussion
In this study, COP-DEM with 30 m pixel size has been used for deriving the geo-morphometric and topo-hydrological attributes of the BRB. The drainage basin parameters such as basin size, slope, shape, drainage density, and stream length etc. of the basin have been computed using ArcGIS software. The CLC method based drainage delineation accuracy was calculated as 0.92, which denotes high match between extracted drainage networks with delineated drainage networks. Total 3522 numbers of streams of all orders mostly with NE and SW orientation has been calculated in the basin. An inverse relation has been observed between stream number (Nμ) and increasing order of the streams (μ). The 1st order streams shares about 80% of the total streams and number of successive higher-order streams from the 2nd order to 7th order decreases with inconsistency and abruptly. Higher number of streams in 1st order especially in the upper reaches of the basin reflects semi-permeable lithology and low infiltration rate of the terrain. The river basins are natural, unique, hydrologically self-contained, and fundamental entities of the water resource planning and management (Moore et al., Citation1991). They are very sensitive and dynamic component of the fluvial landscape that naturally keeps changing their state (Brunsden, Citation2001; Fryirs, Citation2017). Hence, an assessment of both geo-morphometric and topo-hydrological parameters of a drainage basin gives essential information about three-dimensional geometry of the basin, which helps in planning and management of that basin (Bryndal et al., Citation2020; Rajasekhar et al., Citation2020). The COP-DEM based basic morphometric parameters of the basin viz. elevation, relative relief ,slope and aspect has been shown in . The results of the linear, areal and relief aspects of present study are as follows.
5.1. Linear morphometric parameters
A linear aspect reflects details about one-dimensional morphometric parameter such as stream order, stream number, stream length, bifurcation ratio, and sinuosity index etc. It indicates topological characteristics of the stream segments and channel patterns of the drainage system. Linear aspects of a drainage basin exhibit spatio-temporal variations, hence influences hydrological behavior of a basin (Soni, Citation2017). The result of linear morphometric parameters are given in the .
Table 5. Results of linear geo-morphometric parameters.
5.1.1. Stream order (μ)
The first step towards drainage basin analysis is stream ordering. It is a measure of stream branching extent in a basin. Stream ordering is performed by designating a rank to a stream in the hierarchy of tributaries (Leopold et al., Citation2020). It provides an idea about the size of drainage basin and approximate index of stream flow. The stream order is generally controlled by the tectonic and physiographic set-up of the basins (Yadav et al., Citation2018; Kanhaiya et al., Citation2019). The smallest, fingertip stream that has no tributaries are designated as first-order streams; the confluence of two first-order streams forms a second-order stream and so on. Therefore, with the increase of stream order, decrease in number of stream is obvious. The order and size of the streams in a basin generally depends on its physiographic and structural terrain properties (Haghipour & Burg, Citation2014). Our analysis shows that BRB is a seventh-order drainage basin with 78.53% and 16.98% streams of first and second-order respectively (). Venkatesh and Anshumali (Citation2019) have used SRTM-DEM with 90 m pixels for morphometric analysis of BRB and reported it as sixth-order drainage basin. The stream order difference can be attributed to the fine resolution of COP-DEM used for the study. The drainage pattern of the BRB is mostly sub-dendritic to rectilinear in nature, which represents the plateau–plain sub-humid environment and reflects terrain gradient based river channels of the basin (Wakode et al., Citation2013). The numbers of higher order streams (4th, 5th, 6th order) in the basin are less in number due to dominance of alluvial plain course in lower reaches.
5.1.2. Stream number (Nμ)
The order wise cumulative measurement of stream segment gives stream number (Yadav et al., Citation2014). It signifies hydrological character of a basin especially factors like surface runoff. Stream number provides substantial information about the surface runoff features i.e., a higher number of first-order streams indicate higher permeability and erodible topography. According to Horton (Citation1945), stream number shows reciprocal geometric sequence with the order unit, i.e., with increase in stream order the stream number decreases. The streams in the BRB are supporting the Horton’s principle and total number of streams computed in the study area is 3522. Higher number of headwater streams indicates highly erodible topography with erosional landform in upper reaches and affects surface runoff characteristics in lower reaches of basin (Singh & Awasthi, Citation2011). depicting inverse relation between stream order and stream number of BRB.
5.1.3. Stream length (Lμ)
Stream length is the total length of a stream measured from its genesis upto its outlet and depicts development stages of successive stream segments (Allan et al., Citation2020). It expresses the hydrological characteristics such as surface runoff and sediment properties as well as contributing area of a basin (Magesh et al., Citation2011). Generally, 1st order streams have higher combined stream length and better distribution than that of next higher orders (Rai et al., Citation2018). Such type of stream arrangement represents the chronological evolution of the rivers. The shorter stream lengths indicate areas with larger slopes and finer textures whereas longer stream lengths are normally indicative of flatter gradient. The number of streams in different order in the basin was counted along with their lengths measurement from origin to drainage divide. The calculated stream length for each stream order is listed in . The result shows that the 5th and 7th order streams have relatively longer length of 153.58 Km and 124.00 Km respectively than their lower order streams. It was unexpected outcome and probably corresponds to the unique geophysical characteristics of the BRB. The cumulative length of all order streams in the basin is 16,493.279 Km. The first order stream which shares half of the total stream length of the BRB is ephemeral in dry season and perennial during rest of the seasons. The perennial length of first order stream in BRB decreases during dry seasons especially in upstream regions of the basin. The higher mean stream length (153.58 km) of 5th order streams indicates comparatively higher hydraulic conductivity by this order of streams than the streams of other orders in the basin. Further, slightly steeper slope of hilly regions in upper middle reaches of the basin have produced shorter stream length while low slope in lower reaches have generated longer stream length in the basin.
5.1.4. Stream length ratio (RL)
The stream length ratio denotes ratio between total stream lengths of a particular order and total stream length of its subsequent previous order (Horton, Citation1945). Stream length ratio, to extent, corresponds to the hydrological characteristics of a basin (Kabite & Gessesse, Citation2018). Variation of stream length ratio between successive orders of stream corresponds to the differences in topographic and slope characteristics of the basin and hence relates with surface flow discharge and different stages of erosion in the basin (Horton, Citation1945; Magesh & Chandrasekar, Citation2014). An increasing trend in stream length ratio from lower order to higher orders depicts attainment of geomorphic maturity (Thomas et al., Citation2010) while a deviation in trend corresponds to the delayed youth stage of stream’s geomorphic development (Sreedevi et al., Citation2009). The highest stream length ratio belongs to 5th order stream (0.79) whereas the lowest is of 6th order (0.24; ).
The inconsistencies in stream length ratio between successive stream orders represent uneven topography and spatial variation in infiltration rate in the basin. The calculated stream length ratio values of BRB are indicative of its mature geomorphic developments and low water regimes. The relatively higher stream length ratio of lowest order streams correspond the areas with low permeable formations basically the upper and middle regions of BRB.
5.1.5. Mean stream length ratio (Lsm)
The mean stream length represents the drainage network characteristics and properties with respect to basin surface features (Horton, Citation1945; Strahler, Citation1964). It has been derived by dividing the sum of calculated stream lengths ratios by total of stream segment number. The computed mean stream length ratio of BRB is 0.531. Generally, lower mean stream length ratio value corresponds to mountain environment and plateau or plain morphology is associated with higher value of mean stream length ratio (Rai et al., Citation2017). Normally, with the increase in streams order, the mean stream length also increases (Shrestha et al., Citation2017) but in this study, mean stream length depicts anomalies with respect to increasing order of streams. The higher value of mean stream length of 5th order streams in BRB represents that the streams of this order have continued their channel lengthening processes while successive order streams have aborted their channel lengthening. These abnormalities in mean stream length of BRB, corresponds to the variations in terrain gradient, uneven topography, coarse drainage pattern, and mature stages of geological developments of the basin. It has also brought discrepancies in stream length ratio and affects surface runoff characteristics of the basin.
5.1.6. Bifurcation ratio (Rb)
Bifurcation ratio is highly linked with the stream segmentation pattern and degree of stream distribution within the drainage network, hence signifies the hydro-morphological characteristics of a river basin. Bifurcation ratio in a drainage system is a proportion of the total numbers of stream segments of a specified order to the total number of stream of the subsequent higher order (Schumm, Citation1956). It reflects the level of integration among tributaries of various stream orders in a basin. Normally the bifurcation ratio value varies between 2 to 5 (Joji et al., Citation2013). Chow (Citation1964) stated that the bifurcation ratio values ranges from 3 to 5 reflect independency of drainage pattern from the basin’s geologic structures. Low mean bifurcation ratio values denote well-dissected, elongated drainage basins having high overland flow, high infiltration rate, high structural complexity, extended peak of discharge, and less chances of soil erosion and flood risk (Strahler, Citation1957; Thomas et al., Citation2010). The high mean values of bifurcation ratio imply circular drainage system with low infiltration rate, sharp peak flow, less groundwater recharge, higher chances of soil erosion, and flood risk (Reddy et al., Citation2004). Bifurcation ratio values () in BRB shows significant variation from 2 to 5.6, for different stream orders depicting it as a natural drainage system with dendritic drainage pattern (Joji et al., Citation2013). The higher bifurcation ratio between 1st and 2nd order streams than their successive higher orders represents accelerated state of erosion due to higher overland flow. The higher values of bifurcation ratio represent mature topographic regions of BRB while lower values imply plans regions of the basin (Magesh & Chandrasekar, Citation2014). The computed value of mean bifurcation ratio of entire BRB is 4.0. The result also signifies that the drainage network pattern in the basin is more or less gradient dependent rather than structurally controlled (Chow, Citation1964). The higher number of first-order streams and low bifurcation ratio in the BRB reflects its semi-elongated shape (Choudhari et al., Citation2018; Magesh et al., Citation2012). The low bifurcation ratio values between 5th to 6th and 6th to 7th order streams depicts the lower reaches of the basin having less number of streams of these orders under water stressed conditions. The higher bifurcation ratio values between 4th to 5th order streams in BRB indicate variation of terrain gradient and collection of bounteous water in upper basin area. The low mean bifurcation ratio of the BRB indicates that the Betwa drainage system is slope dependent with low water carrying capacity, low flood susceptibility and high water scarcity.
