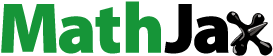
ABSTRACT
Salt-affected soils are a global challenge, affecting 1 billion ha of land, with 200 million ha found in Africa. The challenge brings adverse impacts on agricultural productivity, food security, environmental sustainability, and food security. In Tanzania, more than 2 million ha of land are salt-affected, of which 1.7 million ha are saline soil and 0.3 million ha are sodic soil. To cope with this threat, it is necessary to have a thorough understanding of its extent (coverage), existing types, and available management strategies. This review presents a comprehensive account of the challenges and opportunities of salt-affected soils in Tanzania and examines management options that have been observed to increase agricultural productivity in rice-growing areas. A systematic review of relevant articles published in databases was carried out using PRISMA guidelines and flowcharts. This review highlights the origin, extent, types, and various techniques for alleviating salt-affected soil problems. It also emphasize on the use of inorganic and organic amendments, salt-tolerant varieties, irrigation water quality, and drainage infrastructure. We revealed that farmers, use burned and unburned rice husks, sawdust, gypsum, and farm yard manure (FYM) as copping mechanisms. Furthermore, there have been continuing efforts to develop salt-tolerant rice varieties, coupled with maintenance of irrigation infrastructure and site-specific soil management options, as appropriate solutions to tackle salt issues. Given the light of existing data, the review recommends using RS and GIS for updating information on salt-affected soils, particularly in irrigated areas, as an essential component of sustainable management and preventing further loss of agricultural land.
Introduction
Background information
The global estimates of salt-affected soils from various sources indicate that around 1 billion hectares of land are affected on all continents (Ivushkin et al., Citation2019; Wicke et al., Citation2011), with hotspots in India, Pakistan, China, the United States, Argentina, Sudan, and Central and Western Asia (Butcher et al., Citation2016). Salt-affected soils are characterized by having either high contents of soluble salts or substantial amounts of exchangeable sodium ions, which have a direct impact on the growth and sustainability of most plants (Shahid et al., Citation2018; Wallender & Kenneth, Citation2012). Such soils are mostly found in arid and semiarid regions of the world, which are characterized by evaporation and evapotranspiration that exceed precipitation (Amini et al., Citation2016; Butcher et al., Citation2016; Corwin & Scudiero, Citation2019; Omuto et al., Citation2020). Salt-affected soils contain major cations of sodium (Na+), potassium (K+), calcium (Ca2+), and magnesium (Mg2+), and anions of chloride (Cl−), sulfate (SO42-), bicarbonate (HCO3−), carbonate (CO32−), and nitrate (NO3−) (P. Kumar & Sharma, Citation2020; Munns et al., Citation2020; Shahid et al., Citation2018; Wallender & Kenneth, Citation2012; Wallender & Kenneth, Citation2012; C. Zhao et al., Citation2020; Zörb et al., Citation2019; Zhao et al. The harmful effect of soil salinization varies depending on climatic and soil conditions, light intensity, and the ability of plant species to grow and tolerate the environment (Acosta-Motos et al., Citation2017).
The estimates predict that 50% of arable land around the world will become impacted by salt-affected soils by 2050, consequently increasing food insecurity (Butcher et al., Citation2016), hence making soil salinization increase a serious global threat to agricultural production (Butcher et al., Citation2016; Corwin & Scudiero, Citation2019). The prevailing climate change is likely to intensify the effect of salt-affected soils (Corwin & Scudiero, Citation2019; Eswar et al., Citation2021; Wen et al., Citation2021), leading to dramatic impacts on crop growth and productivity and consequently reducing yield by more than 50%, especially in arid and semi-arid regions (Anami et al., Citation2020; Ivushkin et al., Citation2019). Thus, in light of the expected enormous growth in the global population, salt-affected soils are one of the key challenges to food production (Chen & Mueller, Citation2018; Egal, Citation2020; Wen et al., Citation2021; Zörb et al., Citation2019).
In sub-Saharan Africa, soil salinization affects 19 million ha of land (Tully et al., Citation2015), whereas in Tanzania, over 3 million ha of land are affected (FAO, Citation2000). Research conducted by Kashenge-Killenga et al. (Citation2016) in some irrigation schemes indicated that 7–15 percent of the schemes had salt-affected soils and that about 2–10 percent of rice fields were abandoned. Furthermore, Kashenge-Killenga et al. (Citation2016) and Meliyo et al. (Citation2016) reported high values of electrical conductivity (EC) ranging from 14 to 19 dSm−1 in various irrigation schemes. Consequently, the average yield losses ranged from 5 to 70 percent (Kashenge-Killenga et al., Citation2016), while in extreme cases the yield losses were up to 100 percent. To address the effect of salt-affected soils on cash and food crop production, stakeholders’ awareness of the problem and the most effective management options deserve global attention.
To date, different approaches have been proposed for the prevention, mitigation, and reclamation of salt-affected soils, including the use of organic and microbial soil remediation (Amini et al., Citation2016; Gunarathne et al., Citation2020; H. Sun et al., Citation2017; Leogrande & Vitti, Citation2019; Saifullah Dahlawi et al., Citation2017; U. B. Singh et al., Citation2021); breeding for salt-tolerant varieties for different crops (Dehnavi et al., Citation2020; Kamran et al., Citation2020; Sunita et al., Citation2020), engineering and water serving technologies (Mohanavelu et al., Citation2021), precision application of chemicals and fertilizers (El Hasini et al., Citation2019; G. Qadir et al., Citation2017; Gangwar et al., Citation2019), and phytoremediation (A. Kumar et al., Citation2020; Nouri et al., Citation2017). However, most of these technologies are quite expensive and unaffordable for farmers in rural areas. Hence, in order to effectively manage salt-affected soils, a combination of technologies is required (Butcher et al., Citation2016), so that farmers can choose the technology that best suits their needs.
Though salt-affected soils have significant negative impacts on agricultural production by turning productive lands into barren grounds and spoiling water quality and ecosystem services, research results show that if such soils are properly managed, they are highly productive with significant economic potential (G. Qadir et al., Citation2017; Stavi et al., Citation2021; Wicke et al., Citation2011). The economic potential of salt-affected soils can only be realized if the extent and characteristics of such soils are well established to allow the proper selection of technologies for their effective management options. However, in Tanzania in particular, the information on the extent and characteristics of salt-affected soils is scanty and scattered (Butcher et al., Citation2016; Ivushkin et al., Citation2019), thus posing a challenge to how to effectively manage such soils for sustainable agricultural production. Therefore, this systematic literature review was conducted to explore the currently available information on salt-affected soils in Tanzania, including their extent, types, origin, and distribution, and what have been the coping strategies for sustainable farming on such soils in both irrigated and rainfed agro-ecologies.
Rational of the review
Soil salinization has become a serious challenge in sustainable agriculture for food and nutritional security in developing countries (A. Singh, Citation2021; Butcher et al., Citation2016). The global reports estimate the extent of salt-affected soils to be around 1 billion ha, whereas the information down to individual countries is not well documented (Ivushkin et al., Citation2019; Omuto et al., Citation2020). Tanzania inclusive, the data is more significant for planning and management strategies. The problem of salt-affected soils is an increasing concern in Tanzania, like in other parts of the world, especially in rice-growing areas (Kashenge-Killenga et al., Citation2013, Citation2016; Makoi & Ndakidemi, Citation2007; Meliyo et al., Citation2016). Although the negative impacts of salt-affected soils on farmers fields have been reported, including loss of crop yields and the abandonment of arable land in some areas after becoming unproductive, the information is not well documented (Kashenge-Killenga et al., Citation2016). Thus, the need to meet increasing food demand for the growing population under condition of declining agricultural land necessitates prioritizatio of research on salt-affected soils (P. Kumar & Sharma, Citation2020) and effective management options for increasing crop production.
Knowledge and a comprehensive understanding of the current information are the way forward toward the alleviation of this challenge, thereby increasing productivity and food security (Shokat & Großkinsky, Citation2019). Therefore, it is very important to have real-time data about the extent, distribution, and types of salt-affected soils to support and guide informed decision-making to improve agricultural production in Tanzania. This review presents the current status of the available information and existing gaps and proposes a way forward that guides research priorities targeting the development of salt-affected soil management options for improved crop production, specifically in rice irrigation schemes. In this regard, conducting a review of the pertinent scholarly literature is crucial for assessing existing knowledge and identifying research gaps in a particular area of research interest, which helping to expand the knowledge base (Snyder, Citation2019).
Methodology adopted for the review
Search strategy, keywords and criteria of selection
The work adopted the Preferred Reporting Items for Systematic Reviews and Meta-Analyses (PRISMA) framework, an approach that demonstrates a clear path, guarantees scientific quality, and ensures transparency for conducting a systematic review (Grant & Booth, Citation2009; Page et al., Citation2021). Several systematic review flow charts have been developed and adopted to conduct systematic review and meta-analysis, including SALSA (search, appraisal, synthesis, analysis) by Grant and Booth (Citation2009), the Protocol, Search, Appraisal, Synthesis, Analysis, and Report (PSALSAR) framework by Mengist et al. (Citation2020), and the PRISMA approach by Moher et al. (2010). This review opted to follow a modified PRISMA flowchart, which is a widely accepted checklist designed to improve the reporting standards of comprehensive literature reviews and meta-analyses (Jafarzadeh et al., Citation2021). As shown in Figure , it involves four phases: identification, screening, eligibility, and inclusion. The paper used nine (9) search engines, namely Elsevier, MDPI, Frontiers, Global Science, Springer, Wiley, Taylor & Francis, PubMed, and Web Research Gate, to download and collect published articles from peer-reviewed journals. In areas with limited published works, unpublished reports that had relevant information were also considered. Keywords were used in searching papers to minimize unwanted papers and avoid the risk of missing relevant papers. Broad themes were also used, covering aspects such as the origin of salt-affected soils, the extent of salt-affected soils, the distribution of salt-affected soils, management options for salt-affected soils, the challenges and opportunities of salt-affected soils, and the economic implications of salt-affected soils.
Figure 1. Systematic review pathway based on the PRISMA approach, modified by Page et al. (Citation2021).

