Abstract
In reproduction, inflammatory processes play important roles in the development of many pregnancy complications such as preterm labor/birth, recurrent pregnancy loss, recurrent implantation failure, and preeclampsia. Inflammation can be initiated by both microbial and non-microbial causes. Bacterial infection in the feto-maternal interface and uterus can provoke preterm labor/birth, miscarriage, and chronic endometritis. By contrast, inflammation without infection, or ‘sterile inflammation,’ can also lead to many kinds of complications, such as preterm labor/birth, miscarriage, or preeclampsia. Aberrant inflammation is facilitated by immune cells such as macrophages, dendritic cells, natural killer cells, and invariant natural killer T cells. In addition, cytokines, chemokines, and several kinds of inflammatory mediators are involved. On the other hand, appropriate inflammation is required for a successful offspring during the progression of the entire pregnancy. Herein, we discuss the relation between pregnancy and inflammation with immunological alterations. Understanding the role of inflammation in complications during pregnancy may establish new perspectives of the progress of normal pregnancy as well as treatments during pregnancy complications.
1. Introduction
Inflammation is part of the host defensive response against microbial infection. It encompasses the phagocytic function of macrophages and neutrophils during bacterial infection; recognition, activation, and cytotoxic activity of T cells and B cells for viral infection; and the critical roles of these immune cells to eliminate microbes. However, the concept of inflammation has been widely recognized as an immune response regardless of pathogen infection. Aberrant, excessive, or suppressive inflammation is observed in many pathologies such as cancer, autoimmune diseases, and metabolic disease. Inflammation in the absence of infection is known as ‘sterile inflammation,’ [Citation1] and immune cells (innate and acquired immune cells) and their interactions are responsible for this inflammation.
In reproduction, many complications during pregnancy are associated with inadequate inflammation. Microbial infection is an important agent for the development of complications during pregnancy; however, sometimes no obvious pathogens are detected in these complications. These findings indicate that the development of complications during pregnancy can be induced by either microbial or non-microbial causes [Citation2,Citation3]. Acute chorioamnionitis (aCAM) is thought to be of particular importance for the development of preterm labor/birth; however, recent studies have reported that many clinical cases of preterm labor/birth occur without obvious infection. Indeed, antibiotics do not always provide benefits in treating preterm labor/birth [Citation4]. Preeclampsia, which is characterized by hypertension, proteinuria, and edema during pregnancy, is associated with an excessive maternal inflammatory response without microbial infection [Citation5]. In addition, chronic endometritis is sometimes diagnosed in patients with infertility, and antibiotics can improve the implantation rate [Citation6–8]. Interestingly, inflammation can conversely have beneficial effects on pregnancy progress. Adequate inflammation is thought to be required during the entire pregnancy progress, such as for ovulation [Citation9], implantation of the embryo to the maternal endometrium [Citation10,Citation11]. In addition, inflammatory mediators have a critical role in human parturition [Citation12].
In this review, we focus on pregnancy complications and characteristic features in terms of inflammation induced by both microbial and non-microbial causes with a delicate balance of immune function.
1.1. Acute chorioamnionitis
Preterm labor/birth is considered the most common complication during pregnancy, which sometimes determines neonatal morbidity and mortality [Citation13]. The presence of acute chorioamnionitis (aCAM), which is characterized by neutrophil infiltration into the chorioamniotic membranes, is usually considered related to bacterial infection and is often observed in the placenta with preterm birth. Previous studies reported that bacterial infection provokes the production of pro-inflammatory cytokines (e.g., interleukin (IL)-1β, tumor necrosis factor (TNF)-α, and IL-8), inflammatory mediators (e.g., platelet-activating factor, prostaglandins, leukotrienes, reactive oxygen species, and nitric oxide, NO), and chemokines from macrophages and other host cells during pregnancy [Citation14]. IL-1 production of the decidua can facilitate prostaglandin production from the decidua and amnion and is an important factor for triggering labor during intrauterine infection [Citation15]. In addition, IL-1 can induce myometrial contractions leading to preterm labor [Citation16]. TNF-α produced from the decidua in response to bacterial infection also can facilitate prostaglandin production from the decidua, amnion, and myometrium [Citation17]. TNF-α and IL-8 can induce the production of matrix metalloproteinases (MMPs), which facilitate cervical ripening and rupture of the membranes (amniorrhexis) in human pregnancy [Citation18–24]. Thus, aCAM related to bacterial infection is an important finding for the initiation of preterm cervical ripening, labor, and rupture of the membranes.
Preterm birth is classified into the following three categories according to gestational age at delivery: extremely preterm birth (less than 28+0 weeks), moderately preterm birth (28+0–33+6 weeks), and late preterm birth (34+0–36+6 weeks) [Citation13]. The histological findings of aCAM are involved in about 20%–60% of placenta with preterm birth [Citation25]. In particular, the prevalence of aCAM becomes higher with earlier gestational ages [Citation25], and the onset of extremely preterm birth is mainly caused by aCAM [Citation26,Citation27]. Ascending or hematogenous bacterial infection can initiate the onset of preterm birth and rupture of the membranes [Citation3,Citation28,Citation29]. The major microorganisms that result in intrauterine infection are Ureaplasma urealyticum [Citation30,Citation31], Mycoplasma hominis [Citation32], Streptococcus agalactiae, Escherichia coli, Fusobacterium, and Gardnerella vaginalis [Citation31]. Thus, infection with one or more of these microorganisms are related to histological aCAM. However, recent studies have revealed that many clinical cases of aCAM in the placenta and excessive cytokines in the amniotic fluid do not always involve apparent microorganism infection [Citation33–35]. Thus, aCAM, which presents as excessive inflammation with neutrophil accumulation, can be induced by microbial or non-microbial causes (see ‘Sterile inflammation’ section); bacterial infection is not always associated with aCAM [Citation35].
More importantly, excessive progression of infection or inflammation in uterine fluid can provoke neonatal problems such as respiratory distress, necrotizing enterocolitis, neurodevelopmental disorder, and psychiatric disorders [Citation36–39]. Indeed, histological aCAM is diagnosed after, not before, delivery. Therefore, assessment of the excessive cytokine milieu and the level of aCAM during pregnancy is strongly required for determination of the appropriate delivery mode and timing. Recent studies have suggested that the level of IL-8 in the amniotic fluid is a useful marker for the prediction of histological aCAM [Citation40], and the accurate detection of intra-amniotic microbes by amniocentesis and highly sensitive PCR can be helpful to determine appropriate antibiotic therapy for the patient with preterm labor [Citation41]. Collectively, aCAM and excessive intrauterine inflammation induced by microbial or non-microbial causes sometimes increases the incidence of serious disorders in neonates.
1.2. Chronic chorioamnionitis
Chorioamnionitis (CAM) is divided into two categories: aCAM and chronic CAM (cCAM). The former is associated with neutrophil infiltration into the chorioamniotic membranes as mentioned above and is traditionally called simply ‘CAM’ [Citation32,Citation42,Citation43]. The latter is characterized by the infiltration of lymphocytes, plasma cells, and macrophages, and it has been defined only in the last decade [Citation44]. A recent report suggested that aCAM is the most common cause of extremely preterm birth, whereas cCAM is the most common cause of late preterm birth [Citation45]. Another report showed that 39% of cCAM cases were observed in preterm labor/birth [Citation46]. In general, aCAM is mainly caused by infection; however, the etiology of cCAM not only involves infection but also other factors. Previous reports indicated the presence of intrauterine viral infections such as adenovirus, cytomegalovirus, parvovirus, and enterovirus [Citation47,Citation48]; nevertheless, specific infectious etiology was not detected in cCAM [Citation49]. In addition, another report suggested that cCAM may be have non-infectious, immunologic causes [Citation50]. Arenas-Hernandez et al. suggested that effector and activated T cells elicit pathological inflammation on the feto-maternal interface without neutrophil infiltration, and this inflammation causes preterm birth [Citation51].
Recently, the relationship between cCAM and preterm labor/birth related to sterile inflammation has been discussed [Citation44–46]. In addition, innate immune cells such as invariant natural killer (iNKT) and dendritic cells (DCs) have been studied in the context of inflammation at the feto-maternal interface. The iNKT cell is a subset of innate lymphocytes that recognizes lipid or glycosphingolipid antigens on nonpolymorphic CD1 molecules [Citation52–54]. The population of iNKT cells in human peripheral blood is very low [Citation55]; however, iNKT cells have multiple effector functions and can rapidly produce large numbers of many types of cytokines [Citation56,Citation57]. Indeed, iNKT cells are present in the decidua in a small but robust proportion in humans and mice [Citation58–61]. DCs are one of the key players for antigen recognition and presentation to T cells, and they are involved in maintaining healthy pregnancy in mice and humans [Citation11,Citation62]. After recognition of antigens by pattern recognition receptors (PRRs) on DCs, DCs regulate T cells via direct interaction between the T-cell receptor (TCR) and the major histocompatibility complex (MHC) or via indirect pathways of cytokine networks. In our recent study, accumulation of iNKT cells and DCs were observed in the decidua of late preterm births without aCAM in humans, and there were functional differences between the DCs obtained from decidua with aCAM and those obtained from decidua without aCAM [Citation63]. Another study described that activated iNKT-like cells are more abundant in the decidua basalis during late preterm birth, where the aCAM abundance is low, than in those of term birth [Citation58].
Collectively, it is possible that these innate cells play important roles in the onset of preterm labor/birth with cCAM or sterile inflammation (see the ‘Sterile inflammation’ section). Accurate assessment of aCAM and cCAM, including the kinetics of innate and effector cells, may be required for understanding their mechanistic involvement in preterm labor/birth.
1.3. Sterile inflammation
1.3.1. Alarmins and PRRs
Inflammation processes are essential for pregnancy: in particular, implantation, protection against exogenous pathogens, and parturition. However, excessive inflammation can trigger many kinds of complications related to pregnancy such as infertility [Citation64], recurrent pregnancy loss [Citation65,Citation66], premature delivery [Citation33,Citation34], and preeclampsia [Citation5]. Recently, ‘sterile inflammation’, or inflammation without apparent pathogenic infection, has been implicated in many kinds of diseases such as cancer [Citation67], diabetic kidney disease [Citation68], cardiovascular disease [Citation69,Citation70], pulmonary disorders [Citation71], and environmental microparticles [Citation1,Citation72,Citation73]. Sterile inflammation is induced by endogenous molecules such as high mobility group box 1 (HMGB1), IL-α, IL-33, heat shock protein (HSP), and S100 protein that are released by tissue and cellular damage in the absence of infection. These molecules are collectively called alarmins. PRRs recognize these alarmins by TLRs, NOD-like receptors (NLRs), C-type lectin receptors (CLRs), and receptors for advanced glycation end-products (RAGEs) on antigen presenting cells (APCs), macrophages, and DCs [Citation74,Citation75]. After the immunostimulatory activities of APCs are upregulated by alarmins, these cells can directly and indirectly stimulate other immune cells, T cells, neutrophils, NK cells, and iNKT cells, leading to the production of pro-inflammatory cytokines and chemokines [Citation1]. Sterile inflammation can contribute to the onset of parturition and other obstetric complications: implantation failure, miscarriage, preterm labor/birth, fetal growth restriction, and preeclampsia during pregnancy (reviewed in [Citation2]). Indeed, we often experience many clinical cases of preeclampsia, fetal growth restriction, and preterm labor/birth without apparent pathogen infection. The TLRs NLRs, and CLRs are expressed on not only APCs but also in the decidua, placenta, membranes, and myometrium [Citation74–76]. Therefore, the feto-maternal interface can also be a direct target for the alarmins.
