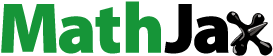
ABSTRACT
The catastrophic and enormous increase in atmospheric greenhouse gas levels leads to global climate change, and an immediate need to investigate the effects of increased CO2 levels on natural vegetation is complex. The goal of this study was to see how nitrogen addition affected Eucalyptus globulus Labill. species development in both ambient (408 ppm) and different elevated (450 ppm, 500 ppm, 550 ppm, and 600 ppm) carbon dioxide environments with OSC S-1000 L plant growth chamber. In this investigation, nitrogen was added in the form of potassium nitrate in various amounts (0, 25, 50, 100, and 200 mg/kg of soil). The plant’s growth responses (leaf length, leaf width, and shoot length) were investigated. To calculate the carbon content, a multiple regression equation was employed to fit the model. The maximum carbon content value 52.37 ± 0.03% was recorded at 200 mg/kg nitrogen addition level for an elevated CO2 condition of 600 ppm. The results of this study conclude that the nitrogen addition had a positive impact on the plant growth and carbon sequestration potential of Eucalyptus globulus under ambient as well as elevated CO2 conditions.
Introduction
Global climate change is associated with increased atmospheric carbon dioxide (CO2) concentrations generated by fossil fuel burning and increased nitrogen (N) deposition induced by human activities (Martinez et al., Citation2017). Specific plant communities and their associations are likely to be affected by these factors (Churkina, Citation2016). Most vegetation has the well-established effects of increasing the level of CO2 on photosynthesis, biomass production, and plant water-use efficiency, and these effects vary depending on vegetation and nutrient levels (Bhargava & Mitra, Citation2021). The increasing N restriction produced by higher CO2 growth stimulation is thought to limit the sustainability of ecological responses to CO2 (Z. Xu et al., Citation2016). As a result, scientists from all around the world have been studying the relationship between CO2 and soil N availability, with varying results reported in several studies (Farhate et al., Citation2018).
N emissions and deposition rates are expected to quadruple by 2050 due to anthropogenic activities dominating global N cycles, resulting in additional regions receiving potentially dangerous levels of N inputs. The quantity of N deposited across the planet varies dramatically between locations (Verma & Sagar, Citation2020). Enhancement of biologically reactive N by human activity alters the nutrient cycles in soils and vegetation structure and limits biological diversity (Kenkel et al., Citation2016). In many terrestrial habitats, N is the most important nutrient for plant development. As a result, N fertilizer is frequently employed in terrestrial N-limited habitats to boost plant growth and production by increasing soil N availability (Ganesh & Pragasan, Citation2019). The pace and duration of N deposition can affect cellular and soil N status, and deposition rates can surpass plant N absorption capability (Carter et al., Citation2017). N deposition has been shown to have varied impacts on many plant species (Boutin et al., Citation2017), depending on whether N saturation occurs at a given deposition rate (Lu et al., Citation2016). However, the physiological adaptations of the species present influence the responses of forest trees and shrubs to N deposition (Penuelas et al., Citation2020).
Nature has its own “reservoirs” or “sinks” for sequestering and storing carbon (C). Over 50% of the CO2 discharge produced by humans has been stored in natural terrestrial and ocean ecosystems. Forests plays a critical role in the C cycle and C storage, and they store more than half of all terrestrial C and have a high potential to absorb atmospheric CO2 (Abeysekara et al., Citation2018). Trees are an important component of forest ecosystems because they function as a significant CO2 sink, collecting CO2 from the atmosphere and eliminating it through photosynthesis in fixed woody biomass (Martinez et al., Citation2017).
Agroforestry practices have a long history in India. C capture occurs both belowground and aboveground in many forest ecosystems, as in forms of soil C and root growth augmentation, as well as C deposited in standing biomass. In India, the forestry system consists of farm trees, social forestry, and a variety of indigenous forest conservation and ethno forestry approaches. The practice of planting trees on farms dates back centuries and has mostly stayed constant. These trees are multifunctional, serving as shade, fodder, fuelwood, fruit, vegetables, and medicinal purposes (Basu, Citation2014).