Generally, the search identified a total of 419 journal papers listed in search engines and 5 reports. Out of 419 articles collected, 56 were duplicates and were removed. Further screening was done for the remaining 363 papers, and the 173 papers were rejected because the titles, abstracts, and texts of the articles did not directly match the target of the review. Further screening was done, and 57 articles out of the remaining 190 were removed for having no direct relevant information in the main text. Finally, 133 articles and one report were used to examine and discuss various aspects of salt-affected soils in Tanzania. Figure illustrates the flow of the review from search sites to the final decision for articles used in this review using the PRISMA approach (Grant & Booth, Citation2009; Page et al., Citation2021).
Results and discussion
Origin of salt-affected soils
The genesis of salt-affected soils occurs through natural processes influenced by salty parent materials, mineralogy, groundwater, windblown salt particles, and floods (Omuto et al., Citation2020; Stavi et al., Citation2017). While human-induced activities such as irrigation (with poor drainage or irrigation with saline water), inappropriate fertilizer application, improper waste disposal, inappropriate use of wastewater, misuse of soil amendments, inappropriate soil-water management, and land-use change (Amini et al., Citation2016; Shahid et al., Citation2018; Stavi et al., Citation2021). Whether natural or human-induced soil salinization emerges as a major environmental constraint impeding soil productivity, agricultural sustainability, and food security, particularly in arid and semi-arid regions of the world (Chiconato et al., Citation2019; Corwin & Scudiero, Citation2019; Mukhopadhyay et al., Citation2021; Shrivastava & Kumar, Citation2015).
Spatial distribution, extent, types, and the causes of salt-affected soils in Tanzania
Spatial distribution, extent, and types of salt-affected soils in Tanzania were reported by Mnkeni (Citation1996), who indicated that 3.6 million ha of land had salt problems, of which 83% were saline and 16% were sodic. The occurrence of these soils is most extensive in areas with arid and semi-arid climates and in coastal regions where there is an incursion of sea water through estuaries and rivers. Additionally, in inland areas, shallow groundwater influences are also present. On the other hand, FAO (Citation2000) estimated that over 1.7 million ha were saline, whereas 300,000 ha were sodic. According to Mnkeni (Citation1996), salt-affected soils mainly occur in alluvial plains and valleys that receive soils influenced by the nature of the parent materials eroded by surface runoff or colluvial from highland areas. In the northern highlands, water sources of the Pangani river run within and over the volcanic lava and ashes of Kilimanjaro and Meru mountains, eroding salt materials and overflowing in flat areas (Mnkeni, Citation1996). Salt-affected soils in the internal drainage basin and swamps like those in the central zone regions and within the rift valley were due to long-term collection of water, poor drainage (Mnkeni, Citation1996), and higher evapotranspiration than precipitation.
Recently, climate change has been perceived as one of the contributing factor that accelerates the problem of salt-affected soils in Tanzania (Kashenge-Killenga et al., Citation2016). This is evidenced by the experiences of increasing drought and the decreasing length of the growing seasons caused by poor distribution of rainfall patterns where evapotranspiration exceeds precipitation (Kashenge-Killenga et al., Citation2016). Besides natural factors, the problem of salt-affected soils is being intensified by management factors, including inadequate irrigation infrastructure and poor agricultural practices (Meliyo et al., Citation2016; Omar et al., Citation2022). For example, there is a lack of farm inlets and outlet canals that encourage farmers to share irrigation water across farm boundaries. While both natural and management factors are contributing to intensifying salt-affected soils, the current extent and spatial distribution of salt-affected soils remain a challenge due to a lack of up-to-date information (Kashenge-Killenga et al., Citation2014). Figure indicates the distribution of salt-affected soils in Tanzania (De Pauw, Citation1984).
Figure 2. Spatial Distribution of Salt-affected Soils in Tanzania (De Pauw, Citation1984).

Classification and general properties of salt-affected soils
Salt-affected soils are classified as saline, sodic, and saline-sodic soils based on their chemical and physical properties (Table ), which are the electrical conductivity of saturated paste extract (ECe), pH, the calculated exchangeable sodium percentage (ESP), and the sodium adsorption ratio (SAR) (Wallender & Kenneth, Citation2012). The saline soils are the ones with excess accumulation of soluble salts in the root zone and have high ECe values (>4 dSm−1). Sodic soils, on the other hand, are characterized by the accumulation of high levels of exchangeable sodium relative to other cations, hence with SAR>13 and/or ESP>15% (Cuevas et al., Citation2019; Hassani et al., Citation2020). On the other hand, saline-sodic soils are characterized by having both highly soluble salts and exchangeable sodium, ECe>4 dSm−1, SAR>13, and ESP>15% (Wallender & Kenneth, Citation2012).
Table 1. Classification of salt-affected soils based on ECe, ESP, SAR and pH values
Saline soils
Saline soils contain high levels of soluble salts in the soil solution, enough to cause adverse effects on the growth of most crops. The dominant cations in saline soils are calcium (Ca2+), magnesium (Mg2+) and/or potassium (K+), as well as anions of chlorides (Cl−), sulphates (SO42−), and carbonates (CO2−) and bicarbonates (HCO3−) (Richards & USDA, Citation1954; Wallender & Kenneth, Citation2012). Although NaCl dominates, nitrates may be present on a few occasions (Osman, Citation2018). An accumulation of soluble salts in the root zone alters the pH and composition of soil solution and interferes with plant absorption processes (osmosis/osmotic pressure) of water and essential nutrients, causing imbalances and deficiencies (Akhatar, Citation2019; C. Zhao et al., Citation2020). Plants growing on saline soils are forced to activate different physiological and biochemical mechanisms that help them cope with the resulting stress, including changes in morphology, anatomy, photosynthesis, water relations, toxic ion distribution, and biochemical adaptation (A. Kumar & Bandhu, Citation2005; Rahneshan et al., Citation2018). The primary effect induced in plants by excessive soluble salts is to limit the ability of plant roots to absorb soil water, even under wet soil conditions (Munns et al., Citation2020). Soil water flows from a higher osmotic potential (low salt concentration) in plant cells to a low osmotic potential (high salt concentration) in the soil solution, causing symptoms like a drought effect (Munns et al., Citation2020). With the high concentration of soluble salts, the ratio of Na+ to the major dominant ions, Cl− and SO42− is less than 1 (Table ). The preponderance of chloride and sulphate ions gives saline soils a brownish-white appearance (Wallender & Kenneth, Citation2012).
Table 2. Characterization of salt-affected soils based on exchangeable ions
Saline soils have a good soil structure because excess soluble salts keep the clay particles in a flocculated state, which helps the particles cling together and initiate binding into aggregates that are usually porous and permeable (Wallender & Kenneth, Citation2012). Saline soils are the dominant salt-affectesoil class in Tanzania, although their curent extent and distribution are not very clear
Sodic soils
Sodic soils are denoted by a high concentration of exchangeable sodium ions (Na+) in the soil exchange complex, where sodium ions (Na+) compete with calcium (Ca2+), potassium (K+), and other cations and occupy more than 15% of the soil cation exchange capacity (CEC) (Richards & USDA, Citation1954). Sodium replaces other cations in the exchange complex, causing the dispersion of soil particles (Wallender & Kenneth, Citation2012), because the monovalent sodium cation has a weaker binding force compared to the divalent cations of calcium (Ca2+) and magnesium (Mg2+). The dominant cations in sodic soils are sodium (Na+), and the dominant anions are carbonate (CO32−), bicarbonate (HCO3−), and/or chloride (Cl−), and sulphate (SO42−) (Richards & USDA, Citation1954). The major challenge caused by excessive sodium is damage to the soil structure, accompanied by an increase in the compactness of soils and a decrease in infiltration, hydraulic conductivity, and oxygen availability in the root zone (Wallender & Kenneth, Citation2012). A high concentration of sodium increases soil pH (alkalization) through the presence of HCO3− and CO32− (Leogrande & Vitti, Citation2019; Shahid et al., Citation2018).
The forces that bind clay particles together are greatly weakened when excessive sodium comes between them, causing clay particles to expand, swell, and disperse (Wallender & Kenneth, Citation2012). The soil dispersion reduces the stability of soil aggregates, with the consequence of accelerating soil erosion (Gangwar et al., Citation2019). Sometimes sodicity is formed as a secondary effect where leaching has washed away soluble salts into the subsoil and left sodium bound to the negative charges of the clay (Wallender & Kenneth, Citation2012). Soil sodicity has detrimental effects on water permeability and soil aeration, water holding capacity, root penetration, seedling emergence, and microbial communities and their activity (Akhatar, Citation2019; Irakoze et al., Citation2021; Sheoran et al., Citation2021). Due to low soluble salts but high exchangeable sodium, the ratio of sodium ions to the sum of major anions is greater than 1 in sodic soils (Chhabra, Citation2005).
The extent of salt-affected soils reported by Mnkeni (Citation1996) and FAO (Citation2000) indicated a lower proportion of saline-sodic soil compared to saline and sodic soils in Tanzania. This is most likely because saline-sodic soils can turn into sodic soils after washing or leaching of soluble salts if sodium amendments are not made, thereby increasing the extent of sodic soils (Abate et al., Citation2021; C. Zhao et al., Citation2020).
Saline-sodic soils
Saline-sodic soils are the group of salt-affected soils having a combined effect of both salinity and sodicity (Alcívar et al., Citation2018; Wallender & Kenneth, Citation2012). These are considered to be highly degraded in terms of soil physical, chemical, and biological properties and hence least productive (Chaganti et al., Citation2015). Saline-sodic soils are more challenging in reclamation because separate treatments are required for both salinity and sodicity (Alcívar et al., Citation2018; Osman, Citation2018). The dominant cations in saline-sodic soils are sodium (Na+), calcium (Ca2+), magnesium (Mg2+), and/or potassium (K+), while dominant anions are chloride (Cl−), sulphate (SO42−), bicarbonate (HCO3−) and nitrate (NO3−) (Richards & USDA, Citation1954). The proportionally high concentration of soluble salts (saline-sodic soil) keeps the colloids flocculated and well aggregated, but careless leaching of saline-sodic soils may cause dispersion, accompanied by degradation of soil structure and loss of water permeability (Osman, Citation2018), and the turning of saline-sodic soil into sodic soil. These soils are reported to be of limited extent in Tanzania, but they are also difficult to distinguish from sodic and saline soils by most soil users. This justifies the need to update the characterization of salt-affected soils in Tanzania.
