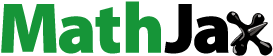
Abstract
There are on-going investigations on the utilization of pineapple leaf fibre (PALF) as a cheap, safe, and eco-friendly natural fibre. The present work aimed to improve PALF preparation using ultrasonication, investigate the adsorption kinetic and thermodynamic aspects of PALF dyed with berberine dye, and to assess their associated molecular interactions. It was found that concomitant PALF exposure to NaOH solution (5% w/v) plus ultrasonication for 20 min successfully removed non-cellulosic substances from the fibre surface in a very much shorter time compared with the traditional non-ultrasound assisted method (24 h). An adsorption isotherm study of dyeing, the treated fibre with the natural yellow dye berberine revealed a strong adsorption dependence on the pH of the berberine solution with optimal uptake occurring at pH 9–10. A deeper assessment of the mode of dye binding was undertaken by DFT − based calculations on a model cellulose unit and berberine. It revealed that the interaction between berberine and cellulose (PALF) at pH 9.00 was likely to be substantially stronger than at lower pH. From the adsorption kinetic results, a higher affinity and more dye uptake were observed before equilibrium was reached. The adsorption isotherm data could be represented by the Langmuir isotherm (R2 > 0.99). The enthalpy change (ΔH°) and entropy change (ΔS°) values of dyeing were found to be −17.0 kJ mol−1 and −19.4 J mol−1 K−1 respectively, which pointed to the dye adsorption being an exothermic process. The negative standard affinity (Δµo) values at the different temperatures of 30, 60, and 80 °C were −11.2, −10.6, and −10.2 kJ mol−1, respectively. It affirmed the dye adsorption process as a spontaneous one. Higher temperatures resulted in a lower affinity and reduced dye uptake compatible with the process being exothermic. Berberine is a potentially useful natural dye for PALF without the need for additives.
1. Introduction
Pineapple leaf material with its fibre component (Pineapple Leaf Fibre; PALF) is a significant food-industrial by-product in Thailand. In an effort to manage and reduce such waste, the utilization of PALF as a natural, bio-degradable, eco-friendly, safe and cheap fibre has been increasing exponentially (Todkar & Patil, Citation2019). PALF is a white, smooth and glossy fibre similar to in texture to silk, with a high tensile strength and good flexural and torsional characteristics (Asim et al., Citation2015; Py, Lacoeuilhe, & Teisson, Citation1987). It has a soft surface and absorbs water well due to its hydrophilicity from the high cellulose content (Bengtsson, Gatenholm, & Oksman, Citation2005; Cherian et al., Citation2010; George, Sreekala, & Thomas, Citation2001). PALF is normally extracted from pineapple leaves by mechanical and retting methods (Das, Chakravarty, Sinha, & Ghosh, Citation1978). PALF represents about 2–3% by weight of the pineapple leaf. PALF is a lignocellulosic fibre being composed of α-cellulose (81.3%), hemicellulose (12.3%), and lignin (3.5%), the rest being wax, pectin, and other compounds (Jose, Salim, & Ammayappan, Citation2016; Rahman, Citation2011). PALF is characterized by a higher cellulose content compared with other plant-derived fibres such as those from banana stems (Khalil, Alwani, & Omar, Citation2006).
As well as natural fibres themselves, there is much research interest in using non-synthetic dyes for the dyeing of these fibres with some of the driving forces being their non-polluting and eco-compatible nature when compared with most synthetic dyes which are not biodegradable and can cause ecological problems (Haji, Citation2010; Uddin et al., Citation2022). Natural dyes come from various natural sources, but mainly plants, and structurally cover a range of different types including alkaloids as exemplified by the yellow benzylisoquinoline-derived quaternary alkaloid berberine (Che & Yang, Citation2022; Gupta, Citation2019; Pargai, Jahan, & Gahlot, Citation2020). In addition, some of these dyes have other desirable properties such as ultraviolet protection capability, fire retardancy, antioxidant, and antibacterial activity (Kamel & Mashaly, Citation2013). Berberine (), which can be extracted from a range of plants (Neag et al., Citation2018), also has antibacterial activity and can be used as a colorant for textile dyeing, especially for silk fibre. A thermodynamic study of the adsorption of berberine on silk indicated consistency with the Langmuir isotherm (Ke, Xu, & Yu, Citation2008). In that study, they found that the negative value of the enthalpy change (ΔH°) pointed to an exothermic dyeing process, in line with the increased adsorption capacity seen with a decrease in temperature. Berberine extracted from Berberis vulgaris wood has also been used to dye wool fibre together with Rumex hymenosepolus extract as a bio-mordant (Haji, Citation2010). In this case, the tannin-containing bio-mordant extract enhanced fastness properties of the dyed material. Previously published studies have also reported that berberine dye can be used as a natural antimicrobial agent as well as an antibacterial agent on textile fibres such as cotton (Liu, Lin, & Liang, Citation2019) and polyamide (Ravikumar, Kim, & Son, Citation2007).
The dyeing of cotton by berberine has been described (Du, Xu, Yue, Xu, & Wang, Citation2022; Haji, Citation2013) as well as berberine dyeing of cellulosic fibres modified with a reactive anionic agent (Kim & Son, Citation2005). When cotton fabric was pretreated with an anionic agent, the exhaustion-fixation efficiency increased compared to the non-treated fabric. The amount of berberine dye required to exhaustively dye cotton fibres modified with a reactive s-triazinyl anionic reagent has been determined (Kim, Yoon, & Son, Citation2004). This work revealed that the berberine exhaustion-fixation onto the cotton fibres treated with this triazinyl reagent was much higher than that for the untreated fibres. The anionic sites allowed for significant Coulombic interactions with the positively charged berberine molecules. Furthermore, the reactive anionic agent did not significantly affect the color shade of the dyed cotton fibre. In addition, cotton dyed with berberine has high antibacterial activity because of its cationic quaternary ammonium ion functionality (Kim & Son, Citation2005).