1.3.2. Preterm labor/birth
Recently, several studies have been reported for preterm labor/birth associated with sterile inflammation. Yoneda et al. reported that about 70% of patients with preterm labor contained microbe-negative amniotic fluid [Citation41]. In addition, sterile inflammation in the amniotic fluid is more frequent than microbial-associated inflammation in patients with preterm labor [Citation35]. In this study, patients with high concentrations of HMGB1 in their amniotic fluid delivered earlier than those with low concentrations of HMGB1. HMGB1 ubiquitously exists in the nucleus to maintain nucleosome stability and is released extracellularly in response to tissue damage and cell death. Extracellular HMGB1 binds to multiple receptors including toll-like receptor (TLR)2, TLR4, TLR9, RAGE, and CD24 [Citation77], and leads to the activation of nuclear factor-kappa B (NF-κB), extracellular signal-regulated kinase1/2, p38 mitogen-activated protein kinase, and Src-family kinases [Citation78]. Eventually, excessive inflammation is provoked. Another study revealed that HMGB1 level increased in patients with preterm labor [Citation79]. In our study for preterm birth without aCAM, we observed that the production of HMGB1 in viable APCs in the patients with preterm labor and/or rupture of membranes were significantly higher than in those without preterm labor and/or rupture of membrane (manuscript submitted). These results indicate that HMGB1 is a trigger or inducer of preterm birth without aCAM. In a murine model, intra-amniotic injection of HMGB1 facilitated preterm labor and birth [Citation80]. Thus, it is possible that HMGB1 prompts the onset of preterm labor/birth to some extent.
Cell-free fetal DNA (cffDNA) from placenta that migrates into the maternal system has also been studied for preterm labor/birth as an alarmin. This cffDNA was observed in maternal plasma and could be a predictive marker and a risk factor for preterm birth [Citation81–83].
IL-1 has critical roles in inflammation and infection and includes IL-1α and IL-1β [Citation84]. The production of IL-1β is strictly controlled via the inflammasome in response to endogenous and exogenous antigens (see the ‘Inflammasome’ section). By contrast, IL-1α is ubiquitously expressed in the cytoplasm, but during necrosis or apoptosis it is released extracellularly, where it functions as an alarmin [Citation85,Citation86]. Several studies have shown an increased level of IL-1α during preterm labor/birth. Levels of IL-1α in amniotic fluid with sterile intra-amniotic inflammation was significantly higher than in that without sterile intra-amniotic inflammation [Citation87]. Figueroa et al. showed that among patients undergoing amniocentesis who showed negative amniotic fluid culture, a statistical difference arose in IL-1α (and IL-6 and IL-8) concentrations but not IL-1β concentrations between patients who delivered within seven days compared with those that delivered after seven days of amniocentensis [Citation88]. These results indicate that IL-1α in the amniotic fluid may affect the development of inflammation leading to the onset of preterm labor/birth.
As mentioned above, alarmins may have critical roles in excessive inflammation and the onset of preterm birth; however, the mechanisms leading to their release is unclear. In fact, placental tissues including trophoblasts contain numerous alarmins, HMGB1, cffDNA, IL-1α, and uric acid, which can provoke the sterile inflammatory response [Citation2,Citation89–91]. Therefore, hypoxia from placental dysfunction, trauma, and abnormal maternal metabolism may facilitate the alarmins, and can then promote sterile inflammation (reviewed in [Citation2]).
Interestingly, some studies have pointed out that iNKT cells may contribute to the onset of preterm labor/birth in the absence of microbial-associated inflammation. iNKT cells can be activated indirectly or directly by APCs via IL-12 secretion and CD1d-invariant TCR interaction and exist at the feto-maternal interface [Citation92–94]. Louis et al. suggested that the amounts of iNKT-like cells in the decidua obtained from patients with late preterm birth are abundant compared with those undergoing term birth [Citation58]. In addition, rosiglitazone, a peroxisome proliferator-activated receptor γ agonist, prevents murine preterm birth induced by α-galactosylceramide (α-GalCer), which is one of the glycolipid antigens originally identified in marine sponges and known to be specific activator of iNKT cells [Citation58]. In our previous study for patients with preterm birth, we observed that the number of iNKT cells in decidua without aCAM was higher than in those with aCAM [Citation63]. In addition, Rinaldi et al. observed no statistical differences in the number of iNKT cells between preterm birth with labor and preterm birth without labor; however, CD1d expression, which is recognized by iNKT cells, is increased in preterm birth with labor [Citation95]. In our recent study for preterm birth without aCAM, expression of CD1d, TLR4, and RAGE in preterm birth with signs of parturition (labor and/or rupture of the membranes) were higher than those in preterm birth without parturition signs (manuscript submitted). Collectively, alarmins, innate immune cells such as APCs and iNKT cells, and the interaction of these cells may be involved in inducing preterm labor/birth related to sterile inflammation.
1.3.3. Miscarriage and infertility
Sterile inflammation and innate immune cells also have important roles in the onset of miscarriage and infertility. In particular, these complications are thought to be linked to the function of DCs. In humans, DCs consist of plasmacytoid DCs, monocyte-derived DC, and conventional (classical) DC (cDC) subtypes [Citation96–98]. The cDC type can be further divided into two major subsets: cDC1 and cDC2. The cDC1 subset has the capacity to establish T helper (Th)1 polarization [Citation99], and the cDC2 subset can induce Th2 polarization [Citation100–102]. In mice, cDCs are also divided into cDC1 and cDC2 subsets [Citation100,Citation103]. Similarly to humans, mouse cDC1s can activate Th1 cells, and cDC2 cells can induce Th2 dominance. DCs contribute to successful pregnancy in both mice and humans [Citation11,Citation62]. In mice, depletion of uterine DCs induced implantation failure and embryo resorption [Citation104]. DCs entrapped in the decidua can establish tolerance for the embryo [Citation105]. Our study showed that the depletion of the cDC2 subset during pregnancy induced murine miscarriage without infection [Citation106]. We also examined the murine pregnancy in mice treated with α-GalCer, which is a specific activator of iNKT cells, as an abortion model induced by sterile inflammation. The administration of α-GalCer provoked murine miscarriage with the accumulation of cDC1 as well as activated iNKT cells [Citation107]. Furthermore, adoptive transfer of DC1s co-cultured with α-GalCer into pregnant mice, and the adoptive transfer of iNKT cells after intrperitoneal administration of α-GalCer into iNKT-deficient mice also induced miscarriage [Citation108]. These results indicate the activated innate immune cells DCs and iNKT cells directly lead to miscarriage without microbial-associated inflammation. Interestingly, miscarriage and preterm birth induced by α-GalCer were ameliorated by treatment with rosiglitazone [Citation58] and Tokishakuyakusan, a traditional Japanese medicine [Citation109]. Innate immune cells, DCs, and iNKT cells are therefore associated with not only preterm both but also miscarriage in the absence of infection.
Alarmins are also implicated in miscarriage and implantation failure. HMGB1 level decreased on the day of implantation in pregnant rats, and administration of HMGB1 on day 3 post-coitum induced pregnancy loss [Citation110]. In humans, the interaction between HMGB1 and its receptor RAGE facilitated inflammation where monocyte migration occurred via elevated production of cytokines and chemokines such as IL-6, IL-8, and CCL2 in the first trimester [Citation111]. Recent observations revealed a relation between S100 protein and pregnancy loss. Nair et al. reported that the systemic level of S100A8 protein was elevated in patients with early pregnancy loss [Citation112]. This indicates that the S100A8 protein may be useful as a prediction marker for early pregnancy loss. For inflammation processes in early pregnancy, levels of IL-12, IL-18, IFN-γ, intracellular adhesion molecule-1, leukemic inhibitory factor, and migration inhibitory factor were abundant in endometrial cells and blood samples during the mid-secretory phase in patients with recurrent pregnancy loss compared with those in fertility; therefore, the inflammatory response is associated with recurrent pregnancy loss [Citation113]. Namely, inflammation related to alarmins may be associated with the onset of miscarriage and implantation failure without microbial-associated infection.
Aspirin, low-molecular weight heparin (LMWH), and the combination of these drugs are considered to be beneficial therapies for recurrent pregnancy loss and recurrent implantation failure. It has been reported that these treatments have not both an anticoagulant effect and also an anti-inflammatory effect [Citation114]. In addition, LMWH blocks HMGB1-RAGE interaction and demonstrates the anti-inflammatory effects on the placenta (see ‘Preeclampsia’ section) [Citation115,Citation116]. Recently, immunomodulatory drugs including steroids [Citation117,Citation118], intravenous immnunoglobulins [Citation119], TNF-α antagonist [Citation120], granulocyte colony-stimulating factor (G-CSF) agonist [Citation121], and intravenous intralipids [Citation122] also have been attempted for treatment of recurrent pregnancy loss and recurrent implantation failure (reviewed in [Citation123]). The effects of these treatments are controversial [Citation124].
Elevated levels of alarmins released before pregnancy increase the risk for infertility and recurrent pregnancy loss; the latter can be caused by HMGB1 gene polymorphisms affecting gene expression in chorionic villi [Citation125]. Our recent findings also show that HMGB1 levels are higher in non-immune cells and APCs obtained from endometrial cysts than those obtained from non-endometrial cysts (manuscript in preparation). A recent report suggested IL-33 is found in cystic fluid of ovarian tumors and is linked to the pathogenesis of endometriosis and infertility [Citation126]. These findings indicate that the increased level of alermin releasing in pre-pregnancy might be the risk of infertility and recurrent pregnancy loss.
1.3.4. Preeclampsia
Preeclampsia is characterized by hypertension, proteinuria, and edema during pregnancy and sometimes determines maternal and fetal mortality. Preeclampsia is associated with an excessive maternal inflammatory response [Citation5]. Although the pathogenesis of preeclampsia had been unknown for a long time, the two-stage model for the onset of preeclampsia has recently been proposed [Citation127,Citation128]. In the first trimester, extravillous trophoblast invasion to the maternal tissue is impaired, resulting in the restriction of placental vessel remodeling and insufficient placentation (first stage). In the insufficient placenta, oxidative stress, suppression of autophagy, and aberrant placental perfusion are induced. Subsequently, excessive inflammatory responses including endothelial disorders, apoptosis of trophoblasts, upregulation of soluble endoglin, soluble fms-like tyrosine kinase-1, and pro-inflammatory cytokines (e.g., IL-1β, IL-6, IL-12, and TNF-α) as well as downregulation of anti-inflammatory cytokines (e.g., IL-10) and placental growth factors occur (second stage) [Citation129–133]. Eventually, these abnormalities cause maternal hypertension and sometimes interfere the intrauterine fetal growth restriction associated with placental hypoplasia and disfunction [Citation134,Citation135]. These inflammatory responses occur without apparent microbial infection, and preeclampsia is considered a disease associated with sterile inflammation. The inflammatory milieu in the feto-maternal interface initiates the release of micro particles form trophoblasts and placental tissues into the maternal systemic circulation. Indeed, many kinds of alarmins, uric acid, HMGB1, cffDNA, S100 protein, HSP, and adenosine triphosphate exist in the placenta [Citation89]. Pradevand et al. reported that serum HMGB1 levels of patients with preeclampsia were significantly higher than those of non-pregnant women and healthy pregnant women [Citation136]. RAGEs, TLR2, and TLR4 are the major recognition receptors for HMGB1. HMGB1-RAGE signaling has been implicated in the pathogenesis of preeclampsia [Citation137], and the expression of TLR4 is increased in trophoblasts of patients with preeclampsia [Citation138]. Interestingly, a recent study has suggested that LMWH, which has been used for the treatment of patients with recurrent pregnancy loss, blocks the HMGB1-RAGE interaction, and LMWH may exert anti-inflammatory effects on the placenta under preeclampsia [Citation116]. Indeed, heparin can modulate the conformation of HMGB1 and reduce the affinity of HMGB1 toward RAGE [Citation115]. Thus, alarmins may be significantly involved in the development of preeclampsia, and investigating the function of alarmins may provide new therapeutic approaches for the prevention of preeclampsia.