Management of salt-affected soils
In order to ensure the sustainable reclamation of salt-affected soils, management options should be developed based on the type of salt-affected soil, degree of effect, and availability of site-specific amendment materials that are affordable by the farmers. Several advocated best-bet options can reverse salinity, sodicity, and saline-sodic effects in soils. However, most of the options take time to address the problems, and in every case, they are expensive. The best solutions to be used are dictated by the type of salt-affected soils and their gravity. The choice also depends on whether one is working in irrigation schemes with canals or on non-irrigated soils without canals. Depending on the situation, improving the efficiency of irrigation canals and drainage canals, capturing and treating salty drained water, and increasing the amount of water that gets into aquifers are prerequisites for the effective management of salt-affected soils. Use of soil amendments that improve infiltration and mulching to save soil water from evaporation losses can also help to manage the detrimental effects of salt-affected soils on crops. Details of best-bet options for the management of salt-affected soil are presented below.
Organic remediation
Research in various parts of the world shows that various forms of organic soil amendments, such as biochar, green manure compost, and municipal solid waste compost, improve the physical, biochemical, and microbial properties of salt-affected soils. These amendments can be used individually or in combination for the reclamation of degraded soils, especially salt-affected soils (El Hasini et al., Citation2019; M. Huang et al., Citation2019; Sheoran et al., Citation2021). Other researchers reported the combined application of green waste compost, sugarcane compost, and gypsum to reclaim saline soils (Diacono & Montemurro, Citation2015; El Hasini et al., Citation2019; Leogrande & Vitti, Citation2019). The literature further shows that organic matters stimulate nitrogen mineralization in salt-affected soils by increasing organic matter solubilization (X. W. Wang et al., Citation2019). For example, Y. P. Singh, Mishra, et al. (Citation2019) reported that the application of vermicompost at 10 t ha−1 saved 25% N without a significant reduction in the grain yield of rice and wheat in sodic soils.
In recent years, the application of biochar as an amendment for salt-affected soils has been reported and has attracted considerable attention from several researchers (A. Yang et al., Citation2020; Bin Yousaf et al., Citation2022; Kul et al., Citation2021; M. Singh et al., Citation2022; Phuong et al., Citation2020; Saifullah Dahlawi et al., Citation2017; Y. Sun et al., Citation2022), with emphasis on the improvement of the physical, chemical, and biological properties of salt-affected soils. Though numerous researchers reported positive results using biochar as an amendment for salt-affected soils, the efficacy is controlled by various factors such as temperature, feedstock, soil salt types, and biotic interactions (Almutairi et al., Citation2023; Freitas et al., Citation2020; H. Huang et al., Citation2021; Ippolito et al., Citation2020). The combined effects of these factors have an impact on the quality and performance of biochar (Kavitha et al., Citation2018). The high cost of production and high application rate are among the major constraints of using biochars for the amelioration of salt-affected soils (Saifullah Dahlawi et al., Citation2017). Organic amendments are therefore the solution for organic farming to improve soil physicochemical properties, enhance crop yield, and reduce greenhouse gas emissions (Amini et al., Citation2016; Fidel et al., Citation2019; Gunarathne et al., Citation2020; Kammann et al., Citation2017; Kubaczyński et al., Citation2020). Moreover, organic amendments help to improve soil aggregate formation and stability by binding the small particles in saline-sodic and sodic soils to form large, water-stable aggregates (Diacono & Montemurro, Citation2015). Table summarizes some of the reported amendment materials and their application rates across several countries.
Table 3. Summary of reviewed amendments used to manage salt-affected soils in different countries
In addition, biochar may reduce salt injury through the following three mechanisms: (1) transient binding of sodium (Na+) on its exchange sites, which reduces Na+ uptake; 2) rising potassium (K+) in soil solution, which maintains the Na+/K+ ionic balance, hence preventing Na+ uptake; and 3) improving water retention, thus rising soil moisture content, which causes dilution effects and hence reduces Na+ uptake (A. Yang et al., Citation2020; Abo Elyousr et al., Citation2022; Ali et al., Citation2021; Ketehouli et al., Citation2019; Mehmood et al., Citation2020). Figure illustrates the mechanisms.
Regardless of the good results reported on the use of organic amendments in the management of salt-affected soil, there is limited information available for Tanzania. Meliyo et al. (Citation2016) recommended 3–8 tons/ha of farmyard manure (FYM) to boost soil organic matter and improve the infiltration rate, thereby accelerating the leaching of soluble salts down the root zone on clay soil. According to Makoi and Ndakidemi (Citation2007), the use of FYM at 25 t ha−1 was adequate to improve the infiltration rate and available water capacity of sodic soils. However, the majority of small-scale farmers may not be able to obtain recommended quantities of FYM for salt-affected soil management unless alternative organic sources are considered (Makoi & Ndakidemi, Citation2007). Moreover, research results (Meliyo et al., Citation2016), at Ndungu Irrigation Scheme, Same District, show that locally available organic amendments like rice husk, rice straw, and sawdust incorporated in salt-affected soils 45 days before flashing are effective in improving soil infiltration, ECe, and yield comparable to gypsum. It was found that ECe dropped from 14 dS/m to less than 4 dS/m, which is appropriate for rice and many other crops, and this was attributed to improved infiltration that allowed proper flashing out of salts from the root zone.
Irrigation water quality and drainage infrastructure
The quality of irrigation water plays a vital role in sustaining the productivity of agricultural land (Awan et al., Citation2021; Prasad et al., Citation2022). It is common for irrigation water to contain some amount of salts; thus, depending on the frequency of irrigation and drainage conditions, salts brought into the field with irrigation water can accumulate over the soil surface with time (Mohanavelu et al., Citation2021). Based on global estimates, more than 20% of the irrigated area is affected by salinization induced by irrigation practices (M. Qadir et al., Citation2014). Numerous resources and strategies, including the use of non-saline or less-saline water for irrigation and the development of proper drainage facilities (artificial drainage), have been highlighted to reduce soil salinization in irrigation schemes (Liu et al., Citation2019; Mohanavelu et al., Citation2021). In most cases, the source of irrigation water is surface water, which along the pathway can dissolve salts from parent materials and transfer them to accumulate in the irrigation schemes (Shouse et al., Citation2010). Soluble salts can accumulate over the soil surface if the internal drainage is insufficient to drain the salts that are brought in with irrigation water (Connor et al., Citation2012). Among the management options for removing access salts from the topsoil layer is leaching with non-saline water. But if the water table is near the surface, like in humid and sub-humid areas, the leached salts can percolate below the plant root zone and lead to contamination of groundwater (Shahid et al., Citation2018).
In many cases, irrigation water in Tanzania is of good quality either without or with little dissolved salts at acceptable levels (Kashenge-Killenga et al., Citation2016). However, most irrigation schemes are found on the foot slopes of the Rift Valley Escarpment, which are characterized by high temperatures, most often on leeward sides, and hence unreliable or low precipitations. This is the case for all irrigation schemes in the Usangu plains, Lake Rukwa valley, Korogwe district in Tanga region, and Kilimanjaro region. Other areas are hinterland depressions or valleys equally characterized by high temperatures and low precipitation, including the Singida, Tabora, and Shinyanga irrigation schemes. These areas are characterized by poor leaching due to high water tables just near the soil surface, making salts accumulate over the surface. The problems are aggravated by poor irrigation infrastructure (canals without lining) and old canals without adequate renovation and close supervision (Meliyo et al., Citation2016). Nearly 90 percent of irrigation schemes had inadequate irrigation infrastructures, which increases the possibility of spreading salt concentration (Kashenge-Killenga et al., Citation2016). Unfortunately, the country lacks up-to-date information on the extent of salt-affected soils in irrigated areas, so one can hardly specify the actual contribution of poor irrigation water quality and drainage infrastructures on soil salinization.
Planting salt tolerant crops
In salt-affected soil environments, salt-tolerant crops are an alternative coping mechanism. The ability of plants to grow and complete their life cycle in a harsh environment containing high concentrations of soluble salt is one of the ways to manage salt-affected soils (Irakoze et al., Citation2021; Manuel et al., Citation2017). Salt-tolerant crop varieties have been developed to sustain growth under osmotic effects, ion toxicities, and nutrient imbalance conditions (Manuel et al., Citation2017; Zhang & Dai, Citation2019). The osmotic effect induces metabolic changes in the plant like those caused by water stress ‘wilting’ induced by drought (Chiconato et al., Citation2019; Munns & Gilliham, Citation2015). Some important sereal crops varieties (Table ) tolerant to various levels of salt concentration, have been released but their tolerance varies with weather growth stages and crop species (Farooq et al., Citation2017; Liang et al., Citation2017; Phogat et al., Citation2018). Maas (Citation1993) developed a linear model to estimate a yield loss under the unit-increased salinity level beyond the threshold. The model explained that for any given crop, the relative yield loss (Yt) for soil salinities exceeding the threshold can be estimated using the following equation:
Table 4. Selected cereal crops released for salt-tolerance
Where Yt is the relative yield; a is the salinity threshold expressed in (dS/m); b is the slope that represents the percentage of yield expected to be reduced for each unit of added salinity above the threshold; and ECe is the mean electrical conductivity of a saturated soil extract taken from the root zone.
Crop plants are more sensitive to salts during the early growth stages (at germination and seedling stages), but the degree of sensitivity differs critically between species and is more similar among the cultivars within similar species (Farooq et al., Citation2017). Crops naturally develop a large number of physiological and biochemical strategies to cope with salt stresses (Sharma et al., Citation2016; W. Wang et al., Citation2003; Zörb et al., Citation2019), but differ in their threshold levels (C. Zhao et al., Citation2020). Table shows some cereal crops released for salt tolerance along with their tolerance level.
Salt-tolerant rice varieties, such as SATO 1 and SATO 9 (Kashenge-Killenga et al., Citation2016), were developed and released in 2016 as part of a soil salinity management strategy and can grow in a salt-affected soil environment. However, seed availability and adoption by the farmer are still slow because, although the variety is high yielding and grows well under salinity, it is a non-aromatic one, which is not in high demand in local markets. Additionally, the majority of farmers in Tanzania prefer rice varieties with high yield, early maturity, aromatic flavor, and hence high market value (Suvi et al., Citation2020). Thus, a rice breeding program must focus on meeting farmers’ preferred traits to accelerate the adoption rate of newly released varieties. It is also important to caution that even salt-tolerant rice varieties have a threshold that they can cross, and hence the development of salt-affected soil reclamation technologies and/or integrated management options remains an important undertaking.
