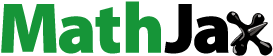
ABSTRACT
This study investigates the financial feasibility of using harvest residue for bioelectricity and its financial impact on conventional plantation forestry in South Africa. The biomass gasification system used is a 5-megawatt (MW) plant by GreenForze. Data were collected on the investment cost, operational and maintenance costs, timber harvest and transport costs, wages and salaries, and revenues from electricity sales through interviews with forestry stakeholders and a literature search. The cost details used in the investment and financial risk analysis of the conventional plantation forestry operations were from Forestry Economics Services (FES). An excel spreadsheet model was developed and used for the financial feasibility analysis using net present value (NPV), and internal rate of return (IRR) as indicators. A Monte Carlo sensitivity analysis was integrated into the model to analyze the investment risk. Results revealed that harvest residue gasification for electricity is financially feasible with an NPV of 7,049,336.64 South African Rand/Zuid-Afrikaanse Rand (ZAR) and an IRR of 15.8%. The integration of bioelectricity production with conventional plantation forestry operations improved the IRR from 15.10% to 18.31%, while at the same time improving the NPV from ZAR 10,334.86 to ZAR 15,378.46. Sensitivity analysis forecasts low-cost risks for investors. The gasification plant’s NPV was found to be highly sensitive to the investment cost, and transport cost of harvest residues. The study deduces that integrating conventional plantation forestry with timber harvest residue gasification for bioelectricity is a feasible pathway to sustainable electricity production in the South African context.
1. Introduction
The evolving policy discourse on energy security and climate change is leading to a rethink of forest management strategies (Jin & Sutherland, Citation2018; I (Citation2013); Jean-François et al., Citation2011; Miner & Lucier, Citation2004). This is because of the growing policy attention to forest bioenergy (A term used to describe renewable energy generated from the combustion of woody biomass or fuels derived from woody biomass: Cheng-Lin et al., Citation2017; J. S. Cardoso et al., Citation2020; Jean-François et al., Citation2011) as an environmentally friendly and cost-effective climate change mitigation strategy (Camia et al., Citation2021; Lyons-White et al., Citation2018; Weiss et al., Citation2020), capable of spurring sustainable development (Wu et al., Citation2002; Yagi & Nakata, Citation2011). Hence the need for appropriate modalities for adapting forest management regimes to facilitate the integration of conventional forestry operations with forest-based climate change mitigation strategies (Kizha & Han-Sup, Citation2015; Premer et al., Citation2019). Among the forest bioenergy strategies being promoted, forest residue gasification for electricity production has gained policy traction in many developing countries because of the abundance of forest biomass, and their affordability, given that they are a by-products of conventional forestry functioning (Colantoni et al., Citation2021; Daioglou et al., Citation2016; Jin & Sutherland, Citation2018; Porcu et al., Citation2019; Ratnasingam et al., Citation2013). Additionally, unlike the sporadic nature of wind and solar, forest residue gasification for electricity can be uninterrupted, and modified to meet switch in energy demand (Baker et al., Citation2018). Forest industries are well positioned to play a prominent role in facilitating the adoption and upscale of forest bioenergy strategies in response to energy security and climate change challenges (Cambero et al., Citation2015; Lyons-White et al., Citation2018). However, the economic costs of forest residue utilization for bioelectricity production and its potential financial impact on conventional forestry operations are poorly represented in forest bioenergy studies (Beke et al., Citation1996; Burgess, Citation1993; Daioglou et al., Citation2016). Exploring these opportunities, particularly in developing country contexts can be buttressed by investigations that assess the financial feasibility of joint production for timber and bioelectricity (Jean-François et al., Citation2011; Ratnasingam et al., Citation2013).
Policy efforts to promote forest bioenergy share of domestic and industrial energy consumption in Africa have continued to gain momentum (Boerner et al., Citation2016; International Renewable Energy Agency: IRENA, Citation2017; Yagi & Nakata, Citation2011), with majority of the policies targeting multiple objectives such as net zero emissions, energy security, employment creation, and reduction of air pollution (Clarke et al., Citation1997; Pokharel et al., Citation2017; Situmorang et al., Citation2020). Bioenergy in South Africa currently accounts for 8.7% of energy sources used in the industrial sector and 80% of domestic energy consumption in rural communities (Bali, Citation2018). In South Africa, several current planning policies are anticipated to expand renewable energy production over the next 10 years period (Bali, Citation2018; International Institute for Environment and Development IIED, Citation2013; International Renewable Energy Agency: IRENA, Citation2017). Forest bioenergy is anticipated to play a significant part in the renewable energy scenario of South Africa (Bali, Citation2018; International Institute for Environment and Development IIED, Citation2013). In this regard, the need to utilize forest residues for bioelectricity production continues to be a suggested policy instrument for a more environmentally benign economic development (Beke et al., Citation1996). The utilization of timber harvest residue for bioelectricity production can create employment in rural communities, enhance the competitiveness of forest product industries, and activate vibrant forest enterprise development in rural areas (Huang et al., Citation2020; Yagi & Nakata, Citation2011). However, the investment feasibility and risk sensitivity of such investment in South Africa are relatively unknown (Bali, Citation2018; International Institute for Environment and Development IIED, Citation2013; International Renewable Energy Agency: IRENA, Citation2017).