1.3.5. Endometriosis
Endometriosis affects more than 10% of women of reproductive age and causes dysmenorrhea, dyspareunia, and infertility [Citation139]. Endometrial tissues grow ectopically outside the uterine endometrium, leading to the adhesion of ectopic endometrial tissues to the other organs, the peritoneum, ovaries, and the myometrium. The clinical features of endometriosis are characterized by intraperitoneal adhesion, endometriotic cysts, and uterine adenomyosis. Endometriosis is also associated with inflammation of the intrauterine tissue, peritoneum, and ovaries. A recent review highlighted that non-specific bacterial infection and subsequent sterile inflammation and oxidative stress are essential for the development of endometriosis [Citation140]. Chronic inflammation related to danger signals, HSP, S100 protein, fibronectin, oxidized low density lipoprotein, neutrophil elastase, and hyaluronan promotes the development of endometriosis via TLRs and NF-κB signaling [Citation141]. Together, these data indicate that the pathogenesis of endometriosis consists of excessive inflammation. By contrast, other studies suggest that insufficient removal of retrograde menstrual tissue in the pelvic cavity is responsible for the development of endometriosis [Citation142]. Elevation of regulatory T cells and the decrease of Th17 cells were observed in patients with endometriosis [Citation143]. This indicates that the anti-inflammatory milieu enables ectopic endometrial lesions to survive in the pelvic cavity. IL-33, a known alarmin and a number of the IL-1 family that induces cytokines of the Th2 subtype, was expressed on endometriosis lesions [Citation126,Citation144]. Ono et al. showed that IL-33 polarizes peritoneal macrophages to the M2 immunosuppressive phenotype with the elevation of IL-1β mRNA levels; furthermore, IL-1β induces IL-33 in endometriotic stromal cells [Citation126]. In general, macrophages consist of two plastic subsets, M1 and M2 [Citation145,Citation146]. The M1 phenotype can produce pro-inflammatory cytokines and promote Th1 responses [Citation147,Citation148]. By contrast, the M2 phenotype promotes the Th2 response and has immunosuppressive effects responsible for tissue remodeling, resolution of chronic inflammation, and tumor progression [Citation148,Citation149]. Therefore, IL-33 polarization of macrophages to the M2 phenotype in endometriosis lesions would preclude the removal of menstrual tissue or debris. Moreover, the cycle of IL-1β and IL-33 production may further exacerbate the symptoms of endometriosis.
The pathogenesis of endometriosis is also linked to NK cell function. The cell number and the cytotoxic activity of NK cells were diminished in patients with endometriosis [Citation150–153]. A recent study reported that the expression of NKp46, which is an activation marker, on NK cells in the peritoneal fluid of severe endometriosis was significantly lower than that of controls [Citation154]. These results indicate that NK cells promote defective clearance of retrograde menstrual tissue.
Collectively, these data show that excessive inflammation occurs in endometriosis and that appropriate inflammation by macrophages and NK cells to eliminate menstrual tissue may be required for the prevention of endometriosis.
1.4. Chronic endometritis
The concept of chronic endometritis has been established for decades, and it was initially thought to have few significant clinical symptoms [Citation155]. However, resent studies have revealed that chronic endometritis promotes implantation failure and pregnancy loss [Citation8,Citation156–158]. The pathological feature of chronic endometritis is the infiltration of CD138 (Syndecan-1)-positive plasma cells into the endometrium [Citation156,Citation159–161]. Hysteroscopic observation showed stromal edema and thickening, micro polyps, and focal or diffuse hyperemia in patients with chronic endometritis [Citation6,Citation156,Citation162]. Aberrant inflammatory responses are expected in the uterine lumen; indeed, pro-inflammatory cytokines such as IL-6, IL-1β, and TNF-α increased in menstrual effluents of women with chronic endometritis [Citation163]. Pathogenesis of chronic endometritis is thought to be caused by infection with bacteria such as Escherichia coli, Streptococcus spp., Staphylococcus spp., Enterococcus faecalis, Corynebacterium, Mycoplasma, and Ureaplasma [Citation156,Citation162]. Chronic endometritis is a frequent finding in patients with recurrent pregnancy loss and repeated, unexplained implantation failure [Citation6,Citation8]. Importantly, antibiotics for patients with chronic endometritis can improve the pregnancy rate and live birth rate in these patients [Citation6–8]. This clinical indication confirms that chronic endometritis is caused by microbial infection. However, cases have arisen for which antibiotics did not improve chronic endometritis [Citation6]. Therefore, chronic endometritis is caused not only by microbial infections but also by other factors, intrauterine leiomyomas, endometrial biopsy, and endometriosis [Citation161,Citation164]. Further investigation into the etiology and more appropriate treatments for chronic endometritis are required.
1.5. Inflammasomes
Inflammasomes are composed of a protein complex including inflammasome sensor molecules such as NLRs, the adaptor protein, apoptosis-associated speck-like protein containing a caspase recruitment domain, and the caspase-1 precursor [Citation165]. In particular, NOD-, LRP-, and pyrin domain-containing protein 3 (NLRP3) among the NLRs has been widely investigated as a sensor of endogenous and exogenous danger signals [Citation166]. In response to stimulation of these danger signals in the cytoplasm, the NLRP3 inflammasome is assembled to induce cleavage of pro-caspase-1 into its activate form. Subsequently, active caspase-1 converts pro-IL-1β and pro-IL-18 to mature IL-1β and IL-18 as well as converting gasdermin D (GSDMD) to the N-terminal fragment, which forms GSDMD pores in the plasma membrane [Citation167]. These pro-inflammatory cytokines are secreted extracellularly through GSDMD pores, thus inducing the inflammatory cell death pathway of pyroptosis and provoking inflammation in organs and tissues. Therefore, the NLRP3 inflammasome is considered to be the intracellular innate sensor of alarmins and strictly modulates the production of IL-1β and IL-18. The NLRP3 inflammasome has been implicated in many inflammatory diseases, metabolic disorders, cancer, atherosclerosis, ischemic brain injury, and type 2 diabetes [Citation1,Citation168–170]. In reproduction, several studies of inflammasomes have been performed for many pregnancy complications. Mulla et al. reported that inflammasomes in trophoblast cells are stimulated by uric acid, an alarmin that increases in patients with preeclampsia [Citation171]. Uric acid also facilitates placental inflammation and fetal growth restriction [Citation172]. Kohli et al. showed that the injection of maternal extracellular vesicles released from the placenta induces a preeclampsia-like phenotype in pregnant mice via inflammasome activation in trophoblasts [Citation173]. In women with spontaneous term labor, a higher concentration of NLRP3 and NOD1 as well as an increase in the active form of caspase-1 and caspase-4 were observed with higher amounts of IL-1β but not IL-18 [Citation174]. In addition, increased levels of NLRP3 were observed in human myometrial cells with spontaneous labor [Citation175]. In patients with recurrent pregnancy loss, endometrial NLRP3, caspase-1, and IL-1β expression were upregulated by lipopolysaccharide (LPS) invading maternal circulation via leakage of the gut [Citation176]. Exogenous and endogenous particles also stimulate NLRP3 activity [Citation177]. Nanoparticles, silica, and titanium dioxide, can induce fetal growth restriction and placental dysfunction [Citation178]. Therefore, investigating inflammasomes during reproduction may enable establishment of new prediction tools and new therapeutic targets for complications during pregnancy.
1.6. Beneficial effects of inflammation
1.6.1. Ovulation
The hypothesis that ovulation is considered to be an inflammatory response was presented by Espey [Citation9] and is now widely accepted (reviewed in [Citation179]). Ovulation is initiated by the luteinizing hormone (LH) surge. The invasion of vessels into the granulosa cell region with the disruption of granulosa basal lamina allows the infiltration of theca cells and leukocytes into this region. After dilatation and permeability of the vessels occur, the cumulus oocyte complex is detached from the surrounding granulosa cells and enlarged (cumulus expansion). The cumulus-enclosed oocyte is released after the follicle deteriorates and ruptures; afterwards, the ruptured tissue is repaired.
Thus, ovulation is a complex interaction of the oocyte, granulosa cells, theca cells, endothelial cells, and resident and infiltrated immune cells with the secretion of inflammatory mediators, prostaglandins, reproductive hormones, matrix metalloproteinases, cytokines, and chemokines. In fact, the preovulatory follicle contains inflammatory cytokines, such as IL-1, IL-2, IL-6, TNF-α, granulocyte-macrophage colony-stimulating factor (GM-CSF), and macrophage colony-stimulating factor (M-CSF) [Citation180–185]. The LH surge produces chemokines that can attract various immune cells, such as neutrophils, monocytes, macrophages, NK cells, B cells, and T cells [Citation186–191]. Neutrophils produce cytotoxic peptides and proteases, which can degrade the follicular wall [Citation192,Citation193]. Neutrophils are recruited by bone morphogenetic protein 6, which also suppresses the function of protease inhibitors [Citation194]. Macrophages are crucial for follicular growth and rupture, and they secrete cytokines and chemokines that facilitate the migration of other immune cells [Citation195–197]. NK cells and their chemokine receptors are involved in ovulation and angiogenesis [Citation191,Citation198]. Ovarian DCs are essential for cumulus expansion and ovulation and for restricting ovulatory-associated inflammation [Citation199].
Luteinized unruptured follicle (LUF) is frequent among patients with infertility [Citation200–202] and is defined as the absence of follicular rupture that then results in ovulatory dysfunction. LUF formation could be related to the dysregulation of ovulation-associated inflammation in the ovary. Systemic depletion of neutrophils results in the decrease of the ovulation rate in an animal model [Citation203]. In humans, G-CSF concentration increases in peripheral blood in the late follicular phase of the normal ovulatory cycle [Citation204], and LUF can be improved by administration of G-CSF in the clomiphene citrate cycle [Citation205,Citation206]. These results suggest that insufficient inflammatory response by granulocytes may cause the pathogenesis of LUF.
Polycystic ovary syndrome (PCOS) is a major cause of infertility and is characterized by endocrine disorder, polycystic ovarian morphology, and ovulatory dysfunction [Citation207]. PCOS is also characterized by systemic low-grade inflammation [Citation208,Citation209]. Some studies show that PCOS patients had an elevated C-reactive protein [Citation210] and inflammatory cytokines [Citation211,Citation212]. HMGB1 increases in adolescents with PCOS, and levels of HMGB1 can be decreased by the administration of myo-inositol and alpha-lipoic acid, both considered to be anti-inflammatory [Citation213]. Ovulatory dysfunction related to PCOS may result from an excessive inflammatory response. The inflammation involved in the ovulation process is considered sterile, but infection by pathogens can reduce follicular growth. Granulosa cells express LPS receptors TLR4, CD14, and MD-2, and exposure of granulosa cells to LPS causes disturbance of estradiol production and follicular growth failure in an animal model [Citation214,Citation215].
1.6.2. Early pregnancy
Excessive inflammation in reproduction is associated with the onset of preterm labor/birth, miscarriage, preeclampsia, fetal growth restriction, and neonatal disorders. However, appropriate inflammation is necessary for successful pregnancy outcomes. In humans, pro-inflammatory responses including the secretion of IL-6, IL-8, and TNF-α are required for the acquisition of uterine receptivity [Citation10,Citation216,Citation217]. Moreover, elevation of IL-12, IL-1β, TNF-α, IL-6, and NO promote embryo attachment to the decidua [Citation218]. Endometrial biopsy with mechanical injury induces the production of macrophage inflammatory protein 3 beta, TNF-α, CXCL1, osteopontin, and IL-15 with the abundance of macrophages and DCs [Citation219]. Implantation rates, in vitro fertilization, and clinical pregnancy rates in patients with unexpected infertility were improved by inducing DC and macrophage accumulation via pro-inflammatory cytokine upregulation [Citation219–223]. Indeed, DCs are recruited to the uterus prior to implantation and modulate the cytokine profile at the feto-maternal interface [Citation62,Citation104,Citation224,Citation225], and the adequate inflammation induced by DCs is required for ensuring successful implantation and preventing miscarriage in the first trimester [Citation11]. In our recent study, we observed a low level of DC1s (CD141+ DCs), which establish Th1 polarization, at the region of the uterine septum in patients with septate uterus [Citation226]. In general, the septate uterus is considered a major risk factor of recurrent pregnancy loss [Citation227]. Hence, we concluded that the low CD141+ DC accumulation in uterine septate may establish a hostile immune environment in early pregnancy.
