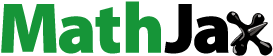
Abstract
Phthalocyanine is a safe and better biocompatible compound, and it has been used in diagnostic and cancer imaging. But the application in the treatment of colon and cervical cancer is elusive. In the present study, we have synthesized Iron (II) phthalocyanine and Zinc phthalocyanine respectively. The bonding, structure, and physical characteristics of the prepared products were analyzed and confirmed by FT-IR, XRD, SEM, EDX methods. We have also examined the anti-cancer properties of the compounds on two different cancer cells (colon cancer HCT-116 and cervical cancer HeLa) by MTT assay. The cell viability assay results showed that a noteworthy reduction in the cell viability post-treatments of Iron (II) phthalocyanine. However, treatment of Zinc phthalocyanine did not show any inhibitory action on both HCT-116 and HeLa cancer cells. We have also calculated the inhibitory concentration (IC50) post treatment of Zinc phthalocyanine and Iron (II) phthalocyanine on HCT-116 and HeLa cells. The IC50 was 30 µg/ml for HCT-116 cells treated with Iron (II) phthalocyanine, whereas it was 33 µg/ml for the HeLa cells. We have also examined the impact of Zinc phthalocyanine and Iron (II) phthalocyanine on non-cancerous cells (HEK-293), and MTT results showed that Iron (II) phthalocyanine and Zinc phthalocyanine induced no significant inhibitory action on HEK-293 cells. In addition, we have also examined the anti-fungal action of Zinc phthalocyanine and Iron (II) phthalocyanine. We have also examined the impact of Iron (II) phthalocyanine on cancer cells using DAPI staining, we have found that was a major reduction in cancer cells population, but there is no reduction in the Iron (II) phthalocyanine -treated cells. We have found that Iron (II) phthalocyanine induced significant antifungal activity against Candida albicans, whereas no significant antifungal activity was observed with Zinc phthalocyanine.
1. Introduction
There is escalating apprehension pertaining to the antimicrobial drug resistance that has caused approximately 700,000 deaths per annum around the world. There are various treatments such as antimicrobial drugs are available, but these as antimicrobial drugs develop resistance and fail to cure the diseases (WHO, Citation2014). Furthermore, multidrug-resistant bacteria also pose serious problem to the patients globally (Liu et al., Citation2016; Xavier et al., Citation2016).
In 1890, Raab has initially explained the concept of photodynamic antimicrobial chemotherapy (PACT). Likewise, the concept of the toxic acridine orange against Paramecia caudatum was also initiated by Raab and other researchers; they further explained the dependency of light introduced due to these cells (Maisch, Citation2007; Ryskova, Buchta, & Slezak, Citation2010). Later, it was also elaborated by the teacher of Raab named van Tappeiner that the toxic effects were not produced in light due to the heating effect. The expression of “Photodynamic reaction” was developed by Tappeiner in 1904 (Von Tappeiner, Citation1904). The understanding of the scientific basis of PACT is still in its initial stages, but it follows a similar principle to that of photodynamic therapy (PDT) of cancer. PACT utilizes non-toxic photosensitizer over the pathogenic cellular structures, subsequently; the inactivation through the light of adequate wavelength is performed. The photo-exited photosensitizer such as singlet oxygen is used to produce the Reactive Oxygen Species (ROS) in the microbial cells (Battersby, Fookes, Matcham, & McDonald, Citation1980). The drug groups are required to be transformed because of the existence of resistant methicillin-resilient in resilient Staphylococcus aureus (MRSA) (Battersby et al., Citation1980).
Type I and Type II mechanisms are included in the photoinactivation mechanism (). The prior encompasses an electron transfer to an oxygen molecule from the photosensitizer in the triplet state, due to which ROS is formulated. In the triplet state, the photosensitizer causes the movement of the energy towards molecular oxygen in the latter that also result in producing cytotoxic singlet oxygen. PACT generally assumes the mechanism of Type II (Battersby et al., Citation1980).
Figure 1. Type I and Type II, the photochemical graphical depiction has been presented for photoinactivation of bacteria which illustrated the transformation between electronically excited state (S1), ground singlet state (S0), the triplet state of intersystem crossing (ISC), fluorescence (F) and nth excited singlet state (Sn) along with also calculating the value of ROS = reactive oxygen species Battersby et al., Citation1980).

Bacteria fall into two categories, Gram (+ve) and Gram (-ve) bacteria. Gram (+ve) strain such as S. aureus has 15–80 nm thick cell wall that is composed of a thick peptidoglycan layer, while Gram (-ve) strain is characterized by a thin peptidoglycan cell wall sandwiched between an inner cytoplasmic cell membrane and a bacterial outer membrane. The significant difference between the two bacterial strains is illustrated in . The Gram (+ve) strain is more susceptible to photoinactivation than the Gram (-ve) strain such as Escherichia coli (E. coli) due to absence of an outer membrane in the former as compared to the latter.
Figure 2. Gram (+ve) and Gram (-ve) structures (Krautler, Citation1987).