While PALF has been used in dyeing studies, the dyes involved have been synthetic ones (Shamsul Alam, Arifuzzaman Khan, & Abdur Razzaque, Citation2009) including, recently, the synthetic reactive dye LevafixTM (de Queiroz, da Silva, Broega, & Souto, Citation2020) and MX Basic Red 310 N 11b Procion MX reactive dye (Amin et al., Citation2021). The grades of color change in color fastness to laundering of PALF from two species, curaua (A. lucidus) and red pineapple (A. bracteatus (Lindl.), dyed with LevafixTM were all at the good rating level of 5. Dyeing of PALF with Procion MX reactive dye using an infrared dyeing machine gave fibre with a golden shade and a rating level of 3 in color change to washing fastness. However, there has been relatively little work with natural dyes (Sricharussin, Ree-Iam, Phanomchoeng, & Poolperm, Citation2009) and none with berberine, despite its ready availability and interesting functional properties. In the work of Sricharussin et al. (Citation2009), mainly flavonoid dyes originating from different plant sources were used in conjunction with enzymatic pre-treatment of pineapple leaf fibres and improved dyeing results were seen with PALF treated in this way.
The aim of the present work was to determine whether a different natural dye, the alkaloid berberine, could also effectively dye PALF directly. Such dyeing of PALF with berberine has not been reported previously. In this context, our work then focused initially on the efficient treatment of PALF to remove non-cellulosic substances using ultrasonication compared with the traditional non-ultrasound assisted method, and any consequent effects on dyeing. Further adsorption kinetic and thermodynamic studies of the dyeing process of PALF with berberine dye then followed. This was done to assess, quantitatively, the amenability of PALF to dyeing with this dye, in conjunction with an investigation of the associated molecular interactions using Density Functional Theory (DFT). The key thermodynamic parameters evaluated included standard affinity (Δµo), enthalpy change (ΔH°) and entropy change (ΔS°) with respect to different dyeing temperatures. In addition, the important effects of pH, contact time, and adsorption rate constant (k) values at different dyeing temperatures on dye adsorption were determined. Moreover, in order to deepen understanding of the molecular basis of the dye-fibre interaction, Density Functional Theory (DFT) − based computations were undertaken to examine the possible interactions between berberine and cellulose (PALF) and to understand the effect of pH on dye uptake. It was expected this would then provide a basis to rationally fine tune dyeing conditions for the most effective dyeing of PALF in the future.
2. Materials and methods
2.1. Materials
The National Metal and Materials Technology Center (MTEC), Pathum Thani, Thailand provided the PALF material. Berberine chloride, (C20H18ClNO4, Natural Yellow 18, CAS 633-65-8; Sigma-Aldrich, India), sodium hydroxide (NaOH ≥ 99% assay; Merck, Germany) and glacial acetic acid (CH3COOH 100% anhydrous, 99.6% assay; Merck, Germany) used in this study were analytically pure.
2.2. Instruments
The PALF was cleaned with an ultrasonic cleaner (Elmasonic P 30 H, 50/60 Hz, 230–240 V, Germany) was used to clean the PALF. Use was made of a thermostatted shaker bath (SWB 5050 shaking water bath; National Labnet Company, USA) for dyeing and an Orion 42 A pH meter (Thermo Scientific, USA) for pH measurements. The surface morphology work was undertaken with a field emission scanning electron microscope (FESEM) (Merlin Compact; Zeiss, Germany) and CIELab values for all samples were measured on a LabScan XE 0/45 colorimeter (Hunter Lab, USA). Solution absorbance measurements were obtained with a Unicam 310 UV-vis spectrophotometer (Unicam Instruments, UK) using 1 cm path length quartz cells. A universal testing machine (Lloyd Instruments Ltd, UK) was used for the PALF tensile strength measurements before and after cleaning with NaOH solution (5.0% w/v) under ultrasonication. A Bruker/Tensor 27 (USA) FT-IR spectrometer was employed to investigate the ATR-FTIR spectra of PALF and the PALF dyed with berberine dye.
2.3. Methods
2.3.1. PALF preparation
An ultrasound-assisted NaOH solution combination method was used to treat PALF and this was compared with the traditional non-ultrasound assisted method (Tangtubtim & Saikrasun, Citation2019) of preparing PALF. To remove any remaining impurities and covering materials, PALF was sonicated with a NaOH solution (1%, 3%, and 5% w/v) in an ultrasonic bath, used at a frequency of 80 kHz, 100 W, and pulse mode at 40 °C, while maintaining a 1:100 material to liquor ratio. The effect of treatment time (10, 20, 30, and 60 min) was also studied.
In a separate experiment following the traditional method, PALF was immersed in a solution of NaOH (5% w/v), shaken for 10 min, and then allowed to soak at room temperature for 24 h. Finally, the obtained PALF from both methods were squeezed to remove excess solvent, washed with tap water until the rinsed water was at near neutral pH (6.8), and then dried in air at room temperature (24 h).
2.3.2. Characterization of PALF
Surface morphologies of PALF samples from both methods were characterized using FESEM prior to the isotherm study. The treated and untreated PALF samples were also assessed for fibre strength with the universal testing machine. This machine provided tensile strength values for the PALF samples, given an average diameter of 0.065 mm, gauge length of 10 mm, and pulling speed of 50 mm min−1. This experiment gave data on tensile strength changes in the treated PALF samples compared with the untreated PALF sample. ATR-FTIR spectra of PALF before and after dyeing with berberine dye were carried out using the horizontal reflection ATR accessory with a zinc selenide crystal and a scan number of 32 scans in the range of 4000–500 cm−1.
2.3.3. Color measurement
All samples were wound around the skein holder, and for each measurement, the average of three readings was evaluated. The reflectance and the values of CIE L*a*b* coordinates of berberine dyed fibre samples were measured using a benchtop LabScan XE spectrophotometer (a reflectance Specular Exclude (SPEX) instrument) operating with 0o/45o optical geometry, illuminant D65 and 10o standard observer. The CIE L*a*b* coordinates were obtained from the tristimulus values, X, Y and Z under illuminant. Color was described by the color indices derived from the CIE L*a*b* values. The ΔE* (color difference) value was determined using EquationEquation (1)(1)
(1) :
(1)
(1)
in which ΔE* is the color difference value, ΔL* = L*treated sample − L*untreated sample; Δa* = a*treated sample − a*untreated sample; Δb* = b*treated sample − b*untreated sample; “L*” delineates lightness, “a*” evaluates redness (+positive) or greenness (−negative) and “b*” is indicative of yellowness (+positive) or blueness (−negative). Depth of shade (K/S) values for all samples were determined by the light reflectance technique and applying the Kubelka-Munk EquationEquation (2)
(2)
(2) (Judd & Wyszecki, Citation1975):
(2)
(2)
with R being the observed reflectance, K the absorption coefficient, and S the light scattering coefficient. A higher K/S value was commensurate with better dye uptake by the fibres.