Agroforestry has been estimated to store 9, 21, 50, and 63 Mg C per hectare (ha) of C in semiarid, sub-humid, humid, and temperate areas, respectively. Early research of effective sequestering carbon in forest ecosystems and other land-use systems in India indicated a capacity of 68 to 228 Mg C per hectare (Murthy et al., Citation2013), equivalent to 25 tonnes C per ha over 96 million ha of land (Rao et al., Citation2016). However, depending on biomass production, this number varies by area (Sun & Liu, Citation2020). Eucalyptus provides a variety of medical benefits, including antibacterial characteristics, effectiveness as an insect repellent, acting as a painkiller, triggering an immunological response, treating skin wounds and fungi, and acting as an expectorant to release mucus and alleviate congestion (Khan et al., Citation2020).
With the primary objective of reducing atmospheric C concentration by trapping it in plant biomass to mitigate greenhouse effect, which causes global warming and climate change. Although one cannot accurately foresee the climate of the future, it is anticipated that the amount of CO2 would rise; thus, how would the current species store C under this circumstance? or, to put it another way, how would the species lower the level of C in the atmosphere? Whether they would sequester more, less, or not at all in proportion to rising levels of atmospheric C? An extensive literature search found that only a few research studies on the impact of N on C storage have been conducted across the world. Hence, to fill the knowledge gap, the current work was conducted to determine the effects of N addition (Nadd) on the C storage capacity of Eucalyptus globulus under ambient and different elevated CO2 environment conditions.
Materials and methods
Study species
The current study was focused on the tree species Eucalyptus globulus (Myrtaceae). A pot experiment was conducted at ambient (408 ppm) and different elevated CO2 (450 ppm, 500 ppm, 550 ppm, and 600 ppm) conditions using a plant growth chamber (OSC S-1000 L) at Environmental Ecology Laboratory, Environmental Sciences Department, Bharathiar University.
Experimental design and treatments
This work was conducted in an OSC S-1000 L plant growth chamber with various levels of CO2 conditions. The plants were kept alive by a 16-/8-hour light/dark cycle. The chambers were kept at a temperature of 25 ± 3.1°C, which was close to room temperature. The seeds of the Eucalyptus globulus species were procured from Tamil Nadu Agricultural University (TNAU) in Coimbatore and were germinated on seedling trays. After 3 weeks of germination, 25 productive plants were selected and each of them was transplanted separately in a pot with 1 kg of soil under ambient CO2 (408 ppm) and different elevated CO2 (450 ppm, 500 ppm, 550 ppm and 600 ppm) environment conditions for 98 days. The characteristics of soil were analysed before and after the experiment. For each experimental condition, N was supplied in the form of potassium nitrate at rates of 0, 25, 50, 100, and 200 mg/kg soil, with five replicates for each experimental setup (Pragasan & Ganesh, Citation2021). The pots were sprinkled with water at regular intervals to keep the soil moisture at 30–35%.
Plant growth analysis
Growth parameters such as shoot length (SL), leaf length (LL), and leaf width (LW) were recorded once in two weeks. The plants were then collected and utilized for further investigation. SL, LL, and LW were measured using a measuring scale, and C content was calculated using the loss on ignition method.
Model fit equation
A model fit equation was developed using linear regression analysis to predict the C content of the Eucalyptus globulus species under various Nadd levels and different levels of CO2 environmental conditions. For the model fit, a multiple regression equation was employed (Abdullah et al., Citation2012),
where Y is the dependent variable C content, X1 is the independent variable as Nadd levels, X2 is the independent variable as different levels of CO2 conditions, and β0, β1, and β2 are the coefficients.
Statistical analysis
Analysis of Variance (ANOVA, F-test) was made to check statistically significant changes between treatments by using SPSS (ver. 21). The F-test was used to reveal significant differences in the C content of the Eucalyptus globulus species (p < 0.05) among the various Nadd levels and CO2 conditions. In addition, an equation for determining the C content was developed by using linear regression analysis.
Results
Plant growth analysis
depict the growth of Eucalyptus globulus in ambient (408 ppm) and elevated (450, 500, 550, and 600 ppm) CO2 environments with various levels of Nadd. N promotes the growth of SL, LL, and LW of the transplanting seedlings for all the environmental setups throughout the study. The SL, LL, and LW were recorded maximum for Nadd at 200 mg/kg for the transplanted Eucalyptus globulus seedlings to the pots. At 408 ppm, the values of SL, LL, and LW were 24.74 ± 0.50 cm, 4.42 ± 0.42 cm, and 2.46 ± 0.11 cm, respectively (). Likewise, for elevated CO2 conditions 450 ppm, 500 ppm, 550 ppm, and 600 ppm, the values were 23.92 ± 0.61 cm, 3.68 ± 0.22 cm, and 2.36 ± 0.31 cm (); 22.94 ± 0.64 cm, 4.26 ± 0.34 cm, and 3.54 ± 0.15 cm (),;24.3 ± 1.22 cm, 4.32 ± 0.28 cm, and 3.02 ± 0.31 cm (), and 24.42 ± 1.18 cm, 4.28 ± 0.27 cm, and 2.7 ± 0.27 cm ().