Inorganic amendments
Inorganic (chemical) amendments such as gypsum (CaSO4) which provide a source of calcium (Ca2+) that replaces the exchangeable sodium (Na+) on the exchangeable sites, are commonly used to reclaim alkaline (sodic) soils. Gypsum application is one of the best-known methods for the reclamation of alkaline (sodic) soils (Bello et al., Citation2021; Lastiri-Hernández et al., Citation2019). In alkaline soil, Ca2+ can replace a substantial amount of the exchangeable Na+ from the exchange site, and subsequently, Na+ combine with SO42- to generate soluble salts that are leached out of the root zone via an irrigation water system (Sarwar et al., Citation2011). The ionic exchange reaction at the soil exchange site in alkaline soil is shown in Eq. (1). This reaction improves soil stability and permeability (i.e. increases soil porosity, oxygen availability, and infiltration capacity) while decreasing soil pH, EC, and ESP (Alcívar et al., Citation2018; Sarwar et al., Citation2011; Y. P. Singh, Mishra, et al., Citation2019). It also stimulates soil microbial activity and increases nutrient availability (Kim et al., Citation2018). For gypsum to be effective in the amelioration of salt-affected soil, adequate water should be available to irrigate the soil after gypsum application to enhance the quick leaching out of displaced Na+ from the rhizosphere (Bello et al., Citation2021).
Globally, several reclamation techniques have been recommended to reduce the negative impact of salt-affected soils by improving physical, chemical, and biological soil properties. However, limited information is available in Tanzania, where little research has been conducted to develop area-specific recommendations on the application rates for inorganic amendments. The study conducted by Makoi and Ndakidemi (Citation2007) revealed that the combination of farmyard manure (FYM) and gypsum at rates of 25 t ha−1 and 12.5 t ha−1 significantly reduced EC, ESP, and increased infiltration rate compared to the treatment of FYM and gypsum alone in sodic soil. Furthermore, it has been demonstrated that the integrated use of gypsum and various organic amendments improved the quality and productivity of alkaline (sodic) and saline-sodic soils through changes in soil properties (Alcívar et al., Citation2018; Bello et al., Citation2021).
Land and soil resource management practices for reclamation of salt-affected soils
Salt scraping
The scraping method involves removing the salt crust that has accumulated on the soil surface, particularly in saline-affected land (Endo & Kang, Citation2015). Salt scraping can be used to remediate saline soils if the dynamics of salt precipitation are taken into account (Shaygan & Baumgartl, Citation2022). Evaporation is the main factor influencing the upward movement of saltwater to the surface of the soil, while high rainfall events can leach salts deep into the soil profile (Shaygan & Baumgartl, Citation2022). The effectiveness of this method requires that the leaching process be followed to remove the remaining soluble salts (Endo & Kang, Citation2015).
Soil ripping
In most cases, sodic and saline-sodic soils have dispersive behavior (Shaygan & Baumgartl, Citation2022), which results in low soil porosity with massive structure and high soil strength, especially when dry, which prevents root extension; a low infiltration rate, resulting in waterlogging or perched water tables, which drowns roots; and poor movement of air into the subsoil, resulting in low oxygen availability to growing plants. In this situation, ripping technology can be used as a means to reduce soil compaction and accelerate salt leaching (Mageshen et al., Citation2022). It is, however, recommended to combine ripping with organic and inorganic amendments for long-term improvements in soil physical conditions (Endo & Kang, Citation2015).
Salt leaching
For the prevention of salt accumulation on the soil surface and in the root zone caused by evaporation and evapotranspiration, a significant amount of fresh water is required to overcome the rate of evaporation and evapotranspiration. A fraction of the applied water flow through the root zone and flush away the excess salts (Ebrahim Yahya et al., Citation2022). Therefore, the amount of water applied must be optimized to allow leaching without a water-table rise (Ebrahim Yahya et al., Citation2022). However, poor soil properties, such as low permeability, make this process ineffective (Xu et al., Citation2020). Without leaching, salts accumulate at the soil surface (Shaygan & Baumgartl, Citation2022) and can limit plant establishment.
There are several other techniques reported for managing and preventing salt accumulation that are not discussed in this article. These include subsurface drainage technologies (Okuda et al., Citation2020; Y. Yang et al., Citation2022), particularly in arid and semi-arid areas; salt-tolerant microbes (Egamberdieva et al., Citation2019; Kumawat et al., Citation2022; Otlewska et al., Citation2020); and land-shaping techniques (Bandyopadhyay et al., Citation2009).
Economic implications in reclamation of salt-affected soils
Estimation of the costs attributed to the reclamation of salt-affected soils is very heterogeneous due to different methodologies used in the assessment process, differences in soil types, the intensity of salinization, and the type of reclamation required (Munns & Gilliham, Citation2015). Yield loss is the most detrimental factor used to assess damage induced by salt-affected soils on a local scale (Thimmappa et al., Citation2016). The major economic cost of soil salinization is the reduced income to farmers caused by the reduced yield (Munns & Gilliham, Citation2015), which differs from one country to another and is largely influenced by the costs of inputs against the profit farmers can make in the seasons with average rainfall (Munns & Gilliham, Citation2015). At the farm level, net income decreases sharply with increasing salinity, which increases the cost of production (Thimmappa et al., Citation2016).
Consistent losses of crop yield caused by soil salinization as a result of climate change or the rising water table may alter the cropping system and make it impossible for crop production; ultimately, the land usage may revert to pasture production using salt-tolerant grasses or other species, including halophytes (Munns & Gilliham, Citation2015). Not only that, but farmers in salt-affected areas are also affected by food insecurity due to the devastating consequences of salt-induced costs in crop production (Haider & Hossain, Citation2013; P. Kumar & Sharma, Citation2020). According to M. Qadir et al. (Citation2014), the global estimate of salt-induced costs due to crop failure in irrigated areas could reach US $27.3 billion, with an estimated cost of US $441 per ha of land (M. Qadir et al., Citation2014), and economic loss could be as high as the estimated values if the salinity levels increase without proper management (M. Qadir et al., Citation2014).
In Tanzania, low yields have been reported in most rice-producing irrigation schemes (Kashenge-Killenga et al., Citation2013; Makoi & Ndakidemi, Citation2007), and some of the farms have even been abandoned (Meliyo et al., Citation2016). The estimated area loss by salt-affected soils in irrigation schemes ranges from 5 to 25% for more than 70% of the schemes surveyed (Kashenge-Killenga et al., Citation2016). Similarly, the study conducted by Omar et al. (Citation2022) based on farmers’ views indicated that about 25% of the land in the surveyed irrigation schemes was salt-affected. On the other hand, yield losses in salt-affected areas range from 5–100% depending on severity levels and irrigation water availability (Kashenge-Killenga et al., Citation2016). Unfortunately, the cost induced by salt-affected soils has not been reported for Tanzania as a case study because the current extent of salt-affected soils is not well established and no cost estimations have been done. Nevertheless, despite the negative impact of salt-affected soils on agricultural systems, it was established that if successfully managed, land with salt-affected soils becomes productive again and can hence be used for crop production (M. Qadir et al., Citation2014).
Based on a few reviewed case studies for Tanzania, salt-affected soils have been reported to exist in different agro-ecologies but are more alarming in rainfed lowlands and irrigated areas. Climate change is becoming a critical factor that has a significant impact on soil salinity (Corwin, Citation2021). Climate change is expected to increase the frequency of extreme weather events, with unusually low precipitation, higher temperatures, higher potential evapotranspiration, and more frequent droughts in parts of the world (Malhi et al., Citation2021).
Similarly, to other Sub-Saharan African countries, climate change and population growth rates are major factors influencing food insecurity in Tanzania (Bjornlund et al., 2020). The challenge of climate change is expected to worsen the situation of food insecurity (Ires, Citation2021; Randell et al., Citation2022). For instance, the central and some parts of the northern highlands of Tanzania experienced moderate to severe drought (Mdemu, Citation2021; Verhoeve et al., Citation2021), which caused serious threats to water availability, food security, and local livelihoods (Mdemu, Citation2021). There is a potential for climate change to exacerbate the hazards of soil salinity, especially in arid and semi-arid regions (Eswar et al., Citation2021).
Climate change could accelerate the hazards of soil salinity, especially in arid and semi-arid regions, and has the potential to spread the problem in the near future to currently unaffected areas (Eswar et al., Citation2021). Under this scenario, areas affected by salt contamination become worse, and it needs attention for the future of agriculture and its ability to satisfy the growing food demand for the fast-growing population (Mukhopadhyay et al., Citation2021). Therefore, it is important to address the challenges caused by salt-affected soils (i.e. high osmotic stress, nutritional disorders, toxicities, poor physical soil conditions, and reduced crop productivity) promptly. Minimizing the negative effects of climate change and reclamation of salt-affected soils are among the critical solutions to future food security (Corwin, Citation2021). Thus, research focusing on the extent, types, and management options of salt-affected soils is critically important. Thus, understanding the salt-affected soils aerial extent, types, and severity for a specific area is of critical importance for developing effective management options.
Conclusion and the way forward
Salt-affected soils are a challenge to agricultural land and have a substantial impact on the sustainability of food and nutritional security. In the face of its severe impact in some parts of the world, it calls for intervention to ensure food sustainability. Thus, to fulfill the demand for food security at regional and national levels, appropriate information is a key factor in lessening salt stress on agricultural land and improving crop sustainability. This review aimed to explore the currently available data on salt-affected soils in Tanzania, including the distribution extent, types, origin, and what have been the coping strategies for sustainable farming under both irrigated and rainfed agro-ecologies. Soil salinity affects plant physicochemical properties, including increasing osmotic pressure and oxidative stresses, which adversely affect crop growth, yield, and quality. There have been various mitigation techniques developed to manage salt-affected soils around the world, such as the use of organic and inorganic amendments, phytoremediation, salt-tolerant microbes, land-shaping techniques, and salt-tolerant crops. It is imperative to note, however, that the effectiveness of each technique varies based on the soil’s physicochemical properties, water availability, and the climatic conditions of a particular location. Additionally, the integration of leaching techniques and salt-tolerant varieties could be the most effective way to overcome salt stress challenges in Tanzania. In light of the conclusions above, it is recommended that comprehensive soil characterization studies be conducted to determine the extent (coverage) and the severity of the problem. In irrigated and lowland rainfed rice-producing areas, soil types should be classified and sources of salinization identified. Data from the above studies will be useful for guiding future research regarding the management options of salt-affected soils for the improvement of crop production. The information will also be used to predict the future impact of climate change on salt-affected soils as well as to develop affordable soil management options for reclaiming salt-affected soils.