Macrophages with flexible plasticity also play an important role in early pregnancy [Citation218]. The M2 phenotype has important roles for the establishment of folliculogenesis [Citation228]. During the implantation period, macrophages are skewed to the M1 type, which produces pro-inflammatory cytokines [Citation10]. After implantation, macrophages switch to the M1/M2 mixed type and the M2 type to suppress maternal rejection of the fetus as the pregnancy progresses [Citation218,Citation229]. At the end of pregnancy, M1 macrophages secreting pro-inflammatory cytokines are again required for parturition [Citation218,Citation230]. Moreover, IFN-γ, a representative pro-inflammatory cytokine that is mainly produced by uterine NK cells at the feto-maternal interface, has crucial roles in uterine arterial remodeling during early pregnancy (reviewed in [Citation231]). Thus, temporally appropriate inflammation is necessary; however, excessive or insufficient inflammation may induce complications, particularly during early pregnancy.
1.6.3. Parturition
The mechanism of labor onset is controversial at the end of pregnancy; however, inflammation processes are involved in parturition [Citation12]. Prior to term labor, pro-inflammatory cytokines IL-1β, IL-6, IL-8, and TNF-α were upregulated in human fetal membranes, decidua, cervix, and myometrium with the accumulation of leukocytes (mainly neutrophils and macrophages) [Citation232]. Bacterial infections can certainly induce labor as shown during preterm labor with aCAM (see the ‘aCAM’ section); nevertheless, at term labor/birth with normal pregnancy progress, pathogenic microbes are often irrelevant, and the baby is born without infectious signs. These clinical findings imply the existence of sterile inflammation during term delivery. The cffDNA, an alarmin, is increased with pregnancy progress [Citation233], and it is considered that a high level of cffDNA is associated with preterm birth [Citation83]. In addition, the NLRP3 inflammasome is associated with spontaneous term labor [Citation174] as described above (see the ‘Inflammasome’ section). HMGB1 and its receptors TLR2, TLR4, and RAGE are expressed in the human cervix, and HMGB1 may contribute to cervical ripening [Citation234]. Macrophages also have crucial roles in parturition, as they accumulate to the decidua prior to labor in humans and rats [Citation230,Citation232]. Another study showed that macrophages are recruited into the human cervix and contribute to cervical ripening for vaginal delivery [Citation235]. Thus, it is expected that various inflammatory and immune responses occur at the end of pregnancy for successful parturition.
Sterile inflammation may facilitate the parturition; although, the initial trigger of this inflammation is unclear. In the onset of parturition, there are changes in progesterone levels, sensitivity of progesterone receptors, and expression of oxytocin receptors [Citation236,Citation237]. We recently showed in a pregnant murine model that progesterone suppressed the immunostimulatory activity of APCs and recognition of alarmins on PRRs (manuscript under preparation). Thus, we suppose that progesterone may regulate APCs as they act to induce inflammation in the feto-maternal interface. In a study of dental stress, mechanical stresses were shown to induce inflammation and upregulation of IL-8 secretion via IL-1β [Citation238]. We suspect that mechanical stresses during pregnancy, such as uterine construction and stretching of the uterine wall, may trigger the release of alarmins and induce inflammation in the feto-maternal interface. We are currently planning experiments to induce inflammation with mechanical stresses to uterine tissues.
1.7. Reception and rejection roles of sterile inflammation
As mentioned above, sterile inflammation is critical for a successful pregnancy, but pregnancy complications can be caused by excessive or insufficient inflammation. In the same way, sterile inflammation can have both reception and rejection roles for the semi-allogeneic fetus. Increased production of IFN-γ, an important Th1 type cytokine, is associated with infertility and the antiphospholipid syndrome (APS) [Citation239–241]. IFN-γ production, upregulated by excessive inflammation, can cause fetal rejection. However, it has been reported that IFN-γ contributes to uterine vascular modification [Citation231,Citation242], and the proportion of IFN-γ and TNF-α positive cells decreased in the endometrium of patients with recurrent pregnancy loss [Citation243]. These findings indicate that IFN-γ secretion can also have a positive role in maintaining a successful pregnancy. Human chorionic gonadotropin (hCG) is a placental glycoprotein hormone that increases significantly in early pregnancy. It induces IL-8 secretion from monocytes [Citation244] and contributes to the differentiation of the endometrium and the implantation via the modulation of immune cell activity [Citation245–248]. By contrast, overexpression of hCG provokes an excessive inflammatory state and pregnancy failures. In clinical cases, elevation of hCG production is observed in patients with ovarian hyperstimulation syndrome (OHSS). It has been reported that the pro-inflammatory cytokines in plasma and ascitic fluid can lead to reproductive failure and other adverse pathologies in the patients with OHSS [Citation249]. In addition, excessive hCG stimulation can cause multiple defects in the differentiation of the fetal gonad [Citation250,Citation251]. HMGB1 has dual roles in sterile inflammation. It can act as a trigger of excessive inflammation and is associated with infertility [Citation77,Citation110]. However, HMGB1 is also known to induce immunological tolerance that leads to tumor progression [Citation252,Citation253] and vascular remodeling [Citation254]. Thus, sterile inflammation can be associated with both immunogenic and tolerogenic milieu.
Collectively, reception and rejection of semi-allogeneic fetus in the maternal system may depend on the delicate balance of sterile inflammation, and controlled inflammatory responses may be required for the progression of a successful pregnancy.
2. Conclusions
Inflammation is needed throughout a normal pregnancy. A controlled inflammatory response is required for successful ovulation, implantation and placental formation, retention of the semi-allogeneic fetus, protection against external pathogens for the fetus, and, finally, for parturition. However, these reproductive events can also be associated with harmful inflammation and microbial infection, which can provoke various reproductive disorders, such as endometriosis, implantation failure, recurrent pregnancy loss, preterm labor/birth, and preeclampsia. The inflammatory milieu in each disease is complex and involves the function of various immune cells; consequently, it is difficult to determine strictly whether each disorder is induced by microbial or non-microbial causes (). Further investigation of these diseases in terms of inflammation may lead to new etiological perspectives of pregnancy complications. Inflammation during reproductive disorders involve complex dynamics of immune responses related to the kinetics of immune cells, the secretion of cytokines and chemokines, and the activation of inflammasomes. A deeper understanding of inflammation during reproduction may allow us to establish new therapeutic approaches for these complications and the proper control of pregnancy progression.
Figure 1. Schematic drawing of the pathogenesis of complications during pregnancy involving inflammation. Inflammation induced by microbial or non-microbial causes facilitate pregnancy complications such as preterm labor/birth, miscarriage, implantation failure, preeclampsia, chronic endometritis, and endometriosis. The causes of each disease tend to be of either microbial or non-microbial etiology; however, they often overlap. aCAM: acute chorioamnionitis; cCAM: chronic chorioamnionitis.
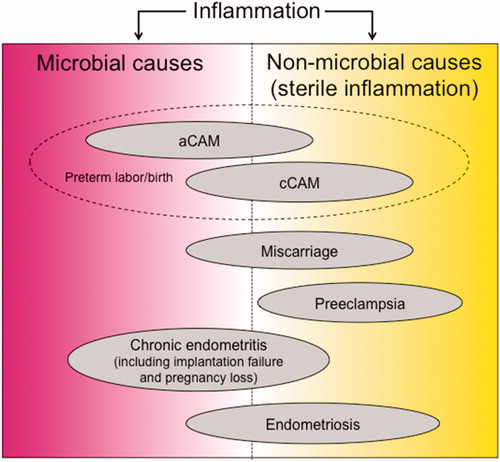
Acknowledgments
Y.N. expresses gratitude for the opportunity to write this review article. The authors thank Enago (www.enago.jp) for the English language review.
Disclosure statement
No potential conflict of interest was reported by the author(s).
References
- Chen GY, Nunez G. Sterile inflammation: sensing and reacting to damage. Nat Rev Immunol. 2010;10(12):826–837.
- Nadeau-Vallee M, Obari D, Palacios J, et al. Sterile inflammation and pregnancy complications: a review. Reproduction. 2016;152(6):R277–R292.
- Romero R, Gomez R, Chaiworapongsa T, et al. The role of infection in preterm labour and delivery. Paediatr Perinat Epidemiol. 2001;15(Suppl 2):41–56.
- Flenady V, Hawley G, Stock OM, et al. Prophylactic antibiotics for inhibiting preterm labour with intact membranes. Cochrane Database Syst Rev. 2013;(12):CD000246.
- Redman CW, Sacks GP, Sargent IL. Preeclampsia: an excessive maternal inflammatory response to pregnancy. Am J Obstet Gynecol. 1999;180(2 Pt 1):499–506.
- Cicinelli E, Matteo M, Tinelli R, et al. Prevalence of chronic endometritis in repeated unexplained implantation failure and the IVF success rate after antibiotic therapy. Hum Reprod. 2015;30(2):323–330.
- McQueen DB, Bernardi LA, Stephenson MD. Chronic endometritis in women with recurrent early pregnancy loss and/or fetal demise. Fertil Steril. 2014;101(4):1026–1030.
- Johnston-MacAnanny EB, Hartnett J, Engmann LL, et al. Chronic endometritis is a frequent finding in women with recurrent implantation failure after in vitro fertilization. Fertil Steril. 2010;93(2):437–441.
- Espey LL. Ovulation as an inflammatory reaction-a hypothesis. Biol Reprod. 1980;22(1):73–106.
- Mor G, Cardenas I, Abrahams V, et al. Inflammation and pregnancy: the role of the immune system at the implantation site. Ann N Y Acad Sci. 2011;1221:80–87.
- Dekel N, Gnainsky Y, Granot I, et al. The role of inflammation for a successful implantation. Am J Reprod Immunol. 2014;72(2):141–147.
- Thomson AJ, Telfer JF, Young A, et al. Leukocytes infiltrate the myometrium during human parturition: further evidence that labour is an inflammatory process. Hum Reprod. 1999;14(1):229–236.
- Blencowe H, Cousens S, Oestergaard MZ, et al. National, regional, and worldwide estimates of preterm birth rates in the year 2010 with time trends since 1990 for selected countries: a systematic analysis and implications. Lancet. 2012;379(9832):2162–2172.
- Splichal I, Trebichavsky I. Cytokines and other important inflammatory mediators in gestation and bacterial intraamniotic infections. Folia Microbiol (Praha). 2001;46(4):345–351.
- Romero R, Durum S, Dinarello CA, et al. Interleukin-1 stimulates prostaglandin biosynthesis by human amnion. Prostaglandins. 1989;37(1):13–22.
- Romero R, Brody DT, Oyarzun E, et al. Infection and labor. III. Interleukin-1: a signal for the onset of parturition. Am J Obstet Gynecol. 1989;160(5 Pt 1):1117–1123.
- Romero R, Mazor M, Wu YK, et al. Infection in the pathogenesis of preterm labor. Semin Perinatol. 1988;12(4):262–279.
- Athayde N, Edwin SS, Romero R, et al. A role for matrix metalloproteinase-9 in spontaneous rupture of the fetal membranes. Am J Obstetr Gynecol. 1998;179(5):1248–1253.
- Fortunato SJ, Menon R, Lombardi SJ. Role of tumor necrosis factor-alpha in the premature rupture of membranes and preterm labor pathways. Am J Obstet Gynecol. 2002;187(5):1159–1162.