Figure 1. Effect of various levels of Nadd on the growth of Eucalyptus globulus Labill under ambient CO2 conditions (408 ppm).

Figure 2. Effect of various levels of Nadd on the growth of Eucalyptus globulus Labill under 450 ppm eCO2 conditions.

Figure 3. Effect of various levels of Nadd on the growth of Eucalyptus globulus Labill under 500 ppm eCO2 conditions.

Carbon content
depicts the C content of Eucalyptus globulus under various degrees of Nadd and CO2 conditions. shows the results of the F-test ANOVA values for C content. The F-test findings show that varying amounts of Nadd, CO2 environmental conditions and the combination of Nadd and CO2 conditions all has a significant impact on the C content of Eucalyptus globulus. We reject the null hypothesis because the alpha value (0.05) is larger than the p-value (0.000; ). Likewise, a significant difference exists across the impacts of different CO2 environmental setups on C content, as revealed by the ANOVA value. Furthermore, the C content differs significantly between Nadd and various CO2 situations.
Figure 6. Effect of various levels of nitrogen addition on the carbon content of Eucalyptus globulus Labill at different CO2 environmental conditions.

Table 1. F-test ANOVA values for the carbon content of Eucalyptus globulus under various levels of Nadd and different CO2 environmental conditions.
Model fit equation
To assess the C content of the Eucalyptus globulus species, a multiple regression equation was applied for model fit. Linear regression analysis was used to determine the coefficient values () for the model fit equation and model summary () to estimate the C content with various levels of Nadd and CO2 environmental setups. Multiple regression R2 values for Eucalyptus globulus are shown in the scatterplot diagram ().
Table 2. Values of model coefficients for the dependent variable (carbon content).
Table 3. Model summary for the dependent variable (carbon content).
The coefficient values of β0 (47.547), β1 (0.187), and β2 (0.712) were implemented to the model fit to find out C content in the Eucalyptus globulus species by using (1),
where Y is the C content, X1 is the Nadd level, and X2 is the CO2 level.
Discussion
Climate change has emerged as the most significant environmental issue in recent years, owing to growing CO2 levels in the atmosphere. These problems are linked to several issues, which comprise a variation in primary production and natural ecosystem carbon dynamics. The current work is focused on studying the impacts of Nadd on the growth and C sequestration potential of Eucalyptus globulus under ambient and elevated CO2 environmental conditions. The outcome of this experimental work reveals that the impact of Nadd had a positive impact on the growth and CO2 potential of Eucalyptus globulus species at ambient and elevated CO2 environment conditions. Under ambient CO2 conditions, the C content has recorded a maximum of 49.6 ± 0.07 at the 50 mg/kg Nadd level. For the elevated CO2 condition 450 ppm, the maximum C content 50.02 ± 0.05% was recorded at the 50 mg/kg Nadd level. While, for the elevated CO2 condition at 500 ppm, the maximum carbon content 50.07 ± 0.05% was recorded at 0 mg/kg Nadd level, for the elevated CO2 conditions at 550 ppm and 600 ppm, the maximum C content values (51.87 ± 0.03% and 52.37 ± 0.03%, respectively) were recorded at the 200 mg/kg Nadd level. Similarly, plant growth parameters such as SL, LL, and LW showed positive growth along with an increase in Nadd and CO2 concentrations.