Additionally, bacteria can be categorized into two classes i.e. Gram (+ve) and Gram (-ve). The positive Gram bacteria are exemplified S. aureus which has contained a thick wall of 15–80 nm followed by a composition of thick peptidoglycan layer, while a narrow cell wall of peptidoglycan has constituted a Gram (-ve) sprain that is during an inner cytoplasmic structure of cell membrane and an outer membrane of a bacteria. The basic distinction between the two has been illustrated in . Moreover, in the case of Escherichia coli, the Gram (+ve) strain are vulnerable towards the properties of photoinactivation as compared to the Gram (-ve) bacteria strain.
Porphyrins are significant from the biochemical aspect as they have interesting compounds synthetically and are quite medically effective (Smith, Citation1975). Porphyrins are more important in supporting biochemical reactions (Senge, Sergeeva, & Hale, Citation2021). The porphyrins contain chlorophylls, cytochromes, hemes, myoglobins, peroxidases, and catalases. Due to their usage in various crucial processes like facilitating transport, handling photosynthesis and catering to other issues, they are also labeled as the “Lifetime pigments” (Smith, Citation1975). Porphyrins are conjugate acids of ligands that bind metals to form complexes (Senge et al., Citation2021). In , the porphyrin macrocycle entails the 4 rings of pyrrole which are integrated with 4 interpyrrolic methane structures linked by 18 π-electron macrocycles.
In porphyrin structure, two different kinds of substituents i.e. meso-substituted porphyrins and the β have been found, in which the β has a close similarity to porphyrins as also depicted in . Moreover, due to the existence of easy synthesis properties, Meso-substituted porphyrins have gained more recognition in this regard.
Phthalocyanines were identified in 1907 (Linstead & Lowe, Citation1934), while its further classification occurred in the 1930s (Durmuş, Yaman, Göl, Ahsen, & Nyokong, Citation2011). Currently, this information has fascinated various second-generation photosensitizers. Pcs are aromatic 18 π-electron compounds that are formulated through the integration of four isoindole unites by nitrogen atoms (). The structure can be replaced on β or α positions to introduce new features like biocompatibility and solubility (Okura, Citation2001). The Pc complexes have been utilized in a multitude of applications because of their fascinating features and chemical stability. Their applications can be further explored in gas sensors (Shaabani, Citation1998), optical limiting uses (Shaabani, Citation1998), catalysts and photodynamic therapy (Liu et al., Citation2004).
In addition, Shaabani (Shaabani, Maleki-Moghaddam, Maleki, & Rezayan, Citation2007), Villemin (Nazeeruddin, Humphry-Baker, Grätzel, & Murrer, Citation1998) and Davis (Pannemann, Dyakonov, Parisi, Hild, & Wöhrle, Citation2001) have reported a high-yield microwave heating method for the preparation of phthalocyanine, metallophthalocyanines, dyes and pigments. Subsequently, another research highlighted the integration of metallophthalocyanines and metal-free bearing tetra aza macrocyclic moieties through microwave irradiation.
Further, Phthalocyanines (Pcs) are categorized as colorations and possess pigment elemental characteristics as well. Likewise, their properties of electrical and ocular features also allow merging several technological tools such as photoconductors and xerographic machines, fabrication systems of LED, demonstrations of electrochromic systems, optical limiters features, the inclusion of photovoltaic properties in solar cells, liquid crystalline (CL) (Allen, Sharman, & Van Lier, Citation2001), multiple catalytic methods and for the dying layers of DVDs (Bonnett, Citation2000). For the application of photodynamic cancer treatment (PDT), Phthalyocyanines are contained significant elements which are considered as photosensitizing agents (Shaabani et al., Citation2007) along with the extensive absorption of visible light within the red portion (Pannemann et al., Citation2001).
In addition, phthalocyanines are reported to possess anti-cancer and anti-tumor activities in many studies (Pannemann et al., Citation2001; Youssef & Mohamed, Citation2011). In addition, Fe (II) phthalocyanine is related porphyrin-based photosensitizers and possesses better safety and biocompatibility (Allen et al., Citation2001). The Fe (II) phthalocyanine is structurally similar to porphyrin that has better photothermal effects in tissues. There is much application of Fe (II) phthalocyanine in the diagnosis and treatments (Hofman et al., Citation2007). There are reports where Fe (II) phthalocyanine loaded with aptamers nanoparticles showed inhibitory action on the breast cancer cells (Bonnett, Citation2000). In another study, it was found that treatment of Fe phthalocyanine coupled with albumin induced anti-cancer activity (Huang et al., Citation2003). In the work reported here, we synthesized Zn phthalocyanine, and Fe (II) phthalocyanine at low temperature by using lithium diisopropylamide (LDA) as nucleophile in THF solution. We have also managed to assess the biological presentations of these compounds by treating bacterial cells and cancer cells to explore whether these synthesized compounds possessed anti-bacterial and anti-cancer capabilities ().
Table 1. XRD data for compounds 1 and 2.