The South African forests (Natural forests, woodlands, and tree plantations) occupy about 46 million ha, providing appropriate feedstock for woody biomass based gasification plant (Ofoegbu & Ifejika-Speranza, Citation2021). South Africa’s forest industries generate an estimate of 3376 kilotonnes of forest residue per year (kt/y) that can be used as feedstocks for bioelectricity production (International Renewable Energy Agency: IRENA, Citation2017; Lundqvist, Citation2020). However, investment in woody biomass based gasification plant is propelled primarily by financial performance. Nevertheless, the financial performance of woody biomass based gasification technology remains relatively unknown in the South African context (International Institute for Environment and Development IIED, Citation2013; Ofoegbu, Citation2010; Penniall & Williamson, Citation2009; Tripathi et al., Citation1997). There are growing concerns about the financial feasibility of using timber harvest residue for electricity production (Premer et al., Citation2019). As a feedstock source, timber harvest residues are by-products whose utilization feasibility are based on reduced production costs (J. Cardoso et al., Citation2019; Penniall & Williamson, Citation2009). Therefore, incorporating the cost of timber harvest residue recovery for bioenergy into the operational cost of traditional tree plantation operations has been mooted as a means to facilitate the financial feasibility of producing electricity from timber harvest residues (Puttock, Citation1995; Saunders et al., Citation2012). Doing this will require internalizing production costs in conventional tree plantation operations (Cambero et al., Citation2015; Mayaki, Citation2008; Savvides, Citation1994). Hence, to develop new forest management practices and regimes that can support South Africa’s climate change mitigation and adaptation aspiration (Miner & Lucier, Citation2004; Ratnasingam et al., Citation2013), a systematic analysis of the financial feasibility of plantation forestry functioning and its sensitivity to the integration of forest bioenergy strategies is required (Weiss et al., Citation2020).
Despite the growing policy directives and mandates to produce electricity from woody biomass, the uncertainty around the financial feasibility and risks to investors continue to impede the transition to this renewable energy pathway, particularly in developing countries where the demand are the highest (J. S. Cardoso et al., Citation2020; Kizha & Han-Sup, Citation2015; Leung et al., Citation2004). This is because investments in forest bioenergy projects are exposed to high levels of financial risks (Baker et al., Citation2018; Colantoni et al., Citation2021; Scudder et al., Citation2019). The high capital costs, operation costs, and maintenance costs of harvest residue-based gasification plant and their associated risks can keep the potential investor from investing in a forest-based bioelectricity project. Therefore, a comprehensive assessment of the financial risks associated with forest bioenergy investment is required to support bioenergy investments (Pereira et al., Citation2014). This study deals with this issue by using a Monte Carlo Simulation (MCS) approach to risk analysis to analyze the financial risks of investing in a harvest residue-based gasification plant. This study primarily aims to determine if bioelectricity production from harvest residues could be incorporated into conventional tree plantation operations in South Africa. The study investigated the following three research questions:
Is it financially feasible to utilize timber harvest residue for electricity production?
Is it financially feasible to integrate conventional forestry operations with harvest residue recovery and gasification for electricity production?
How sensitive are the investment feasibility indicators (IRR and NPV) to the cost components of the forest residue gasification?
It is important to point out that Eucalyptus plantations and harvest residue utilization for bioelectricity could have a negative consequences on the environment (Call et al., Citation2017; Malkamäki et al., Citation2018). Soil quality, water table and rural livelihood could be negatively impacted. In South Africa, all these factors are already taking into consideration before permits are issued for tree plantation establishment. Nearly all plantation in the study sites are Forest Stewardship Council (FSC) certified reienforcing their adherence to socio-environmental sustainability standards. Therefore, harvest residue utilization for electricity production as investigated in this study is recommended for certified plantations and plantation managed according to South Africa’s Department of Agriculture, Fisheries and Forestry regulations.