Also, our earlier studies on Azadirachta indica (Ganesh & Pragasan, Citation2019) and Syzygium cumini (Pragasan & Ganesh, Citation2021) have revealed that the C sequestration potential of plants species had positive effects with Nadd and increasing CO2 environment conditions. Similar results were seen on enhanced photosynthetic capacity, and biomass production included how plants grown in elevated CO2 environments would react to CO2 enrichment (Oliveira & Marenco, Citation2019). Plant growth and terrestrial ecosystem development can have positive effects from increased N availability (W. Li et al., Citation2015; H. Zhang et al., Citation2018). The plant’s response to CO2 is influenced by several factors that include air temperature, N, phosphorous (P), various nutrients, growing period, symbiosis with mycorrhiza and availability of water (Baslam et al., Citation2012; Bicharanloo et al., Citation2021). CO2 impacts on growth are only seen in completely watered plants; those that are just moderately watered have no CO2 effects (Irigoyen et al., Citation2014). Plants grow rapidly when subjected to a result of high CO2 concentrations and high-temperature circumstances (Barickman et al., Citation2021).
In this study, Eucalyptus globulus species recorded a maximum C content of 52.37 ± 0.03% at the 200 mg/kg Nadd level for the elevated CO2 condition 600 ppm. Similar results were reported for Azadirachta indica (54.82 ± 0.53%, Ganesh & Pragasan, Citation2019) and for Syzygium cumini (53.3 ± 0.09%, Pragasan & Ganesh, Citation2021). The application of N has complex impacts on seedling growth and survival (Razaq et al., Citation2017). N enhancement has a significant impact on the shoot morphology and nutritional status of nursery seedlings in prior research (F. Xu et al., Citation2020). Moreover, N supplementation increases the seedling’s height as well as the diameter of the root collar (Hu et al., Citation2019). Similarly, the results of this study found that supplementing with N enhances Eucalyptus globulus growth (SL, LL, and LW). This demonstrates that N fertilizers are required for maximum plant development. Brienen et al. (Citation2020) have revealed that the responses of plants to increased CO2 levels in the atmosphere have enhanced net photosynthesis, development, and biomass production of plants, and increased CO2 stimulates faster growth rates in young seedlings than in older trees, while Eastman et al. (Citation2021) stated that the addition of N encourages the storage of additional C from the environment in respective plant species. Furthermore, the most prevalent inorganic cation is potassium (K), which is crucial for promoting healthy plant development. It contributes significantly to the development of yield and quality enhancement. In addition to being crucial for plant development and function, K is also crucial for cell proliferation (F. Xu et al., Citation2020).
Multiple regression analysis was used in this study to develop a model fit equation to assess the C content. The R2 value is 0.881 ( and ), and this indicates that the equation has an accuracy of 88.1% in predicting the C content of Eucalyptus globulus species under various N addition levels (0–200 mg/kg) and different CO2 environmental conditions (408–600 ppm). This equation is suitable for predicting the C content of Eucalyptus globulus species within the prescribed ranges of Nadd and CO2 levels.
The ability of elevated CO2 to enhance plant development is highly dependent on the growth potential of the plant’s species (Silva et al., Citation2020). Every species has responded to elevated CO2 environments in a distinctive manner (Gagne et al., Citation2020), including changes in aboveground growth (Gupta et al., Citation2020). represents how N addition and CO2 levels affect the growth of plant species reported earlier. A positive relationship between increased CO2 and N implies that soil N limitations may gradually reduce beneficial photosynthetic carbon uptake and biomass reactions with elevated CO2 conditions (Wang, Citation2007). Species that can enhance their nutritional supply in the presence of an increased CO2 environment either via root absorption and mycorrhizal symbiosis or through increased N fixation will react very strongly to rising environmental conditions (Diagne et al., Citation2020).
Table 4. Growth response of plant species to Nadd/CO2 levels reported so far.
Generally, plants acclimatize and adjust to their surroundings to maximize their survival and growth. Some plant species that grow well in high CO2 environments when cultivated alone have been found to show less growth in different plant communities (Velasquez et al., Citation2018). This impact is most probably because the direct benefits of increased CO2 are offset by the negative consequences of promoting competition growth. As CO2 levels rise in the atmosphere, plant communities may alter, as certain species benefit more than others from the increasing atmospheric CO2 concentrations. When the CO2 level in the atmosphere rises, the first effect is on photosynthetic enzymes. This main impact is the source of all subsequent modifications. Plants adapt their physiological functions as well as their biochemical processes in response to environmental factors. Variations in biochemical composition, carbonic anhydrase, and nitrate reductase activity have been reported in CO2 enriched studies, and the responses are species-specific (Aswathi et al., Citation2018).