Acknowledgements
The authors gratefully acknowledge the Climate Smart Africa Rice Project, Grant No.19-03-KU, for the financial Support. We are also very thankful to the unspecified reviewers and editors whose comments helped to improve the quality of this manuscript.
Data availability statement
No new data were created or analyzed in this review article. Therefore, data sharing does not apply to this article.
Disclosure statement
No potential conflict of interest was reported by the authors.
References
- Abate, S., Belayneh, M., & Ahmed, F. (2021). Reclamation and amelioration of saline-sodic soil using gypsum and halophytic grasses: Case of Golina-addisalem irrigation scheme, raya kobo valley, Ethiopia. Cogent Food and Agriculture, 7(1), 1–19. https://doi.org/10.1080/23311932.2020.1859847
- Abo Elyousr, K. A. M., Mousa, M. A. A., Ibrahim, O. H. M., Alshareef, N. O., & Eiss, M. A. (2022). Calcium-rich biochar stimulates salt resistance in pearl millet (Pennisetum glaucum L.) plants by improving soil quality and enhancing the antioxidant defense. Plants, 11(10), 1–11. https://doi.org/10.3390/plants11101301
- Acosta-Motos, J. R., Ortuño, M. F., Bernal-Vicente, A., Pedro, D., Sanchez-Blanco, M. J., & Hernandez, J. A. (2017). Plant responses to salt stress: Adaptive mechanisms. Agronomy, 7(1), 1–38. https://doi.org/10.3390/agronomy7010018
- Akhatar, M. S. (2019). Salt stress, microbes, and plant interactions: Causes and solution (Vol. 1). Springer. https://doi.org/10.1007/978-981-13-8801-9
- Alcívar, M., Zurita-Silva, A., Sandoval, M., Muñoz, C., & Schoebitz, M. (2018). Reclamation of saline-sodic soils with combined amendments: Impact on quinoa performance and biological soil quality. Sustainability, 10(9), 1–17. https://doi.org/10.3390/su10093083
- Ali, E. F., Al-Yasi, H. M., Kheir, A. M. S., & Eissa, M. A. (2021). Effect of biochar on CO2 sequestration and productivity of pearl millet plants grown in saline sodic soils. Journal of Soil Science and Plant Nutrition, 21(2), 897–907. https://doi.org/10.1007/s42729-021-00409-z
- Almutairi, A. A., Ahmad, M., Rafique, M. I., & Al-Wabel, M. I. (2023). Variations in composition and stability of biochars derived from different feedstock types at varying pyrolysis temperature. Journal of the Saudi Society of Agricultural Sciences, 22(1), 25–34. https://doi.org/10.1016/j.jssas.2022.05.005
- Amini, S., Ghadiri, H., Chen, C., & Marschner, P. (2016). Salt-affected soils, reclamation, carbon dynamics, and biochar: A review. Journal of Soils and Sediments, 16(3), 939–953. https://doi.org/10.1007/s11368-015-1293-1
- Anami, B. S., Malvade, N. N., & Palaiah, S. (2020). Classification of yield affecting biotic and abiotic paddy crop stresses using field images. Information Processing in Agriculture, 7(2), 272–285. https://doi.org/10.1016/j.inpa.2019.08.005
- Awan, S., Ippolito, J. A., Ullman, J. L., Ansari, K., Cui, L., & Siyal, A. A. (2021). Biochars reduce irrigation water sodium adsorption ratio. Biochar, 3(1), 77–87. https://doi.org/10.1007/s42773-020-00073-z
- Bandyopadhyay, B. K., Burman, D., Sarangi, S. K., MandaL, S., & BAL, A. R. (2009). Land shaping techniques to alleviate salinity and waterlogging problems of land shaping techniques to alleviate salinity and waterlogging problems of mono-cropped coastal land for multi-crop cultivation. Journal of the Indian Society of Coastal Agricultural Research, 27(1), 13–17. Retrieved from. https://krishi.icar.gov.in/jspui/bitstream/123456789/49751/1
- Bello, S. K., Alayafi, A. H., Al-Solaimani, S. G., & Abo Elyousr, K. A. M. (2021). Mitigating soil salinity stress with gypsum and bio-organic amendments: A review. Agronomy, 11(9), 1–18. https://doi.org/10.3390/agronomy11091735
- Bin Yousaf, M. T., Nawaz, M. F., Yasin, G., Cheng, H., Ahmed, I., Gul, S., Rizwan, M., Rehim, A., Xuebin, Q., & Ur Rahman, S. (2022). Determining the appropriate level of farmyard manure biochar application in saline soils for three selected farm tree species. PloS One, 17(4), 1–17. https://doi.org/10.1371/journal.pone.0265005
- Butcher, K., Wick, A. F., Desutter, T., Chatterjee, A., & Harmon, J. (2016). Soil salinity: A threat to global food security. Agronomy Journal, 108(6), 2189–2200. https://doi.org/10.2134/agronj2016.06.0368
- Chaganti, V., Crohn, D., & Šimůnek, J. (2015). Leaching and reclamation of a biochar and compost amended saline-sodic soil with moderate SAR reclaimed water. Agricultural Water Management, 158(1), 255–265. https://doi.org/10.1016/j.agwat.2015.05.016
- Chen, J., & Mueller, V. (2018). Coastal climate change, soil salinity and human migration in Bangladesh. Nature Climate Change, 8(11), 981–987. https://doi.org/10.1038/s41558-018-0313-8
- Chhabra, R. (2005). Classification of salt-affected soils. Arid Land Research and Management, 19(1), 61–79. https://doi.org/10.1080/15324980590887344
- Chiconato, D. A., Sousa, S., Maria, D., & Munns, R. (2019). Adaptation of sugarcane plants to saline soil. Environmental and Experimental Botany, 162(1), 201–211. https://doi.org/10.1016/j.envexpbot.2019.02.021
- Connor, J. D., Schwabe, K., King, D., & Knapp, K. (2012). Irrigated agriculture and climate change: The influence of water supply variability and salinity on adaptation. Ecological Economics, 77(1), 149–157. https://doi.org/10.1016/j.ecolecon.2012.02.021
- Corwin, D. L. (2021). Climate change impacts on soil salinity in agricultural areas. European Journal of Soil Science, 72(2), 842–862. https://doi.org/10.1111/ejss.13010
- Corwin, D. L., & Scudiero, E. (2019). Review of soil salinity assessment for agriculture across multiple scales using proximal and/or remote sensors. In Advances in Agronomy Vol. 158, 1st ed, Elsevier. https://doi.org/10.1016/bs.agron.2019.07.001
- Cuevas, J., Daliakopoulos, I. N., Del Moral, F., Hueso, J. J., & Tsanis, I. K. (2019). A review of soil-improving cropping systems for soil salinization. Agronomy, 9(6), 1–22. https://doi.org/10.3390/agronomy9060295
- Dehnavi, A. R., Zahedi, M., Ludwiczak, A., Perez, S. C., & Piernik, A. (2020). Effect of salinity on seed germination and seedling development of sorghum (Sorghum bicolor (L.) Moench) genotypes. Agronomy, 10(6), 1–15. https://doi.org/10.3390/agronomy10060859
- De Pauw, E. (1984). Soils, Physiography and Agro-Ecological Zones of Tanzania. Crop Monitoring and Early Warning Systems Project, FAO. Ministry of Agriculture, Dar es Salaam. GCPS/URT/047/NET.
- Diacono, M., & Montemurro, F. (2015). Effectiveness of organic wastes as fertilizers and amendments in salt-affected soils. Agriculture, 5(2), 221–230. https://doi.org/10.3390/agriculture5020221
- Ebrahim Yahya, K., Jia, Z., Luo, W., YuanChun, H., & Ame, M. A. (2022). Enhancing salt leaching efficiency of saline-sodic coastal soil by rice straw and gypsum amendments in Jiangsu coastal area. Ain Shams Engineering Journal, 13(5), 101721. https://doi.org/10.1016/j.asej.2022.101721
- Egal, F. (2020). Review of the state of food security and nutrition in the world, 2019. In World Nutrition (Vol. 10. p. 320). Food and Agriculture Organization of the United Nations. https://doi.org/10.26596/wn.201910395-97
- Egamberdieva, D., Wirth, S., Bellingrath-Kimura, S. D., Mishra, J., & Arora, N. K. (2019). Salt-tolerant plant growth promoting rhizobacteria for enhancing crop productivity of saline soils. Frontiers in Microbiology, 10, 1–18. https://doi.org/10.3389/fmicb.2019.02791
- El Hasini, S., Iben Halima, O., El Azzouzi, M., Douaik, A., Azim, K., & Zouahri, A. (2019). Organic and inorganic remediation of soils affected by salinity in the Sebkha of Sed El Mesjoune – Marrakech (Morocco). Soil and Tillage Research, 193, 153–160. https://doi.org/10.1016/j.still.2019.06.003
- Endo, A., & Kang, D. J. (2015). Salt removal from salt-damaged agricultural land using the scraping method combined with natural rainfall in the Tohoku district, Japan. Geoderma Regional, 4(November 2014), 66–72. https://doi.org/10.1016/j.geodrs.2014.11.001
- Eswar, D., Karuppusamy, R., & Chellamuthu, S. (2021). Drivers of soil salinity and their correlation with climate change. Current Opinion in Environmental Sustainability, 50, 310–318. https://doi.org/10.1016/j.cosust.2020.10.015
- FAO. 2000. Land resource potential and constraints at regional and country levels. World Soil Resources Reports, 90. Retrieved from ftp://ftp.fao.org/agl/agll/docs/wsr.pdf
- Farooq, M., Gogoi, N., Hussain, M., Barthakur, S., Paul, S., Bharadwaj, N., Migdadi, H. M., Alghamdi, S. S., & Siddique, K. H. M. (2017). Plant Physiology and Biochemistry Effects , tolerance mechanisms and management of salt stress in grain legumes. Plant Physiology Et Biochemistry, 118, 199–217. https://doi.org/10.1016/j.plaphy.2017.06.020
- Fidel, R. B., Laird, D. A., & Parkin, T. B. (2019). Effect of biochar on soil greenhouse gas emissions at the laboratory and field scales. Soil Systems, 3(1), 1–18. https://doi.org/10.3390/soilsystems3010008
- Foronda, D. A. (2022). Reclamation of a saline-sodic soil with organic amendments and leaching. Environmental Sciences Proceedings, 16(1), 1–4. https://doi.org/10.3390/environsciproc2022016056
- Freitas, A. M., Nair, V. D., & Harris, W. G. (2020). Biochar as Influenced by Feedstock Variability: Implications and opportunities for phosphorus management. Frontiers in Sustainable Food Systems, 4(September), 1–11. https://doi.org/10.3389/fsufs.2020.510982
- Gangwar, P., Singh, R., Trivedi, M., & Tiwari, R. K. (2019). Sodic soil: Management and reclamation strategies. Environmental Concerns and Sustainable Development: Volume 2: Biodiversity, Soil and Waste Management, 1(1), 175–190. https://doi.org/10.1007/978-981-13-6358-0_8
- Grant, M. J., & Booth, A. (2009). A typology of reviews: An analysis of 14 review types and associated methodologies. Health Information and Libraries Journal, 26(2), 91–108. https://doi.org/10.1111/j.1471-1842.2009.00848.x
- Gunarathne, V., Senadeera, A., Gunarathne, U., Biswas, J. K., Almaroai, Y. A., & Vithanage, M. (2020). Potential of biochar and organic amendments for reclamation of coastal acidic-salt affected soil. Biochar, 2(1), 107–120. https://doi.org/10.1007/s42773-020-00036-4
- Haider, M. Z., & Hossain, M. Z. (2013). Impact of salinity on livelihood strategies of farmers. Journal of Soil Science and Plant Nutrition, 13(2), 417–431. https://doi.org/10.4067/S0718-95162013005000033
- Hailu, B., Mehari, H., & Tamiru, H. (2020). Evaluation of sorghum for salt stress tolerance using different stages as screening tool in Raya Valley, Northern Ethiopia. Ethiopian Journal of Agricultural Sciences, 30(4), 265–276.