2. Materials and Methods
2.1 Data collection
The biomass gasification system used is a 5 mega-watt (MW) plant by GreenForze that is designed to use chips from timber harvest residue as feedstock for electricity production (Figure ). The workforce needed to operate the gasification plant is presumed to be comprised of 6 full-time employees. The technical features of the modelled 5 MW plant are presented in Table .
Figure 1. Principle scheme of harvest residue gasification plant for bioelectricity (Source: Abdul et al., Citation2010)

Table 1. Features of the modelled 5 MW gasification plant
To carry out the financial feasibility and risk analysis of the designed 5 MW gasification plant, data were collected on the investment cost, operational and maintenance costs, timber harvest and transport costs, wages and salaries, and revenues from electricity sales through interviews with forestry stakeholders and literature search. The cost details used in the investment and financial risk analysis of the conventional plantation forestry operations were from Forestry Economics Services (FES). FES is an independent South African organization that annually publishes business reports and benchmark costs of South Africa tree plantations (Forest Economics Services FES, Citation2008). Previous study (Ofoegbu, Citation2010) has identified transporting of residue from landing and chipping at energy plant as the cost-effective way of transporting residue in South Africa. This is the strategy applied in this study. The cost details are presented in Table below. The modeled conventional plantation forestry is a Eucalyptus plantation managed on an 8 years rotation basis for pulp and paper production.
ZAR is the symbol for South African currency known as the South African Rand
Table 2. Cost details for analysis of conventional forestry plantation operations
2.2 Developing the financial model
Discounted cash flow (DCF) was used to analyze and compare the costs and income of bioelectricity production from harvest residue, the cost and income from conventional plantation forestry, and the cost and income from the integrated scenario of timber and bioelectricity production. The study used DCF to estimate the Net Present Value (NPV), and Internal Rate of Return (IRR) of cash flows generated by the three scenarios of harvest residue gasification for bioelectricity, conventional plantation forestry, and integrated timber and bio-electricity production. The DCF model was constructed in Microsoft excel spreadsheet. The discount rate selected for the DCF model was 5.62%, which is the 10-year average deposit interest rate in South Africa issued by the Reserve Bank of South Africa (Trading economics, Citation2021). The discounted cash flow analysis was developed by dividing the annual cash flow in each year by one plus the discount rate elevated to the related year (J. Cardoso et al., Citation2019). Each of the two indicators NPV and IRR used to assess the financial analysis was carried out as follows:
The net present value (NPV) method estimates the present value of the future costs and revenues of a project minus its initial investment. The NPV of each of the three scenarios of conventional plantation forestry, woody biomass gasification for electricity, and integrated conventional plantation forestry and woody biomass gasification was calculated using equation 1 below:
Where: n = year, R = revenues, C = costs, i = annual discount rate, N = Project duration
A zero NPV means the project is not expected to result in any significant gain or loss. A positive NPV means the project will be profitable, and a negative NPV means the project is expected to result in a net loss (J. S. Cardoso et al., Citation2020; Klemperer, Citation2003).
The internal rate of return (IRR) represents the interest rate at which the cost of the investment leads to the profits from the investment (J. S. Cardoso et al., Citation2020; Klemperer, Citation2003). A IRR higher than the discount rate, means the project is feasible. The IRR was calculated using equation 2 below:
Where: n = year, R = revenues, C = costs, i = annual discount rate, N = Project duration
Sensitivity
Given the uncertainty in the future renewable energy policy of South Africa, a sensitivity analysis is performed to explore the financial risks connected with investment in timber harvest residue gasification for bioelectricity production in South Africa. The study used ‘@RISK’ software for the Monte Carlo risk analysis. The software is a Microsoft Excel Add-in manufactured by the Palisade Corporation (Savvides, Citation1994; Scudder et al., Citation2019). The risk analysis simulation followed the approach used by J. S. Cardoso et al. (Citation2020). This was done by assigning normal distribution to all cash flow variables determined to have values with inherent uncertainty. The DCF model was designed to randomly select a value within the assigned distribution range of each uncertain variable (from the costs and revenue variables) for each iteration of the simulation. The number of iterations for the Monte Carlo risk simulation was set at 100,000 (J. S. Cardoso et al., Citation2020; Savvides, Citation1994; Scudder et al., Citation2019).