Conclusion
We conclude from this experimental study that the effect of Nadd had a positive impact on the plant growth (SL, LL, and LW), and C sequestration potential of Eucalyptus globulus species under ambient and different elevated CO2 environmental conditions. The current study was performed under constrained environments, in terms of Nadd and different CO2 setups. Furthermore, a model fit equation was developed to assess the C content of Eucalyptus globulus species grown at various Nadd levels (0–200 mg/kg soil) and CO2 concentrations (408–600 ppm). Thus, the current study provides valuable information on the growth and C sequestration potential of Eucalyptus globulus under Nadd and different CO2 conditions. We recommend plantation of Eucalyptus globulus, not only for its medicinal purposes but also for its C sequestration potential in mitigation of global warming and climate change.
Author contributions
Dr L. Arul Pragasan, Assistant Professor, the corresponding author contributed by conceptualization, funding acquisition, supervision, and final approval of the paper. Mr K.P. Ganesh is a research scholar who contributed by carrying out the work, data collection, laboratory analysis, and drafted the paper.
Acknowledgments
The authors sincerely thank DST-SERB, Government of India for the financial support for this research work.
Disclosure statement
The authors declare that they have no known competing financial interests or personal relationships that could have appeared to influence the work reported in this paper.
Additional information
Funding
References
- Abdullah, N., Zainodin, H. J., & Ahmed, A. (2012). Comparisons between Huber’s and Newton’s multiple regression models for stem biomass estimation. Malaysian Journal of Mathematical Sciences, 6(1), 1–28. https://einspem.upm.edu.my/journal/fullpaper/vol6no1/1.%20Noraini%20edit%204.1.12.pdf
- Abeysekara, A. M. S. K., Yatigammana, S. K., & Premakantha, K. T. (2018). Biomass and carbon stock estimation of Udawattakele forest reserve in Kandy district of Sri Lanka. Journal of Tropical Forestry and Environment, 8(2), 12–28. http://dx.doi.org/10.31357/jtfe.v8i2.3760Awasthi
- Awasthi, J. P., Paraste, K. S., Rathore, M., Varun, M., Jaggi, D., & Kumar, B. (2018). Effect of elevated CO2 on Vigna radiata and two weed species: Yield, physiology and crop–weed interaction. Crop Pasture Science, 69(6), 617–631.
- Bai, Y., Yan, R., Schellenberg, M. P., Wang, H., Han, G., Zhang, R., Wei, Z., & Wei, Z. (2020). Nitrogen increased aboveground biomass of Leymus chinensis grown in semi-arid grasslands of inner Mongolia, China. Agronomy Journal, 112(1), 511–522. https://doi.org/10.1002/agj2.20080
- Barickman, T. C., Olorunwa, O. J., Sehgal, A., Walne, C. H., Reddy, K. R., & Gao, W. (2021). Interactive impacts of temperature and elevated CO2 on Basil (Ocimum Basilicum L.) root and shoot morphology and growth. Horticulturae, 7(5), 112. https://doi.org/10.3390/horticulturae7050112
- Baslam, M., Erice, G., & Goicoechea, N. (2012). Impact of arbuscular mycorrhizal fungi (AMF) and atmospheric CO2 concentration on the biomass production and partitioning in the forage legume alfalfa. Symbiosis, 58(1), 171–181. https://doi.org/10.1007/s13199-012-0199-6
- Basu, J. P. (2014). Agroforestry, climate change mitigation and livelihood security in India. New Zealand Journal of Forestry Science, 44(Suppl 1), S11. https://doi.org/10.1186/1179-5395-44-S1-S11
- Bhargava, S., & Mitra, S. (2021). Elevated atmospheric CO2 and the future of crop plants. Plant Breeding, 140(1), 1–11. http://dx.doi.org/10.1111/pbr.12871