2.3.4. Initial pH effect of the berberine dye solution
In the current study, the initial pH of the dye solution was modulated over the pH range (3–10) by adjustment with a glacial acetic acid or NaOH solution (2.00 mol L−1). These experiments involved shaking treated PALF samples with an initial dye bath concentration of 250 mg L−1 at different starting pH values and at a material to liquor ratio of 1:100 in a screw-capped conical flask in a thermostatted shaker bath (operated at 150 strokes min−1 for 60 min). After 60 min, the dyed PALF samples were withdrawn quickly, washed with tap water to eliminate the unfixed dye, and then air dried at room temperature for 24 h. The residual dye concentration in the bath liquor was determined via UV-Visible spectrophotometry at λmax 340 nm, using a dye standard calibration curve (y = 0.0457x, R2 = 0.999). The amount of dye adsorbed per gram of treated PALF at equilibrium (qe, mg g PALF−1) was calculated using EquationEquation (3)(3)
(3) .
(3)
(3)
In this equation, Ci and Ce are the initial dye and the equilibrium dye concentrations (mg L−1), respectively, while V is the volume of the dye solution (L) and W is the weight of treated PALF (g).
2.3.5. Batch adsorption kinetic experiments
Berberine dye solution (250 mg l−1) was freshly prepared in distilled water at pH 9.0. The required dye solution in a screw-capped conical flask (500 ml) was shaken in a thermostatted shaker bath operated at 150 strokes min−1. PALF was pre-warmed in a thermostatted bath for 30 min and then was directly immersed in the dye solution keeping a material to liquor ratio of 1:100 at the required temperature. At each selected time interval, the dye solution (0.80 ml) was pipetted and then diluted with distilled water to 10.00 ml (final pH 9.0) and its absorbance then measured at λmax 340 nm. Concentrations of berberine dye at time zero and at subsequent times were determined using a calibration curve based on absorbance at λmax 340 nm vs dye concentrations in standard berberine dye solutions (y = 0.0457x, R2 = 0.999). The amount of dye adsorbed per gram of PALF (qt, mg g PALF−1) at a selected time was calculated using the mass balance EquationEquation (3)(3)
(3) . Bath temperatures of 30, 60, and 80 °C were used for the adsorption kinetic studies.
2.3.6. Batch isotherm experiments
Different initial concentrations of berberine dye solution (10–600 mg L−1) were each freshly prepared in a NaOH solution at an initial pH of 9.0. The experiments were carried out by shaking treated PALF samples with different dye concentrations at 30, 60, and 80 °C in a thermostatted shaker bath under the conditions as described in Section 2.3.4. After attaining equilibrium (60 min), the dyed PALF samples were taken out of the bath rapidly, washed with tap water to remove the unfixed dye, and then dried in air at room temperature for 24 h. Dye concentrations were determined at time zero and at the equilibrium time by using the berberine dye standard calibration curve (Section 2.3.4). EquationEquation (3)(3)
(3) was then applied to ascertain the amount of dye adsorbed per gram of treated PALF at equilibrium (qe, mg g PALF−1).
2.3.7. Computational details
Density Functional Theory (DFT) − based structure optimizations were computed using the DMol3 module in the materials studio software package (version 7.0) (Delley, Citation1990; Delley, Citation2000). The generalized gradient approximation (GGA) using the Perdew − Burke − Ernzerhof (PBE) functional (Perdew, Burke, & Ernzerhof, Citation1996) formed the basis for the exchange and correlation interactions and the all electron density functional was applied. The localized double numerical basis set together with polarization functions (DNP) was employed for all the atoms to enlarge the Kohn-Sham orbitals. The DNP basis set is similar to 6–31 G** Gaussian basis sets (Wiberg, Citation1986) but with a better accuracy for a similar basis set size. The DFT − D correction methods were represented by the Grimme scheme (Grimme, Citation2006). All the calculations were done with a real-space global orbital cutoff radius of 3.7 Å. For the convergence tolerances, the geometry optimization was set to 10−5 Ha for the energy, 0.002 Ha/Å for the force, and 0.005 Å for the displacement. The electronic SCF tolerance used was 10−5 Ha. The k-point set of (5 × 5 × 1) was used for supercell calculations with a thickness of 20 Å. The optimized structures of the cellulose model unit and berberine are shown in . The CH2OH residues and carboxylic acid (–COOH) groups in cellulose were chosen as active sites at pH 5 (), whereas the carboxylate anion (–COO–) and the CH2OH residues in cellulose were chosen as active sites at pH 9.0 (). At pH 9.0, the very weakly acidic primary alcohol group (expected pKa ca. 16) would be essentially unionized.
Figure 2. The model of cellulose from PALF at (a) pH 5.0 and (b) pH 9.0 and (c) the optimized structure of berberine.

The adsorption energies () of the berberine molecule and cellulose from PALF were defined as follows in EquationEquation (4)
(4)
(4) :
(4)
(4)
where
and
are the total energies of berberine with the cellulose, berberine molecules, and cellulose molecules, respectively. The more negative
is indicative of a higher adsorption strength.
3. Results and discussion
3.1. PALF preparation
To reduce time and cost for the PALF preparation, ultrasound-assistance was employed in combination with exposure to NaOH solution in contrast to the traditional NaOH only method (Tangtubtim & Saikrasun, Citation2019). Therefore, the determination of optimum conditions for PALF treatment was studied such as the treatment time and concentration of NaOH solution used. The effects of treatment time (10, 20, 30, and 60 min) and different concentrations of NaOH solution (1%, 3%, and 5% w/v) were investigated by color measurement, FESEM and tensile strength. The effect of treatment time on the treated PALF samples with the combination of the ultrasound-assisted method and NaOH solution (5% w/v) at different treatment times compared with PALF samples treated only with NaOH solution (5% w/v) for 24 h (traditional method) is shown in . It was found that, the color difference (E*) and L* values of the NaOH-ultrasound treated PALF samples at different treatment times increased when the treatment time increased from 10 to 20 min and then remained constant from 20 to 60 min. At the 20 min treatment time, no significant changes to the color difference (
E*) and CIE L*a*b* values were observed compared with the treated PALF with NaOH solution (5% w/v) alone but it reduced the time and cost for treatment. This can be explained by the fact that ultrasonication enhanced the removal of any remaining impurities and non-cellulosic material (hemicellulose, lignin, pectin and other compounds) in the cuticle primary wall of the cell into the water probably as a result of the accompanying diffusion, dispersion and degassing effects. Ultrasound-induced asymmetric cavity bubble collapse can produce very small water surges directed towards the cell wall, resulting in erosion and consequently increased removal of other non-cellulosic fibre components.