3. Results and Discussions
3.1 Financial viability of bioelectricity from forest residue
The financial feasibility analysis of harvest residue utilization for bioelectricity production was found to be a profitable venture with an NPV of ZAR 7,049,336.64 and an IRR of 15.8%. The NPV profile calculations (Figure ) are presented at various discount rates, from 5 to 35%, determining whether the project is feasible or not. The feasibility indicator is, if the NPV is higher than zero the project is feasible and not feasible if otherwise. A higher discount rate increased the denominate in the NPV calculation (EquationEquation (1)(1)
(1) ), making the project financially unfeasible. Whereas, a lower discount rate caused NPV to increase, thereby improving the financial feasibility of the project. The harvest residue gasification plant becomes unprofitable at a discount rate of 16% and above. The NPV profile behavior is inversely proportional to the discount rate. The NPV variation underlines its sensitivity to the discount rate.
Figure 2. Harvest residue gasification feasibility as a function of discount rate and NPV profile variation.

South Africa’s Department of Energy in its Integrated Resource Plan (IRP) for electricity supply 2010–30 predicted that energy demand will increase by an average of 4.6 percent over the next 20 years (Bali, Citation2018; International Institute for Environment and Development IIED, Citation2013). The profitability of harvest residue gasification for bioelectricity as illustrated in this study offers a prospect for expanding the renewable energy share of this projected increase in energy demand. The forest residue gasification plant showed reasonable financial feasibility making this process fit for integrated timber and bioelectricity production (J. S. Cardoso et al., Citation2020). Previous studies have cited the high cost of forest residues supply chain as a hindrance to their use in energy production (Cambero et al., Citation2015). However, the finding from this study has shown that when harvest residues are internalized as a by-product of existing forest operations, they are financially feasible feedstocks for bio-electricity production (Daioglou et al., Citation2016).
The current reliance on large-scale coal and a centralized grid has proven to be no solution for the long-term energy demand in South Africa (International Institute for Environment and Development IIED, Citation2013). It, therefore, makes great sense to support the development of harvest residue gasification for electricity production in conjunction with conventional plantation forestry operations. South Africa has a low share of renewable electricity. Only 3.1% of total electricity production in the South is from renewables as of 2016 (Bali, Citation2018). Currently, in South Africa, only 1.5% of timber harvest residues are utilized (DOE, 2011). Interestingly, only 0.1% of renewable electricity produced in South Africa is from biomass (Bali, Citation2018). There is therefore huge potential and opportunity for harvest residue gasification for electricity production. The implications for rural development could be far-reaching if generated bioelectricity can supply a notable portion of the energy needs.
3.2 The financial impact of integrating forest residue gasification into conventional plantation forestry investment
Table shows the result of the financial impact of integrating timber harvest residue gasification for electricity production into conventional forestry operations. The comparison was based on per ha and per annum cost and revenue of integrated conventional plantation forestry and bioelectricity production. The integration of bioelectricity production improved the IRR from 15.10% to 18.31% while at the same time improving the NPV from ZAR 10,334.86 to ZAR 15,378.46.
Table 3. Financial impact of integrated plantation forestry and bioelectricity production
Figure graphically illustrates the responses of NPVs of conventional plantation forestry and integrated conventional forestry and harvest residue gasification plant to different discounting rates. The integrated approach tends to be more profitable to lower discount rates at a higher rate than the conventional approach. Even at a higher discount rate, the NPV of the conventional approach is more likely to become unprofitable than the NPV of the integrated approach. For instance, at the discount rate of 10%, the NPV improves from 20,334.86- to 38,000.00. This further reinforces the finding that forest residue gasification for electricity produces a positive impact on the financial feasibility of conventional plantation forestry performance.
Figure 3. NPV comparison of conventional plantation forestry and integrated forestry and bioelectricity production.

The gasification plant is designed to utilize timber harvest residue from the industrial tree plantations in the district as a feedstock. Hence the plant is not directly competitive with traditional demands for timber. In this regard, harvest residue is seen as a by-product of conventional forestry operations (J. Cardoso et al., Citation2019). As evidenced by the study found, the integration of bioelectricity production from harvest residue can improve the financial performance of conventional forestry operations significantly. Integrated timber and bioelectricity production can provide additional benefits beyond financial benefits (Saunders et al., Citation2012). A 2010 European Commission report on conversion efficiency essentials for biomass electricity found that harvest residue-based bioelectricity production is more carbon neutral than other biomass sources (Baker et al., Citation2018; Jean-François et al., Citation2011). Additionally, Claudia Cambero et al. (Citation2015) found that harvest residue-based bioelectricity production has the capacity to generate added profit for the forest industries. These findings correspond with the findings of this study and make harvest residue-based bioelectricity production a worthwhile investment in actualizing economic growth and sustainable energy in rural communities in developing countries.