- Hassani, A., Azapagic, A., & Shokri, N. (2020). Predicting long-term dynamics of soil salinity and sodicity on a global scale. Proceedings of the National Academy of Sciences of the United States of America, 117(52), 33017–33027. https://doi.org/10.1073/PNAS.2013771117
- Huang, H., Reddy, N. G., Huang, X., Chen, P., Wang, P., Zhang, Y., & Garg, A.(2021). Effects of pyrolysis temperature, feedstock type and compaction on water retention of biochar amended soil. Scientific Reports, 11(1), 1–19. https://doi.org/10.1038/s41598-021-86701-5
- Huang, M., Zhang, Z., Zhu, C., Zhai, Y., & Lu, P. (2019). Effect of biochar on sweet corn and soil salinity under conjunctive irrigation with brackish water in coastal saline soil. Scientia horticulturae, 250(1), 405–413. https://doi.org/10.1016/j.scienta.2019.02.077
- Ippolito, J. A., Cui, L., Kammann, C., Wrage-Mönnig, N., Estavillo, J. M., Fuertes-Mendizabal, T., Cayuela, M. L., Sigua, G., Novak, J., Spokas, K., & Borchard, N. (2020). Feedstock choice, pyrolysis temperature and type influence biochar characteristics: A comprehensive meta-data analysis review. Biochar, 2(4), 421–438. https://doi.org/10.1007/s42773-020-00067-x
- Irakoze, W., Prodjinoto, H., Nijimbere, S., Bizimana, J. B., Bigirimana, J., Rufyikiri, G., & Lutts, S. (2021). NaCl- and Na 2 so 4 -induced salinity differentially affect clay soil chemical properties and yield components of two rice. Agronomy, 11(3), 1–15. https://doi.org/10.3390/agronomy11030571
- Ires, I. (2021). Intensive agriculture as climate change adaptation? economic and environmental tradeoffs in securing rural livelihoods in Tanzanian River Basins. Frontiers in Environmental Science, 9(November), 1–17. https://doi.org/10.3389/fenvs.2021.674363
- Ismail, A. M., & Horie, T. (2017). Genomics, physiology, and molecular breeding approaches for improving salt tolerance. Plant Biology, 68(1), 405–434. https://doi.org/10.1146/annurev-arplant-042916-040936
- Ivushkin, K., Bartholomeus, H., Bregt, A. K., Pulatov, A., Kempen, B., & de Sousa, L. (2019). Global mapping of soil salinity change. Remote Sensing of Environment, 231(1), 1–23. https://doi.org/10.1016/j.rse.2019.111260
- Jafarzadeh, H., Mahdianpari, M., Homayouni, S., Mohammadimanesh, F., & Dabboor, M. (2021). Oil spill detection from Synthetic Aperture Radar Earth observations: A meta-analysis and comprehensive review. GIScience & Remote Sensing, 58(7), 1022–1051. https://doi.org/10.1080/15481603.2021.1952542
- Kammann, C., Ippolito, J., Hagemann, N., Borchard, N., Cayuela, M. L., Estavillo, J. M., Fuertes-Mendizabal, T., Jeffery, S., Kern, J., Novak, J., Rasse, D., Saarnio, S., Schmidt, H. P., Spokas, K., & Wrage-Mönnig, N. (2017). Biochar as a tool to reduce the agricultural greenhouse-gas burden–knowns, unknowns and future research needs. Journal of Environmental Engineering and Landscape Management, 25(2), 114–139. https://doi.org/10.3846/16486897.2017.1319375
- Kamran, M., Parveen, A., Ahmar, S., Hussain, S., Chattha, M. S., Saleem, M. H., Adil, M., Heidari, P., & Chen, J. (2020). An Overview of Hazardous Impacts of Soil Salinity in Crops , Tolerance Mechanisms , and Amelioration through Selenium Supplementation. International Journal of Molecular Sciences, 21(1), 1–27. https://doi.org/10.3390/ijms21010148
- Kashenge-Killenga, S., Meliyo, J., Urassa, G., & Kongo, V. (2016). Extent of salt-affected soils and their effects in irrigated and lowland rain-fed rice growing areas of Southwestern Tanzania. In E. T. A. L. O. Lal, R. Kraybill, & D. HansenO. Singh, B. R. Mosogoya (Eds.), Climate Change and Multi-Dimensional Sustainability in Agriculture: Climate Change and Sustainability in Agriculture (pp. 97–126). Springer. https://doi.org/10.1007/978-3-319-41238-2_3
- Kashenge-Killenga, S., Tongoona, P., & Derera, J. (2013). Morphological and physiological responses of Tanzania rice genotypes under saline condition and evaluation of traits associated with stress tolerance. International Society for Development and Sustainability, 2(2), 1457–1475. Retrieved from. http://isdsnet.com/ijds-v2n2-76.pdf
- Kashenge-Killenga, S., Tongoona, P., Derera, J., & Kanyeka, Z. (2014). Farmers’ perception of salt affected soils and rice varieties preferences in the north-eastern Tanzania and their implications in breeding. International Journal of Development and Sustainability, 33(66), 2168–8662. Retrieved from. https://isdsnet.com/ijds-v3n6-2.pdf
- Kavitha, B., Reddy, P. V. L., Kim, B., Lee, S. S., Pandey, S. K., & Kim, K. H. (2018). Benefits and limitations of biochar amendment in agricultural soils: A review. Journal of Environmental Management, 227(June), 146–154. https://doi.org/10.1016/j.jenvman.2018.08.082
- Ketehouli, T., Carther, K. F. I., Noman, M., Wang, F. W., Li, X. W., & Li, H. Y. (2019). Adaptation of plants to salt stress: Characterization of Na+ and K+ transporters and role of Cbl gene family in regulating salt stress response. Agronomy, 9(11), 687. https://doi.org/10.3390/agronomy9110687
- Khatun, M., Shuvo, M. A. R., Salam, M. T. B., & Rahman, S. M. H. (2019). Effect of organic amendments on soil salinity and the growth of maize (Zea mays L.). Plant Science Today, 6(2), 106–111. https://doi.org/10.14719/pst.2019.6.2.491
- Kim, H. S., Kim, K. R., Lee, S. H., Kunhikrishnan, A., Kim, W. I., & Kim, K. H. (2018). Effect of gypsum on exchangeable sodium percentage and electrical conductivity in the Daeho reclaimed tidal land soil in Korea-a field scale study. Journal of Soils and Sediments, 18(2), 336–341. https://doi.org/10.1007/s11368-016-1446-x
- Kubaczyński, A., Walkiewicz, A., Pytlak, A., & Brzezińska, M. (2020). New biochars from raspberry and potato stems absorb more methane than wood offcuts and sunflower husk biochars. International Agrophysics, 34(3), 355–364. https://doi.org/10.31545/INTAGR/126762
- Kul, R., Arjumend, T., Ekinci, M., Yildirim, E., Turan, M., & Argin, S. (2021). Biochar as an organic soil conditioner for mitigating salinity stress in tomato. Soil Science and Plant Nutrition, 67(6), 693–706. https://doi.org/10.1080/00380768.2021.1998924
- Kumar, A., & Bandhu, A. (2005). Salt tolerance and salinity effects on plants: A review Cytosol and organelle space. Ecotoxicology and Environmental Safety, 60(3), 324–349. https://doi.org/10.1016/j.ecoenv.2004.06.010
- Kumar, A. N., Fatima, T., Mishra, J., Mishra, I., Verma, S., Verma, R., Verma, M., Bhattacharya, A., Verma, P., Mishra, P., & Bharti, C. (2020). Halo-tolerant plant growth promoting rhizobacteria for improving productivity and remediation of saline soils. Journal of Advanced Research, 26, 69–82. https://doi.org/10.1016/j.jare.2020.07.003
- Kumar, P., & Sharma, P. K. (2020). Soil Salinity and Food Security in India. Frontiers in Sustainable Food Systems, 4(1), 1–15. https://doi.org/10.3389/fsufs.2020.533781
- Kumawat, C., Kumar, A., Parshad, J., Sharma, S. S., Patra, A., Dogra, P., Yadav, G. K., Dadhich, S. K., Verma, R., & Kumawat, G. L. (2022). Microbial Diversity and Adaptation under Salt-Affected Soils : A Review. 9280(14), 1–24. https://doi.org/10.3390/su14159280
- Lastiri-Hernández, M. A., Alvarez-Bernal, D., Bermúdez-Torres, K., Cárdenas, G. C., & Ceja-Torres, L. F. (2019). Phytodesalination of a moderately saline soil combined with two inorganic amendments. Bragantia, 78(4), 579–586. https://doi.org/10.1590/1678-4499.20190031
- Leogrande, R., & Vitti, C. (2019). Use of organic amendments to reclaim saline and sodic soils: A review. Arid Land Research and Management, 33(1), 1–21. https://doi.org/10.1080/15324982.2018.1498038
- Liang, W., Ma, X., Wan, P., & Liu, L. (2017). Plant salt-tolerance mechanism: A review. Biochemical and Biophysical Research Communications, 1(1), 1–6. https://doi.org/10.1016/j.bbrc.2017.11.043
- Liu, Z., Jiao, X., Lu, S., Zhu, C., Zhai, Y., Guo, W., & Farooq, S. (2019). Effects of winter irrigation on soil salinity and jujube growth in arid regions. PloS One, 14(6), 1–11. https://doi.org/10.1371/journal.pone.0218622
- Maas, E. V. (1993). Plant growth response to salt stress. https://doi.org/10.1007/978-94-011-1858-3_31
- Mageshen, V., Vidyapeetham, A. V., R, M. K., & Gajendiren, M. (2022, March). Latest trends in soil science (Volume - 3). Latest Trends in Soil Science, 3. https://doi.org/10.22271/int.book.107
- Makoi, J. H. J. R., & Ndakidemi, P. A. (2007). Reclamation of sodic soils in northern Tanzania, using locally available organic and inorganic resources. African Journal of Biotechnology, 6(16), 1926–1931. https://doi.org/10.5897/ajb2007.000-2292
- Malhi, G. S., Kaur, M., & Kaushik, P. (2021). Impact of climate change on agriculture and its mitigation strategies: A review. Sustainability (Switzerland), 13(3), 1–21. https://doi.org/10.3390/su13031318
- Mamo, L., Bekele, T., Nekir, B., & Worku, A. (2021). Screening of Different Sesame (Sesamum Indicum L .) Accessions for Salt Tolerance at Different Growth Stages. International Journal of Novel Research in Life Sciences, 8(1), 21–27.