5.1.7. Sinuosity index (SI)
Sinuosity index or meander ratio is a factor which explains deviation of a river form its expected straight path (Schumm, Citation1956). It is calculated as proportion of the straight distance measured between the end points against actual length by the side of river meandering. Sinuosity index of a straight river will be 01. The sinuosity index value from 1 to 1.5 represents the river course as sinuous and sinuosity index value from 1.5 to 4 denotes river course as meandering (Leopold & Wolman, Citation1957). In this study, the calculated sinuosity index for the highest order of stream i.e., 7th order stream was 1.53. Hence, the stream course is basically in between sinuous and meandering typology.
5.1.8. Hydrological storage coefficient (Rho)
The hydrological storage coefficient or “Rho” coefficient (ρ) reflects storage potential of the drainage system (Horton, Citation1945). It correspond degree of drainage development with respect to the physiographic growth of the basin. The ratio of stream length to the bifurcation ratio is defined as “Rho” coefficient and it is influenced by geological, climatic, geo-morphological, and biological factors of the basin (Horton, Citation1945). Mean “Rho” coefficient of BRB is 0.15 which signifies that the basin has lower hydrological storage capacity.
5.2. Areal Aspects of River Basin Morphometry
Areal aspects deals with two-dimensional quantitative morphometric parameters such as basin area and shape, drainage texture, elongation ratio, circularity ratio, drainage density, stream frequency, and form factor. These factors more or less depend on the lithology and hydro-geomorphic conditions of the basins and relates with overland flow in different stream orders (Veeranna et al., Citation2017). Basin area implies a horizontal plane enclosed within water divides or ridgelines and encompasses streams of all orders of a basin, flowing to a common outlet. Discharge per unit area inversely relates with the size of the basin (Chorley, Citation1957). Perimeter denotes length of entire boundary stretch of a Basin. It illustrates shape and size of a drainage system and influences parameters like elongation ratio and circulatory ratio. shows that the computed basin length of the BRB is 437.93 Km. The total areal extension of the BRB is computed as 43,346.11 Km2 while (the outer boundary of the BRB that encloses its area) is calculated as 1643.74 Km.
Table 6. Results of areal geo-morphometric parameters.
5.2.1. Drainage density (Dd)
Drainage density (Dd) represents the ratio of cumulated length of streams of all orders within the basin to the total area of the drainage basin (; Horton, Citation1945). It is an expression of intricacy of stream arrangement in a drainage basin (Horton, Citation1932) and denoted in terms of Km/Km2. Generally, drainage density value in humid region ranges between 0.55 to 2.09 Km/Km2 (Joji et al., Citation2013). It is influenced by the climate, rock types, soil, terrain relief, permeability, runoff intensity, and vegetation cover of the basin (Bhat et al., Citation2019). Drainage density also reveals susceptibility of basins towards hydrological hazards, especially in areas where hydro-meteorological data are scared or absent (Obeidat et al., Citation2021). Generally, drainage density is categorize into four classes i.e., very high > 6 Km/Km2, high 4–6 Km/Km2, moderate 2–4 Km/Km2, low < 2 Km/Km2 (Gregory & Walling, Citation1968). Low drainage density value implies an area having coarse drainage texture, low relief, permeable sub-soil, compact vegetation cover, high infiltration capacity, less surface runoff, and higher potential of ground water whereas high drainage density values is characterized with fine drainage texture, elevated relief, impermeable sub-soil, peak runoff, low infiltration capacity, and sparse vegetation cover (Choudhari et al., Citation2018; Sahu et al., Citation2017). Drainage texture represents relative spacing of streams in a basin and depends on precipitation, soil type, vegetation, lithology, stage of development, and infiltration capacity . The underlying lithology and hard rock structure of BRB strongly influences the drainage network in the basin. The drainage density value of the basin is 0.38 Km/Km2 which reflect presence of poor and coarse drainage texture, lower relief with variation in vegetation coverage and to some extent permeable subsoil in the basin ().
5.2.3. Stream frequency (Fs)
Stream frequency (Fs) of a basin represents the ratio between cumulative stream segment number irrespective of their orders and basin area (Horton, Citation1945). Generally, drainage density and stream frequency exhibit a positive correlation, although it is also possible to have different stream frequencies with same drainage density. The climatic conditions, rock and soil types, vegetation thickness, terrain relief, infiltration capacity, permeability, and other runoff processes of a basin play vital role in controlling stream frequency distribution (Bali et al., Citation2012). Low value of stream frequency represents flat terrain and high infiltration or penetrability of the basin surface (Farhan, Citation2017). Greater stream frequency value of a basin indicates faster runoff and risk of flooding. The calculated stream frequency value for the BRB is 0.08 per Km2 () which indicates poor drainage network, plateau–plain, semi-humid environment, low permeability of rock, lesser relative relief and less available surface flow (Bali et al., Citation2012; Thomas et al., Citation2010).
5.2.4. Drainage texture (Dt)
Drainage texture is the ratio of the total number of stream sections of all orders per unit perimeter of a basin (Horton, Citation1945). It describes relative arrangement of drainage network in a basin. Drainage network arrangement generally depends on climate, slope, soil type, vegetation, rainfall, lithology and infiltration capacity of the basin (Horton, Citation1945; Smith and Clark, 1950; Soni, Citation2017). The regions having soft rock unprotected by vegetation bear finer texture, while resistant rocks results coarse drainage texture (Fenta et al., Citation2017). Finer the texture higher will be the dissection and erosion. As per the drainage texture classification of Smith and Clark, (1950), drainage texture value less or equal to 02 Km−1 represents very coarse texture, between 02 to 04 Km−1 denotes coarse texture, between 04 to 06 Km−1 implies moderate texture, between 06 to 08 Km−1indicates fine texture and values greater than 08 Km−1 shows ultra fine texture (Rai et al., Citation2018). The drainage texture value of BRB is 0.03 Km−1 which is less than 02 Km−1; hence the basin encompasses coarse drainage texture formed on basalt and granitic rocks spread in the form of mounds, ridges and plains having moderate degree of slopes ().
5.2.5. Form factor (Rf)
Form factor ratio is a dimensionless proportion between basin area and the square of the basin length (Horton, Citation1945). It indicates the flow intensity of a basin on a defined scale (Sreedevi et al., Citation2013). The form factor varies between zero to unity where value “0” indicates strongly elongated shape of basin and “1” suggests near-circular shape of basin. The form factor also depicts the runoff characteristics of a basin. Smaller form factor value with elongated basin shape will generate low runoff with long runoff duration whereas higher form factor value with circular basin, will produce high runoff for short duration leading floods (Bali et al., Citation2012). Elongated basin is generally associated with plateau–plain front where flood management is easier than that of circular basin of Mountain–plains front. The form factor value for a perfectly circular basin will always be less than the maximum threshold value of 0.7854 (Waikar & Nilawar, Citation2014). The estimated form factor value for the BRB is 0.23, which indicates elongated shape of the basin and slightly narrow rather than circular and thus it will generate low peak flow for longer duration.
5.2.6. Elongation ratio (Re)
Elongation ratio (Re) denotes the proportion of the diameter of a circle, whose area is identical with the area of the drainage basin to the maximum length of the basin (Schumm, Citation1956). Elongation ratio is dimensionless and represents the basin shape and hydrological characteristics. The value of elongation ratio generally ranges between “0” to “1” where zero depicts highly elongated basin shape and one represents highly circular shape of basin (Strahler, Citation1964). Generally, elongation ratio value near “1” is attained by the geo-morphologically controlled, sub-humid, mountainous, circular river basins (Magesh et al., Citation2013). Strahler (Citation1964) classified elongation ratio values and associated basin shape as circular (0.9–0.10), oval (0.8–0.9), less elongated (0.7–0.8), elongated (0.5–0.7) and more elongated (< 0.5) whereas Kumar et al. (Citation2015) have categorized elongation ratio value and related basin shape as circular (> 0.9), oval (0.9 to 0.8) and elongated (< 0.7). A circular basin is more vulnerable to flooding due to inflated runoff and short time of concentration than an elongated basin. The elongation ratio value of the BRB is 0.61 which infers that the basin have nearly elongated shape. Though, it is not entirely elongated but slightly elliptical in middle reaches due to the heterogeneous lithology in this portion of the basin. Elongation dominates upper and lower reaches of the basin. Elongation ratio also depicts that the basin have low terrain relief, gentle gradient with low peak discharge and plateau–plain front river basin.