Table 1. CIE L*a*b* values of untreated and treated PALF samples with the combination of ultrasound-assistance and NaOH solution (5% w/v) (Method A) at different treatment times compared with PALF treated with NaOH solution (5% w/v) (Method B) for 24 h using the traditional method.
To optimize the concentration of NaOH solution, PALF samples were sonicated with different concentrations of NaOH solution (1%, 3%, and 5% w/v) in an ultrasonic bath for 20 min (the optimum treatment time) compared with the treated PALF sample with NaOH solution (5% w/v) for 24 h using the traditional method. The results are displayed in . An increase in the NaOH concentration from 1% to 5% w/v led to an enhancement in the color difference (E*) and lightness (L*) values. The PALF sample treated with a NaOH solution (5% w/v) under ultrasonication did not result in any significant changes in the color difference (
E*) and lightness (L*) values. Therefore, the optimum concentration of NaOH solution for PALF treatment was found to be at 5% w/v.
Table 2. CIE L*a*b* values of untreated and treated PALF samples with the combination of the ultrasound-assisted procedure and different concentrations of NaOH solution (Method A) using a 20 min treatment time compared with PALF treated with NaOH solution (5% w/v) for 24 h using the traditional method (Method B).
FESEM was used to assess the surface morphologies of all treated PALF samples compared with untreated PALF sample as shown in . The results indicated that the untreated PALF sample had cell wall fragments remaining on the surface of the fibre bundles as seen in . The other non-cellulosic substances were removed by NaOH treatment (traditional method) or using the combination of the ultrasound-assisted method and NaOH solution as shown in . From the literature, the cell walls of PALF exhibit obviously different primary and secondary layers (Gholampour & Ozbakkaloglu, Citation2020). From , the treated PALF with 1% and 3% w/v NaOH solutions had removed non-cellulosic materials in the cuticle primary wall. In the current study, PALF treated with a NaOH solution (5% w/v) had removed primary wall material and non-cellulosic materials in the cuticle secondary wall ().
Figure 3. Scanning electron micrographs of (a) untreated PALF, (b) treated PALF with the traditional method (5% w/v NaOH, 24 h), and treated PALF with (c) 1%, (d) 3%, and (e) 5% w/v NaOH solutions using the ultrasound-assisted method (20 min) at magnification 1000X.

The untreated and treated PALF samples were also tested for tensile strength to further assess if any physical changes had occurred in the PALF after treatment with NaOH solution under ultrasonication. It was found that PALF resulting from such treatment underwent a significant decline in tensile strength. This decline may be due to the structural changes caused by alkali treatment under ultrasonication, as indicated in the FESEM image () which revealed degradation of non-cellulosic substances at the fibre surface. The tensile strength of the untreated PALF in this study was found to be 1.59 ± 0.57 N. The tensile strength of PALF treated with a NaOH solution (5% w/v) under ultrasonication for 20 min (0.89 ± 0.19 N) was similar to that observed for the PALF treated with a NaOH solution (5% w/v) alone for 24 h (0.96 ± 0.50 N; traditional method) (Sricharussin et al., Citation2009). These results confirmed that PALF treatment using the combination of the ultrasound-assistance and NaOH solution (5% w/v) is effective in removing non-cellulosic substances from the fibre surface in a much shorter time compared with the traditional method although there was some decrease in fibre tensile strength.
3.2. The influence of initial pH on uptake of dye
An important controlling factor in dye uptake is quite often the pH of the dye solution. The effect of initial pH on berberine dye adsorption on PALF at constant initial dye concentration (250 mg L−1), a contact time of 60 min and a temperature of 60 °C is demonstrated in . It was found that the dye uptake into PALF continued to increase with increasing pH values from 3 to 9; the highest dye uptake was observed at pH 9.0. Berberine chloride is a quaternary ammonium salt with a formal positive charge on nitrogen and chloride as the counter ion. The quaternary ion form is present in acidic, neutral and mildly basic solutions. In strongly alkaline solutions at pH 12, it is not stable and undergoes reaction with hydroxide ion to give the neutral compound 8-hydroxy-7,8-dihydroberberine (berberinol; Dostál et al., Citation2004) and ultimately oxyberberine and dihydroberberine (Jeffs, Citation1967). The increased berberine dye binding seen with PALF under alkaline conditions is most likely due mainly to the ionized carboxylic acid groups (carboxylate ion; negative charge) in the cellulose interacting by ion-ion interactions with berberine (positive charge) in a mildly basic solution at pH 9; hydrogen bond interactions (including CH···O interactions) are also likely to occur as illustrated in . The carboxylic acid groups in cellulose can arise by oxidation probably mainly of the CH2OH residues and to a small extent from aldehyde groups. The pKa of the COOH groups would be ca. 4.0, so they would essentially be fully ionized in basic solution. There would be less ionized form present in acidic solutions (ca. 50% ionized at pH 4.0) and hence less ion-ion interaction with berberine under acidic conditions. The starting pH of the berberine dye solution was then fixed at 9.0 for the adsorption thermodynamic study.
Figure 4. (a) The effect of initial pH of berberine dye adsorption on treated PALF (initial dye concentration of 250 mg L−1, 60 °C and contact time of 60 min) and (b) schematic representation of the interactions between the quaternary berberine ion and a model cellulose unit (4 sugar residues shown) from PALF under mildly basic conditions (pH 9.0).

3.3. Computational study of the molecular interactions
Prior to examining the adsorption isotherm, we used DFT calculations to go deeper into the possible interactions between berberine and cellulose at pH 5.0 and pH 9.0. shows the optimized structures of cellulose and berberine (berberine core); these structures are in line with those obtained from crystallographic studies (Kornilova, Glebov, Castañeda, & Timofeeva, Citation2022; Nishiyama, Langan, & Chanzy, Citation2002). Two hydrogen bonds and an ion–dipole interaction were noted at pH 5.0 (see ). The bond distance of the ion-dipole interaction of N···(OHCH2–) was calculated to be 3.71 Å with an energy of adsorption of −1.64 eV. In the case of pH 9.0 (), the interaction between cellulose and berberine showed anion-ion attraction and hydrogen bonds, including CH···O interactions. We found that the bond length of N···(OCO–) is shorter than N···(OHCH2–), with the stronger adsorption energy of −2.70 eV. Charge transfers were also considered in order to obtain additional information by using the charge analysis described by Hirshfeld (Hirshfeld, Citation1977). The results showed that the charge transfer differences of berberine (ΔQ (BBR)) have a negative value, indicating that berberine received the electrons from cellulose. Moreover, ΔQ (BBR) at pH 9.0 showed more negative charge transfer by 0.474e than at pH 5.0, which infers that a greater number of differential charge transfers results in a higher adsorption energy. In summary, the DFT calculations revealed that pH 9.0 was the best pH for adsorbing berberine which is consistent with the initial pH experimental results ().