- Manuel, R., Machado, A., & Serralheiro, R. P. (2017). Soil salinity: Effect on vegetable crop growth. management practices to prevent and mitigate soil salinization. Horticulturae, 3(2), 1–13. https://doi.org/10.3390/horticulturae3020030
- Mdemu, M. V. (2021). Community’s vulnerability to drought-driven water scarcity and food insecurity in central and northern semi-arid areas of Tanzania. Frontiers in Climate, 3(October), 1–14. https://doi.org/10.3389/fclim.2021.737655
- Mehmood, S., Ahmed, W., Ikram, M., Imtiaz, M., Mahmood, S., Tu, S., & Chen, D. (2020). Chitosan modified biochar increases soybean (Glycine max l.) resistance to salt-stress by augmenting root morphology, antioxidant defense mechanisms and the expression of stress-responsive genes. Plants, 9(9), 1–25. https://doi.org/10.3390/plants9091173
- Meliyo, J. L., Kashenge-Killenga, S., Victor, K. M., Mfupe, B., Hiza, S., Kihupi, A. L., Boman, B. J., & Dick, W. (2016). Evaluation of Salt Affected Soils for Rice (Oryza Sativa) Production in Ndungu Irrigation Scheme Same District, Tanzania. Sustainable Agriculture Research, 6(1), 24–38. https://doi.org/10.5539/sar.v6n1p24
- Mengist, W., Soromessa, T., & Legese, G. (2020). Method for conducting systematic literature review and meta-analysis for environmental science research. MethodsX, 7, 100777. https://doi.org/10.1016/j.mex.2019.100777
- Mnkeni, P. (1996). Salt affected soils: Their origin, identification, reclamation and Management. Inter Press Ltd, 1, 1–250.
- Mohanavelu, A., Naganna, S. R., & Al-Ansari, N. (2021). Irrigation Induced salinity and sodicity hazards on soil and groundwater: An overview of its causes, impacts and mitigation strategies. Agriculture, 11(10), 1–17. https://doi.org/10.3390/agriculture11100983
- Mukhopadhyay, R., Sarkar, B., Jat, H. S., Sharma, P. C., & Bolan, N. S. (2021). Soil salinity under climate change: Challenges for sustainable agriculture and food security. Journal of Environmental Management, 280(1), 14. https://doi.org/10.1016/j.jenvman.2020.111736
- Munns, R., & Gilliham, M. (2015). Salinity tolerance of crops - what is the cost? Tansley insight Salinity tolerance of crops – what is the cost? The New Phytologist, 208(1), 668–673. Retrieved from, https://nph.onlinelibrary.wiley.com/doi/10.1111/nph.13519
- Munns, R., Passioura, J. B., Byrt, C. S., & Colmer, T. D. (2020). Tansley insight Osmotic adjustment and energy limitations to plant growth in saline soil. New Phytologist, 1091–1096. https://doi.org/10.1111/nph.15862
- Nekir, B., Mamo, L., & Worku, A. (2019). Evaluation of wheat varieties/lines for salt tolerance at different growth stages. Greener Journal of Soil Science and Plant Nutrition, 6(1), 1–7. https://doi.org/10.15580/GJSSPN.2019.1.060619105
- Nouri, H., Chavoshi Borujeni, S., Nirola, R., Hassanli, A., Beecham, S., Alaghmand, S., Saint, C., & Mulcahy, D. (2017). Application of green remediation on soil salinity treatment: A review on halophytoremediation. Process Safety and Environmental Protection, 107, 94–107. https://doi.org/10.1016/j.psep.2017.01.021
- Okuda, Y., Onishi, J., Shirokova, Y. I., Kitagawa, I., Kitamura, Y., & Fujimaki, H. (2020). Water and salt balance in agricultural lands under leaching with shallow subsurface drainage used in combination with cut-drains. Water (Switzerland), 12(11), 1–16. https://doi.org/10.3390/w12113207
- Omar, M. M., Shitindi, M. J., Massawe, B. H. J., Fue, K. G., Pedersen, O., & Meliyo, J. L. (2022). Exploring farmers’ perception, knowledge, and management techniques of salt-affected soils to enhance rice production on small land holdings in Tanzania. Cogent Food and Agriculture, 8(1). https://doi.org/10.1080/23311932.2022.2140470
- Omuto, C., Vargas, R., El Mobarak, A., Mohamed, N., Viatkin, K., & Yigini, Y. (2020). Mapping of salt-affected soils. Technical Manual, FAO. Rome., 151pp. https://doi.org/10.4060/ca9215en
- Osman, K. T. (2018). Management of soil problems. In Management of Soil Problems (p. 474). Springer. https://doi.org/10.1007/978-3-319-75527-4
- Otlewska, A., Migliore, M., Dybka-Stępień, K., Manfredini, A., Struszczyk-Świta, K., Napoli, R., Białkowska, A., Canfora, L., & Pinzari, F. (2020). When Salt Meddles Between Plant, Soil, and Microorganisms. Frontiers in plant science, 11(1). https://doi.org/10.3389/fpls.2020.553087
- Page, M. J., McKenzie, J. E., Bossuyt, P. M., Boutron, I., Hoffmann, T. C., Mulrow, C. D., Shamseer, L., Tetzlaff, J. M., Akl, E. A., Brennan, S. E., Chou, R., Glanville, J., Grimshaw, J. M., Hróbjartsson, A., Lalu, M. M., Li, T., Loder, E. W., Mayo-Wilson, E., McDonald, S., & Moher, D. (2021). The PRISMA 2020 statement: An updated guideline for reporting systematic reviews. The BMJ, 372. https://doi.org/10.1136/bmj.n71
- Phogat, V., Pitt, T., Cox, J. W., Š, J., & Skewes, M. A. (2018). Soil water and salinity dynamics under sprinkler irrigated almond exposed to a varied salinity stress at di ff erent growth stages. Agricultural Water Management, 201(1), 70–82. https://doi.org/10.1016/j.agwat.2018.01.018
- Phuong, N. T. K., Khoi, C. M., Ritz, K., Linh, T. B., Minh, D. D., Duc, T. A., Sinh, N. V., Linh, T. T., & Toyota, K. (2020). Influence of rice husk biochar and compost amendments on salt contents and hydraulic properties of soil and rice yield in salt-affected fields. Agronomy, 10(8), 1–23. https://doi.org/10.3390/agronomy10081101
- Prasad, K., Devkota, M., Rezaei, M., & Oosterbaan, R. (2022). Managing salinity for sustainable agricultural production in salt-affected soils of irrigated drylands. Agricultural Systems, 198(1), 1–16. https://doi.org/10.1016/j.agsy.2022.103390
- Prasertsuk, S., & Wijitkosum, S. (2021). Innovative use of rice husk biochar for rice cultivation in salt-affected soils with alternated wetting and drying irrigation. Engineering Journal, 25(9), 1–14. https://doi.org/10.4186/ej.2021.25.9.19
- Qadir, G., Ahmad, K., Qureshi, M., Saqib, A., Zaka, M., Sarfraz, M., Warraich, I., & Ullah, S. (2017). Integrated use of inorganic and organic amendments for reclamation of salt affected soil. International Journal of Biosciences (IJB), 11(2), 1–10. https://doi.org/10.12692/ijb/11.2.1-10
- Qadir, M., Quillérou, E., Nangia, V., Murtaza, G., Singh, M., Thomas, R. J., Drechsel, P., Noble, A. D., Qadir, M., Quillérou, E., Nangia, V., Murtaza, G., & Singh, M. (2014). Economics of salt-induced land degradation and restoration. HAL Open Science, 1(1), 1–27.