2. Materials and methods
2.1. Materials
For carrying out the alterations of nitrogen, standard Schlenk mechanisms were used. Moreover, lithium diisopropylamide, sulfoxide (DMSO), Fe (CH3COO)2, ZnCl2, Tetrahydrofuran (THF), and potassium carbonate were obtained from (Aldrich) and utilized after buying. Furthermore, other mixtures and matters were also acquired from reputable commercial suppliers. Before the process of distillation and purification of solvents, standardized procedures were adopted prior to the experiment. Standardized procedures were utilized for purification of solvents, and they were also distilled prior to use.
2.2. Physical and FT-IR measurements
By utilization of Bruker spectrometer model 400 MHZ, 1H spectra were recorded. With the experiment of dissolving the 0.6 ml quantity into DMSO-d6 0.5 ml measurement, the samples were prepared. The formalization of KBr with pellets recorded a Bruker IFS 48 spectrometer was also utilized. Similarly, the Elemental Analyzer 1106 was also conducted in this experiment also with taking primary analysis of Carlo Erba. For assessing the ground electron absorbing properties, we utilized a Perkin-Elmer spectrophotometer in our experiment along with the application of ultraviolet of dimethyl sulfoxide. For recording the emission spectra, fluorescence excitation and phosphorescence profiles, a spectro fluorimeter tube vessel with the length of 1 cm along with the properties of Hitachi F-4500 Pc was also applied under normal conditions. By layering the red portion, infrared fallouts and the ultraviolet sources in the light, a quartz lamp image irradiation has been performed along with the application of a cutoff filter (Schott) between 639–730 nm. In order to restrain the light intensity, a potentiometer can also be utilized.
2.3. Synthesis of compounds
2.3.1. Synthesis of iron (II) phthalocyanine Equation(1)
(1)
(1)
A blend of 29H,31H-Phthalocyanine (Zheng, Wan, Zhang, Li, & You, Citation2015) (0.5 g, 0.97 mmol), Fe(CH3COO)2 (154 mg, 0.890 mmol) and undec-7-ene (DBU), both drips of 1,8-diazabicyclo [5.4.0] were warmed in an arid n-pentanol (3 ml) at 25 °C within a wrapped cylinder and mixed for 3h. Towards the end, the green substance was deposited due to the inclusion of 20 ml ethanol after which it was sieved. The green solid product has refluxed for 120 minutes alongside ethanol properties (30 ml), sieved over again, and cleaned through filtered water, diethyl ether and heated ethanol. The product was gone through the process of chromatographed with chloroform-methanol solvent (95:5) after drying it on a normal area.
Yield (79%), as a dark brown solid. IR (KBr): v = 660, 766, 838, 933, 1042, 1315, 1500, 1600, 3013 and 3400 cm−1.
1H NMR: (δ, ppm): 9.40 (m, 2H, Ar-H) and 8.20 (d, 2H, Ar-H) as shown in the spectrum.
13C NMR: (δ, ppm): 161.16 (2 C, -C = N-), 152.19 (2 C, N-C = C-), 136.79 (2 C, -C = N-), 132.99 (4 C, Ar-C), 130.89 (4 C, Ar-C), 128.25 (2 C, Ar-C), 126.57 (2 C, Ar-C), 123.40 (2 C, Ar-C), 122.65 (2 C, Ar-C), 119.68 (2 C, Ar-C), 108.76 (2 C, -C = C-).
2.3.2. Synthesis of zinc phthalocyanine (2)
In addition, the mixtures of ZnCl2 (105 mg, 0.77 mmol) and 29H, 31H-Phthalocyanine (Zheng et al., Citation2015) (0.5 g, 0.97 mmol) were processed within a double drip of undec-7-ene 1,8-diazabicyclo and an arid n-pentanol (3 ml) at 25 °C within a wrapped cylinder and mixed for 3h. Equally, the quantity of 20 ml ethanol and sieved were used to deposit the green substance. Later, the quantity of 30 ml of ethanol and sieved was also used for the refluxing of the green compact mixture for 120 minutes approximately. After that process, the purified water such as warmed ethanol and diethyl ether were used for purifying purposes. After the drying of the product, the process of chromatography was applied to it with usual alumina support and solvent of methanol chloroform (98:2).
Yield (87%), as a dark blue solid. IR (KBr): v = 766, 830, 935, 1042, 1300, 1500, 1610, 3010 and 3330 cm−1.
1H NMR: (δ, ppm): 9.60 (m, 2H, Ar-H) and 8.40 (d, 2H, Ar-H) as shown in the spectrum.
13C NMR: (δ, ppm): 151.46 (2 C, N-C = N-), 137.09 (2 C, -C = N-), 131.78 (4 C, Ar-C), 123.89 (2 C, Ar-C).