- Maymon E, Romero R, Pacora P, et al. Evidence of in vivo differential bioavailability of the active forms of matrix metalloproteinases 9 and 2 in parturition, spontaneous rupture of membranes, and intra-amniotic infection. Am J Obstetr Gynecol. 2000;183(4):887–894.
- Osmers RG, Adelmann-Grill BC, Rath W, et al. Biochemical events in cervical ripening dilatation during pregnancy and parturition. J Obstet Gynaecol (Tokyo 1995). 1995;21(2):185–194.
- Rath W, Winkler M, Kemp B. The importance of extracellular matrix in the induction of preterm delivery. J Perinat Med. 1998;26(6):437–441.
- Romero R, Chaiworapongsa T, Espinoza J, et al. Fetal plasma MMP-9 concentrations are elevated in preterm premature rupture of the membranes. Am J Obstetr Gynecol. 2002;187(5):1125–1130.
- Watari M, Watari H, DiSanto ME, et al. Pro-inflammatory cytokines induce expression of matrix-metabolizing enzymes in human cervical smooth muscle cells. Am J Pathol. 1999;154(6):1755–1762.
- Lahra MM, Jeffery HE. A fetal response to chorioamnionitis is associated with early survival after preterm birth. Am J Obstet Gynecol. 2004;190(1):147–151.
- Goldenberg RL, Culhane JF, Iams JD, et al. Epidemiology and causes of preterm birth. Lancet. 2008;371(9606):75–84.
- McElrath TF, Hecht JL, Dammann O, et al. Pregnancy disorders that lead to delivery before the 28th week of gestation: an epidemiologic approach to classification. Am J Epidemiol. 2008;168(9):980–989.
- Goncalves LF, Chaiworapongsa T, Romero R. Intrauterine infection and prematurity. Ment Retard Dev Disabil Res Rev. 2002;8(1):3–13.
- Romero R, Salafia CM, Athanassiadis AP, et al. The relationship between acute inflammatory lesions of the preterm placenta and amniotic fluid microbiology. Am J Obstet Gynecol. 1992;166(5):1382–1388.
- Romero R, Mazor M, Morrotti R, et al. Infection and labor. VII. Microbial invasion of the amniotic cavity in spontaneous rupture of membranes at term. Am J Obstet Gynecol. 1992;166(1 Pt 1):129–133.
- Romero R, Sirtori M, Oyarzun E, et al. Infection and labor. V. Prevalence, microbiology, and clinical significance of intraamniotic infection in women with preterm labor and intact membranes. Am J Obstet Gynecol. 1989;161(3):817–824.
- Hillier SL, Martius J, Krohn M, et al. A case-control study of chorioamnionic infection and histologic chorioamnionitis in prematurity. N Engl J Med. 1988;319(15):972–978.
- Christiaens I, Zaragoza DB, Guilbert L, et al. Inflammatory processes in preterm and term parturition. J Reprod Immunol. 2008;79(1):50–57.
- Romero R, Espinoza J, Goncalves LF, et al. Inflammation in preterm and term labour and delivery. Semin Fetal Neonatal Med. 2006;11(5):317–326.
- Romero R, Miranda J, Chaiworapongsa T, et al. Prevalence and clinical significance of sterile intra-amniotic inflammation in patients with preterm labor and intact membranes. Am J Reprod Immunol. 2014;72(5):458–474.
- Rudolph MD, Graham AM, Feczko E, et al. Maternal IL-6 during pregnancy can be estimated from newborn brain connectivity and predicts future working memory in offspring. Nat Neurosci. 2018;21(5):765–772.
- Gomez R, Romero R, Ghezzi F, et al. The fetal inflammatory response syndrome. American J Obstetr Gynecol. 1998;179(1):194–202.
- Romero R, Gomez R, Ghezzi F, et al. A fetal systemic inflammatory response is followed by the spontaneous onset of preterm parturition. Am J Obstetr Gynecol. 1998;179(1):186–193.
- Gantert M, Been JV, Gavilanes AW, et al. Chorioamnionitis: a multiorgan disease of the fetus? J Perinatol. 2010;30 Suppl:S21–S30.
- Yoneda S, Shiozaki A, Ito M, et al. Accurate prediction of the stage of histological chorioamnionitis before delivery by amniotic fluid IL-8 level. Am J Reprod Immunol. 2015;73(6):568–576.
- Yoneda S, Shiozaki A, Yoneda N, et al. Antibiotic therapy increases the risk of preterm birth in preterm labor without intra-amniotic microbes, but may prolong the gestation period in preterm labor with microbes, evaluated by rapid and high-sensitive PCR system. Am J Reprod Immunol. 2016;75(4):440–450.
- Khong TY, Mooney EE, Ariel I, et al. Sampling and definitions of placental lesions: amsterdam placental workshop group consensus statement. Arch Pathol Lab Med. 2016;140(7):698–713.
- Chellam VG, Rushton DI. Chorioamnionitis and funiculitis in the placentas of 200 births weighing less than 2.5 kg. Br J Obstet Gynaecol. 1985;92(8):808–814.
- Kim CJ, Romero R, Kusanovic JP, et al. The frequency, clinical significance, and pathological features of chronic chorioamnionitis: a lesion associated with spontaneous preterm birth. Mod Pathol. 2010;23(7):1000–1011.
- Lee J, Kim JS, Park JW, Park CW, et al. Chronic chorioamnionitis is the most common placental lesion in late preterm birth. Placenta. 2013;34(8):681–689.
- Kim CJ, Romero R, Chaemsaithong P, et al. Chronic inflammation of the placenta: definition, classification, pathogenesis, and clinical significance. Am J Obstet Gynecol. 2015;213(4 Suppl):S53–S69.
- Romero R, Espinoza J, Goncalves LF, et al. The role of inflammation and infection in preterm birth. Semin Reprod Med. 2007;25(1):21–39.
- Tsekoura EA, Konstantinidou A, Papadopoulou S, et al. Adenovirus genome in the placenta: association with histological chorioamnionitis and preterm birth. J Med Virol. 2010;82(8):1379–1383.
- Gersell DJ, Phillips NJ, Beckerman K. Chronic chorioamnionitis: a clinicopathologic study of 17 cases. Int J Gynecol Pathol. 1991;10(3):217–229.
- Jacques SM, Qureshi F. Chronic chorioamnionitis: a clinicopathologic and immunohistochemical study. Hum Pathol. 1998;29(12):1457–1461.
- Arenas-Hernandez M, Romero R, Xu Y, et al. Effector and activated T cells induce preterm labor and birth that is prevented by treatment with progesterone. J Immunol. 2019;202(9):2585–2608.
- Bendelac A. Positive selection of mouse NK1+ T cells by CD1-expressing cortical thymocytes. J Exp Med. 1995;182(6):2091–2096.
- Kawano T, Cui J, Koezuka Y, et al. Natural killer-like nonspecific tumor cell lysis mediated by specific ligand-activated Valpha14 NKT cells. Proc Natl Acad Sci USA. 1998;95(10):5690–5693.
- Zhou D, Mattner J, Cantu C, et al. Lysosomal glycosphingolipid recognition by NKT cells. Science. 2004;306(5702):1786–1789.
- Montoya CJ, Pollard D, Martinson J, et al. Characterization of human invariant natural killer T subsets in health and disease using a novel invariant natural killer T cell-clonotypic monoclonal antibody, 6B11. Immunology. 2007;122(1):1–14.
- Kawano T, Cui J, Koezuka Y, et al. CD1d-restricted and TCR-mediated activation of valpha14 NKT cells by glycosylceramides. Science. 1997;278(5343):1626–1629.
- Spada FM, Koezuka Y, Porcelli SA. CD1d-restricted recognition of synthetic glycolipid antigens by human natural killer T cells. J Exp Med. 1998;188(8):1529–1534.
- St Louis D, Romero R, Plazyo O, et al. Invariant NKT cell activation induces late preterm birth that is attenuated by rosiglitazone. J Immunol. 2016;196(3):1044–1059.
- Boyson JE, Rybalov B, Koopman LA, et al. CD1d and invariant NKT cells at the human maternal-fetal interface. Proc Natl Acad Sci USA. 2002;99(21):13741–13746.
- Ito K, Karasawa M, Kawano T, et al. Involvement of decidual Valpha14 NKT cells in abortion. Proc Natl Acad Sci USA. 2000;97(2):740–744.
- Tsuda H, Sakai M, Michimata T, et al. Characterization of NKT cells in human peripheral blood and decidual lymphocytes. Am J Reprod Immunol. 2001;45(5):295–302.
- Laskarin G, Kammerer U, Rukavina D, et al. Antigen-presenting cells and materno-fetal tolerance: an emerging role for dendritic cells. Am J Reprod Immunol. 2007;58(3):255–267.
- Negishi Y, Shima Y, Takeshita T, et al. Distribution of invariant natural killer T cells and dendritic cells in late pre-term birth without acute chorioamnionitis. Am J Reprod Immunol. 2017;77(6):e12658.
- Gupta S, Goldberg JM, Aziz N, et al. Pathogenic mechanisms in endometriosis-associated infertility. Fertil Steril. 2008;90(2):247–257.
- Laird SM, Tuckerman EM, Cork BA, et al. A review of immune cells and molecules in women with recurrent miscarriage. Hum Reprod Update. 2003;9(2):163–174.
- von Wolff M, Thaler CJ, Strowitzki T, et al. Regulated expression of cytokines in human endometrium throughout the menstrual cycle: dysregulation in habitual abortion. Mol Hum Reprod. 2000;6(7):627–634.
- Coussens LM, Werb Z. Inflammation and cancer. Nature. 2002;420(6917):860–867.
- Tang SCW, Yiu WH. Innate immunity in diabetic kidney disease. Nat Rev Nephrol. 2020;16(4):206–222.
- Freigang S, Ampenberger F, Weiss A, et al. Fatty acid-induced mitochondrial uncoupling elicits inflammasome-independent IL-1α and sterile vascular inflammation in atherosclerosis. Nat Immunol. 2013;14(10):1045–1053.
- Ross R. Atherosclerosis-an inflammatory disease. N Engl J Med. 1999;340(2):115–126.
- Mossman BT, Churg A. Mechanisms in the pathogenesis of asbestosis and silicosis. Am J Respir Crit Care Med. 1998;157(5 Pt 1):1666–1680.
- Hornung V, Bauernfeind F, Halle A, et al. Silica crystals and aluminum salts activate the NALP3 inflammasome through phagosomal destabilization. Nat Immunol. 2008;9(8):847–856.
- Leso V, Fontana L, Iavicoli I. Nanomaterial exposure and sterile inflammatory reactions. Toxicol Appl Pharmacol. 2018;355:80–92.
- Lappas M. NOD1 and NOD2 regulate proinflammatory and prolabor mediators in human fetal membranes and myometrium via nuclear factor-kappa B. Biol Reprod. 2013;89(1):14.
- Koga K, Mor G. Toll-like receptors at the maternal-fetal interface in normal pregnancy and pregnancy disorders. Am J Reprod Immunol. 2010;63(6):587–600.
- Zhang YY, Chen H, Sun C, et al. Expression and functional characterization of NOD2 in decidual stromal cells isolated during the first trimester of pregnancy. PloS One. 2014;9(6):e99612.
- Venereau E, Schiraldi M, Uguccioni M, et al. HMGB1 and leukocyte migration during trauma and sterile inflammation. Mol Immunol. 2013;55(1):76–82.
- Andersson U, Tracey KJ. HMGB1 is a therapeutic target for sterile inflammation and infection. Annu Rev Immunol. 2011;29:139–162.
- Romero R, Chaiworapongsa T, Alpay Savasan Z, et al. Damage-associated molecular patterns (DAMPs) in preterm labor with intact membranes and preterm PROM: a study of the alarmin HMGB1. J Matern Fetal Neonatal Med. 2011;24(12):1444–1455.