5.2.7. Circulatory ratio (Rc)
Circularity ratio (Rc) is defined as dimensionless proportion of basin area with respect to a circle area having identical circumference to the basin perimeter (Miller, Citation1953; Pareta & Pareta, Citation2011; Strahler, Citation1964). The drainage density, stream frequency, geological structure, climatic conditions, relief, slope, land use/land cover etc. of any basin directly influences value of Circularity ratio. Miller (Citation1953) had classified basin shape on the basis of circulatory ratio varies between 0 (highly elongated) to 1 (highly circular). High, medium and low, values of the circularity ratio represent the older, mature and younger stages of basin development respectively. Generally, river basin having mountainous origin tends to form circular basins (high value of circularity ratio). They used to have young morphological profile and generate peak discharge during high rainfall seasons. Contrary to that elongated basin are generally found in plateau–plains (low value of circularity ratio) representing mature morphological adjustment and low discharge properties of the basin (Shaikh et al., Citation2022; Thomas et al., Citation2010). The calculated circularity ratio value of BRB is 0.20, which indicates low discharge of runoff, less prone to floods because excess water takes long time to reach at basin outlet. The low circularity ratio of BRB indicates near elongated characteristics of the basin with early to late mature landforms showing active denudational process in basin.
5.2.8. Length of overland flow (Lg)
The length of overland flow (Lg) is horizontally projected non-channel length of flow path of water on the ground, before it gets concentrated into certain stream channels (Horton, Citation1945). Length of overland flow shows inverse relation with terrain gradient hence low relief results a higher overland flow and vice versa (Kumar et al., Citation2015). Infiltration (exfiltration) and percolation properties of basin soil, average slope of the channel and basin drainage density significantly affects length of overland flow (Schmid, Citation1997). Higher length of overland flow value, in a basin represents longer distance covered by the water before getting accumulated into the stream channels. The length of overland flow in BRB is computed as 1.31 Km−1, which indicates low relief and rainfall has to add substantial amount of water to generate surface runoff in the region ().
5.2.9. Constant channel maintenance(C)
The reciprocal of drainage density is termed as Constant of channel maintenance (C; Schumm, Citation1956). It denotes the number of sq. unit of drainage area required to carry, maintain and develop one linear unit length of drainage channel and expressed in units of km2/km (Rekha et al., Citation2011). It informs about the infiltration and runoff characteristics of a basin. Dimensionally it is length and hence increases in magnitude with the increases in the scale of the landform unit. Higher constant of channel maintenance value represents the basin area dominated with lower order drainages, sparse vegetation cover, higher flood potentiality and young geo-morphological adjustment and vice-versa (Samal et al., Citation2015). The calculated constant channel maintenance of the BRB is 2.63 Km2/Km. It shows that on an average 2.63 Km2 basin surface area are needed for the development and maintenance of one Km long linear stream channel. Constant channel maintenance value of the BRB characterizes basin as plateau–plain environment with moderate permeability, moderate gradient, low drainage density, moderate bare soil infiltration and moderate surface runoff.
5.3. Relief morphometric parameters
Relief is an important aspect of any drainage system. It denotes the difference of elevation between lowest and highest point in any region. It represents three-dimensional morphometric parameters like basin relief, ruggedness number, relief ratio, gradient ratio, slope, river profile, and hypsometric curve. (a-d) shows the drainage density, stream frequency, dissection index and drainage texture of the BRB.
5.3.1. Basin relief (R)
Basin relief reflects actual elevation difference from the highest point (divide ridge) of the basin surface to the lowest point (basin outlet) on common basin surface. It represents basin’s denudational characteristics and significantly influences the landforms development, development of drainage, permeability, surface and subsurface water flow, flood patterns, erosional properties, sediment amount that can be transported and erosional stages of any river basin (Raux et al., Citation2011). Basin relief maintains the drainage channel gradient and hence represents basin’s potential energy of the draining.
An increase in basin relief leads steeper slopes, higher stream gradients, high erosion, low infiltration, high runoff conditions, and less time of concentration thereby increase in flood peak (Patton & Baker, Citation1976). The highest elevation of the BRB is 717 m which corresponds basically to the upper hilly regions of the basin and the lowest is 86 m. The total relative relief of the whole BRB is 631 m (). The basin relief value of BRB indicates dissected and erosional landforms, moderate stream gradient, moderate runoff and geo-morphologically mature stages of the basin development (Rai et al., Citation2017).
Table 7. Results of relief aspects and respective unit.
5.3.2. Relief ratio (Rh)
Relief ratio (Rh) denotes the dimensionless proportion of basin relief to the longest parallel length along the principle drainage channel towards the outlet (Schumm, Citation1956). It basically refracts the slope scenario of the basin which affects hydro-geomorphic characteristics of a basin (Rajasekhar et al., Citation2020). Higher value of relief ratio represents steeper slope, high basin discharges, faster runoff, greater erosive power and vice-versa. Generally, the value of relief ratio decreases with increase in the area and size of the basin. Mountainous river basins having circular basin shape, high basin relief and small basin area generates high value of relief ratio while low values of relief ratio are associated with the plateau river basin having ridges and mounds with moderate degree of slope (Thomas et al., Citation2010). The calculated relief ratio value of the BRB is 0.0014, which is very low and represents nearly elongated shape of the basin, moderate basin relief and maximum denudation stages of geomorphic developments ().
5.3.3. Ruggedness number (Rn)
Ruggedness number is calculated by multiplying drainage density with relative relief of basin. It is a dimensionless unit which depicts the structural complexity and unevenness of the terrain. High drainage density and high relative relief results high ruggedness number (Ansari et al., Citation2012). The Rn value depends on climate, slope, steepness, geology, geomorphology, vegetation cover, etc., of a region. It affects stream energy, surface storage capacity, runoff velocity, and routing at the basin scale. High Rn reflects extremely uneven topography, increased peak discharge, primary stage of denudation activities and vice-versa (Ozdemir & Bird, Citation2009). Mountainous basins have usually higher ruggedness number value than that of plateau river basin. The calculated Rn value for BRB is 0.24, which indicates maximum denudation and mature stages of the basin (). It is also characterized by the granite–gneiss dominated geology and rolling plateau fringe landforms.
5.3.4. Dissection index (Dis)
Dissection index of any physiographic region attributes phases of its landform development, degree of vertical erosion and magnitude of terrain dissection in the region (Schumm, Citation1956). The proportion of maximum relative relief to the maximum absolute relief of the basin gives dissection index. It represents ratio of actual dissection created by the rivers to the potential dissection up to base levels. Dissection index value ranges between 0 to 1, where 0 implies presence of flat terrain, less degree of dissection, absence of vertical erosion and old stage of the basin while 1 indicates undulated terrain, hill slope escarpments, cliffs or at seashore. The value of dissection index of BRB is ranges from 0.06 to 0.3 (), which reflects less degree of dissection and mature stage of the basin ().
5.3.4. Hypsometric curve
A graphical plot derived using relative basin area along abscissa and relative altitudes along ordinate represents hypsometric curve. It is performed to examine the connection between horizontal cross-sectional areas of a basin with its elevation and has also been known as drainage-basin relief graph. It reveals information pertinent to the stages of geological successions and susceptibility of basin towards erosion (Singh & Singh, Citation2018). It is strongly influenced by the climate, tectonics, lithology and other geological conditions of a basin. The hypsometric curve reflects stages of erodibility in different parts of the basin. It tells about the regions in the basin which are more or less eroded or denuded in comparison to other regions. Strahler (Citation1952a) stated that, the convex hypsometric curve represents non-equilibrium youth stage of a basin i.e., the basin is highly susceptibility towards erosion. An S-shape hypsometric curve which is concave upwards at higher altitudes and convex downwards at lower elevations represents mature stage of basin (Strahler, Citation1952b). A basin at mature stage shows less susceptibility towards erosion (Sharma & Mahajan, Citation2020). A concave hypsometric curve indicates that the basin is in monadnock phase, i.e., highly matured stage which is least susceptible to erosion. A more mature river basin produces more concave hypsometric curve. We have performed hypsometric curve analysis of BRB using formula of Ritter et al. (Citation2002). The S-shape curve of BRB shown in indicates that the upper portion of the basin is in equilibrium stage and less prone to erosion while the lower portion of the basin is in an in-equilibrium young stage and more susceptible for erosion. shows longitudinal profile of BRB along Betwa River and corresponds to the differences in altitude from origin to the confluence with Yamuna River. It also depicts that the upper to middle portion of the basin have moderate stream gradient compare to the steeper stream gradient of lower portion of the basin.