3.4. Rate of dyeing
The effect of temperature on PALF dyed with berberine dye at 30, 60, and 80 °C is shown in . At the initial stage, the increase of the amount of the dye uptake with increasing temperature because of the more rapid dye diffusion to cellulose chain (PALF) at higher temperatures (Burkinshaw, Citation2016). After the equilibrium time, the amount of dye uptake at high temperature was lower than at low temperature. It pointed to the berberine dyeing on PALF at pH 9.0 being exothermically controlled. Our data showed that the equilibrium times were 30, 25, and 10 min at dyeing temperatures of 30, 60, and 80 °C, respectively. This indicated that the dyeing rate into PALF occurred much more rapidly at higher temperatures, resulting in the shorter time to reach equilibrium. However, the isotherm study of PALF dyed with berberine dye was fixed at 60 min to ensure that full equilibrium had been attained.
Figure 6. (a) The influence of temperature on dye adsorption with an initial dye concentration of 250 mg l−1 at pH 9.0 and (b) a plot of versus ln t for the dye adsorption on PALF with an initial dye concentration 250 mg l−1 at pH 9.0.
![Figure 6. (a) The influence of temperature on dye adsorption with an initial dye concentration of 250 mg l−1 at pH 9.0 and (b) a plot of ln [−ln (1−qt2/qe2)] versus ln t for the dye adsorption on PALF with an initial dye concentration 250 mg l−1 at pH 9.0.](/cms/asset/d200fb7c-3acd-47a7-af96-5f0fbdf1641f/tabs_a_2218199_f0006_c.jpg)
The modified Cegarra-Puente (EquationEquation (5)(5)
(5) ) can be applied to a rapid dye sorption rate which happens in the initial stage of the dyeing process. The adsorption rate constants (k) at the different dye bath temperatures over the full range of exhaustion values (Burkinshaw, Citation2016; Cegarra, Puente, & Fiadeiro, Citation1986; Shibusawa, Citation1988) were analyzed using EquationEquation (5)
(5)
(5) .
(5)
(5)
The values of a, t and k refer to a coefficient depending on exhaustion, the dyeing time, and the adsorption rate constant, respectively. The values of the adsorption rate constant (k) at different temperatures were determined from the slopes and the intercepts of a plot of versus ln t as shown in . It was found that the adsorption rate constants (k) at 30, 60, and 80 °C increased with increasing dyeing temperatures as listed in . The mobility of the dye molecules would be expected to increase at higher temperatures resulting in the possibility of more dye molecule interactions with active sites at the PALF surface.
Table 3. Dye adsorption rate constant (k) at different temperatures of PALF dyed with berberine dye with an initial dye concentration of 250 mg l−1 at pH 9.0.
3.5. Adsorption isotherm study
Langmuir and Freundlich isotherm models were used in this study for analyzing the dye adsorption information at equilibrium. The obtained coefficients of determination (R2) values from the equations for these models were used as criteria to select the best fit linear theoretical isotherm. The Langmuir isotherm applies to monolayer adsorption on a surface with a finite number of identical sites (Hussain, Citation2018). This isotherm can be expressed as EquationEquation (6)(6)
(6) , from which the linear form is obtained (EquationEquation 7
(7)
(7) ).
(6)
(6)
(7)
(7)
In EquationEquation (6)(6)
(6) , qmax (mg g PALF−1) refers to the maximum adsorption capacity of dye molecules to form a complete monolayer on the fibre surface. Ce (mg L−1) is the amount of dye in the equilibrium or the equilibrium dye concentration, while qe (mg g PALF−1) is the amount of dye adsorbed per unit weight of fibre at equilibrium and b (L mg−1) is the Langmuir constant value related to the adsorption energy. The values of qmax and b can then be readily determined from the slopes and intercepts of the linear plot of Ce/qe vs Ce.
Another empirical isotherm provided by the Freundlich equation can be used to describe adsorption data. The Freundlich isotherm, which is normally used to describe the multilayer adsorption on the heterogeneous surface of adsorbents (Sá, Abreu, Machado, & Moura, Citation2017; Wang & Guo, Citation2020), can be expressed by EquationEquation (8)(8)
(8) .
(8)
(8)
From this, the linear form of the Freundlich isotherm can be derived as EquationEquation (9)(9)
(9) :
(9)
(9)
In EquationEquation (9)(9)
(9) , KF (mg g PALF−1) is the Freundlich constant or maximum adsorption capacity and 1/n is the adsorption intensity. The linear form of the Freundlich isotherm provides the constants KF and 1/n. Therefore, KF and 1/n can be determined from intercepts and slopes from the linear plot of log qe against log Ce at different temperatures. In the Freundlich model, the adsorbate can be easily adsorbed onto the adsorbent surface when 1/n is less than 1. Values of 1/n between 0 and 1 represent a chemisorption process. However, for adsorption occurring on a more heterogeneous surface, the 1/n value is close to 0.
Langmuir adsorption isotherms of berberine dye on PALF at 30, 60, and 80 °C (contact time 60 min and pH 9.0 in each case) are shown in . In this experiment, the linear forms of both the Langmuir and Freundlich isotherms were used to fit the equilibrium data of dye adsorption on PALF. Based on the correlation coefficient (R2) listed in , the Langmuir isotherm showed a better fit to the adsorption data with a high correlation coefficient (R2 > 0.99) than that of the Freundlich isotherm (R2 < 0.97) at the dyeing temperatures used. It was found that lower temperatures led to higher dye uptake over the temperature range of 30 − 80 °C, indicative of berberine adsorption on PALF being an exothermic process. In dyeing adsorption according to the Langmuir model, the berberine dye would be adsorbed at a limited number of specific sites within PALF. All of these adsorption sites on the PALF surface are energetically equivalent (homogeneous) and there would be no interactions between adjacent adsorbed dye molecules. Thus when a dye molecule occupied an adsorption site on the fibre, it implies that the site becomes incapable of any further adsorption.