- Gomez-Lopez N, Romero R, Plazyo O, et al. Intra-amniotic administration of HMGB1 induces spontaneous preterm labor and birth. Am J Reprod Immunol. 2016;75(1):3–7.
- Leung TN, Zhang J, Lau TK, et al. Maternal plasma fetal DNA as a marker for preterm labour. Lancet. 1998;352(9144):1904–1905.
- Farina A, LeShane ES, Romero R, et al. High levels of fetal cell-free DNA in maternal serum: a risk factor for spontaneous preterm delivery. Am J Obstetr Gynecol. 2005;193(2):421–425.
- Jakobsen TR, Clausen FB, Rode L, et al. High levels of fetal DNA are associated with increased risk of spontaneous preterm delivery. Prenat Diagn. 2012;32(9):840–845.
- Lukens JR, Gross JM, Kanneganti TD. IL-1 family cytokines trigger sterile inflammatory disease. Front Immunol. 2012;3:315.
- Cohen I, Rider P, Carmi Y, et al. Differential release of chromatin-bound IL-1alpha discriminates between necrotic and apoptotic cell death by the ability to induce sterile inflammation. Proc Natl Acad Sci USA. 2010;107(6):2574–2579.
- Eigenbrod T, Park JH, Harder J, et al. Cutting edge: critical role for mesothelial cells in necrosis-induced inflammation through the recognition of IL-1 alpha released from dying cells. J Immunol. 2008;181(12):8194–8198.
- Romero R, Grivel JC, Tarca AL, et al. Evidence of perturbations of the cytokine network in preterm labor. Am J Obstet Gynecol. 2015;213(6):836.e1–836.e18.
- Figueroa R, Garry D, Elimian A, et al. Evaluation of amniotic fluid cytokines in preterm labor and intact membranes. J Matern Fetal Neonatal Med. 2005;18(4):241–247.
- Brien ME, Baker B, Duval C, et al. Alarmins at the maternal-fetal interface: involvement of inflammation in placental dysfunction and pregnancy complications1. Can J Physiol Pharmacol. 2019;97(3):206–212.
- Chen Q, Yin YX, Wei J, et al. Increased expression of high mobility group box 1 (HMGB1) in the cytoplasm of placental syncytiotrophoblast from preeclamptic placentae. Cytokine. 2016;85:30–36.
- Liu X, Zhou M, Wu J, et al. HMGB1 release from trophoblasts contributes to inflammation during Brucella melitensis infection. Cell Microbiol. 2019;21(10):e13080.
- Boyson JE, Aktan I, Barkhuff DA, et al. NKT cells at the maternal-fetal interface. Immunol Invest. 2008;37(5):565–582.
- Brennan PJ, Brigl M, Brenner MB. Invariant natural killer T cells: an innate activation scheme linked to diverse effector functions. Nat Rev Immunol. 2013;13(2):101–117.
- Godfrey DI, MacDonald HR, Kronenberg M, et al. NKT cells: what’s in a name? Nat Rev Immunol. 2004;4(3):231–237.
- Rinaldi SF, Makieva S, Saunders PT, et al. Immune cell and transcriptomic analysis of the human decidua in term and preterm parturition. Mol Hum Reprod. 2017;23(10):708–724.
- Merad M, Sathe P, Helft J, et al. The dendritic cell lineage: ontogeny and function of dendritic cells and their subsets in the steady state and the inflamed setting. Annu Rev Immunol. 2013;31:563–604.
- Satpathy AT, Wu X, Albring JC, et al. Re(de)fining the dendritic cell lineage. Nat Immunol. 2012;13(12):1145–1154.
- Steinman RM. Decisions about dendritic cells: past, present, and future. Annu Rev Immunol. 2012;30:1–22.
- Bozzacco L, Trumpfheller C, Siegal FP, et al. DEC-205 receptor on dendritic cells mediates presentation of HIV gag protein to CD8+ T cells in a spectrum of human MHC I haplotypes. Proc Natl Acad Sci USA. 2007;104(4):1289–1294.
- Collin M, Bigley V, Haniffa M, et al. Human dendritic cell deficiency: the missing ID? Nat Rev Immunol. 2011;11(9):575–583.
- Guilliams M, Ginhoux F, Jakubzick C, et al. Dendritic cells, monocytes and macrophages: a unified nomenclature based on ontogeny. Nat Rev Immunol. 2014;14(8):571–578.
- Dzionek A, Fuchs A, Schmidt P, et al. BDCA-2, BDCA-3, and BDCA-4: three markers for distinct subsets of dendritic cells in human peripheral blood. J Immunol. 2000;165(11):6037–6046.
- Reynolds G, Haniffa M. Human and mouse mononuclear phagocyte networks: a tale of two species? Front Immunol. 2015;6:330.
- Plaks V, Birnberg T, Berkutzki T, et al. Uterine DCs are crucial for decidua formation during embryo implantation in mice. J Clin Invest. 2008;118(12):3954–3965.
- Collins MK, Tay CS, Erlebacher A. Dendritic cell entrapment within the pregnant uterus inhibits immune surveillance of the maternal/fetal interface in mice. J Clin Invest. 2009;119(7):2062–2073.
- Negishi Y, Wakabayashi A, Shimizu M, et al. Disruption of maternal immune balance maintained by innate DC subsets results in spontaneous pregnancy loss in mice. Immunobiology. 2012;217(10):951–961.
- Ichikawa T, Negishi Y, Shimizu M, et al. α-Galactosylceramide-activated murine NK1.1(+) invariant-NKT cells in the myometrium induce miscarriages in mice. Eur J Immunol. 2016;46(8):1867–1877.
- Negishi Y, Ichikawa T, Takeshita T, et al. Miscarriage induced by adoptive transfer of dendritic cells and invariant natural killer T cells into mice. Eur J Immunol. 2018;48(6):937–949.
- Nagamatsu T, Fujii T, Schust DJ, et al. Tokishakuyakusan, a traditional Japanese medicine (Kampo) mitigates iNKT cell-mediated pregnancy loss in mice. Am J Reprod Immunol. 2018;80(4):e13021.
- Bhutada S, Basak T, Savardekar L, et al. High mobility group box 1 (HMGB1) protein in human uterine fluid and its relevance in implantation. Hum Reprod. 2014;29(4):763–780.
- Shirasuna K, Seno K, Ohtsu A, et al. AGEs and HMGB1 increase inflammatory cytokine production from human placental cells, resulting in an enhancement of monocyte migration. Am J Reprod Immunol. 2016;75(5):557–568.
- Nair RR, Khanna A, Singh K. Association of increased S100A8 serum protein with early pregnancy loss. Am J Reprod Immunol. 2015;73(2):91–94.
- Comba C, Bastu E, Dural O, et al. Role of inflammatory mediators in patients with recurrent pregnancy loss. Fertil Steril. 2015;104(6):1467–1474 e1.
- Quaranta M, Erez O, Mastrolia SA, et al. The physiologic and therapeutic role of heparin in implantation and placentation. PeerJ. 2015;3:e691.
- Ling Y, Yang ZY, Yin T, et al. Heparin changes the conformation of high-mobility group protein 1 and decreases its affinity toward receptor for advanced glycation endproducts in vitro. Int Immunopharmacol. 2011;11(2):187–193.
- Zenerino C, Nuzzo AM, Giuffrida D, et al. The HMGB1/RAGE pro-inflammatory axis in the human placenta: modulating effect of low molecular weight heparin. Molecules. 2017;22(11):1997.
- Fawzy M, Shokeir T, El-Tatongy M, et al. Treatment options and pregnancy outcome in women with idiopathic recurrent miscarriage: a randomized placebo-controlled study. Arch Gynecol Obstet. 2008;278(1):33–38.
- Gomaa MF, Elkholy AG, El-Said MM, et al. Combined oral prednisolone and heparin versus heparin: the effect on peripheral NK cells and clinical outcome in patients with unexplained recurrent miscarriage. A double-blind placebo randomized controlled trial. Arch Gynecol Obstet. 2014;290(4):757–762.
- Yamada H, Morikawa M, Furuta I, et al. Intravenous immunoglobulin treatment in women with recurrent abortions: increased cytokine levels and reduced Th1/Th2 lymphocyte ratio in peripheral blood. Am J Reprod Immunol. 2003;49(2):84–89.
- Winger EE, Reed JL. Treatment with tumor necrosis factor inhibitors and intravenous immunoglobulin improves live birth rates in women with recurrent spontaneous abortion. Am J Reprod Immunol. 2008;60(1):8–16.
- Scarpellini F, Sbracia M. Use of granulocyte colony-stimulating factor for the treatment of unexplained recurrent miscarriage: a randomised controlled trial. Hum Reprod. 2009;24(11):2703–2708.
- Coulam CB, Acacio B. Does immunotherapy for treatment of reproductive failure enhance live births? Am J Reprod Immunol. 2012;67(4):296–304.
- Mekinian A, Cohen J, Alijotas-Reig J, et al. Unexplained recurrent miscarriage and recurrent implantation failure: is there a place for immunomodulation? Am J Reprod Immunol. 2016;76(1):8–28.
- Achilli C, Duran-Retamal M, Saab W, et al. The role of immunotherapy in in vitro fertilization and recurrent pregnancy loss: a systematic review and meta-analysis. Fertil Steril. 2018;110(6):1089–1100.
- Jin H, Wu J, Yang Q, et al. High mobility group box 1 protein polymorphism affects susceptibility to recurrent pregnancy loss by up-regulating gene expression in chorionic villi. J Assist Reprod Genet. 2015;32(7):1123–1128.
- Ono Y, Yoshino O, Hiraoka T, et al. IL-33 exacerbates endometriotic lesions via polarizing peritoneal macrophages to M2 subtype. Reprod Sci. 2020;27(3):869–876.
- Redman CW, Sargent IL. Placental stress and pre-eclampsia: a revised view. Placenta. 2009;30 (Suppl A):S38–S42.
- Roberts JM, Hubel CA. The two stage model of preeclampsia: variations on the theme. Placenta. 2009;30 Suppl A:S32–S37.
- Levine RJ, Lam C, Qian C, et al. Soluble endoglin and other circulating antiangiogenic factors in preeclampsia. N Engl J Med. 2006;355(10):992–1005.
- Aoki A, Nakashima A, Kusabiraki T, et al. Trophoblast-specific conditional Atg7 knockout mice develop gestational hypertension. Am J Pathol. 2018;188(11):2474–2486.
- Nakashima A, Yamanaka-Tatematsu M, Fujita N, et al. Impaired autophagy by soluble endoglin, under physiological hypoxia in early pregnant period, is involved in poor placentation in preeclampsia. Autophagy. 2013;9(3):303–316.
- Myatt L, Webster RP. Vascular biology of preeclampsia. J Thromb Haemost. 2009;7(3):375–384.
- Sanchez-Aranguren LC, Prada CE, Riano-Medina CE, et al. Endothelial dysfunction and preeclampsia: role of oxidative stress. Front Physiol. 2014;5:372.
- Khong TY, De Wolf F, Robertson WB, et al. Inadequate maternal vascular response to placentation in pregnancies complicated by pre-eclampsia and by small-for-gestational age infants. Br J Obstet Gynaecol. 1986;93(10):1049–1059.
- Srinivas SK, Edlow AG, Neff PM, et al. Rethinking IUGR in preeclampsia: dependent or independent of maternal hypertension? J Perinatol. 2009;29(10):680–684.
- Pradervand PA, Clerc S, Frantz J, et al. High mobility group box 1 protein (HMGB-1): a pathogenic role in preeclampsia? Placenta. 2014;35(9):784–786.
- Zhu L, Zhang Z, Zhang L, et al. HMGB1-RAGE signaling pathway in severe preeclampsia. Placenta. 2015;36(10):1148–1152.
- Kim YM, Romero R, Oh SY, et al. Toll-like receptor 4: a potential link between “danger signals,” the innate immune system, and preeclampsia? Am J Obstet Gynecol. 2005;193(3 Pt 2):921–927.