5.4. The topo-hydrological aspects
To assess the overland flow, erosion risk and sediment transport in the basin topographic indices were computed (EquationEquations. 2(2)
(2) –Equation5
(5)
(5) ). The topo-hydrological features of BRB have been extracted from COP DEM. , Maps showing the values of the topo-hydrological factors within the study area. (a) topographic wetness index (TWI), (b) stream power index (SPI), (c) terrain ruggedness index (TRI), (d) sediment transport index (STI).
Figure 11. Maps showing the values of the topo-hydrological indices within the study area, (a) topographic wetness index (TWI), (b) stream power index (SPI), (c) terrain ruggedness index (TRI), and (d) sediment transport index (STI).

5.4.1. Topographic wetness index (TWI)
It is a topo-hydrological factor introduced by Beven et al. (Citation1984). It represents the effects of topography on hydrological processes e.g., over land flow generation and accumulation; identification of hydrological flow paths; spatial patterns of soil moisture etc. in the river basin (Gallant & Wilson, Citation1996; Sørensen & Seibert, Citation2007). Areas having higher value of TWI represent wetter & gentle slope areas, having greater potential to be saturated by water before the surrounding areas and vice versa. It indicates area with higher propensity to water accumulation and runoff-generation in the basin. Moore et al. (Citation1991) have introduced following equation for TWI computation assuming uniform soil properties and steady state conditions in the study area:
Where, “ln” denotes the natural logarithm, “As” represents specific basin area (m2m−1) i.e., the upslope contributing area per unit contour length and “β” stands for the slope gradient in degree.
The computed TWI value in BRB ranges from 3.13 to 26.25, where higher value spread along the flat plains in the basin and lower values are associated with rocky high lands and hills of the basin ()).
5.4.2. Stream power index (SPI)
It represents the interconnection between stream’s energy expenditure with time and quantity of sediment transported by the stream (Bagnold, Citation1966). It is a product of tangent of the slope in degrees and area of the basin. SPI is an important parameter in sediment transport studies and reflects stream’s power to transport water and sediment per unit of flow length. It is an important factor which initiates channel development in a river basin (Barman et al., Citation2021). It is closely related with TWI and escalates with the increase in slope steepness and size of the basin. It reflects potential erosive power of over land flow and influenced by the soil quality, soil profile, vegetation types, coverage and distribution, precipitation and climate (Moore et al., Citation1991). A positive SPI value indicates potential erosive areas while negative SPI value shows topographic areas having potential for depositional. Moore et al. (Citation1991) had provided following equation for SPI calculation with an assumption that the discharge is proportional to the basin area.
Where, “As” denotes specific basin area (m2m−1) i.e., the upslope contributing area per unit contour length and “β” refers to the slope gradient in degree.
The SPI value in BRB varies from 0 to 6 ()). The low range of SPI value reflects old stage of the basin and shows that the overland flow in the basin has lower erosive power. Most of the area in BRB showed SPI value zero that is less susceptibility towards erosion while few regions with high flow accumulation potential (river bed) have shown value 6.
5.4.3. Terrain roughness index (TRI)
The TRI factor influence stream energy, runoff velocity, surface storage capacity, and routing at the basin scale. It reflects the amount of elevation difference between neighboring cells and calculated by measuring the elevation differences between the focal cell and eight neighboring cells. It reflects average elevation change between any point on a grid and its surrounding areas.
Where, Abs means absolute and max and min are elevation in meter,
TRI value in BRB ranged from 0 to 30 ()). TRI value zero corresponds to the flat plains of the basin while 30 indicate hills and ridges of the basin.
5.4.4. Sediment transport index (STI)
It has been introduced by Moore and Burch (Citation1986) for characterization of erosion and deposition processes. It is correlated with soil erosion as it demonstrates the convergence and divergence of sediments flow from the region of higher elevation to the lower elevation region which are susceptible for inundation and sediment accumulation.
Where, “As” is the specific basin area (m2m−1) i.e., the upslope contributing area per unit contour length, “β” denotes the local slope gradient in degrees, “m” is area exponent and “n” is slope exponent.
STI value in BRB ranged from 0 to 121.4 ()). Larger value of STI generally belongs to areas dominated by steep slopes, ravines and water streams, and corresponding areas having higher susceptibility to soil erosion and degradation.
The appendix (field photographs, 1–4) depicting the intermittent nature of river in upper reaches, geological formations, vegetations and erosive and depositional characteristics of Betwa River Basin.
6. Conclusion
The present study demonstrates that the newly released COP-DEM is an efficient DEM for geo-morphometric and topo-hydrological analysis. The CLC analysis showed that the drainage networks delineation was performed with better accuracy. Our analysis suggests that the BRB falls under seventh order basin with high number of headwater streams. High number of headwater streams dominates upper to middle reaches of the BRB and infers relatively higher relief with steeper slopes, rocky outcrops, dissected plateau and hills. The drainage basin have mostly dendritic to sub-dendritic (parallel) drainage pattern while at some places radial and trellised patterns also co- exists which implies that the river channel is following the terrain gradient. The slope map of the basin also affirms that the basin have mostly east facing and relatively gentle slope gradient. However, due to local geological factors such as rugged terrain, moderately dissected topography with underlain rocks of Archaean–Proterozoic age and Deccan Trap rocks of Cretaceous age at few places the river shows sinuous and meandering phenomenon. Lower mean bifurcation ratio, less circularity ratio, low elongation ratio, very low overall drainage density, low stream frequency and small drainage texture ratio of BRB infers well-dissected, slope dependent, near elongated drainage basin having high overland flow, low infiltration rate and high structural complexity. A high stream frequency and drainage density have been noticed in Deccan Trap part of the Betwa River basin as compare to those of Vindhyan Supergroup and Bundelkhand complex.
Conspicuously coarse drainage texture is characteristically present in Bundelkhand massif which is due to presence of sub-surface jointed and fractured lithology of granite dominated terrain. Further, the basin relief, ruggedness number, relief ratio and hypsometric curve depict less erosion intensity in lower parts of the basin due to attainment of mature and maximum denudation stages of erosion in this region and low terrain relief of the basin. In addition to that the values of topo-hydrological indices viz. TWI, SPI, TRI and STI also infer that the upper portion of the BRB have less soil moisture content, high overland flow, high ruggedness and higher erosion potential. We recommend special attention towards the upper part of the Betwa River Basin which needs immediate basin conservation interventions to ensure sustainability of BRB.
Acknowledgments
The first author wishes to acknowledge the support received from the University Grants Commission (UGC), New Delhi, India for providing grant under UGC NET-JRF fellowship (Ref no. 3622/(NET-JUNE 2014). Authors also express thanks to DST-FIST, New Delhi, India for providing necessary facilities for the research and development activities at our Centre.
Disclosure statement
No potential conflict of interest was reported by the author(s).
Additional information
Funding
References
- Abrams, M., Crippen, R., & Fujisada, H. (2020). ASTER global digital elevation model (GDEM) and ASTER global water body dataset (ASTWBD). Remote Sensing, 12(7), 1156. https://doi.org/10.3390/rs12071156
- Ahlawat, R. (2010). Space-time variation in rainfall and runoff: Upper Betwa catchment. International Journal Environmental Earth Science, 1(2), 90–96. http://citeseerx.ist.psu.edu/viewdoc/summary?doi=10.1.1.309.9352
- Alilou, H., Rahmati, O., Singh, V. P., Choubin, B., Pradhan, B., Keesstra, S., Ghiasi, S. S., & Sadeghi, S. H. (2019). Evaluation of watershed health using Fuzzy-ANP approach considering geo-environmental and topo-hydrological criteria. Journal of Environmental Management, 232, 22–36. https://doi.org/10.1016/j.jenvman.2018.11.019
- Allan, J. D., Castillo, M. M., & Capps, K. A. (2020). Stream ecology: Structure and function of running waters. Springer Nature.