Figure 7. (a) Langmuir adsorption isotherm of berberine dye on PALF at 30, 60, and 80 °C and (b) linear plot of Ce/qe vs Ce from the Langmuir isotherm of berberine dye on PALF at 30, 60, and 80 °C (contact time 60 min, pH 9.0, and initial concentration dye solution of 10–600 mg L−1).

Table 4. Constants and parameters of the Langmuir and Freundlich isotherms for adsorption of berberine dye on PALF (contact time 60 min, pH 9.0, and initial dye concentrations of 10–600 mg L−1).
The linear plot of Ce/qe vs Ce based on the Langmuir isotherm, as seen in , enabled the values of qmax and b () to be calculated from the slopes and intercepts of the different straight lines, representing the different temperatures. Furthermore, the qmax values clearly decreased with increasing temperature. The decrease of the amount of the dye adsorbed per gram of fibre with increasing temperature indicated that the adsorption of berberine dye onto the PALF was exothermically controlled. The (dye + PALF ⇋ dye⋅PALF) equilibrium would thus be shifted to the left at higher temperatures with a lower dye adsorption outcome. The b values indicated that PALF has a maximum affinity for berberine at 30 °C.
3.6. Thermodynamic parameters
To assess energy and entropy changes implicit in the berberine adsorption process, the fundamental thermodynamic parameters of enthalpy change (ΔHo) and entropy change (ΔSo) of dyeing at different temperatures for the adsorption of berberine dye on PALF were determined from EquationEquation (10)(10)
(10) :
(10)
(10)
where Ce (mg mL−1) is the amount of dye in the equilibrium or the equilibrium dye concentration, qe (mg g PALF−1) is the amount of dye adsorbed per unit weight of fibre at equilibrium, R (8.314 J mol−1 K−1) is the gas constant and T (K) is the temperature used for the PALF dyeing. The values of ΔH° and ΔS° are calculated from the slopes and intercepts of the linear graphs of ln (qe/Ce) vs 1/T (y = 2049.4x − 2.34, R2 = 0.999). These values were then employed in EquationEquation (11)
(11)
(11) to calculate the value of the standard affinity of dyeing (Δµo).
(11)
(11)
The enthalpy change (ΔH°) and entropy change (ΔS°) values of dyeing were −17.0 kJ mol−1 and −19.4 J mol−1 K−1 with a high correlation coefficient (R2 > 0.99), respectively. The enthalpy change (ΔH°) reflects the amount of thermal energy released when the berberine dye molecules were adsorbed on PALF (Ke et al., Citation2008), while the negative value of ΔH° indicated that the berberine dye molecules strongly interacted with the fibre. The negative entropy change (ΔS°) of dyeing revealed that the adsorbed dye molecules became more constrained within the fibres than in the dye solution phase, as would be expected on dye binding.
The negative standard affinity (Δµo) values at the different temperatures of 30, 60, and 80 °C are shown in . These values pointed to the spontaneous nature of the PALF dyeing process. Additionally, the decrease in the negative values of Δµo with an increase in temperature was consistent with the adsorption being more favorable at lower temperature. It can be concluded that increasing the temperature led to lower affinity and less dye being adsorbed at equilibrium.
Table 5. The thermodynamic parameters for adsorption of berberine dye on PALF (contact time 60 min, pH 9.0, and initial dye concentration of 10–600 mg L−1).
3.7. Color measurements and ATIR-FTIR spectrum of PALF dyed with berberine dye
The CIE L*a*b* values of the dyed PALF samples at the different temperatures (30, 60, and 80 °C) are reported in . The results revealed that all dyed PALF samples showed a shade of yellow with different L*, a*, and b* values. At higher dyeing temperatures, the yellowness (b*) values decreased when the temperature was increased from 30 to 80 °C, consistent with more adsorbed dye molecules at lower temperature.
Table 6. Color measurements of the dyed PALF samples at different temperatures (initial dye concentration of 250 mg L−1, contact time 60 min).
In addition, the ATR-FTIR spectra for PALF before and after dyeing with berberine dye are shown in . A new absorption peak at 1604 cm−1 of the PALF dyed with berberine was assigned to the iminium (C = N+) bond of berberine dye (Ghaffarzadegan, Khoee, & Rezazadeh, Citation2020) confirming PALF interacted with berberine dye in the dyeing process.
4. Conclusion
An efficient method was developed for the preparation of PALF which involved treatment with a NaOH solution (5% w/v) with simultaneous ultrasonication for 20 min. From FESEM and color measurement studies, this method was shown to be effective in removing the non-cellulosic substances from the fibre surface within a short time compared with PALF treated with a NaOH solution (5% w/v) alone for 24 h using the traditional method.
Optimal conditions for dyeing of PALF with the natural dye berberine were determined and it was found that the adsorption capacity was dependent on the contact time and pH of the dye solution. The optimum dye uptake occurred at pH 9.0 (after 60 min), in line with the computational modeling results. The adsorption kinetic of PALF dyeing with berberine was also investigated in this work. In the initial stage of dye adsorption, the dye adsorption rate constant (k) increased with increasing temperature from 30 to 80 °C consistent with the dye uptake being a kinetically controlled process. On the other hand, the decrease in dye uptake at higher temperatures after equilibrium had been reached then reflected exothermic control.
The experimental data for the adsorption isotherm of berberine dye on PALF fitted well with the Langmuir model (R2 > 0.99). The key thermodynamic parameters for the adsorption process, namely the enthalpy change (ΔH°), entropy change (ΔS°) and the standard affinity (Δµo) of dyeing were also determined. The enthalpy change (ΔH°) and entropy change (ΔS°) values of dyeing were −17.0 kJ mol−1 and −19.4 J mol−1 K−1, respectively. The negative values found for the enthalpy change (ΔH°) and the entropy change (ΔS°) of dyeing were consistent with the adsorption being an exothermic and favourable process. The negative standard affinity (Δµo) values at the different temperatures of 30, 60, and 80 °C were −11.2, −10.6, and −10.2 kJ mol−1, respectively. This was indicative of a spontaneous dye adsorption process in the temperature range of 30 - 80 °C. From the low enthalpy change on dyeing and the quick time to reach equilibrium, coupled with the interactions predicted from the DFT studies, it is considered likely that the adsorption of berberine on PALF is a physisorption process mediated by non-covalent intermolecular interactions (ion-ion interactions and hydrogen bonds) and not from chemical bond formation.