2.4. Characterization techniques
The X-ray diffraction (XRD) was utilized for crystallizing the product with XRD-7000 alongside monochromatic increased-power radiation of Cu Kα (λ = 1.5406 Å) with a value of 0.5°/min and 2θ = 10–90°.approximately. In the IR region, Fourier transformation infrared spectroscopy (FT-IR) was utilized to differentiate the bonding of both compounds in this study. The development of major peaks was recorded in of 2-theta range, as the application of XRD spectra was conducted between10 and 40º. The direct scanning of the electron microscope (SEM) (FEI, Inspect S50) was applied for developing the structure, formation, and distribution of the samples along with also concerning on X-Rays (EDX) spectroscopy detector intensive energy seekers for this assessment. Moreover, the two magnifications were shown for the leading SEM images with the values of: five and two µm. for confirming the existence of constituent elements and their distribution, the spectra and mapping of EDX were also evidenced ().
Table 2. Summaries XRD data of the two compounds.
2.5. Anti-cancer activity
2.5.1. Treatment of Iron (II) phthalocyanine and Zinc phthalocyanine on cancerous cells
In this study, Human cervical and human carcinoma colorectal (HeLa) and (HCT-116) were utilized for both cancer cell lines respectively, also determining the influence of this study on Iron (II) phthalocyanine and Zinc phthalocyanine within practicability and propagation of them. In order to scrutinize the specific nature of Iron (II) phthalocyanine and Zinc phthalocyanine, human non-cancer cell lines have also been included in this study, like developing kidney cells which are denoted by (HEK-293).To preserve and refine the cells, the DMEM media is used, 1% of streptomycin, 5% of L-glutamine, 1% of penicillin, 1% of selenium chloride and 10% of FBS as described in the previous method. In a test, the cells were recorded to grow with 96 plates with a value of 5% in a CO2 incubator at the value of 37 °C, and 75–80% value is recorded for the confluence cells and cells are treated for MTT assay.
2.5.2. Cancer cell viability by MTT assay
As per the preceding study (Ansari et al., Citation2021), the MTT assay was established. The cells were used to preserve with Zinc phthalocyanine and Iron (II) phthalocyanine along with maintaining the varied quantities of 2.0 µg till 50 µg/ml values. Additionally, the MTT assay is used to treat the cells for 48 hours consistently and check the cell viability. Zinc phthalocyanine and Iron (II) phthalocyanine were not included in the control group. To examine the specific action of the Iron (II) phthalocyanine and Zinc phthalocyanine, the non-cancer cell of humans was also included, such as embryonic cells of the kidney (HEK-293) in this test. During this assessment, both Zinc phthalocyanine and Iron (II) phthalocyanine-treated groups are tested with 10 µl of 5 mg/ml (MTT) along with these cells are preserved in an oxygen incubator for four hours. After completing the treatment, DMSO (1%) has taken the place of cell culture media and with applying of plate reader ELISA (Bio-tek Instruments, USA), the 96-well plate was then investigated at the distance of 570 nm. The cell practicality percentage was investigated for the analysis.
2.5.3. Apoptotic DAPI staining
Value of DAPI staining evaluation has assisted to examine the DNA of cancer cells (Rehman et al., Citation2020). Two clusters have been formed for testing cells, first one was the control group within which no Zinc phthalocyanine and Iron (II) phthalocyanine was added and the second one is the experimental group, in which Iron (II) phthalocyanine and Zinc phthalocyanine (30 µg/ml) was added. The two groups were converted into the value of 4% of ice-cold in paraformaldehyde and later with Triton X-100 in phosphate-buffered saline were recorded after 48-hour treatment. After that, in an enclosed and dark place, DAPI (1.0 μg/mL) was used to stain the cells for 5 minutes only and then cells were finally washed with PBS along with covering slipped. Moreover, Confocal Scanning Microscope (Zeiss, Germany) was used to examine the DNA staining. The data obtained from triplicates was presented in the form of standard deviation (SD) and mean (±). Besides, GraphPad Software, USA has introduced the analytical software which was used for ANOVA followed by the post of Dennett hoc test in the final statistical analysis.
2.6. Anticandidal Activity
To evaluate the antifungal impact of Iron (II) phthalocyanine and Zinc phthalocyanine, Candida albicansATCC 14053 was selected. The activity was obtained by using the broth dilution technique. This technique analyses the least inhibitory concentration (MIC) and minimum fungicidal act (MFC) of the test compound. Briefly, the attestation of compounds was started from 16 to 1 mg/ml in Sabauraud broth medium (SDB) was prepared with the utilization of sterile tubes and supplemented to the entire nighttime of C. albicans grown SDB (value of 107 CFU/ml was accordingly adjusted). Further, in this experiment, the growth factor without the development of the compound was included. The tubes were left in a shaker incubator for 48 h at 37° C.
After the incubation period was over, the aliquot from each tube was withdrawn and streaked on freshly prepared SDA plates. At the degree of 37 °C, the inoculated plates were additionally incubated for further experiment. The plates were evaluated and observed for MIC and MFC after 48h. The concentration of a test compound that showed 80 to 90% of growth inhibition was considered as the MIC, whereas the concentration of a drug that showed 100% arrest of growth or the colonies less than three was considered as MBC of the test compound (Rehman et al., Citation2020).