- Anish, A. U., Baiju, K. R., Thomas, P. K., Anns, M., Rajkumar, P. B., & Babu, S. (2021). Status of GIS-enabled morphometric analysis of river basins of Kerala, Southern India: A review and assessment. Regional Studies in Marine Science, 44, 101792. https://doi.org/10.1016/J.RSMA.2021.101792
- Ansari, Z. R., Rao, L. A. K., & Yusuf, A. (2012). GIS based morphometric analysis of Yamuna drainage network in parts of Fatehabad area of Agra District, Uttar Pradesh. Journal of the Geological Society of India, 79(5), 505–514. https://doi.org/10.1007/s12594-012-0075-2
- Arulbalaji, P., & Padmalal, D. (2020). Sub-watershed prioritization based on drainage morphometric analysis: A case study of Cauvery River Basin in South India. Journal of the Geological Society of India, 95(1), 25–35. https://doi.org/10.1007/s12594-020-1383-6
- Avtar, R., Singh, C. K., Shashtri, S., Singh, A., & Mukherjee, S. (2010). Identification and analysis of groundwater potential zones in Ken–Betwa river linking area using remote sensing and geographic information system. Geocarto International, 25(5), 379–396. https://doi.org/10.1080/10106041003731318
- Bagnold, R. A. (1966). An approach to the sediment transport problem from general physics. USGS Professional Paper, US government printing office, Washington, DC. https://doi.org/10.3133/pp422I
- Bali, R., Agarwal, K. K., Nawaz Ali, S., Rastogi, S. K., & Krishna, K. (2012). Drainage morphometry of Himalayan Glacio-fluvial basin, India: Hydrologic and neotectonic implications. Environmental Earth Sciences, 66(4), 1163–1174. https://doi.org/10.1007/s12665-011-1324-1
- Banerjee, A., Singh, P., & Pratap, K. (2017). Morphometric evaluation of Swarnrekha watershed, Madhya Pradesh, India: An integrated GIS-based approach. Applied Water Science, 7(4), 1807–1815. https://doi.org/10.1007/s13201-015-0354-3
- Barman B Kumar, Rao C Udaya, Rao K Srinivasa, Patel A, Kushwaha K and Singh S Kumar. (2021). Geomorphic Analysis, Morphometric-based Prioritization and Tectonic Implications in Chite Lui River, Northeast India. J Geol Soc India, 97(4), 385–395. https://doi.org/10.1007/s12594-021-1696-0
- Beven, K. J., Kirkby, M. J., Schofield, N., & Tagg, A. F. (1984). Testing a physically-based flood forecasting model (TOPMODEL) for three UK catchments. Journal of Hydrology, 69(1–4), 119–143. https://doi.org/10.1016/0022-1694(84)90159-8
- Bhardwaj, A., Jain, K., & Chatterjee, R. S. (2019). Generation of high-quality digital elevation models by assimilation of remote sensing-based DEMs. Journal of Applied Remote Sensing, 13(4), 044502. https://doi.org/10.1117/1.JRS.13.4.044502
- Bhat, A. H., Askary, T. H., Ahmad, M. J., Chaubey, A. K., & Chaubey, A. K. (2019). Description of heterorhabditis bacteriophora (Nematoda: Heterorhabditidae) isolated from hilly areas of Kashmir Valley. Egyptian Journal of Biological Pest Control, 29(1), 1–7. https://doi.org/10.1186/s41938-019-0197-6
- Brunsden, D. (2001). A critical assessment of the sensitivity concept in geomorphology. Catena, 42(2–4), 99–123. https://doi.org/10.1016/S0341-8162(00)00134-X
- Bryndal, T., Kroczak, R., Kijowska-Strugała, M., & Bochenek, W. (2020). How human interference changes the drainage network operating during heavy rainfalls in a medium-high relief flysch mountain catchment? The case study of the Bystrzanka catchment (Outer Carpathians, Poland). Catena, 194, 104662. https://doi.org/10.1016/j.catena.2020.104662
- Buckley, S., Agram, P., Belz, J., Crippen, R. E., Gurrola, E. M., Hensley, S., et al. (2020). NASADEM user guide version 1. Califronia Institute of Technology. https://lpdaac.usgs.gov/documents/592/NASADEM_User_Guide_V1.pdf
- Burrough, P. A., McDonnell, R. A., & Lloyd, C. D. (2015). Principles of geographical information systems. Oxford university press.
- Caglar, B., Becek, K., Mekik, C., & Ozendi, M. (2018). On the vertical accuracy of the ALOS world 3D-30m digital elevation model. Remote Sensing Letters, 9(6), 607–615. https://doi.org/10.1080/2150704X.2018.1453174
- Chandramouli, C. (2011). Census of India. Rural urban distribution of population, provisional population total. Office of the Registrar General and Census Commissioner, India.
- Chaube, U. C., Suryavanshi, S., Nurzaman, L., & Pandey, A. (2011). Synthesis of flow series of tributaries in Upper Betwa basin. International Journal of Environmental Sciences, 1(7), 1459–1475. https://indianjournals.com/ijor.aspx?target=ijor:ijes&volume=1&issue=7&article=007
- Chopra, R., Dhiman, R. D., & Sharma, P. K. (2005). Morphometric analysis of sub-watersheds in Gurdaspur district, Punjab using remote sensing and GIS techniques. Journal of the Indian Society of Remote Sensing, 33(4), 531–539. https://doi.org/10.1007/BF02990738
- Chorley, R. J. (1957). Climate and morphometry. The Journal of Geology, 65(6), 628–638. https://doi.org/10.1086/626468
- Choudhari, P. P., Nigam, G. K., Singh, S. K., & Thakur, S. (2018). Morphometric based prioritization of watershed for groundwater potential of Mula river basin, Maharashtra, India. Geology, Ecology, and Landscapes, 2(4), 256–267. https://doi.org/10.1080/24749508.2018.1452482
- Chow, V. T. (editor). (1964). Handbook of applied hydrology. McGraw Hill.
- Danielson, J. J., & Gesch, D. B. (2011). Global multi-resolution terrain elevation data 2010 (GMTED2010). US Department of the Interior, US Geological Survey.
- de Ferranti, J. (2014) Digital Elevation Data—with SRTM voids filled using accurate topographic mapping. http://viewfinderpanoramas.org/
- Desai, S., Singh, D. K., Islam, A., & Sarangi, A. (2021). Impact of climate change on the hydrology of a semi-arid river basin of India under hypothetical and projected climate change scenarios. Journal of Water and Climate Change, 12(3), 969–996. https://doi.org/10.2166/wcc.2020.287
- Dubey, S. K., Sharma, D., & Mundetia, N. (2015). Morphometric analysis of the Banas River Basin using geographical information system, Rajasthan, India. Hydrology, 3(5), 47–57. https://doi.org/10.11648/j.hyd.20150305.11
- Dubey, S. K., Sharma, D., Babel, M. S., & Mundetia, N. (2020). Application of hydrological model for assessment of water security using multi-model ensemble of CORDEX-South Asia experiments in a semi-arid river basin of India. Ecological Engineering, 143, 105641. https://doi.org/10.1016/j.ecoleng.2019.105641
- EORC, J. (2021). ALOS global digital surface model (DSM). ALOS World, 3D-30m (AW3D30). 1–17. Version, 3(3.1). https://www.eorc.jaxa.jp/ALOS/en/dataset/aw3d30/data/aw3d30v3.2_product_e_e1.2.pdf
- Fahrland, E., Jacob, P., Schrader, H., & Kahabka, H. (2020). Copernicus Digital Elevation Model Validation Report. Tech. Rep. GEO.2018-1988-2, AIRBUS. https://spacedata.copernicus.eu/documents/20126/0/GEO1988-CopernicusDEM-SPE-002_ProductHandbook_I1.00.pdf
- Fairfield, J., & Leymarie, P. (1991). Drainage networks from grid digital elevation models. Water Resources Research, 27(5), 709–717. https://doi.org/10.1029/90WR02658
- Farhan, Y. (2017). morphometric assessment of Wadi Wala Watershed, Southern Jordan using ASTER (DEM) and GIS. Journal of Geographic Information System, 9(2), 158–190. https://doi.org/10.4236/jgis.2017.92011
- Farr, T. G., Rosen, P. A., Caro, E., Crippen, R., Duren, R., Hensley, S., Kobrick, M., Paller, M., Rodriguez, E., Roth, L., Seal, D., Shaffer, S., Shimada, J., Umland, J., Werner, M., Oskin, M., Burbank, D., & Alsdorf, D. (2007). The shuttle radar topography mission. Reviews of Geophysics, 45(2), 1–33. https://doi.org/10.1029/2005RG000183
- Fenta, A. A., Yasuda, H., Shimizu, K., Haregeweyn, N., & Woldearegay, K. (2017). Quantitative analysis and implications of drainage morphometry of the Agula watershed in the semi-arid northern Ethiopia. Applied Water Science, 7(7), 3825–3840. https://doi.org/10.1007/s13201-017-0534-4
- Fryirs, K. A. (2017). River sensitivity: A lost foundation concept in fluvial geomorphology. Earth Surface Processes and Landforms, 42(1), 55–70. https://doi.org/10.1002/esp.3940
- Gallant, J. C., & Wilson, J. (1996). TAPES-G: A grid-based terrain analysis program for the environmental sciences. Computers & Geosciences, 22(7), 713–722. https://doi.org/10.1016/0098-3004(96)00002-7
- Gregory, K. J., & Walling, D. E. (1968). The variation of drainage density within a catchment. Hydrological Sciences Journal, 13(2), 61–68. https://doi.org/10.1080/02626666809493583
- Guth, P. L. (2011). Drainage basin morphometry: A global snapshot from the shuttle radar topography mission. Hydrology and Earth System Sciences, 15(7), 2091–2099. https://doi.org/10.5194/hess-15-2091-2011
- Haghipour, N., & Burg, J. P. (2014). Geomorphological analysis of the drainage system on the growing Makran accretionary wedge. Geomorphology, 209, 111–132. https://doi.org/10.1016/j.geomorph.2013.11.030
- Harsha, J., Ravikumar, A. S., & Shivakumar, B. L. (2020). Evaluation of morphometric parameters and hypsometric curve of Arkavathy river basin using RS and GIS techniques. Applied Water Science, 10(3), 1–15. https://doi.org/10.1007/s13201-020-1164-9
- Hawker, L., Neal, J., & Bates, P. (2019). Accuracy assessment of the TanDEM-X 90 digital elevation model for selected floodplain sites. Remote Sensing of Environment, 232, 111319. https://doi.org/10.1016/j.rse.2019.111319
- Horton, R. E. (1932). Drainage-basin characteristics. Transactions, American Geophysical Union, 13(1), 350–361. https://doi.org/10.1029/TR013i001p00350
- Horton, R. E. (1945). Erosional development of streams and their drainage basins; hydrophysical approach to quantitative morphology. Geological Society of America Bulletin, 56(3), 275–370.