3.3 Sensitivity of investment in forest residue gasification
Figure shows the NPV risk projection histograms as a function of the probability density and the cumulative probability (s-curve). The low standard deviation suggests that the anticipated values within the distribution come close to the mean. The implication is that as the standard deviation increases, the investment risk will increase. In Fig. 3.3, the values for NPV probability distribution tend to concentrate around the mean value, resulting in the low standard deviation value (1129138,63). Hence, the harvest residue gasification project posses low-risk probability of investment loss for potential investors. The projected low risk is mainly due to the NPV’s higher sensitivity to changes in the selected input variables. The probability to obtain a negative NPV is given by the whole probability density area percentage at the left of the scale factor 19.7 value, around 5%, while the probability for this investment to surpass the 5 MV NPV is about 5% to the right of the scale factor 23.5 value (Figure ).
Further analysis of how the uncertainty from the costs variables associated with the forest residue gasification plant affects the NPV are presented in Figure . The figure presents the impact of switch in each input variable on the NPV and their sensitivity range. The results showed that the project’s profitability is most sensitive to the investment costs of the gasification plant, followed by the transport costs for harvest residues, wages and salary, and maintenance costs of the gasification plant. The three variables that the NPV is least sensitive to are our income from a timber sale, harvesting cost, and Depreciation cost. Investment costs, transport costs, wages, and salary show considerable impact change on NPV when compared to the remaining input variables. Further, it can be deduced from Figure that none of the considered critical variables produced a negative effect on the NPV even in a most pessimistic scenario. This makes the harvest residue gasification project investment worth considering.
Conclusion and recommendations
The financial feasibility of timber harvest residue gasification for electricity and its financial impact on plantation forestry management in South Africa was studied. This was prompted by the increasing policy attention to the environmental problems posed by climate change and the ongoing challenge of insufficient electricity supply in South Africa, which have caused the national Government to adopt strategic plans to increase the renewable energy share of electricity production in the country. This policy target has important implications for the forest sector because of the potential to utilize forest biomass in bioelectricity production. In support of this policy strategy, this study conducted a financial feasibility assessment of bioelectricity production from timber harvest residues and the financial impact that such a strategy will have on conventional plantation forestry operations.
A spreadsheet financial model was developed to deliver the financial feasibility analysis using NPV and IRR indicators. The financial feasibility assessment of the 5 MW used for the study showed that the project is economically feasible under current market conditions, showing a positive NPV of ZAR 7,049,336.64 and an IRR of 15.8%. Additionally, the integration of the harvest residue gasification strategy with conventional plantation forestry operations was found to improve the financial performance of conventional forestry operations with improved the IRR from 15.10% to 18.31% while at the same time improving the NPV from ZAR 10,334.86 to and ZAR 15,378.46.
The sensitivity analysis showed a lower risk of failure in the NPV, with investment costs, transport costs for harvest residues, wages and salary, and maintenance costs causing higher impact change over this method. Despite the financial feasibility of the project and the low risk projected by the financial risk analysis, there are some issues worth considering for potential investors. The sensitivity of the system to the initial high investment costs required for the project warrants special consideration when investing in this project. Moreover given the lack of policy guarantee on electricity sale from biomass gasification for electricity in South Africa at the moment, the attractiveness of this project to suitable investors may be hampered, in a situation where the objective is to sell generated electricity to the wider public.
To upscale the adoption of integrated conventional plantation forestry and bioelectricity production, the following recommendations are suggested:
Recovering harvest residues from forestland might impact the ecological condition of forest land in the long term. The study propose that the ecological impact of residue recovery be evaluated in future research with respect to tropical developing country context.
The impact of timber harvest residue recovery for electricity production is closely related to local livelihood strategy in most developing country context. Thus, a region-specific socioeconomic impact assessment should be required for biomass gasification projects in developing country context.
*NB: It is important to point out that that the financial analysis (cost and revenue estimation) of bioelectricity production from harvest residue as carried out in this study, is based on the South Africa National Energy Regulator (NERSA) approved renewable energy feed-in tariff (REFIT) scheme. This scheme has ended but yet to be replaced. Hence, this study at the very least, represents the feasibility of bioelectricity production in South Africa, given the previously existing policy framework.
Disclosure statement
No potential conflict of interest was reported by the author.
Data Availability Statement
The data that support the findings of this study are available from the corresponding author upon reasonable request.