3. Results and discussion
3.1. Synthesis and characterization
At the process of low temperature in THF solution, the Phthalocyanine was recorded as synthesized with the assistance of diisopropylamide as a featured nucleophile in the entire process. The relationship between reaction temperature, time, and reactant molar ratio has been studied. The low temperature was enough to gain Phthalocyanine at a high yield along with mixing phthalonitrile with LDA in tetrahydrofuran. The lithium diisopropylamide (LDA) can be enabled to distant the various diisopropylamide anions in the presence of reactions that can be the reason for targeting the cyan cluster on the characteristics of phthalonitrile also worked as a nucleophile (Zheng et al., Citation2015). Thus, the formulation of intermediate IM-1 occurs because IM-2 can be acquired through a reaction between distinct parts within the same molecule. Cyclization, acidification, reduction, and PCs can also be acquired from nucleophilic reactions where the alkoxy (RO) is the nucleophile.
Moreover, with the assistance of column chromatography, all the PCs have been processed as cleansed and thus obtaining the reasonable plain values of 79% and 87% for compounds 1 and 2 respectively ().
Figure 5. The chemical structure of the synthesized Equation(1)(1)
(1) Iron (II) phthalocyanine and (2) Zinc phthalocyanine.

The FTIR spectra results have shown that the synthesis of PCs can cause the absence of nitrile stretches (CN) at approximately 2230 cm−1 as shown in . Moreover, IR spectra shows broad bands at 3441 and 3335 cm−1 for NH and –OH respectively. New bands appeared at 1624–1550 cm−1 and 1320–1000 cm−1 due to unsaturated bonds (aromatic and N = C) and the C-O bonds.
Figure 6. FTIR spectra of the two compounds; Equation(1)(1)
(1) Iron (II) phthalocyanine and (2) Zinc phthalocyanine.

The UV electronic spectra of the synthesized phthalocyanines Fe (II) phthalocyanine and (2) Zn phthalocyanine in DMF exhibited absorption bands in the Q band at 540, 582, and 588. The B-bands were displayed at 330, 335, 352, and 356 nm ().
Figure 7. The absorption spectra of the two compounds; Equation(1)(1)
(1) Iron (II) phthalocyanine and (2) Zinc phthalocyanine.

The crystal structure of the prepared compounds (iron (II) phthalocyanine and zinc phthalocyanine) was studied by XRD, the results of the XRD are displayed by . For both compounds, the main observed peaks are labelled in each spectrum with 2-theta values. The XRD data was obtained using the Bragg’s equation for 0.15406 nm X-ray radiations. The calculated first nine main d-spaings (nm) along with 2θ-values are given as follows (Iron (II) phthalocyanine): 0.5767 (15.36°), 0.5483 (16.16°), 0.3707 (24°), 0.3583 (24.84°), 0.3533 (25.2°), 0.3447 (25.84°), 0.3345 (26.64°), 0.3238 (27.54°) and 0.3157 nm (28.26°). The maximum relative intensity was found for peak 27.54° with d-spaing of 0.3238 nm. For zinc phthalocyanine, three peaks showed the highest peaks among some other lower intensity peaks. The observed peaks (16-peaks) were labelled as 10.56°, 12.4°, 13.9°, 18.06°, 18.62°, 21.1°, 22.1°, 22.86°, 23.5°, 26.1°, 27.86°, 29.04°, 30.4°, 31.3°, 32.04° and 33.04° with d-spings of 0.8375 nm, 0.7136 nm, 0.6369 nm, 0.4910 nm, 0.4764 nm, 0.4209 nm, 0.4021 nm, 0.3889 nm, 0.3785 nm, 0.3413 nm, 0.3201 nm, 0.3037 nm, 0.2939 nm, 0.2857 nm, and 0.2793 nm, 0.2710, respectively. The peak with maximum relative intensity was appeared at 23.5° with d-spaing of 0.4071 nm.
Figure 8. XRD spectra of the two compounds between 2-theta 10 and 40 degrees; (a) Iron (II) phthalocyanine and (b) Zinc phthalocyanine.

Scherrer EquationEquation (1)(1)
(1) calculated the crystallite size of the compounds (given below) utilizing the complete half-maximum width of FWHM within the most critical peak in the XRD model. For example, the maximum intensity has been found at the value of 27.54° for iron (II) phthalocyanine (compound 1) and 25.52° calculated for zinc phthalocyanine (compound 2) as shown in .
(1)
(1)
İn the following equation, the wavelength of the x-rays Cu-Kɑ-radiations has been computed as 0.15406 nm along with denoting it with λ and the k has denoted the value of 0.9 for Scherrer constant. Moreover, the value of Bragg’s angle has been computed at θ and the maximum width of FWHM has been denoted by the value of β as the lattice peak. For compounds 1 and 2, the values have been computed as 33 and 26 nm for the crystallize size correspondingly.