- Intermap (2018). NEXTMap® World 30TM Data Sheet. https://www.intermap.com/hubfs/NEXTMap_World30_Data-Sheet.pdf
- Joji, V. S., Nair, A. S. K., & Baiju, K. V. (2013). Drainage basin delineation and quantitative analysis of Panamaram Watershed of Kabani River Basin, Kerala using remote sensing and GIS. Journal of the Geological Society of India, 82(4), 368–378. https://doi.org/10.1007/s12594-013-0164-x
- Jothimani, M., Dawit, Z., & Mulualem, W. (2021). Flood susceptibility modeling of Megech river catchment, lake tana basin, north western Ethiopia, using morphometric analysis. Earth Systems and Environment, 5(2), 353–364. https://doi.org/10.1007/s41748-020-00173-7
- Kabite, G., & Gessesse, B. (2018). Hydro-geomorphological characterization of Dhidhessa River basin, Ethiopia. International Soil and Water Conservation Research, 6(2), 175–183. https://doi.org/10.1016/j.iswcr.2018.02.003
- Kanhaiya, S., Singh, B. P., Singh, S., Mittal, P., & Srivastava, V. K. (2018). Morphometric analysis, bed-load sediments, and weathering intensity in the Khurar River Basin, central India. Geological Journal 54 (1) , 466–481. https://doi.org/10.1002/gj.3194
- Kanhaiya, S., Singh, S., Singh, C. K., Srivastava, V. K., & Patra, A. (2019). Geomorphic evolution of the Dongar River Basin, Son Valley, Central India. Geology, Ecology, and Landscapes, 3(4), 269–281. https://doi.org/10.1080/24749508.2018.1558019
- Kumar, A., Samuel, S. K., & Vyas, V. (2015). Morphometric analysis of six sub-watersheds in the central zone of Narmada River. Arabian Journal of Geosciences, 8(8), 5685–5712. https://doi.org/10.1007/s12517-014-1655-9
- Kumar, D., Gautam, A. K., Palmate, S. S., Pandey, A., Suryavanshi, S., Rathore, N., & Sharma, N. (2017). Evaluation of TRMM multi-satellite precipitation analysis (TMPA) against terrestrial measurement over a humid sub-tropical basin, India. Theoretical and Applied Climatology, 129(3), 783–799. https://doi.org/10.1007/s00704-016-1807-9
- Kumar, B., Venkatesh, M., & Triphati, A. (2018). A GIS-based approach in drainage morphometric analysis of Rihand River Basin, Central India. Sustainable Water Resources Management, 4(1), 45–54. https://doi.org/10.1007/s40899-017-0118-3
- Kumar, N., Singh, S. K., & Pandey, H. K. (2018). Drainage morphometric analysis using open access earth observation datasets in a drought-affected part of Bundelkhand, India. Applied Geomatics, 10(3), 173–189. https://doi.org/10.1007/s12518-018-0218-2
- Kumar, N., & Singh, S. K. (2021a). Soil erosion assessment using earth observation data in a trans-boundary river basin. Natural Hazards, 107(1), 1–34. https://doi.org/10.1007/s11069-021-04571-6
- Kumar, N., Singh, S. K., Dubey, A. K., Ray, R. L., Mustak, S., & Rawat, K. S. (2021b). Prediction of soil erosion risk using earth observation data under recent emission scenarios of CMIP6. Geocarto International, 1–24. https://doi.org/10.1080/10106049.2021.1973116
- Lakshmi, S. E., & Yarrakula, K. (2018). Review and critical analysis on digital elevation models. G Eofizika, 35(2), 129–157. https://doi.org/10.15233/gfz.2018.35.7
- Leister-Taylor, V., Jacob, P., Schrader, H., & Kahabka, H. (2020). Copernicus digital elevation model product handbook. Tech. Rep. GEO.2018-1988-2, AIRBUS. https://spacedata.copernicus.eu/documents/20126/0/GEO1988-CopernicusDEM-RP-001_ValidationReport_I3.0.pdf
- Leopold, L. B., & Wolman, M. G. (1957). River channel patterns: Braided, meandering, and straight. US Government Printing Office.
- Leopold, L. B., Wolman, M. G., Miller, J. P., Wohl, E., & Wohl, E. E. (2020). Fluvial processes in geomorphology. Courier Dover Publications.
- Magesh, N. S., Chandrasekar, N., & Soundranayagam, J. P. (2011). Morphometric evaluation of Papanasam and Manimuthar watersheds, parts of Western Ghats, Tirunelveli district, Tamil Nadu, India: A GIS approach. Environmental Earth Sciences, 64(2), 373–381. https://doi.org/10.1007/s12665-010-0860-4
- Magesh, N. S., Chandrasekar, N., & Soundranayagam, J. P. (2012). Delineation of groundwater potential zones in Theni district, Tamil Nadu, using remote sensing, GIS and MIF techniques. Geoscience Frontiers, 3(2), 189–196. https://doi.org/10.1016/j.gsf.2011.10.007
- Magesh, N. S., Jitheshlal, K. V., Chandrasekar, N., & Jini, K. V. (2013). Geographical information system-based morphometric analysis of Bharathapuzha river basin, Kerala, India. Applied Water Science, 3(2), 467–477. https://doi.org/10.1007/s13201-013-0095-0
- Magesh, N. S., & Chandrasekar, N. (2014). GIS model-based morphometric evaluation of Tamiraparani subbasin, Tirunelveli district, Tamil Nadu, India. Arabian Journal of Geosciences, 7(1), 131–141. https://doi.org/10.1007/s12517-012-0742-z
- Malviya, V. P., Arima, M., Pati, J. K., & Kaneko, Y. (2006). Petrology and geochemistry of metamorphosed basaltic pillow lava and basaltic komatiite in the Mauranipur area: Subduction related volcanism in the Archean Bundelkhand craton, Central India. Journal of Mineralogical and Petrological Sciences, 101(4), 199–217. https://doi.org/10.2465/jmps.101.199
- Mark, D. M. (1983). Relations between field-surveyed channel networks and map-based geomorphometric measures, Inez, Kentucky. Annals of the Association of American Geographers, 73(3), 358–372. https://doi.org/10.1111/j.1467-8306.1983.tb01422.x
- Miller, V. C. (1953). A quantitative geomorphic study of drainage basin characteristics in the clinch mountain area Virginia and Tennessee. Department of Geology Columbia University, New York,389–402 .
- Moore, I. D., & Burch, G. J. (1986). Physical basis of the length‐slope factor in the universal soil loss equation. Soil Science Society of America Journal, 50(5), 1294–1298. https://doi.org/10.2136/sssaj1986.03615995005000050042x
- Moore, I. D., Grayson, R. B., & Ladson, A. R. (1991). Digital terrain modelling: A review of hydrological, geomorphological, and biological applications. Hydrological Processes, 5(1), 3–30. https://doi.org/10.1002/hyp.3360050103
- Muralikrishnan, S., Pillai, A., Narender, B., Reddy, S., Venkataraman, V. R., & Dadhwal, V. K. (2013). Validation of Indian national DEM from Cartosat-1 data. Journal of the Indian Society of Remote Sensing, 41(1), 1–13. https://doi.org/10.1007/s12524-012-0212-9
- O’Loughlin, F. E., Paiva, R. C., Durand, M., Alsdorf, D. E., & Bates, P. D. (2016). A multi-sensor approach towards a global vegetation corrected SRTM DEM product. Remote Sensing of Environment, 182, 49–59. https://doi.org/10.1016/j.rse.2016.04.018
- Obeidat, M., Awawdeh, M., & Al Hantouli, F. (2021). Morphometric analysis and prioritisation of watersheds for flood risk management in Wadi Easal Basin (WEB), Jordan, using geospatial technologies. Journal of Flood Risk Management, 14(2), e12711. https://doi.org/10.1111/jfr3.12711
- Observer, P. (2017). PlanetDEM 30 Plus. Available at: https://www.planetobserver.com/products/planetdem/planetdem-30/
- Ozdemir, H., & Bird, D. (2009). Evaluation of morphometric parameters of drainage networks derived from topographic maps and DEM in point of floods. Environmental Geology, 56(7), 1405–1415. https://doi.org/10.1007/s00254-008-1235-y
- Pandey, R. P., Mishra, S. K., Singh, R., & Ramasastri, K. S. (2008). Streamflow drought severity analysis of Betwa river system (India). Water Resources Management, 22(8), 1127–1141. https://doi.org/10.1007/s11269-007-9216-6
- Pandule, A. M. (2019). Morphometric Analysis of Sina basin using GIS and remote sensing techniques. International ResearchJournal EngineersTechnology, 6(4) , 5212–5229. https://www.irjet.net/archives/V6/i4/IRJET-V6I41116.pdf
- Pareta, K., & Pareta, U. (2011). Quantitative morphometric analysis of a watershed of Yamuna basin, India using ASTER (DEM) data and GIS. International Journal of Geomatics and Geosciences, 2(1), 248. http://citeseerx.ist.psu.edu/viewdoc/summary?doi=10.1.1.298.2002
- Patel A, Singh M M, Singh S Kumar, Kushwaha K and Singh R. (2022). AHP and TOPSIS Based Sub-Watershed Prioritization and Tectonic Analysis of Ami River Basin, Uttar Pradesh. J Geol Soc India, 98(3), 423–430. https://doi.org/10.1007/s12594-022-1995-0
- Pati, J. K., Patel, S. C., Pruseth, K. L., Malviya, V. P., Arima, M., Raju, S., Prakash, K., & Prakash, K. (2007). Geology and geochemistry of giant quartz veins from the Bundelkhand Craton, central India and their implications. Journal of Earth System Science, 116(6), 497–510. https://doi.org/10.1007/s12040-007-0046-y
- Patton, P. C., & Baker, V. R. (1976). Morphometry and floods in small drainage basins subject to diverse hydrogeomorphic controls. Water Resources Research, 12(5), 941–952. https://doi.org/10.1029/WR012i005p00941
- Polidori, L., & El Hage, M. (2020). Digital elevation model quality assessment methods: A critical review. Remote Sensing, 12, 3522. https://doi.org/10.3390/rs1221352221
- Prakash, K., Chaubey, K., Singh, S., Mohanty, T., & Singh, C. K. (2017). Morphometric analysis of the Satna river basin, Central India. Indian Journal Geomorphologie, 22(1), 41–60.