Additional information
Notes on contributors

Chidiebere Ofoegbu
Chidiebere Ofoegbu is a forest and environmental scientist with interest in the socioecological aspect of nature, ecosystems services and biodiversity management. He is enthusiastic about the linkages between science and policy development. His research interests focus on landscape and natural resources sustainability and ecosystem services, particularly on the economic, policy, and social dimensions of these. As a broadly trained natural resource scientist, Chidiebere is well suited to understand and communicate the connections among the ecological, economic, policy, and social dimensions of these systems within the context of sustainable development. At the Centre for Landscape and Climate Research, University of Leicester, Chidiebere is part of the project team working on the development of landscape decisions framework for a holistic land use system that is coherent with policy goals on Net zero emissions, renewable energy uptake, climate change mitigation and adaptation, biodiversity conservation, and multifunctional landscapes. This publication contributes to ongoing works on land use systems for sustainable renewable energy.
References
- Abdul Salam, P., Kumar, S., & Siriwardhana, M. (2010). The Status of Biomass Gasification in Thailand and Cambodia. In Pathum Thani 12120. Asia Institute of Technology.
- Baker, J. S., Crouch, A., Cai, Y., Latta, G., Ohrel, S., Jones, J., & Latané, A. (2018). Logging residue supply and costs for electricity generation: Potential variability and policy considerations. Energy Policy, 116, 397–11. https://doi.org/10.1016/j.enpol.2017.11.026
- Bali, T. (2018). South Africa 2018 update: Bioenergy policies and status of implementation. 3063 IEA Bioenergy. chrome-extension://efaidnbmnnnibpcajpcglclefindmkaj/https://www.ieabioenergy.com/wp-content/uploads/2018/10/CountryReport2018_SouthAfrica_final.pdf
- Beke, N., Fox, G., & Mckenney, D. (1996). A Financial Analysis of Using Sawmill Residues for Cogeneration in Northern Ontario. Energy Studies Review, 8(1), 16–26. https://doi.org/10.15173/esr.v8i1.381
- Boerner, J., Baylis, K., Corbera, E., Ezzine de Blas, D., Ferraro, P. J., Honey-Roses, J., Renaud Lapeyre, U., Persson, M., Wunder, S., & Bond Lamberty, B. (2016). Emerging Evidence on the Effectiveness of Tropical Forest Conservation. PloS One, 11(11), e0159152. https://doi.org/10.1371/journal.pone.0159152
- Burgess, J. C. (1993). Timber Production, Timber Trade, and Tropical Deforestation. Ambio, 22(2/3), 136–143. http://www.jstor.org/stable/4314058
- Call, M., Mayer, T., Sellers, S., Ebanks, D., Bertalan, M., Nebie, E., & Gray, C. (2017). Socio-environmental drivers of forest change in rural Uganda. Land Use Policy, 62, 49–58. https://doi.org/10.1016/j.landusepol.2016.12.012
- Cambero, C., Sowlati, T., Marinescu, M., & Röser, D. (2015). Strategic optimization of forest residues to bioenergy and biofuel supply chain. International Journal of Energy Research, 39(4), 439–452. https://doi.org/10.1002/er.3233
- Camia, A., Giuntoli, J., Jonsson, R., Robert, N., Cazzaniga, N. E., Jasinevičius, G., Avitabile, V., Grassi, G., Barredo, J. I., & Mubareka, S. (2021). The use of woody biomass for energy purposes in the EU, EUR 30548. EN, Publications Office of the European Union.
- Cardoso, J., Silva, V., & Eusebio, D. (2019). Techno-economic analysis of a biomass gasification power plant dealing with forestry residues blends for electricity production in Portugal. Journal of Cleaner Production, 212, 741–753. https://doi.org/10.1016/j.jclepro.2018.12.054
- Cardoso, J. S., Silva, V., Eusébio, D., Azevedo, I. L., & Tarelho, L. A. C. (2020). Techno-economic analysis of forest biomass blends gasification for small-scale power production facilities in the Azores. Fuel, 279, 1–11. https://doi.org/10.1016/j.fuel.2020.118552
- Cheng-Lin, J., Zhen-Mei, W., Shu-Wen, W., Zheng-Qun, C., Chen, T., Farahani, M. R., & De Xun, L. (2017). Economic assessment of biomass gasification and pyrolysis: A review. Energy Sources, Part B: Economics, Planning, and Policy, 12(11), 1030–1035. https://doi.org/10.1080/15567249.2017.1358309
- Clarke, J., Clarke, J. M., Foy, T., & Foy, T. J. (1997). The role of the forest industry in rural development and land reform in South Africa. The Commonwealth Forestry Review, 76(3), 175–178. http://www.jstor.org/stable/42608588
- Colantoni, A., Villarini, M., Monarca, D., Carlini, M., Mosconi, E. M., Bocci, E., & Hamedani, S. R. (2021). Economic analysis and risk assessment of biomass gasification CHP systems of different sizes through Monte Carlo simulation. Energy Reports, 7, 1954–1961. https://doi.org/10.1016/j.egyr.2021.03.028
- Daioglou, V., Stehfest, E., Wicke, B., Faaij, A., & Van Vuuren, D. P. (2016). Projections of the availability and cost of residues from agriculture and forestry. GCB Bioenergy, 8(2), 456–470. https://doi.org/10.1111/gcbb.12285
- Forest Economics Services (FES). (2008). Financial analysis and costs of forestry operations, Mpumalanga province-. Forest Economics Services, Mpumalanga, South Africa.