- Rahneshan, Z., Nasibi, F., & Moghadam, A. A. (2018). Effects of salinity stress on some growth, physiological, biochemical parameters and nutrients in two pistachio (Pistacia vera L .) rootstocks. Journal of Plant Interactions, 13(1), 73–82. https://doi.org/10.1080/17429145.2018.1424355
- Randell, H., Gray, C., & Shayo, E. H. (2022). Climatic conditions and household food security: Evidence from Tanzania. Food Policy, 112(November 2021), 102362. https://doi.org/10.1016/j.foodpol.2022.102362
- Richards, L. A., & USDA. (1954). Washington. Soil Science Society of America JournalSoil Science Society of America Journal, 18(3), 348. https://doi.org/10.2136/sssaj1954.03615995001800030032x
- Saifullah Dahlawi, S., Naeem, A., Rengel, Z., Naidu, R., & Naidu, R. (2017). Biochar application for the remediation of salt-affected soils: Challenges and opportunities. The Science of the Total Environment, 625(1), 320–335. https://doi.org/10.1016/j.scitotenv.2017.12.257
- Sarwar, G., Ibrahim, M., Tahir, M. A., Iftikhar, Y., Haider, M. S., Noor-Us-Sabah, N. U. S., Han, K. H., Ha, S. K., & Zhang, Y. S. (2011). Effect of Compost and Gypsum Application on the Chemical Properties and Fertility Status of Saline-Sodic Soil. Korean Journal of Soil Science and Fertilizer, 44(3), 510–516. https://doi.org/10.7745/kjssf.2011.44.3.510
- Shahid, S. A., Zaman, M., & Heng, L. (2018). Introduction to Soil Salinity, Sodicity and Diagnostics Techniques. Guideline for Salinity Assessment, Mitigation and Adaptation Using Nuclear and Related Techniques. https://doi.org/10.1007/978-3-319-96190-3_1
- Sharma, S., Kulkarni, J., & Jha, B. (2016). Halotolerant Rhizobacteria promote growth and enhance salinity tolerance in peanut isolation of bacterial isolates. Frontiers in Microbiology, 7(1), 1–11. https://doi.org/10.3389/fmicb.2016.01600
- Shaygan, M., & Baumgartl, T. Reclamation of Salt-Affected Land: A Review. (2022). Soil Systems, 6(3), 61. 6(3. https://doi.org/10.3390/soilsystems6030061
- Sheoran, P., Kumar, A., Singh, A., Parjapat, K., Sharma, R., & Yadav, R. K. (2021). Pressmud alleviates soil sodicity stress in a rice – wheat rotation: Effects on soil properties, physiological adaptation and yield-related traits. Land Degrad and Development, 1(1), 1–14. https://doi.org/10.1002/ldr.3953
- Shokat, S., & Großkinsky, D. K. (2019). Tackling Salinity in Sustainable Agriculture — what developing countries may learn from approaches of the developed world the saline global area under salinity (Million Hectares). Sustainability, 11(1), 19. https://doi.org/10.3390/su11174558
- Shouse, P. J., Goldberg, S., Skaggs, T. H., Soppe, R. W. O., & Ayars, J. E. (2010). Changes in spatial and temporal variability of SAR affected by shallow groundwater management of an irrigated field, California. Agricultural Water Management, 97(5), 673–680. https://doi.org/10.1016/j.agwat.2009.12.008
- Shrivastava, P., & Kumar, R. (2015). Soil salinity: A serious environmental issue and plant growth promoting bacteria as one of the tools for its alleviation. Saudi Journal of Biological Sciences, 22(2), 123–131. https://doi.org/10.1016/j.sjbs.2014.12.001
- Singh, A. (2021). Soil salinity: A global threat to sustainable development. Soil Use and Management, 1(1), 1–28. https://doi.org/10.1111/sum.12772
- Singh, U. B., Malviya, D., Singh, S., Singh, P., Ghatak, A., Imran, M., Rai, J. P., Singh, R. K., Manna, M. C., Sharma, A. K., & Saxena, A. K. (2021). Salt‐tolerant compatible microbial inoculants modulate physio‐biochemical responses enhance plant growth, zn biofortification and yield of wheat grown in saline‐sodic soil. International Journal of Environmental Research and Public Health, 18(18), 1–53. https://doi.org/10.3390/ijerph18189936
- Singh, Y. P., Mishra, V. K., Bharadwaj, A. K., Arora, S., Singh, A. K., Singh, S., Singh, U. S., & Ismail, A. M. (2019). Synergy of reduced gypsum and pressmud – a cost effective approach for sustainable reclamation of degraded sodic lands. Indian Journal of Agricultural Sciences, 89(6), 1027–1032.
- Singh, M., Singh, S., Parkash, V., Ritchie, G., Wallace, R. W., & Deb, S. K. (2022). Biochar implications under limited irrigation for sweet corn production in a semi-arid environment. Frontiers in Plant Science, 13(April), 1–13. https://doi.org/10.3389/fpls.2022.853746
- Snyder, H. (2019). Literature review as a research methodology: An overview and guidelines. Journal of Business Research, 104(March), 333–339. https://doi.org/10.1016/j.jbusres.2019.07.039
- Stavi, I., Shem-Tov, R., Ragolsky, G., & Lekach, J. (2017). Ancient to recent-past runoff harvesting agriculture in recharge playas of the hyper-arid Southern Israel. Water, 9(12), 1–18. https://doi.org/10.3390/w9120991
- Stavi, I., Thevs, N., & Priori, S. (2021). Soil salinity and sodicity in drylands: a review of causes, effects, monitoring, and restoration measures. Frontiers in Environmental Science, 9(1), 1–16. https://doi.org/10.3389/fenvs.2021.712831
- Sun, Y., Chen, X., Yang, J., Luo, Y., Yao, R., Wang, X., Xie, W., & Zhang, X. (2022). Biochar Effects Coastal Saline Soil and Improves Crop Yields in a Maize-Barley Rotation System in the Tidal Flat Reclamation Zone, China. Water (Switzerland), 14(20). https://doi.org/10.3390/w14203204
- Sunita, K., Mishra, I., Mishra, J., Prakash, J., & Arora, N. K. (2020). Secondary metabolites from halotolerant plant growth promoting rhizobacteria for ameliorating salinity stress in plants. Frontiers in Microbiology, 11(1), 1–12. https://doi.org/10.3389/fmicb.2020.567768
- Sun, H., Lu, H., Chu, L., Shao, H., & Shi, W. (2017). Biochar applied with appropriate rates can reduce N leaching, keep N retention and not increase NH3 volatilization in a coastal saline soil. The Science of the Total Environment, 575(1), 820–825. https://doi.org/10.1016/j.scitotenv.2016.09.137
- Suvi, W. T., Shimelis, H., & Laing, M. (2020). Farmers’ perceptions, production constraints and variety preferences of rice in Tanzania. Journal of Crop Improvement, 00(1), 1–18. https://doi.org/10.1080/15427528.2020.1795771
- Thimmappa, K., Sharma, D., Dagar, J., & Raju, R. (2016). Reclamation of Salt-Affected Soils: Socioeconomic Impact Assessment. In J. C. Dagar, D. K. Sharma, P. C. Sharma, & A. K. Singh (Eds.), Innovative Saline Agriculture (pp. 489–506). https://doi.org/10.1007/978-81-322-2770-0
- Tully, K., Sullivan, C., Weil, R., & Sanchez, P. (2015). The state of soil degradation in sub-Saharan Africa: Baselines, trajectories, and solutions. Sustainability, 7(6), 6523–6552. https://doi.org/10.3390/su7066523
- Verhoeve, S. L., Keijzer, T., Kaitila, R., Wickama, J., & Sterk, G. (2021). Vegetation resilience under increasing drought conditions in northern Tanzania. Remote Sensing, 13(22), 1–20. https://doi.org/10.3390/rs13224592
- Wallender, W. W., & Kenneth, T. K. (Eds). (2012). Agricultural Salinity Assessment and Management. American Society of Civil Engineers, pp. 905. Retrieved from https://ascelibrary.org/doi/book/10.1061/9780784411698
- Wang, X. W., Cai, H., Liu, Y. L., Li, C. L., Wan, Y. S., Song, F. P., & Chen, W. F. (2019). Addition of organic fertilizer affects soil nitrogen availability in a salinized fluvo-aquic soil. Environmental Pollutants and Bioavailability, 31(1), 331–338. https://doi.org/10.1080/26395940.2019.1700827
- Wang, W., Vinocur, B., & Altman, A. (2003). Plant responses to drought, salinity and extreme temperatures: Towards genetic engineering for stress tolerance. Planta, 218(1), 1–14. https://doi.org/10.1007/s00425-003-1105-5
- Wen, W., Timmermans, J., Chen, Q., & van Bodegom, P. M. (2021). A review of remote sensing challenges for food security with respect to salinity and drought threats. Remote Sensing, 13(1), 1–14. https://doi.org/10.3390/rs13010006
- Wicke, B., Smeets, E., Dornburg, V., Vashev, B., Gaiser, T., Turkenburg, W., & Faaij, A. (2011). The global technical and economic potential of bioenergy from salt-affected soils. Energy and Environmental Science, 4(8), 2669–2681. https://doi.org/10.1039/c1ee01029h
- Xu, P., Zhang, Q., Qian, H., & Qu, W. Effect of sodium chloride concentration on saturated permeability of remolded loess. (2020). Minerals, 10(2), 199. 10(2. https://doi.org/10.3390/min10020199
- Yang, A., Akhtar, S. S., Li, L., Fu, Q., Li, Q., Naeem, M. A., He, X., Zhang, Z., & Jacobsen, S. E. (2020). Biochar mitigates combined effects of drought and salinity stress in Quinoa. Agronomy, 10(6). https://doi.org/10.3390/agronomy10060912
- Yang, Y., Li, D., Huang, W., Zhou, X., Li, Z., Dong, X., & Wang, X. (2022). Effects of subsurface drainage on soil salinity and groundwater table in drip irrigated cotton fields in Oasis Regions of Tarim Basin. Agriculture, 12(2167), 1–14. https://doi.org/10.3390/agriculture12122167
- Zhang, Q., & Dai, W. (2019). Plant response to salinity stress. In Stress Physiology of Woody Plants, <. I. I. A. I. <. Dai. Ed., 1st Edition. pp. 155–173. CRC PressTylor & Fransis. Retrieved fromhttps://www.routledge.com/Stress-Physiology-of-Woody-Plants/Dai/p/book/9781032092775
- Zhao, C., Zhang, H., Song, C., Zhu, J. K., & Shabala, S. (2020). Mechanisms of plant responses and adaptation to soil salinity. The Innovation, 1(1), 1–20. https://doi.org/10.1016/j.xinn.2020.100017
- Zörb, C., Geilfus, C. M., Dietz, K. J., & Weber, A. (2019). Salinity and crop yield. Plant Biology, 21(1), 31–38. https://doi.org/10.1111/plb.12884