- Prakash, K., Rawat, D., Singh, S., Chaubey, K., Kanhaiya, S., & Mohanty, T. (2019). Morphometric analysis using SRTM and GIS in synergy with depiction: A case study of the Karmanasa River basin, North central India. Applied Water Science, 9(1), 1–10. https://doi.org/10.1007/s13201-018-0887-3
- Prasannakumar, V., Vijith, H., & Geetha, N. (2013). Terrain evaluation through the assessment of geomorphometric parameters using DEM and GIS: Case study of two major sub-watersheds in Attapady, South India. Arabian Journal of Geosciences, 6(4), 1141–1151. https://doi.org/10.1007/s12517-011-0408-2
- Product description edition 1.2 version 3.2/3.1 (Tech. rep., Japan Aerospace Exploration Agency). https://www.eorc.jaxa.jp/ALOS/en/aw3d30/aw3d30v3.2_product_e_e1.2.pdf
- Rai, P. K., Mohan, K., Mishra, S., Ahmad, A., & Mishra, V. N. (2017). A GIS-based approach in drainage morphometric analysis of Kanhar River Basin, India. Applied Water Science, 7(1), 217–232. https://doi.org/10.1007/s13201-014-0238-y
- Rai, P. K., Chandel, R. S., Mishra, V. N., & Singh, P. (2018). Hydrological inferences through morphometric analysis of lower Kosi river basin of India for water resource management based on remote sensing data. Applied Water Science, 8(1), 1–16. https://doi.org/10.1007/s13201-018-0660-7
- Rajasekhar, M., Raju, G. S., & Raju, R. S. (2020). Morphometric analysis of the Jilledubanderu river basin, Anantapur District, Andhra Pradesh, India, using geospatial technologies. Groundwater for Sustainable Development, 11, 100434. https://doi.org/10.1016/j.gsd.2020.100434
- Raux, J., Copard, Y., Laignel, B., Fournier, M., & Masseï, N. (2011). Classification of worldwide drainage basins through the multivariate analysis of variables controlling their hydrosedimentary response. Global and Planetary Change, 76(3–4), 117–127. https://doi.org/10.1016/j.gloplacha.2010.12.005
- Reddy, G. P. O., Maji, A. K., & Gajbhiye, K. S. (2004). Drainage morphometry and its influence on landform characteristics in a basaltic terrain, Central India–a remote sensing and GIS approach. International Journal of Applied Earth Observation and Geoinformation, 6(1), 1–16. https://doi.org/10.1016/j.jag.2004.06.003
- Rekha, B. V., George, A. V., & Rita, M. (2011). Morphometric analysis and micro-watershed prioritization of Peruvanthanam sub-watershed, the Manimala River Basin, Kerala, South India. Environmental Research, Engineering and Management, 57(3), 6–14. https://erem.ktu.lt/index.php/erem/article/view/472
- Resmi, M. R., Babeesh, C., & Achyuthan, H. (2019). Quantitative analysis of the drainage and morphometric characteristics of the Palar River basin, Southern Peninsular India; using bAd calculator (bearing azimuth and drainage) and GIS. Geology, Ecology, and Landscapes, 3(4) , 295–307. https://doi.org/10.1080/24749508.2018.1563750
- Ritter, D., Kochel, R., & Miller, J. (2002). Process geomorphology. Mc Graw-Hill.
- Rizzoli, P., Martone, M., Gonzalez, C., Wecklich, C., Tridon, D. B., Bräutigam, B., Bachmann, M., Schulze, D., Fritz, T., Huber, M., Wessel, B., Krieger, G., Zink, M., & Moreira, A. (2017). Generation and performance assessment of the global TanDEM-X digital elevation model. ISPRS Journal of Photogrammetry and Remote Sensing, 132, 119–139. https://doi.org/10.1016/j.isprsjprs.2017.08.008
- Robinson, N., Regetz, J., & Guralnick, R. P. (2014). EarthEnv-DEM90: A nearly-global, void-free, multi-scale smoothed, 90m digital elevation model from fused ASTER and SRTM data. ISPRS Journal of Photogrammetry and Remote Sensing, 87, 57–67. https://doi.org/10.1016/j.isprsjprs.2013.11.002
- Sahu, N., Obi Reddy, G. P., Kumar, N., Nagaraju, M. S. S., Srivastava, R., & Singh, S. K. (2017). Morphometric analysis in basaltic Terrain of Central India using GIS techniques: A case study. Applied Water Science, 7(5), 2493–2499. https://doi.org/10.1007/s13201-016-0442-z
- Samal, D. R., Gedam, S. S., & Nagarajan, R. G. I. S. (2015). GIS based drainage morphometry and its influence on hydrology in parts of Western Ghats region, Maharashtra, India. Geocarto International, 30(7), 755–778. https://doi.org/10.1080/10106049.2014.978903
- Schmid, B. H. (1997). Critical rainfall duration for overland flow from an infiltrating plane surface. Journal of Hydrology, 193(1–4), 45–60. https://doi.org/10.1016/S0022-1694(96)03152-6
- Schumm, S. A. (1956). Evolution of drainage systems and slopes in badlands at Perth Amboy, New Jersey. Geological Society of America Bulletin, 67(5), 597–646.
- Shaikh, M. P., Yadav, S. M., & Manekar, V. L. (2022). Assessment of the empirical methods for the development of the synthetic unit hydrograph: A case study of a semi-arid river basin. Water Practice & Technology, 17(1), 139–156. https://doi.org/10.2166/wpt.2021.117
- Sharma, S., & Mahajan, A. K. (2020). GIS-based sub-watershed prioritization through morphometric analysis in the outer Himalayan region of India. Applied Water Science, 10(7), 1–11. https://doi.org/10.1007/s13201-020-01243-x
- Shrestha, A. B., Bajracharya, S. R., Sharma, A. R., Duo, C., & Kulkarni, A. (2017). Observed trends and changes in daily temperature and precipitation extremes over the Koshi river basin 1975–2010. International Journal of Climatology, 37(2), 1066–1083. https://doi.org/10.1002/joc.4761
- Shreve, R. L. (1966). Statistical law of stream numbers. The Journal of Geology, 74(1), 17–37. https://doi.org/10.1086/627137
- Singh, D. S., & Awasthi, A. (2011). Implication of drainage basin parameters of Chhoti Gandak River, Ganga Plain, India. Journal of the Geological Society of India, 78(4), 370–378. https://doi.org/10.1007/s12594-011-0102-8
- Singh, S., & Kanhaiya, S. (2015). Morphometry of the Barakar River Basin, India: A remote sensing and GIS approach. International Journal of Current Research, 7(7), 17948–17955.