- Huang, H., Yan, X., Song, S., Du, Y., & Guo, Y. (2020). An Economic and Technology Analysis of a New High-Efficiency Biomass Cogeneration System: A Case Study in DC County, China. Energies, 13(15), 1–20. https://doi.org/10.3390/en13153957
- International Institute for Environment and Development (IIED). (2013). South African biomass energy: Little heeded but much needed.
- International Renewable Energy Agency: IRENA. (2017). Biofuel Potential in Sub-Saharan Africa: Raising food yields, reducing food waste and utilizing residues, Assessed: 29-March-2022. International Renewable Energy Agency, Abu Dhabi. https://www.irena.org/-/media/Files/IRENA/Agency/Publication/2017/Nov/IRENA_Biofuel_potential_sub-Saharan_Africa_2017.pdf
- Jean-François, C., MacLean, D. A., Erdle, T. A., & Roy, R. J. (2011). Integration of bioenergy strategies into forest management scenarios for Crown land in New Brunswick, Canada. Canadian Journal of Forest Research, 41(6), 1319–1332. https://doi.org/10.1139/x11-048
- Jin, E., & Sutherland, J. W. (2018). An integrated sustainability model for a bioenergy system: Forest residues for electricity generation. Biomaa and Bioenergy, 119, 10–21. https://doi.org/10.1016/j.biombioe.2018.09.005
- Kizha, A. R., & Han-Sup, H. (2015). Forest residues were recovered from whole-tree timber harvesting operations. European Journal of Forest Engineering, 1(2), 46–55.
- Klemperer, W. D. (2003). Forest resource economics and finance. McGraw-Hill inc.
- Leung, D. Y. C., Yin, X. L., & Wu, C. Z. (2004). A review on the development and commercialization of biomass gasification technologies in China. Renewable and Sustainable Energy Reviews, 8(6), 565–580. https://doi.org/10.1016/j.rser.2003.12.010
- Lundqvist, A. (2020). Future development of bioenergy in South Africa. Mälardalen University. chrome-extension://efaidnbmnnnibpcajpcglclefindmkaj/https://www.diva-portal.org/smash/get/diva2:1443396/FULLTEXT01.pdf
- Lyons-White, J., Pollard, E. H. B., Catalano, A. S., & Knight, A. T. (2018). Rethinking zero deforestation beyond 2020 to more equitably and effectively conserve tropical forests. One Earth, 3(6), 714–726. https://doi.org/10.1016/j.oneear.2020.11.007
- Malkamäki, A., D’Amato, D., Hogarth, N. J., Kanninen, M., Pirard, R., Toppinen, A., & Zhou, W. (2018). A systematic review of the socio-economic impacts of large-scale tree plantations, worldwide. Global Environmental Change, 53, 90–103. https://doi.org/10.1016/j.gloenvcha.2018.09.001
- Mayaki, A. I. 2008. Sustainable Bioenergy Development in UEMOA Member Countries [ Online] http://d.scribd.com/docs/lxw2r89y9cpzy1ngj5i.pdf [December 5, 2008].
- Miner, R., & Lucier, A. (2004). A Value Chain Assessment of Climate Change and Energy Issues Affecting the Global Forest-Based Industry. WBCSD Sustainable Forest Products Industry Working Group.