- Thiruchelvam U, Wingfield M, O’Farrelly C. Natural killer cells: key players in endometriosis. Am J Reprod Immunol. 2015;74(4):291–301.
- Kobayashi H, Higashiura Y, Shigetomi H, et al. Pathogenesis of endometriosis: the role of initial infection and subsequent sterile inflammation (Review). Mol Med Rep. 2014;9(1):9–15.
- Kajihara H, Yamada Y, Kanayama S, et al. New insights into the pathophysiology of endometriosis: from chronic inflammation to danger signal. Gynecol Endocrinol. 2011;27(2):73–79.
- Young VJ, Brown JK, Saunders PT, et al. The role of the peritoneum in the pathogenesis of endometriosis. Hum Reprod Update. 2013;19(5):558–569.
- Khan KN, Yamamoto K, Fujishita A, et al. Differential levels of regulatory T cells and T-Helper-17 cells in women with early and advanced endometriosis. J Clin Endocrinol Metab. 2019;104(10):4715–4729.
- Santulli P, Borghese B, Chouzenoux S, et al. Serum and peritoneal interleukin-33 levels are elevated in deeply infiltrating endometriosis. Hum Reprod. 2012;27(7):2001–2009.
- Mills CD. Macrophage arginine metabolism to ornithine/urea or nitric oxide/citrulline: a life or death issue. Crit Rev Immunol. 2001;21(5):399–425.
- Wynn TA, Chawla A, Pollard JW. Macrophage biology in development, homeostasis and disease. Nature. 2013;496(7446):445–455.
- Mills CD, Kincaid K, Alt JM, et al. M-1/M-2 macrophages and the Th1/Th2 paradigm. J Immunol. 2000;164(12):6166–6173.
- Mantovani A, Sica A, Sozzani S, et al. The chemokine system in diverse forms of macrophage activation and polarization. Trends Immunol. 2004;25(12):677–686.
- Martinez FO, Helming L, Gordon S. Alternative activation of macrophages: an immunologic functional perspective. Annu Rev Immunol. 2009;27:451–483.
- Somigliana E, Vigano P, Vignali M. Endometriosis and unexplained recurrent spontaneous abortion: pathological states resulting from aberrant modulation of natural killer cell function? Hum Reprod Update. 1999;5(1):40–51.
- Oosterlynck DJ, Meuleman C, Waer M, et al. The natural killer activity of peritoneal fluid lymphocytes is decreased in women with endometriosis. Fertil Steril. 1992;58(2):290–295.
- Ho HN, Wu MY, Yang YS. Peritoneal cellular immunity and endometriosis. Am J Reprod Immunol. 1997;38(6):400–412.
- Ho HN, Chao KH, Chen HF, et al. Peritoneal natural killer cytotoxicity and CD25+ CD3+ lymphocyte subpopulation are decreased in women with stage III-IV endometriosis. Hum Reprod. 1995;10(10):2671–2675.
- Funamizu A, Fukui A, Kamoi M, et al. Expression of natural cytotoxicity receptors on peritoneal fluid natural killer cell and cytokine production by peritoneal fluid natural killer cell in women with endometriosis. Am J Reprod Immunol. 2014;71(4):359–367.
- Greenwood SM, Moran JJ. Chronic endometritis: morphologic and clinical observations. Obstet Gynecol. 1981;58(2):176–184.
- Kitaya K, Matsubayashi H, Yamaguchi K, et al. Chronic endometritis: potential cause of infertility and obstetric and neonatal complications. Am J Reprod Immunol. 2016;75(1):13–22.
- Polisseni F, Bambirra EA, Camargos AF. Detection of chronic endometritis by diagnostic hysteroscopy in asymptomatic infertile patients. Gynecol Obstet Invest. 2003;55(4):205–210.
- Cicinelli E, Resta L, Nicoletti R, et al. Detection of chronic endometritis at fluid hysteroscopy. J Minim Invasive Gynecol. 2005;12(6):514–518.
- Bayer-Garner IB, Korourian S. Plasma cells in chronic endometritis are easily identified when stained with syndecan-1. Mod Pathol. 2001;14(9):877–879.
- Smith M, Hagerty KA, Skipper B, et al. Chronic endometritis: a combined histopathologic and clinical review of cases from 2002 to 2007. Int J Gynecol Pathol. 2010;29(1):44–50.
- Gilmore H, Fleischhacker D, Hecht JL. Diagnosis of chronic endometritis in biopsies with stromal breakdown. Hum Pathol. 2007;38(4):581–584.
- Cicinelli E, De Ziegler D, Nicoletti R, et al. Chronic endometritis: correlation among hysteroscopic, histologic, and bacteriologic findings in a prospective trial with 2190 consecutive office hysteroscopies. Fertil Steril. 2008;89(3):677–684.
- Tortorella C, Piazzolla G, Matteo M, et al. Interleukin-6, interleukin-1β, and tumor necrosis factor α in menstrual effluents as biomarkers of chronic endometritis. Fertil Steril. 2014;101(1):242–247.
- Takebayashi A, Kimura F, Kishi Y, et al. The association between endometriosis and chronic endometritis. PloS One. 2014;9(2):e88354.
- Strowig T, Henao-Mejia J, Elinav E, et al. Inflammasomes in health and disease. Nature. 2012;481(7381):278–286.
- Lamkanfi M, Dixit VM. Mechanisms and functions of inflammasomes. Cell. 2014;157(5):1013–1022.
- Broz P, Pelegrin P, Shao F. The gasdermins, a protein family executing cell death and inflammation. Nat Rev Immunol. 2020;20(3):143–157.
- Davis BK, Wen H, Ting JP. The inflammasome NLRs in immunity, inflammation, and associated diseases. Annu Rev Immunol. 2011;29:707–735.
- Vandanmagsar B, Youm YH, Ravussin A, et al. The NLRP3 inflammasome instigates obesity-induced inflammation and insulin resistance. Nat Med. 2011;17(2):179–188.
- Ito M, Shichita T, Okada M, et al. Bruton’s tyrosine kinase is essential for NLRP3 inflammasome activation and contributes to ischaemic brain injury. Nat Commun. 2015;6:7360.
- Mulla MJ, Myrtolli K, Potter J, et al. Uric acid induces trophoblast IL-1β production via the inflammasome: implications for the pathogenesis of preeclampsia. Am J Reprod Immunol. 2011;65(6):542–548.
- Brien ME, Duval C, Palacios J, Boufaied I, et al. Uric acid crystals induce placental inflammation and alter trophoblast function via an IL-1-dependent pathway: implications for fetal growth restriction. J Immunol. 2017;198(1):443–451.
- Kohli S, Ranjan S, Hoffmann J, et al. Maternal extracellular vesicles and platelets promote preeclampsia via inflammasome activation in trophoblasts. Blood. 2016;128(17):2153–2164.
- Romero R, Xu Y, Plazyo O, et al. A role for the inflammasome in spontaneous labor at term. Am J Reprod Immunol. 2018;79(6):e12440.
- Lim R, Lappas M. NOD-like receptor pyrin domain-containing-3 (NLRP3) regulates inflammation-induced pro-labor mediators in human myometrial cells. Am J Reprod Immunol. 2018;79(4):e12825.
- Tersigni C, D’Ippolito S, Di Nicuolo F, et al. Recurrent pregnancy loss is associated to leaky gut: a novel pathogenic model of endometrium inflammation? J Transl Med. 2018;16(1):102.
- Shirasuna K, Karasawa T, Takahashi M. Exogenous nanoparticles and endogenous crystalline molecules as danger signals for the NLRP3 inflammasomes. J Cell Physiol. 2019;234(5):5436–5450.
- Shirasuna K, Usui F, Karasawa T, et al. Nanosilica-induced placental inflammation and pregnancy complications: different roles of the inflammasome components NLRP3 and ASC. Nanotoxicology. 2015;9(5):554–567.
- Duffy DM, Ko C, Jo M, et al. Ovulation: parallels with inflammatory processes. Endocr Rev. 2019;40(2):369–416.
- Wang LJ, Norman RJ. Concentrations of immunoreactive interleukin-1 and interleukin-2 in human preovulatory follicular fluid. Hum Reprod. 1992;7(2):147–150.
- Buyalos RP, Watson JM, Martinez-Maza O. Detection of interleukin-6 in human follicular fluid. Fertil Steril. 1992;57(6):1230–1234.
- Wang LJ, Brannstrom M, Robertson SA, et al. Tumor necrosis factor alpha in the human ovary: presence in follicular fluid and effects on cell proliferation and prostaglandin production. Fertil Steril. 1992;58(5):934–940.
- Jasper MJ, Brannstrom M, Olofsson JI, et al. Granulocyte-macrophage colony-stimulating factor: presence in human follicular fluid, protein secretion and mRNA expression by ovarian cells. Mol Hum Reprod. 1996;2(8):555–562.
- Witt BR, Pollard JW. Colony stimulating factor-1 in human follicular fluid. Fertil Steril. 1997;68(2):259–264.
- Nishimura K, Tanaka N, Kawano T, et al. Changes in macrophage colony-stimulating factor concentration in serum and follicular fluid in in-vitro fertilization and embryo transfer cycles. Fertil Steril. 1998;69(1):53–57.
- Nio-Kobayashi J, Kudo M, Sakuragi N, et al. Regulated C-C motif ligand 2 (CCL2) in luteal cells contributes to macrophage infiltration into the human corpus luteum during luteolysis. Mol Hum Reprod. 2015;21(8):645–654.
- Kryczek I, Frydman N, Gaudin F, et al. The chemokine SDF-1/CXCL12 contributes to T lymphocyte recruitment in human pre-ovulatory follicles and coordinates with lymphocytes to increase granulosa cell survival and embryo quality. Am J Reprod Immunol. 2005;54(5):270–283.
- Fainaru O, Amsalem H, Bentov Y, et al. CD56brightCD16- natural killer cells accumulate in the ovarian follicular fluid of patients undergoing in vitro fertilization. Fertil Steril. 2010;94(5):1918–1921.
- Castilla JA, Sampalo A, Molina R, et al. Mononuclear cell subpopulations in human follicular fluid from stimulated cycles. Am J Reprod Immunol. 1990;22(3–4):127–129.
- Arici A, Oral E, Bukulmez O, et al. Monocyte chemotactic protein-1 expression in human preovulatory follicles and ovarian cells. J Reprod Immunol. 1997;32(3):201–219.
- Al-Alem L, Puttabyatappa M, Rosewell K, et al. Chemokine ligand 20: a signal for leukocyte recruitment during human ovulation? Endocrinology. 2015;156(9):3358–3369.
- Ushigoe K, Irahara M, Fukumochi M, et al. Production and regulation of cytokine-induced neutrophil chemoattractant in rat ovulation. Biol Reprod. 2000;63(1):121–126.
- Das S, Bates MD, Vince GS, et al. Follicular fluid expression of alpha-defensins and their role in ovulation. J Assist Reprod Genet. 2008;25(2–3):83–87.
- Akiyama I, Yoshino O, Osuga Y, et al. The role of bone morphogenetic protein 6 in accumulation and regulation of neutrophils in the human ovary. Reprod Sci. 2014;21(6):772–777.
- Van der Hoek KH, Maddocks S, Woodhouse CM, et al. Intrabursal injection of clodronate liposomes causes macrophage depletion and inhibits ovulation in the mouse ovary. Biol Reprod. 2000;62(4):1059–1066.
- Dahm-Kahler P, Ghahremani M, Lind AK, et al. Monocyte chemotactic protein-1 (MCP-1), its receptor, and macrophages in the perifollicular stroma during the human ovulatory process. Fertil Steril. 2009;91(1):231–239.
- Wu R, Van der Hoek KH, Ryan NK, et al. Macrophage contributions to ovarian function. Hum Reprod Update. 2004;10(2):119–133.