- Bicharanloo, B., Cavagnaro, T. R., Keitel, C., & Dijkstra, F. A. (2021). Nitrogen fertilisation increases specific root respiration in ectomycorrhizal but not in arbuscular mycorrhizal plants: A meta-analysis. Frontiers in Plant Science, 12, 1–10. https://doi.org/10.3389/fpls.2021.711720
- Boutin, M., Corcket, E., Alard, D., Villar, L., Jimenez, J. J., Blaix, C., Pornon, A., Corriol, G., Lamaze, T., & Pornon, A. (2017). Nitrogen deposition and climate change have increased vascular plant species richness and altered the composition of grazed subalpine grasslands. Journal of Ecology, 105(5), 1199–1209. https://doi.org/10.1111/1365-2745.12743
- Brienen, R. J., Caldwell, L., Duchesne, L., Voelker, S., Barichivich, J., Baliva, M., Gloor, E., Di Filippo, A., Helama, S., Locosselli, G. M., Lopez, L., Piovesan, G., Schöngart, J., Villalba, R., & Gloor, E. (2020). Forest carbon sink neutralized by pervasive growth-lifespan trade-offs. Nature Communications, 11(1), 1–10. https://doi.org/10.1038/s41467-020-17966-z
- Carter, T. S., Clark, C. M., Fenn, M. E., Jovan, S., Perakis, S. S., Riddell, J., Hastings, M. G., Greaver, T. L., & Hastings, M. G. (2017). Mechanisms of nitrogen deposition effects on temperate forest lichens and trees. Ecosphere, 8(3), 01717. https://doi.org/10.1002/ecs2.1717
- Cha, S., Chae, H. M., Lee, S. H., & Shim, J. K. (2017). Effect of elevated atmospheric CO2 concentration on growth and leaf litter decomposition of Quercus acutissima and Fraxinus rhynchophylla. PloS One, 12(2), 0171197. https://doi.org/10.1371/journal.pone.0171197
- Churkina, G. (2016). The role of urbanization in the global carbon cycle. Frontiers in Ecology and Evolution, 3, 144. https://doi.org/10.3389/fevo.2015.00144
- Diagne, N., Ngom, M., Djighaly, P. I., Fall, D., Hocher, V., & Svistoonoff, S. (2020). Roles of arbuscular mycorrhizal fungi on plant growth and performance: Importance in biotic and abiotic stressed regulation. Diversity, 12(10), 370. https://doi.org/10.3390/d12100370
- Eastman, B. A., Adams, M. B., Brzostek, E. R., Burnham, M. B., Carrara, J. E., Kelly, C., Peterjohn, W. T., Walter, C. A., & Peterjohn, W. T. (2021). Altered plant carbon partitioning enhanced forest ecosystem carbon storage after 25 years of nitrogen additions. New Phytologist, 230(4), 1435–1448. https://doi.org/10.1111/nph.17256
- Farhate, C. V. V., Souza, Z. M. D., Oliveira, S. R. D. M., Tavares, R. L. M., & Carvalho, J. L. N. (2018). Use of data mining techniques to classify soil CO2 emission induced by crop management in sugarcane field. PLoS One, 13(3), 0193537. https://doi.org/10.1371/journal.pone.0193537
- Gagne, M. A., Smith, D. D., & McCulloh, K. A. (2020). Limited physiological acclimation to recurrent heatwaves in two boreal tree species. Tree Physiology, 40(12), 1680–1696. https://doi.org/10.1093/treephys/tpaa102
- Ganesh, K. P., & Pragasan, L. A. (2019). Effect of nitrogen addition on growth and carbon sequestration potential of Azadirachta indica under different climatic conditions. Journal of Emerging Technologies and Innovative Research, 6(5), 2743–2749. 10.1729/Journal.27758
- Gupta, A., Medina, R. A., & Delgado, C. A. I. (2020). The physiology of plant responses to drought. Science, 368(6488), 266–269. https://doi.org/10.1126/science.aaz7614
- Hu, Y. L., Fan, H. H., Xuan, H. F., Mgelwa, A. S., & Chen, S. P. (2019). Distinct growth and nutrient status responses to fertilization regimes in two generations of Chinese Fir Seedlings. Forests, 10(9), 719. https://doi.org/10.3390/f10090719
- Hu, M., & Wan, S. (2019). Effects of fire and nitrogen addition on photosynthesis and growth of three dominant understory plant species in a temperate forest. Journal of Plant Ecology, 12(4), 759–768. https://doi.org/10.1093/jpe/rtz014