3.2. Morphological and elemental analyses
has demonstrated the morphology surface of the Fe (II) phthalocyanine and Zn phthalocyanine two magnifications along with EDX spectra. The higher crystallizing of Fe (II) phthalocyanine in compound 1 has also been depicted through the bundles’ morphology and straight SEM images. The microbundles were composed of very thin needle-like morphological structures with thickness in the range of a few tens of nanometers. On the other hand, Zn phthalocyanine specimen exhibited a completely different morphology with a compact structure in micron-sized compound particles. The main ingredient element "C" has been shown in the EDX spectrum for compound 1 in which its atomic characteristic was computed nearly 84 value and N" with 6 atomic %. Fe appeared with an atomic % of 7 for this specimen.
Figure 9. SEM micrographs (at two magnifications along with EDX spectrum of (a-c) Iron (II) phthalocyanine and (d-f) zinc phthalocyanine. The scale bars are determined as 5 and 2 μm.

For compound 2, the values are 68, 8, 2 and 13 atomic % for the elements of C, N, Fe and Zn respectively . The synthesis of Fe (II) phthalocyanine and Zn phthalocyanine was confirmed by the EDX spectral analysis. The EDX mapping was also utilized for determining the distribution of the elements, the uniformed distributing along also assisting the results for consistent with EDX spectra as shown in .
3.3. Impact of iron (II) phthalocyanine and Zinc phthalocyanine on cancer cells viability
The potential impacts on colon and cervical cancer cells (HeLa) and (HCT-116) due to Iron (II) phthalocyanine and Zinc phthalocyanine were examined respectively. There was a significant reduction in the cell feasibility post-treatments of Iron (II) phthalocyanine as resulted in the cell feasibility assay. However, on both colon and cervical cancer cells, there were no found inhibitory actions due to phthalocyanine.
The cancer cells were recorded growing and spreading due to the dose-dependent inhibitory action of treatments Iron (II) phthalocyanine. In addition to this, the inhibitory concentration (IC50) of Iron (II) phthalocyanine and Zinc phthalocyanine were also calculated. Besides, The IC50 value for Iron (II) phthalocyanine was calculated as 33 µg/ml and 30 µg/ml values for HeLa and HCT-116 accordingly. After testing the impacts of Iron (II) phthalocyanine and Zinc phthalocyanine, there was not found any HCT-116 reaction on non-cancerous cells HEK-293. With the assistance of these observations, it can be proposed that as compared to HEK-293 cells, synthesized Iron (II) phthalocyanine entails an inhibitory impact on HCT-115 and HeLa cells. So, this has become the first study to indicate the cell feasibility of Zinc phthalocyanine and synthesized Iron (II) phthalocyanine in contrast to HeLa cells and HCT-116. We also have formally concluded about the effect of various molecules (plant extracts and nanomaterials) on breast cancer cells and the colon (Aldakheel et al., Citation2020; El Rayes et al., Citation2019).
3.4. Impact of iron (II) phthalocyanine and Zinc phthalocyanine on cancer cell DNA
There was a major reduction within the amount in cells of cancer due to the treatment of Iron (II) phthalocyanine because in the Iron (II) phthalocyanine -treated cells, the DAPI stained cells has considerably reduced in contrast to control cells (). Cell dead can cause the reduction within the cancer cells along with instigating apoptosis or programmed cell death. On the other side, with respect to any adverse reaction on the colon cancer cells, the treatments of Zinc phthalocyanine have not shown any effects ().
Figure 11. Cancer cell death as a result of treatment of Sample 1: It demonstrates the influence of treatment of Iron (II) phthalocyanine on stained DAPI which was impacted by HCT-116 cells after treatment of 48-hours. Additionally, shape A is considered as the reduced cell and shape B has demonstrated with Iron (II) phthalocyanine showing a substantial amount of cancer cells along with having treatment of 30 µg/ml.

Figure 12. Cancer cell death due treatment of Sample 2: The treatment of Zinc phthalocyanine on colon cancer cells (HCT-116) has been analyzed with stained DAPI post-48-hour treatment. In the same regard, Figure A showcases the control cell and Figure B is treated with Zinc phthalocyanine along with observing no loss of any cell with the value of (30 µg/ml).

3.5. Anticandidal Activity
The anticandidal activity of Iron (II) phthalocyanine and Zinc phthalocyanine was examined by C. albicans. In the current study, anticandidal activity was evaluated by determining MICs and MBCs using broth dilution methods. The MICs values of test compound Iron (II) phthalocyanine were obtained as 16 mg/ml whereas zinc phthalocyanine had no significant inhibitory action on Candida. MFC was not found at any tested concentration, hence MFC of Iron (II) phthalocyanine could be greater than 16 mg/ml. The agar plate that was streaked using the aliquot from the treated broth culture was having a moderate activity against the test compound Iron (II) phthalocyanine () and Zinc phthalocyanine treated plate was seen as fully grown with no suppression of Candida cells ().