- Yang Z, Kong B, Mosser DM, et al. TLRs, macrophages, and NK cells: our understandings of their functions in uterus and ovary. Int Immunopharmacol. 2011;11(10):1442–1450.
- Cohen-Fredarow A, Tadmor A, Raz T, et al. Ovarian dendritic cells act as a double-edged pro-ovulatory and anti-inflammatory sword. Mol Endocrinol. 2014;28(7):1039–1054.
- Dal J, Vural B, Caliskan E, et al. Power Doppler ultrasound studies of ovarian, uterine, and endometrial blood flow in regularly menstruating women with respect to luteal phase defects. Fertil Steril. 2005;84(1):224–227.
- Qublan H, Amarin Z, Nawasreh M, et al. Luteinized unruptured follicle syndrome: incidence and recurrence rate in infertile women with unexplained infertility undergoing intrauterine insemination. Hum Reprod. 2006;21(8):2110–2113.
- Wang L, Qiao J, Liu P, et al. Effect of luteinized unruptured follicle cycles on clinical outcomes of frozen thawed embryo transfer in Chinese women. J Assist Reprod Genet. 2008;25(6):229–233.
- Brannstrom M, Bonello N, Norman RJ, et al. Reduction of ovulation rate in the rat by administration of a neutrophil-depleting monoclonal antibody. J Reprod Immunol. 1995;29(3):265–270.
- Hock DL, Huhn RD, Kemmann E. Leukocytosis in response to exogenous gonadotrophin stimulation. Hum Reprod. 1997;12(10):2143–2146.
- Makinoda S, Hirosaki N, Waseda T, et al. Granulocyte colony-stimulating factor (G-CSF) in the mechanism of human ovulation and its clinical usefulness. Curr Med Chem. 2008;15(6):604–613.
- Shibata T, Makinoda S, Waseda T, et al. Granulocyte colony-stimulating factor as a potential inducer of ovulation in infertile women with luteinized unruptured follicle syndrome. Transl Res. 2016;171:63–70.
- Lizneva D, Suturina L, Walker W, et al. Criteria, prevalence, and phenotypes of polycystic ovary syndrome. Fertil Steril. 2016;106(1):6–15.
- Diamanti-Kandarakis E, Kandarakis H, Legro RS. The role of genes and environment in the etiology of PCOS. Endocrine. 2006;30(1):19–26.
- Escobar-Morreale HF, Luque-Ramírez M, González F. Circulating inflammatory markers in polycystic ovary syndrome: a systematic review and metaanalysis. Fertil Steril. 2011;95(3):1048–1058. e1–2.
- Boulman N, Levy Y, Leiba R, et al. Increased C-reactive protein levels in the polycystic ovary syndrome: a marker of cardiovascular disease. J Clin Endocrinol Metab. 2004;89(5):2160–2165.
- Peng Z, Sun Y, Lv X, et al. Interleukin-6 levels in women with polycystic ovary syndrome: a systematic review and meta-analysis. PloS One. 2016;11(2):e0148531.
- Seyam E, Hasan M, Khalifa EM, et al. Evaluation of tumor necrosis factor alpha serum level in obese and lean women with clomiphene citrate-resistant polycystic ovary disease. Gynecol Endocrinol. 2017;33(11):892–898.
- Cirillo F, Catellani C, Lazzeroni P, et al. HMGB1 is increased in adolescents with polycystic ovary syndrome (PCOS) and decreases after treatment with myo-inositol (MYO) in combination with alpha-lipoic acid (ALA). Gynecol Endocrinol. 2020;36(7):588–586.
- Williams EJ, Sibley K, Miller AN, et al. The effect of Escherichia coli lipopolysaccharide and tumour necrosis factor alpha on ovarian function. Am J Reprod Immunol. 2008;60(5):462–473.
- Herath S, Williams EJ, Lilly ST, et al. Ovarian follicular cells have innate immune capabilities that modulate their endocrine function. Reproduction. 2007;134(5):683–693.
- Granot I, Gnainsky Y, Dekel N. Endometrial inflammation and effect on implantation improvement and pregnancy outcome. Reproduction. 2012;144(6):661–668.
- Van Sinderen M, Menkhorst E, Winship A, et al. Preimplantation human blastocyst-endometrial interactions: the role of inflammatory mediators. Am J Reprod Immunol. 2013;69(5):427–440.
- Zhang YH, He M, Wang Y, et al. Modulators of the balance between M1 and M2 macrophages during pregnancy. Front Immunol. 2017;8:120.
- Gnainsky Y, Granot I, Aldo PB, et al. Local injury of the endometrium induces an inflammatory response that promotes successful implantation. Fertil Steril. 2010;94(6):2030–2036.
- Barash A, Dekel N, Fieldust S, et al. Local injury to the endometrium doubles the incidence of successful pregnancies in patients undergoing in vitro fertilization. Fertil Steril. 2003;79(6):1317–1322.
- El-Toukhy T, Sunkara S, Khalaf Y. Local endometrial injury and IVF outcome: a systematic review and meta-analysis. Reprod Biomed Online. 2012;25(4):345–354.
- Gibreel A, Badawy A, El-Refai W, et al. Endometrial scratching to improve pregnancy rate in couples with unexplained subfertility: a randomized controlled trial. J Obstet Gynaecol Res. 2013;39(3):680–684.
- Potdar N, Gelbaya T, Nardo LG. Endometrial injury to overcome recurrent embryo implantation failure: a systematic review and meta-analysis. Reprod Biomed Online. 2012;25(6):561–571.
- Kammerer U. Antigen-presenting cells in the decidua. Chem Immunol Allergy. 2005;89:96–104.
- Miyazaki S, Tsuda H, Sakai M, et al. Predominance of Th2-promoting dendritic cells in early human pregnancy decidua. J Leukoc Biol. 2003;74(4):514–522.
- Negishi Y, Kato M, Ono S, et al. Distribution of dendritic cells in the septate uterus: an immunological perspective. Am J Reprod Immunol. 2020;83(6):e13241.
- Venetis CA, Papadopoulos SP, Campo R, et al. Clinical implications of congenital uterine anomalies: a meta-analysis of comparative studies. Reprod Biomed Online. 2014;29(6):665–683.
- Ono Y, Nagai M, Yoshino O, et al. CD11c + M1-like macrophages (MΦs) but not CD206+ M2-like MΦ are involved in folliculogenesis in mice ovary. Sci Rep. 2018;8(1):8171.
- Jaiswal MK, Mallers TM, Larsen B, et al. V-ATPase upregulation during early pregnancy: a possible link to establishment of an inflammatory response during preimplantation period of pregnancy. Reproduction. 2012;143(5):713–725.
- Hamilton S, Oomomian Y, Stephen G, et al. Macrophages infiltrate the human and rat decidua during term and preterm labor: evidence that decidual inflammation precedes labor. Biol Reprod. 2012;86(2):39.
- Tessier DR, Yockell-Lelièvre J, Gruslin A. Uterine spiral artery remodeling: the role of uterine natural killer cells and extravillous trophoblasts in normal and high-risk human pregnancies. Am J Reprod Immunol. 2015;74(1):1–11.
- Osman I, Young A, Ledingham MA, et al. Leukocyte density and pro-inflammatory cytokine expression in human fetal membranes, decidua, cervix and myometrium before and during labour at term. Mol Hum Reprod. 2003;9(1):41–45.
- Wang E, Batey A, Struble C, et al. Gestational age and maternal weight effects on fetal cell-free DNA in maternal plasma. Prenat Diagn. 2013;33(7):662–666.
- Dubicke A, Andersson P, Fransson E, et al. High-mobility group box protein 1 and its signalling receptors in human preterm and term cervix. J Reprod Immunol. 2010;84(1):86–94.
- Sakamoto Y, Moran P, Bulmer JN, et al. Macrophages and not granulocytes are involved in cervical ripening. J Reprod Immunol. 2005;66(2):161–173.
- Norwitz ER, Caughey AB. Progesterone supplementation and the prevention of preterm birth. Rev Obstet Gynecol. 2011;4(2):60–72.
- Di Renzo GC, Rosati A, Mattei A, et al. The changing role of progesterone in preterm labour. BJOG. 2005;112 Suppl 1:57–60.
- Maeda A, Soejima K, Bandow K, et al. Force-induced IL-8 from periodontal ligament cells requires IL-1beta. J Dent Res. 2007;86(7):629–634.
- Hayakawa S, Karasaki-Suzuki M, Itoh T, et al. Effects of paternal lymphocyte immunization on peripheral Th1/Th2 balance and TCR V beta and V gamma repertoire usage of patients with recurrent spontaneous abortions. Am J Reprod Immunol. 2000;43(2):107–115.
- Visvanathan S, McNeil HP. Cellular immunity to beta 2-glycoprotein-1 in patients with the antiphospholipid syndrome. J Immunol. 1999;162(11):6919–6925.
- Hill JA, Polgar K, Anderson DJ. T-helper 1-type immunity to trophoblast in women with recurrent spontaneous abortion. JAMA. 1995;273(24):1933–1936.
- Ashkar AA, Di Santo JP, Croy BA. Interferon gamma contributes to initiation of uterine vascular modification, decidual integrity, and uterine natural killer cell maturation during normal murine pregnancy. J Exp Med. 2000;192(2):259–270.
- Shimada S, Kato EH, Morikawa M, et al. No difference in natural killer or natural killer T-cell population, but aberrant T-helper cell population in the endometrium of women with repeated miscarriage. Hum Reprod. 2004;19(4):1018–1024.
- Kosaka K, Fujiwara H, Tatsumi K, et al. Human chorionic gonadotropin (HCG) activates monocytes to produce interleukin-8 via a different pathway from luteinizing hormone/HCG receptor system. J Clin Endocrinol Metab. 2002;87(11):5199–5208.
- Fazleabas AT, Donnelly KM, Srinivasan S, et al. Modulation of the baboon (Papio anubis) uterine endometrium by chorionic gonadotrophin during the period of uterine receptivity. Proc Natl Acad Sci Usa. 1999;96(5):2543–2548.
- Kane N, Kelly R, Saunders PT, et al. Proliferation of uterine natural killer cells is induced by human chorionic gonadotropin and mediated via the mannose receptor. Endocrinology. 2009;150(6):2882–2888.
- Schumacher A, Brachwitz N, Sohr S, et al. Human chorionic gonadotropin attracts regulatory T cells into the fetal-maternal interface during early human pregnancy. J Immunol. 2009;182(9):5488–5497.
- Schumacher A, Heinze K, Witte J, et al. Human chorionic gonadotropin as a central regulator of pregnancy immune tolerance. J Immunol. 2013;190(6):2650–2658.
- Abramov Y, Schenker JG, Lewin A, et al. Plasma inflammatory cytokines correlate to the ovarian hyperstimulation syndrome. Hum Reprod. 1996;11(7):1381–1386.
- Matzuk MM, DeMayo FJ, Hadsell LA, et al. Overexpression of human chorionic gonadotropin causes multiple reproductive defects in transgenic mice. Biol Reprod. 2003;69(1):338–346.
- Takasugi N, Iguchi T, Kurihara J, et al. Changes in gonads of male and female offspring of mice receiving a continuous intravenous infusion of human chorionic gonadotropin during gestation. Exp Clin Endocrinol. 1985;86(3):273–283.
- Li C, Zhang Y, Cheng X, et al. PINK1 and PARK2 suppress pancreatic tumorigenesis through control of mitochondrial iron-mediated immunometabolism. Dev Cell. 2018;46(4):441–455 e8.
- Kazama H, Ricci JE, Herndon JM, et al. Induction of immunological tolerance by apoptotic cells requires caspase-dependent oxidation of high-mobility group box-1 protein. Immunity. 2008;29(1):21–32.
- Zabini D, Crnkovic S, Xu H, et al. High-mobility group box-1 induces vascular remodelling processes via c-Jun activation. J Cell Mol Med. 2015;19(5):1151–1161.