- Singh V and Singh S Kumar. (2018). Hypsometric Analysis Using Microwave Satellite Data and GIS of Naina–Gorma River Basin (Rewa district, Madhya Pradesh, India). Water Conserv Sci Eng, 3(4), 221–234. https://doi.org/10.1007/s41101-018-0053-7
- Singh, S., Kanhaiya, S., Singh, A., & Chaubey, K. (2019). Drainage network characteristics of the Ghaghara river basin (GRB), Son valley, India. Geology, Ecology, and Landscapes, 3(3), 159–167. https://doi.org/10.1080/24749508.2018.1525670
- Singh, A. P., Arya, A. K., & Singh, D. S. (2020). Morphometric analysis of Ghaghara River Basin, India, using SRTM data and GIS. Journal of the Geological Society of India, 95(2), 169–178. https://doi.org/10.1007/s12594-020-1406-3
- Singh, S., Singh, A. K., Kumar, P., & Jaiswal, M. K. (2021). Morphotectonic analysis of the Bihar River, Madhya Pradesh, India. Proceedings of the Indian National Science Academy, 87(1), 163–174. https://doi.org/10.1007/s43538-021-00023-8
- Singh, U., & Sharma, P. K. (2022). Drought forecasting using the stochastic model in the Betwa river basin, India. Modeling Earth Systems and Environment, 8(2), 1771–1786. https://doi.org/10.1007/s40808-021-01187-4
- Singh V Gaurav, Singh S Kumar, Kumar N and Singh R Prakash. (2022). Simulation of land use/land cover change at a basin scale using satellite data and markov chain model. Geocarto International , 1–26. https://doi.org/10.1080/10106049.2022.2052976
- Singhai, A., Das, S., Kadam, A. K., Shukla, J. P., Bundela, D. S., & Kalashetty, M. (2019). GIS-based multi-criteria approach for identification of rainwater harvesting zones in upper Betwa sub-basin of Madhya Pradesh, India. Environment, Development and Sustainability, 21(2), 777–797. https://doi.org/10.1007/s10668-017-0060-4
- Smith, M. J., & Clark, C. D. (2005). Methods for the visualization of digital elevation models for landform mapping. Earth Surface Processes and Landforms, 30(7), 885–900. https://doi.org/10.1002/esp.1210
- Soni, S. (2017). Assessment of morphometric characteristics of Chakrar watershed in Madhya Pradesh India using geospatial technique. Applied Water Science, 7(5), 2089–2102. https://doi.org/10.1007/s13201-016-0395-2
- Sørensen, R., & Seibert, J. (2007). Effects of DEM resolution on the calculation of topographical indices: TWI and its components. Journal of Hydrology, 347(1–2), 79–89. https://doi.org/10.1016/j.jhydrol.2007.09.001
- Sreedevi, P. D., Owais, S. H. H. K., Khan, H. H., & Ahmed, S. (2009). Morphometric analysis of a watershed of South India using SRTM data and GIS. Journal of the Geological Society of India, 73(4), 543–552. https://doi.org/10.1007/s12594-009-0038-4
- Sreedevi, P. D., Sreekanth, P. D., Khan, H. H., & Ahmed, S. (2013). Drainage morphometry and its influence on hydrology in a semi arid region: Using SRTM data and GIS. Environmental Earth Sciences, 70(2), 839–848. https://doi.org/10.1007/s12665-012-2172-3
- Stanislawski, L. V., Buttenfield, B. P., & Doumbouya, A. (2015). A rapid approach for automated comparison of independently derived stream networks. Cartography and Geographic Information Science, 42(5), 435–448. https://doi.org/10.1080/15230406.2015.1060869
- Strahler, A. N. (1952a). Dynamic basis of geomorphology. Geological Society of America Bulletin, 63(9), 923–938.
- Strahler, A. N. (1952b). Hypsometric (area-altitude) analysis of erosional topography. Geological Society of America Bulletin, 63(11), 1117–1142.
- Strahler, A. N. (1957). Quantitative analysis of watershed geomorphology. Eos, Transactions American Geophysical Union, 38(6), 913–920. https://doi.org/10.1029/TR038i006p00913
- Strahler, A. (1964) Quantitative Geomorphology of Drainage Basins and Channel Networks. In: Chow, V., Ed., Handbook of Applied Hydrology, McGraw Hill, New York, 439-476.
- Sujatha, E. R., Selvakumar, R., Rajasimman, U. A. B., & Victor, R. G. (2015). Morphometric analysis of sub-watershed in parts of Western Ghats, South India using ASTER DEM. Geomatics, Natural Hazards and Risk, 6(4), 326–341. https://doi.org/10.1080/19475705.2013.845114
- Suryavanshi, S., Pandey, A., Chaube, U. C., & Joshi, N. (2014). Long-term historic changes in climatic variables of Betwa Basin, India. Theoretical and Applied Climatology, 117(3), 403–418. https://doi.org/10.1007/s00704-013-1013-y
- Suryavanshi, S., Pandey, A., & Chaube, U. C. (2017). Hydrological simulation of the Betwa River basin (India) using the SWAT model. Hydrological Sciences Journal, 62(6), 960–978. https://doi.org/10.1080/02626667.2016.1271420
- Tachikawa, T., Hato, M., Kaku, M., & Iwasaki, A. (2011) Characteristics of ASTER GDEM version 2. In: 2011 IEEE international geoscience and remote sensing symposium. IEEE, pp 3657–3660
- Tadono, T., Ishida, H., Oda, F., Naito, S., Minakawa, K., & Iwamoto, H. (2014). Precise global DEM generation by ALOS PRISM. ISPRS Annals of the Photogrammetry, Remote Sensing and Spatial Information Sciences, 2(4), 71. https://doi.org/10.5194/isprsannals-II-4-71–2014
- Takaku, J., Tadono, T., & Tsutsui, K. (2014). GENERATION OF HIGH RESOLUTION GLOBAL DSM FROM ALOS PRISM. ISPRS Annals of Photogrammetry, Remote Sensing & Spatial Information Sciences, 2(4), 243–248. https://doi.org/10.5194/isprsarchives-XL-4-243-2014
- Tesema, T. A. (2021). Impact of identical digital elevation model resolution and sources on morphometric parameters of Tena watershed, Ethiopia. Heliyon, 7(11), e08345. https://doi.org/10.1016/j.heliyon.2021.e08345
- Thomas, J., Joseph, S., & Thrivikramaji, K. P. (2010). Morphometric aspects of a small tropical mountain river system, the southern Western Ghats, India. International Journal of Digital Earth, 3(2), 135–156. https://doi.org/10.1080/17538940903464370
- Vaka, D. S., Kumar, V., Rao, Y. S., & Deo, R. (2019, July). Comparison of various DEMs for height accuracy assessment over different terrains of India. In IGARSS 2019-2019 IEEE international geoscience and remote sensing symposium (pp. 1998–2001). IEEE.
- Veeranna, J., Gouthami, K., Yadav, P. B., & Mallikarjuna, V. R. (2017). Calculating linear and areal and relief aspect parameters using Geo-Spatial Techniques (ArcGIS 10.2 and SWAT model) for Akkeru River Basin Warangal. International Journal of Current Microbiology and Applied Sciences, 6(10), 1803–1809. https://doi.org/10.20546/ijcmas.2017.610.217
- Venkatesh, M., & Anshumali. (2019). A GIS-based assessment of recent changes in drainage and morphometry of Betwa River basin and sub-basins, Central India. Applied Water Science, 9(7), 1–12. https://doi.org/10.1007/s13201-019-1033-6
- Waikar, M. L., & Nilawar, A. P. (2014). Morphometric analysis of a drainage basin using geographical information system: A case study. Int J Multidiscip Curr Res, 2(2), 179–184.
- Wakode, H. B., Dutta, D., Desai, V. R., Baier, K., & Azzam, R. (2013). Morphometric analysis of the upper catchment of Kosi River using GIS techniques. Arabian Journal of Geosciences, 6(2), 395–408. https://doi.org/10.1007/s12517-011-0374-8
- Yadav S Kumar, Singh S Kumar, Gupta M and Srivastava P K. (2014). Morphometric analysis of Upper Tons basin from Northern Foreland of Peninsular India using CARTOSAT satellite and GIS. Geocarto International,29(8), 895–914. https://doi.org/10.1080/10106049.2013.868043
- Yadav S Kumar, Dubey A, Szilard S and Singh S Kumar. (2018). Prioritisation of sub-watersheds based on earth observation data of agricultural dominated northern river basin of India. Geocarto International, 33(4), 339–356. https://doi.org/10.1080/10106049.2016.1265592
- Yadav S Kumar, Dubey A, Singh S Kumar and Yadav D. (2020). Spatial regionalisation of morphometric characteristics of mini watershed of Northern Foreland of Peninsular India. Arab J Geosci, 13(12). https://doi.org/10.1007/s12517-020-05365-z
- Yamazaki, D., Ikeshima, D., Tawatari, R., Yamaguchi, T., O’Loughlin, F., Neal, J. C., Sampson, C. C., Kanae, S., & Bates, P. D. (2017). A High-Accuracy Map of Global terrain elevations. Geophysical Research Letters, 44(11), 5844–5853. https://doi.org/10.1002/2017GL072874
- Zink, M., Moreira, A., Hajnsek, I., Rizzoli, P., Bachmann, M., Kahle, F., Huber, T., Krieger, M., Lachaise, M, G., Wessel, B., Wessel, B., Maurer, E., & Wessel, B. (2021). TanDEM-X: 10 years of formation flying bistatic SAR interferometry. IEEE Journal of Selected Topics in Applied Earth Observations and Remote Sensing, 14, 3546–3565. https://doi.org/10.1109/jstars.2021.3062286
Appendix