4. Conclusions
By using lithium diisopropylamide in THF solution, we synthesized Iron (II) phthalocyanine and Zinc phthalocyanine at low temperatures in this study. The structure and physical characterized were analyzed by FT-IR method. The substantial reduction has been recorded in the cell viability post-treatments of Iron (II) phthalocyanine as established by the cell feasibility evaluation in our research. Further, there was also no adverse action found on both colon and cervical cancer cells due to Zinc phthalocyanine. The cancer cell growth and proliferation can be impacted heavily by dose-dependent inhibitory actions of the treatment of Iron (II) phthalocyanine. Therefore, the reaction of inhibitory concentration (IC50) of Iron (II) phthalocyanine and Zinc phthalocyanine has also been computed in the study. The computed value of IC50 for Iron (II) phthalocyanine was 33 µg/ml for HeLa and for HCT-11 the value 30 µg/ml has been computed, respectively. Moreover, the influence of Zinc phthalocyanine and Iron (II) phthalocyanine on non-cancerous cells; HEK-293, has also been analysed along with establishing non- significant action on HEK-293 cells in this study. Additionally, moderate antifungal activity was seen with Iron (II) phthalocyanine whereas no significant activity was obtained with Zinc phthalocyanine.
Ethical approval
This article does not contain any studies with human participants or animals performed by any of the authors.
Acknowledgements
Authors highly acknowledged the Deanship of Scientific Research (DSR) and Institute for Research & Medical Consultations (IRMC) of Imam Abdulrahman Bin Faisal University for providing the financial assistance for this study.
Disclosure statement
No potential conflict of interest was reported by the authors.
Data availability statement
All data will be available upon request to corresponding author.
Additional information
Funding
References
- Aldakheel, R. K., Rehman, S., Almessiere, M. A., Khan, F. A., Gondal, M. A., Mostafa, A., & Baykal, A. (2020, August 13). Bactericidal and in vitro cytotoxicity of Moringa oleifera seed extract and its elemental analysis using laser-induced breakdown spectroscopy. Pharmaceuticals (Basel), 13(8), 193. PMID: 32823699; PMCID: PMC7464216. doi:10.3390/ph13080193
- Allen, C. M., Sharman, W. M., & Van Lier, J. E. (2001). Current status of phthalocyanines in the photodynamic therapy of cancer. Porphyrins and Phthalocyanines, 5(02), 161–169. doi:10.1002/jpp.324
- Ansari, M. A., Asiri, S. M. M., Alzohairy, M. A., Alomary, M. N., Almatroudi, A., & Khan, F. A. (2021, February 9). Biofabricated fatty acids-capped silver nanoparticles as potential antibacterial, antifungal, antibiofilm and anticancer agents. Pharmaceuticals (Basel), 14(2), 139. PMID: 33572296; PMCID: PMC7915658. doi:10.3390/ph14020139
- Battersby, A. R., Fookes, C. J., Matcham, G. W., & McDonald, E. (1980). Biosynthesis of the pigments of life: Formation of the macrocycle. Nature, 285(5759), 17–21. doi:10.1038/285017a0
- Bonnett, R. (2000). Chemical aspects of photodynamic therapy (1st edn). London: CRC Press.
- Durmuş, M., Yaman, H., Göl, C., Ahsen, V., & Nyokong, T. (2011). Water-soluble quaternized mercaptopyridine-substituted zinc-phthalocyanines: Synthesis, photophysical, photochemical and bovine serum albumin binding properties. Dyes and Pigments, 91(2), 153–163. doi:10.1016/j.dyepig.2011.02.007
- El Rayes, S. M., Aboelmagd, A., Gomaa, M. S., Ali, I. A. I., Fathalla, W., Pottoo, F. H., & Khan, F. A. (2019, October 28). Convenient synthesis and anticancer activity of methyl 2-[3-(3-phenyl-quinoxalin-2-ylsulfanyl) propanamido]alkanoates and N-Alkyl 3-((3-phenyl-quinoxalin-2-yl)sulfanyl)propanamides. ACS Omega, 4(20), 18555–18566. PMID: 31737814; PMCID: PMC6854567. doi:10.1021/acsomega.9b02320
- Hofman, J.-W., van Zeeland, F., Turker, S., Talsma, H., Lambrechts, S. A. G., Sakharov, D. V., … van Nostrum, C. F. (2007). Peripheral and axial substitution of phthalocyanines with solketal groups: Synthesis and in vitro evaluation for photodynamic therapy. Journal of Medicinal Chemistry, 50(7), 1485–1494. doi:10.1021/jm061136w
- Huang, J. D., Fong, W. P., Chan, E. Y., Choi, M. T., Chan, W. K., Chan, M. C., & Ng, D. K. (2003). Photodynamic activities of a dicationic silicon (IV) phthalocyanine and its bovine serum albumin conjugates. Tetrahedron Letters, 44(43), 8029–8032. doi:10.1016/j.tetlet.2003.07.007
- Krautler, B. (1987). The porphinoids-versatile biological catalyst molecules. Chimia, 41(9), 277–292.