This research has shown that PALF is amenable to direct dyeing by the natural dye berberine and as such could provide a further eco-friendly avenue for the development of the fibre. Additional studies will be required in the future, however, to assess the degree of resistance to fading on exposure to different external influences.
Disclosure statement
No potential conflict of interest was reported by the authors.
Additional information
Funding
References
- Amin, A. N. M., Ruznan, W. S., Suhaimi, S. A., Yusuf, J. M., Kadir, M. I. A., & Nor, M. A. M. (2021). Washfastness properties of dyed pineapple leaf fibre using different dyeing techniques. Journal of Academia, 9(1), 66–72.
- Asim, M., Abdan, K., Jawaid, M., Nasir, M., Dashtizadeh, Z., Ishak, M. R., & Enamul, H. M. (2015). A review on pineapple leaves fibre and its composites. International Journal of Polymer Science, 2015, 1–16. doi:10.1155/2015/950567
- Bengtsson, M., Gatenholm, P., & Oksman, K. (2005). The effect of crosslinking on the properties of polyethylene/wood flour composites. Composites Science and Technology, 65(10), 1468–1479. doi:10.1016/j.compscitech.2004.12.050
- Burkinshaw, S. M. (2016). Physico-chemical aspects of textile colouration. Chichester, UK: John Wiley & Sons. doi:10.1002/9781118725658
- Cegarra, J., Puente, P., & Fiadeiro, J. M. (1986). Kinetics of dyeing acrylic fibres with cationic dyes in the presence of polyethoxylated amines. Journal of the Society of Dyers and Colourists, 102(9), 274–278. doi:10.1111/j.1478-4408.1986.tb01080.x
- Che, J., & Yang, X. (2022). A recent (2009–2021) perspective on sustainable color and textile coloration using natural plant resources. Heliyon, 8(10), e10979. doi:10.1016/j.helyon.2022.e10979
- Cherian, B. M., Leao, A. L., Souza, S. F., Thomas, S., Pothan, A., & Kottaisamy, M. (2010). Isolation of nanocellulose from pineapple leaf fibres by steam explosion. Carbohydrate Polymers, 81(3), 720–725. doi:10.1016/j.carbpol.2010.03.046
- Das, B. K., Chakravarty, A. C., Sinha, M. K., & Ghosh, S. K. (1978). Use of polyethylene monofilament in spinning core yarn of jute. Man Made Textile in India, 21(9), 494–498.
- de Queiroz, R. S., da Silva, A. P. V., Broega, A. C. L., & Souto, A. P. G. V. (2020). New Brazilian pineapple leaf fibers for textile application: Cottonization and dyeing performance. SN Applied Sciences, 2(1), 72. doi:10.1007/s42452-019-1855-8
- Delley, B. (1990). An all‐electron numerical method for solving the local density functional for polyatomic molecules. The Journal of Chemical Physics, 92(1), 508–517. doi:10.1063/1.458452
- Delley, B. (2000). From molecules to solids with the DMol3 approach. The Journal of Chemical Physics, 113(18), 7756–7764. doi:10.1063/1.1316015
- Dostál, J., Man, S., Sečkářová, P. n., Hulová, D., Nečas, M., Potáček, M., Toušek, J. r., Dommisse, R., Van Dongen, W., & Marek, R. (2004). Berberine and coptisine free bases. Journal of Molecular Structure, 687(1), 135–142. doi:10.1016/j.molstruc.2003.09.018
- Du, H., Xu, L., Yue, M., Xu, F., & Wang, Y. (2022). Studies on crystal structures, optical, solubility and dyeing properties of two new crystalline dye salts based on berberine with aromatic carboxylic acid. Journal of Molecular Structure, 1260, 132856. doi:10.1016/j.molstruc.2022.132856
- George, J., Sreekala, M. S., & Thomas, S. (2001). A review on interface modification and characterization of natural fiber reinforced plastic composites. Polymer Engineering & Science, 41(9), 1471–1485. doi:10.1002/pen.10846
- Ghaffarzadegan, R., Khoee, S., & Rezazadeh, S. (2020). Fabrication, characterization and optimization of berberine-loaded PLA nanoparticles using coaxial electrospray for sustained drug release. Daru: Journal of Faculty of Pharmacy, Tehran University of Medical Sciences, 28(1), 237–252. doi:10.1007/s40199-020-00335-y
- Gholampour, A., & Ozbakkaloglu, T. (2020). A review of natural fiber composites: Properties, modification and processing techniques, characterization, applications. Journal of Materials Science, 55(3), 829–892. doi:10.1007/s10853-019-03990-y
- Grimme, S. (2006). Semiempirical GGA-type density functional constructed with a long-range dispersion correction. Journal of Computational Chemistry, 27(15), 1787–1799. doi:10.1002/jcc.20495
- Gupta, V. (2019). Fundamentals of natural dyes and its application on textile substrates. Chemistry and Technology of Natural and Synthetic Dyes and Pigments 1-32. doi:10.5772/intechopen.89964
- Haji, A. (2010). Functional dyeing of wool with natural dye extracted from berberis vulgaris wood and Rumex hymenosepolus root as biomordant. Iranian Journal of Chemistry and Chemical Engineering, 29(3), 55–60. doi:10.30492/ijcce.2010.6513
- Haji, A. (2013). Eco-friendly dyeing and antibacterial treatment of cotton. Cellulose Chemistry and Technology, 47(3–4), 303–308.
- Hirshfeld, F. L. (1977). Bonded-atom fragments for describing molecular charge densities. Theoretica Chimica Acta, 44(2), 129–138. doi:10.1007/BF00549096
- Hussain, C. M. (2018). Nanomaterials in chromatography, current trends in chromatographic research technology and techniques, mechanism of adsorption on nanomaterials (1st ed., pp. 89–115). Cambridge, UK: Elsevier Science Publishing.
- Jeffs, P. W. (1967). Chapter 2: The protoberberine alkaloids. In The alkaloids: Chemistry and physiology (pp. 41–115). Academic Press. doi:10.1016/S1876-0813(08)60198-0
- Jose, S., Salim, R., & Ammayappan, L. (2016). An overview on production, properties, and value addition of pineapple leaf fibers (PALF). Journal of Natural Fibers, 13(3), 362–373. doi:10.1080/15440478.2015.1029194
- Judd, D. B., & Wyszecki, G. (1975). Colour in business, science and industry (3rd ed.). New York, USA: John Wiley & Sons.