- Ofoegbu, C. (2010). An evaluation of the socio-economic impact of timber production with and without the inclusion of biomass energy production. MSc Thesis, University of Stellenbosch,
- Ofoegbu, C., & Ifejika-Speranza, C. (2021). Making climate information useable for forest-based climate change interventions in South Africa. Environmental Sociology, 7(4), 279–293. https://doi.org/10.1080/23251042.2021.1904534
- Penniall, C. L., & Williamson, C. J. (2009). Feasibility study into the potential for gasification plant in the New Zealand wood processing industry. Energy Policy, 37(9), 3377–3386. https://doi.org/10.1016/j.enpol.2008.11.046
- Pereira, E. J., Pinho, J. T., Galhardo, M. A. B., & Macêdo, W. N. (2014). Methodology of risk analysis by Monte Carlo Method applied to power generation with renewable energy. Renewable Energy, 69, 347–355. https://doi.org/10.1016/j.renene.2014.03.054
- Persson, T. (2007). Standard & Generic Integrated Gasification and Combined Heat & Power (CHP) Plant. A project proposal submitted to Mondi Zimele by GreenForze.
- Pokharel, R., Grala, R. K., & Grebner, D. L. (2017). Woody residue utilization for bioenergy by primary forest products manufacturers: An exploratory analysis. Forest Policy and Economics, 85, 161–171. https://doi.org/10.1016/j.forpol.2017.09.012
- Porcu, A., Sollai, S., Marotto, D., Mureddu, M., Ferrara, F., & Pettinau, A. (2019). Techno-Economic Analysis of a Small-Scale Biomass-to-Energy BFB Gasification-Based System. Energies, 12(3), 1–17. https://doi.org/10.3390/en12030494
- Premer, M. I., Froese, R. E., & Vance, E. D. (2019). Whole-tree harvest and residue recovery in commercial aspen: Implications to forest growth and soil productivity across a rotation. Forest Ecology and Management, 447, 130–138. https://doi.org/10.1016/j.foreco.2019.05.002
- Puttock, G. D. (1995). Estimating cost for integrated harvesting and related forest management activities. Biomass & Bioenergy, 8(2), 73–79. https://doi.org/10.1016/0961-9534(95)00001-N
- Ratnasingam, J., Wai, L. T., Thanasegaran, G., Florin Ioras, F., Vacalie, C., Coman, C., & Wenming, L. (2013). Innovations in the Forest Products Industry: The Malaysian Experience. Ratnasingam J et al ,Not Bot Horti Agrobo, 41(2), 601–607. https://doi.org/10.15835/nbha4129339
- Saunders, A. M., Aguilar, F. X., Dwyer, J. P., & Stelzer, H. E. (2012). Cost Structure of Integrated Harvesting for Woody Biomass and Solid Hardwood Products in Southeastern Missouri. Journal of Forestry, 110(1), 7–15. https://doi.org/10.5849/jof.10-072
- Savvides, S. (1994). Risk analysis in investment appraisal. Project Appraisal, 9(1), 1. https://doi.org/10.1080/02688867.1994.9726923
- Scudder, M. G., Herbohn, J., & Baynes, J. (2019). Are portable sawmills a financially viable option for economic development in tropical forests? Forest Policy and Economics, 100, 188–197. https://doi.org/10.1016/j.forpol.2018.12.011
- Situmorang, Y. A., Zhao, Z., Yoshida, A., & Abudula, A. (2020). Small-scale biomass gasification systems for power generation (<200 kW class): A review. Renewable and Sustainable Energy Reviews, 117, 1–14. https://doi.org/10.1016/j.rser.2019.109486
- Trading Economics. ( den15 October. 2021). Trading Economics. South Africa - Gross Savings - Gross Savings (% of GDP): https://tradingeconomics.com/south-africa/indicators
- Tripathi, A. K., Iyer, P. V. R., & Kandpal, T. C. (1997). A financial evaluation of biomass-gasifier-based power generation in India. Bioresource Technology, 61, 53–59. https://doi.org/10.1016/S0960-8524(97)84699-8
- Weiss, G., Ludvig, A., & Živojinovića, I. (2020). Four decades of innovation research in forestry and the forest-based industries – a systematic literature review. Forest Policy and Economics, 120(102288), 1–25. https://doi.org/10.1016/j.forpol.2020.102288
- Wu, C. Z., Huang, H., Zheng, S. P., & Yin, X. L. (2002). An economic analysis of biomass gasification and power generation in China. Bioresource Technology, 83(1), 65–70. https://doi.org/10.1016/S0960-8524(01)00116-X
- Yagi, K., & Nakata, Y. (2011). Economic analysis on small-scale forest biomass gasification considering geographical resources distribution and technical characteristics. Biomass & Bioenergy, 35, 2883–2892. https://doi.org/10.1016/j.biombioe.2011.03.032