- Linstead, R. P., & Lowe, A. R. (1934). Phthalocyanines. Part III. Preliminary experiments on the preparation of phthalocyanines from phthalonitrile. The Chemical Society, 1022–1027. doi:10.1039/jr9340001022
- Liu, M. O., Tai, C. H., Wang, W. Y., Chen, J. R., Hu, A. T., & Wei, T. H. (2004). Microwave-assisted synthesis and reverse saturable absorption of phthalocyanines and porphyrins. Organometallic Chemistry, 689(6), 1078–1084. doi:10.1016/j.jorganchem.2004.01.017
- Liu, Y.-Y., Wang, Y., Walsh, T. R., Yi, L.-X., Zhang, R., Spencer, J., … Shen, J. (2016). Emergence of plasmid-mediated colistin resistance mechanism MCR-1 in animals and human beings in China: A microbiological and molecular biological study. The Lancet. Infectious Diseases, 16(2), 161–168. doi:10.1016/S1473-3099(15)00424-7
- Maisch, T. (2007). Anti-microbial photodynamic therapy: Useful in the future. Lasers in Medical Science, 22(2), 83–91. doi:10.1007/s10103-006-0409-7
- Nazeeruddin, M. K., Humphry-Baker, R., Grätzel, M., & Murrer, B. A. (1998). Efficient near IR sensitization of nanocrystalline TiO2 films by ruthenium phthalocyanines. Chemical Communications, 6, 719–720. doi:10.1039/a708834e
- Okura, I. (2001). Photosensitization of porphyrins and phthalocyanines. London: Gordon and Breach Publishers.
- Pannemann, C., Dyakonov, V., Parisi, J., Hild, O., & Wöhrle, D. (2001). Electrical characterization of phthalocyanine-fullerene photovoltaic devices. Synthetic Metals, 121(1–3), 1585–1586. doi:10.1016/S0379-6779(00)01305-9
- Rehman, S., Almessiere, M. A., Khan, F. A., Korkmaz, A. D., Tashkandi, N., Slimani, Y., & Baykal, A. (2020, April). Synthesis, and biological characterization of Mn0.5Zn0.5EuxDyxFe1.8-2xO4 nanoparticles by sonochemical approach. Materials Science & Engineering. C, Materials for Biological Applications, 109, 110534. Epub 2019 Dec 6. PMID: 32228890. doi:10.1016/j.msec.2019.110534
- Ryskova, L., Buchta, V., & Slezak, R. (2010). Photodynamic antimicrobial therapy. Central European of Biology, 5(4), 400–406. doi:10.2478/s11535-010-0032-2
- Senge, M. O., Sergeeva, N. N., & Hale, K. J. (2021). Classic highlights in porphyrin and porphyrinoid total synthesis and biosynthesis. Chemical Society Reviews, 7, 4730–4789. doi:10.1039/C7CS00719A
- Shaabani, A. (1998). Synthesis of metallophthalocyanines under solvent-free conditions using microwave irradiation. Chemical Research, Synopses, 10, 672–673. doi:10.1039/a708858b
- Shaabani, A., Bahadoran, F., & Safari, N. (2001). Synthesis of phthalocyanine derivatives of the Ru, Rh, Pt and Pd metals under solvent free conditions using microwave irradiation. Indian Journal of Chemistry, 40A(02), 195–197.
- Shaabani, A., Maleki-Moghaddam, R., Maleki, A., & Rezayan, A. H. (2007). Microwave assisted synthesis of metal-free phthalocyanine and metallophthalocyanines. Dyes and Pigments, 74(2), 279–282. doi:10.1016/j.dyepig.2006.02.005
- Smith, K. M. (Ed.). (1975). Porphyrins and metalloporphyrins (Vol. 9). Amsterdam: Elsevier.
- Von Tappeiner, H. (1904). Ueber wirking der photodynamichen (fluorescierenden) Stoffe auf Protozoan und Enzyme. Deutsches Archiv für klinische Medicin, 80, 427–487.
- World Health Organization. (2014). Antimicrobial resistance: Global report on surveillance. Geneva, Switzerland: World Health Organization.
- Xavier, B. B., Lammens, C., Ruhal, R., Kumar-Singh, S., Butaye, P., Goossens, H., & Malhotra-Kumar, S. (2016). Identification of a novel plasmid-mediated colistin-resistance gene, mcr-2, in Escherichia coli, Belgium. Eurosurveillance, 21(27), 30280. doi:10.2807/1560-7917.ES.2016.21.27.30280
- Youssef, T. E., & Mohamed, H. H. (2011). Synthesis and photophysicochemical properties of novel mononuclear rhodium (III) phthalocyanines. Polyhedron, 30(12), 2045–2050. doi:10.1016/j.poly.2011.05.017
- Zheng, W., Wan, C. Z., Zhang, J. X., Li, C. H., & You, X. Z. (2015). Facile synthesis of phthalocyanine at low temperature with diisopropylamide anion as nucleophile. Tetrahedron Letters, 56(30), 4459–4462. doi:10.1016/j.tetlet.2015.05.086