- Irigoyen, J. J., Goicoechea, N., Antolin, M. C., Pascual, I., Diaz, S. M., Aguirreolea, J., & Morales, F. (2014). Growth, photosynthetic acclimation and yield quality in legumes under climate change simulations: An updated survey. Plant Science, 226, 22–29. https://doi.org/10.1016/j.plantsci.2014.05.008
- Jeong, H. M., Kim, H. R., Hong, S., & You, Y. H. (2018). Effects of elevated CO2 concentration and increased temperature on leaf quality responses of rare and endangered plants. Journal of Ecology and Environment, 42(1), 1–11. https://doi.org/10.1186/s41610-017-0061-0
- Kenkel, J. A., Sisk, T. D., Hultine, K. R., Sesnie, S. E., Bowker, M. A., & Johnson, N. C. (2016). Indicators of vehicular emission inputs into semi-arid roadside ecosystems. Journal of Arid Environment, 134, 150–159. https://doi.org/10.1016/j.jaridenv.2016.06.007
- Khan, N., Fahad, S., Faisal, S., Akbar, A., & Naushad, M. (2020). Socio-economic and medicinal review of eucalyptus tree in the world. http://dx.doi.org/10.2139/ssrn.3644215
- Li, X., Dong, J., Gruda, N. S., Chu, W., & Duan, Z. (2020). Interactive effects of the CO2 enrichment and nitrogen supply on the biomass accumulation, gas exchange properties, and mineral elements concentrations in cucumber plants at different growth stages. Agronomy Journal, 10(1), 139. https://www.mdpi.com/2073-4395/10/1/139
- Li, W., Jin, C., Guan, D., Wang, Q., Wang, A., Yuan, F., & Wu, J. (2015). The effects of simulated nitrogen deposition on plant root traits: A meta-analysis. Soil Biology and Biochemistry, 82, 112–118. https://doi.org/10.1016/j.soilbio.2015.01.001
- Lu, X., Jiang, H., Liu, J., Zhang, X., Jin, J., Zhu, Q., & Peng, C. (2016). Simulated effects of nitrogen saturation on the global carbon budget using the IBIS model. Scientific Reports, 6(1), 1–10. https://doi.org/10.1038/srep39173
- Martinez, F. M., Vicca, S., Janssens, I. A., Ciais, P., Obersteiner, M., Bartrons, M., & Penuelas, J. (2017). Atmospheric deposition, CO2, and change in the land carbon sink. Scientific Reports, 7(1), 1–13. https://doi.org/10.1038/s41598-017-08755-8
- Murthy, I. K., Gupta, M., Tomar, S., Munsi, M., Tiwari, R., Hegde, G. T., & Ravindranath, N. H. (2013). Carbon sequestration potential of agroforestry systems in India. Journal of Earth Science and Climatic Change, 4(1), 1–7. https://doi.org/10.4172/2157-7617.1000131
- Oliveira, M. F., & Marenco, R. A. (2019). Photosynthesis and biomass accumulation in Carapa surinamensis (Meliaceae) in response to water stress at ambient and elevated CO2. Número, 57(1), 137–146. https://doi.org/10.32615/ps.2019.023
- Penuelas, J., Martínez, F. M., Vallicrosa, H., Maspons, J., Zuccarini, P., Carnicer, J., & Sardans, J. (2020). Increasing atmospheric CO2 concentrations correlate with declining nutritional status of European forests. Communications Biology, 3(1), 1–11. https://www.nature.com/articles/s42003-020-0839-y
- Pragasan, L. A., & Ganesh, K. P. (2021). Impact of nitrogen addition on growth and carbon sequestration potential of Syzygium cumini under different carbon dioxide environment conditions. Acta Ecologica Sinica, In Press. https://doi.org/10.1016/j.chnaes.2021.05.001
- Rao, C. S., Gopinath, K. A., Prasad, J. V. N. S., & Singh, A. K. (2016). Climate resilient villages for sustainable food security in tropical India: Concept, process, technologies, institutions, and impacts. Advances in Agronomy, 140, 101–214. https://doi.org/10.1016/bs.agron.2016.06.003
- Razaq, M., Zhang, P., & Shen, H. L. (2017). Influence of nitrogen and phosphorous on the growth and root morphology of Acer mono. PloS One, 12(2), 0171321. https://doi.org/10.1371/journal.pone.0171321
- Sabo, A., Haland, A., Skre, O., & Mortensen, L. M. (2001). Influence of nitrogen and winter climate stresses on Calluna vulgaris (L.) Hull. Annals of Botany, 88(5), 823–828. https://doi.org/10.1006/anbo.2001.1516