- Kamel, M. M., & Mashaly, H. (2013). Coloration of fibrin fabric with berberine natural dye with ultrasonic technique using ZnO nano powder as light fastness improving. World Applied Sciences Journal, 26(8), 994–1001. doi:10.5829/idosi.wasj.2013.26.08.13545
- Ke, G. Z., Xu, W. L., & Yu, W. D. (2008). Thermodynamic and kinetic studies of adsorption of berberine on silk. Indian Journal of Fibre & Textile Research, 33(2), 185–188. http://nopr.niscair.res.in/handle/123456789/1616.
- Khalil, H. P. S. A., Alwani, M. S., & Omar, A. K. M. (2006). Chemical composition, anatomy, lignin distribution, and cell wall structure of Malaysian plant waste fibers. BioResources, 1(2), 220–232. doi:10.15376/biores.1.2.220-232
- Kim, T. K., Yoon, S. H., & Son, Y. A. (2004). Effect of reactive anionic agent on dyeing of cellulosic fibers with a berberine colorant. Dyes and Pigments, 60(2), 121–127. doi:10.1016/S0143-7208(03)00147-5
- Kim, T. K., & Son, Y. A. (2005). Effect of reactive anionic agent on dyeing of cellulosic fibers with a berberine colorant – Part 2: Anionic agent treatment and antimicrobial activity of a berberine dyeing. Dyes and Pigments, 64(1), 85–89. doi:10.1016/j.dyepig.2004.04.007
- Kornilova, T., Glebov, V., Castañeda, R., & Timofeeva, T. V. (2022). A new pseudopolymorph of berberine chloride: Crystal structure and Hirshfeld surface analysis. Acta Crystallographica. Section E, Crystallographic Communications, 78(Pt 5), 468–472. doi:10.1107/S2056989022003309
- Liu, J., Lin, X., & Liang, H. (2019). Dyed fabrics modified via assembly with phytic acid/berberine for antibacterial, UV resistance, and self-cleaning applications. Journal of Engineered Fibers and Fabrics, 14, 155892501988897. doi:10.1177/1558925019888978
- Neag, M. A., Mocan, A., Echeverría, J., Pop, R. M., Bocsan, C. I., Crişan, G., & Buzoianu, A. D. (2018). Berberine: Botanical occurrence, traditional uses, extraction methods, and relevance in cardiovascular, metabolic, hepatic, and renal disorders. Frontiers in Pharmacology, 9, 557–557. doi:10.3389/fphar.2018.00557
- Nishiyama, Y., Langan, P., & Chanzy, H. (2002). Crystal structure and hydrogen-bonding system in cellulose Iβ from synchrotron X-ray and neutron fiber diffraction. Journal of the American Chemical Society, 124(31), 9074–9082. doi:10.1021/ja0257319
- Pargai, D., Jahan, S., & Gahlot, M. (2020). Functional properties of natural dyed textiles. Chemistry and Technology of Natural and Synthetic Dyes and Pigments 1-19. doi:10.5772/intechopen.88933
- Perdew, J. P., Burke, K., & Ernzerhof, M. (1996). Generalized gradient approximation made simple. Physical Review Letters, 77(18), 3865–3868. doi:10.1103/PhysRevLett.77.3865
- Py, C., Lacoeuilhe, J. J., & Teisson, C. (1987). The pineapple—cultivation and uses. Paris, France: G. P. Maisonneuve & Larose.
- Rahman, M. A. (2011). Study on modified pineapple leaf fiber. Journal of Textile and Apparel, Technology and Management, 7(2), 1–16.
- Ravikumar, K., Kim, S. H., & Son, Y. (2007). Design of experiments for the optimization and statistical analysis of berberine finishing of polyamide substrates. Dyes and Pigments, 75(2), 401–407. doi:10.1016/j.dyepig.2006.06.020
- Sá, A., Abreu, A. S., Machado, A. V., & Moura, I. (2017). Polymeric materials for metal sorption from hydric resources. In Water Purification (pp. 289–322). Academic Press. doi:10.1016/B978-0-12-804300-4.00008-3
- Shamsul Alam, M. D., Arifuzzaman Khan, M. G., & Abdur Razzaque, M. S. (2009). Estimation of main constituents of ananus comosus (Pineapple) leaf fiber and its photo-oxidative degradation. Journal of Natural Fibers, 6(2), 138–150. doi:10.1080/15440470902955369
- Shibusawa, T. (1988). The mathematical expression of rate constants in empirical rate of dyeing equations in disperse dyeing. Journal of the Society of Dyers and Colourists, 104(1), 28–33. doi:10.1111/j.1478-4408.1988.tb01132.x
- Sricharussin, W., Ree-Iam, P., Phanomchoeng, W., & Poolperm, S. (2009). Effect of enzymatic treatment on the dyeing of pineapple leaf fibres with natural dyes. ScienceAsia, 35(1), 31–36. doi:10.2306/scienceasia1513-1874.2009.35.031
- Tangtubtim, S., & Saikrasun, S. (2019). Adsorption behavior of polyethyleneimine-carbamate linked pineapple leaf fiber for Cr(VI) removal. Applied Surface Science, 467–468, 596–607. doi:10.1016/j.apsusc.2018.10.204
- Todkar, S. S., & Patil, A. S. (2019). Review on mechanical properties evaluation of pineapple leaf fibre (PALF) reinforced polymer composites. Composites Part B: Engineering, 174, 106927. doi:10.1016/j.compositesb.2019.106927
- Uddin, A. M., Rahman, M. M., Haque, A. M. N. A., Smriti, A. S., Datta, E., Farzana, N., … Sayem, M. S. A. (2022). Textile colouration with natural colourants: A review. Journal of Cleaner Production, 349, 131489. doi:10.1016/j.jclepro.2022.131489
- Wang, J., & Guo, X. (2020). Adsorption isotherm models: Classification, physical meaning, application and solving method. Chemosphere, 258, 127279. doi:10.1016/j.chemosphere.2020.127279
- Wiberg, K. B. (1986). Ab initio molecular orbital theory. Journal of Computational Chemistry, 7(3), 379–379. doi:10.1002/jcc.540070314