- Silva, R. G. D., Alves, R. D. C., & Zingaretti, S. M. (2020). Increased CO2 causes changes in physiological and genetic responses in C4 crops: A brief review. Plants, 9(11), 1567. https://doi.org/10.3390/plants9111567
- Sun, W., & Liu, X. (2020). Review on carbon storage estimation of forest ecosystem and applications in China. Forest Ecosystems, 7(1), 1–14. https://doi.org/10.1186/s40663-019-0210-2
- Thomson, G., Mollah, M. R., Partington, D. L., Jones, R., Argall, R., Tregenza, J., & Fitzgerald, G. J. (2013). Effects of elevated carbon dioxide and soil nitrogen on growth of two leafy Brassica vegetables. New Zealand Journal of Crop and Horticultural Science, 41(2), 69–77. https://doi.org/10.1080/01140671.2013.772905
- Velasquez, A. C., Castroverde, C. D. M., & He, S. Y. (2018). Plant–pathogen warfare under changing climate conditions. Current Biology, 28(10), 619–634. https://doi.org/10.1016/j.cub.2018.03.054
- Verma, P., & Sagar, R. (2020). Effect of nitrogen (N) deposition on soil-N processes: A holistic approach. Scientific Reports, 10(1), 1–16. https://doi.org/10.1038/s41598-020-67368-w
- Wang, X. (2007). Effects of species richness and elevated carbon dioxide on biomass accumulation: A synthesis using meta-analysis. Oecologia, 152(4), 595–605. https://doi.org/10.1007/s00442-007-0691-5
- Wang, J., Luo, P., Yang, H., Mou, C., & Mo, L. (2016). Different responses of alpine plants to nitrogen addition: Effects on plant-plant interactions. Scientific Reports, 6(1), 1–11. https://doi.org/10.1038/srep38320
- Wang, M., Shi, S., Lin, F., Hao, Z., Jiang, P., & Dai, G. (2012). Effects of soil water and nitrogen on growth and photosynthetic response of Manchurian ash (Fraxinus mandshurica) seedlings in Northeastern China. PloS One, 7(2), 30754. https://doi.org/10.1371/journal.pone.0030754
- Wang, J., Wang, Z., Zhang, X., Zhang, Y., Ran, C., Zhang, J., & Zhang, B. (2015). Response of Kobresia pygmaea and Stipa purpurea grassland communities in Northern Tibet to nitrogen and phosphate addition. Mountain Research and Development, 35(1), 78–86. http://dx.doi.org/10.1659/MRD-JOURNAL-D-11-00104.1
- Xu, F., Chu, C., & Xu, Z. (2020). Effects of different fertilizer formulas on the growth of loquat rootstocks and stem lignification. Scientific Reports, 10(1), 1–11. https://doi.org/10.1038/s41598-019-57270-5
- Xu, Z., Jiang, Y., Jia, B., & Zhou, G. (2016). Elevated CO2 response of stomata and its dependence on environmental factors. Frontiers in Plant Science, 7, 657. https://doi.org/10.3389/fpls.2016.00657
- Zhang, H., Li, W., Adams, H. D., Wang, A., Wu, J., Jin, C., Yuan, F., & Yuan, F. (2018). Responses of woody plant functional traits to nitrogen addition: A meta-analysis of leaf economics, gas exchange, and hydraulic traits. Frontiers in Plant Science, 9, 683. https://doi.org/10.3389/fpls.2018.00683
- Zhang, F. F., Wang, Y. L., Huang, Z. Z., Zhu, X. C., Zhang, F. J., Chen, F. D., Teng, N. J., & Teng, N.-J. (2012). Effects of CO2 enrichment on growth and development of impatiens hawkeri. The Scientific World Journal, 2012, 1–9. https://doi.org/10.1100/2012/601263
- Zhang, L., Wu, D., Shi, H., Zhang, C., Zhan, X., & Zhou, S. (2011). Effects of elevated CO2 and N addition on growth and N2 fixation of a legume subshrub (Caragana microphylla Lam.) in temperate grassland in China. PLoS One, 6(10), 26842. https://doi.org/10.1371/journal.pone.0026842
- Zhang, L., Yang, Y., Zhan, X., Zhang, C., Zhou, S., & Wu, D. (2010). Responses of a dominant temperate grassland plant (Leymus chinensis) to elevated carbon dioxide and nitrogen addition in China. Journal of Environmental Quality, 39(1), 251–259. https://doi.org/10.2134/jeq2009.0109