Abstract
An immunoisolated collection of cells, which communicate and exchange essential factors, co‐stimulatory hormones, as well as providing immunoprotection and immunomodulation, can be prepared, given existing scientific and medical know‐how, within two decades. These “Bioartificial Organ Grafts” have advantages relative to isolated cell therapies, including β‐cell encapsulation for diabetes treatment, and xenotransplantation, which has a de facto moratorium. This paper documents that the majority of the research for the bioartificial organ grafts has been concluded, with the remaining hurdles minimum in comparison. The use of co‐encapsulation and the induction of local immune‐privilege will provide a more sensitive humoral hormonal response and graft survival, without systemic immunosuppression. A call for the staged implementation of bioartificial organ grafts, based on the best available medical practice, materials, tissue and technology available, is advocated. The implementation of bioartificial organ grafts can begin within the next two years, based on allografts succeeded by genetically modified human tissue, without the need to pass through a xenograft stage.
Introduction
The economic costs for standard medical treatment have contributed to the re‐evaluation of the affordability of public and private health care options in many countries. In the United States alone hundreds of billion dollars, and approximately 10% of GNP, are spent on the treatment neurodegenerative and endocrine diseases, inborn errors of metabolism, as well as a variety of acute and chronic organ failures. Eighty five percent of these expenses are due to the morbidity associated with the diseases. For diabetes alone, the incidence of which increases by three to four percent per year (EURODIAB ACE Study Group, [Citation2000]), the annual direct and indirect costs exceeds ninety billion dollars in the US (Citation[[NIH Implementation of the Recommendations of the Congressionally Directed Diabetes Research Working Group]). Selected countries reporting higher per capita costs related to long‐term monitoring and care as well as secondary complications. Given the potential of bioartificial organs to treat these diseases, the societal and pharmacoeconomic impact of such devices would be important. In North America, this includes six million patients which have neurodegenerative diseases such as Alzheimer's and Parkinson's, sixteen million with diabetes and thousands of others with hemophilia or renal and liver failure.
Present therapies can, in certain situations such as the treatment of diabetes, regulate the disease through hormone and drug treatments, though morbidity and mortality remain high. Furthermore, despite excellent recent results (Shapiro et al., [Citation2000]), islet allotransplantation is not a cure since the tissue can be ultimately rejected despite immunosuppression. In addition, due to the side effects of immunosuppression, islet transplantation can be applied only to worst‐case patients such as brittle diabetics. Moreover, the number of patients waiting for such a transplantation proceeding exceeds, by far, the available number of human organs and tissue. Therefore, given the need for continuous immunosuppression and the lack of donors for allogeneic organ, tissue or cell transplantation, research has blossomed in the area of bioartificial organs,Footnotea following pioneering efforts by various groups (Arkadopoulos et al., [Citation1998]; Chang, [Citation1964]; Lim and Sun, [Citation1980]) including the very successful long‐term studies recently reported by Duvivier‐Kali et al. ([Citation2001]).
To date, bioartificial organ research has been directed toward specific, isolated freely or immunoprotected transplanted cells, predominantly hepatocytes (Arkadopoulos et al., [Citation1998]; Sundback and Vacanti, [Citation2000]), islets (Lim and Sun, [Citation1980]; Sun et al., [Citation1996]), keratinocytes (Hollander et al., [Citation2001]; Ronfard et al., [Citation2000]), and chondrocytes (Ibarra et al., [Citation2000]; Jackson et al., [Citation2001]). There have been some spectacular early successes in rodent models, with limited preclinical testing in dogs (Humes et al., [Citation1999]) and primates (Sun et al., [Citation1996]) and some anecdotic clinical reports (Soon‐Shiong, [Citation1999]; Soon‐Shiong et al., [Citation1994]). These include the extracorporeal immunoisolated hepatocytes, which contact plasma indirectly, and bioartificial skin for extensive burns. However, both are used as bridging technologies when comatose patients are on the waiting list for liver transplants, or appropriate natural skin is unavailable.
One of the problems in immunoisolating a given cell is the lack of interaction with the complex milieu of neighboring cells, which characterize any given organ. One could, therefore debate if bioartificial organs should be developed to mimic the body's complexity, or continue, as at present, with alternatives, which provide selected, isolated, function. Could not a defined mixture of cells simultaneously encapsulated and transplanted, mimic organs such as pancreas, liver and kidney, be equally attractive to the promise of cell‐ or tissue‐engineering? At present, cell therapy treating a variety of neurodegenerative diseases such as Huntington's Corea, are under investigation in various centers (Freed et al., [Citation2001]; Freeman et al., [Citation2000]). However, clinical success has been limited. This is also true for hormone deficient diseases such as diabetes where the number of allotransplants carried out to date still numbers in the hundreds and the average length of post‐transplant insulin independence measured in months, prior to 1998 (Hering and Ricordi, [Citation1999]; Oberholzer et al., [Citation2000]; Shapiro et al., [Citation2000]). However, this has now been improved to over one year using the Edmonton Protocol (Ryan et al., [Citation2001]). One could question whether an isolated cell type can restore an altered organ function. Therefore, the authors postulate that an immunoisolated collection of different cell types may better mimic the function of a complex organ. This is the core of the concept of the “Bioartificial Organ Graft,” as is schematized in . Bioartificial grafts could be a clinical possibility within the next two decades, as will be demonstrated herein, based on recent advances and emerging therapies, including
Figure 1. (A) A schematic showing the transplantation site of a bioartificial graft. The cutout shows the anticipated composition of a bioartificial organ graft for the treatment of diabetes, revealing microencapsulated islets, sertoli cells and hepatocytes in a single immunoisolation device. (B) A photomicrograph of a bioartificial islet graft composed of reversibly immortalized human beta, alpha and sertoli cells in a microcapsule, transplanted intraportally.
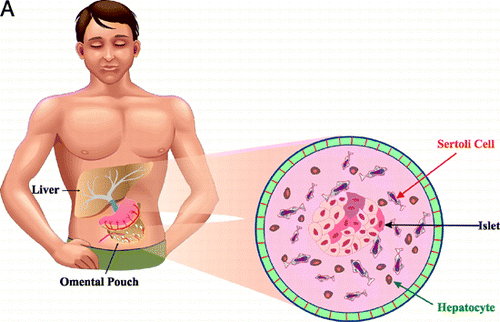
Alternative Cell and Tissue Sources
Stem cells display the ability to differentiate in culture into a variety of cell lineages. They can be of embryonic origin or can be isolated from different type of tissues. Apart from ethical considerations, the use of human embryonic stem cells has various legal and political limitations.
Cell lines. The demonstration that various cell lines (Salmon et al., [Citation2000]) such as hepatocytes (Kobayashi et al., [Citation2000a]) and β‐cells (Ramiya et al., [Citation2000]; Soria et al., [Citation2000]), can be reversibly immortalized (Kobayashi et al., [Citation2000b]), while maintaining their specific characteristics.
Neonatal xenogeneic tissue. This includes porcine islets which are less expensive to isolate, more robust and offer handling and stimulation advantages relative to mature porcine tissue (Korbutt et al., [Citation1997a]; Rayat et al., [Citation1999]). Neonatal porcine islets are also expandable in culture.
Cryopreservation of tissue for banking which is now possible in high yields (Korbutt et al., [Citation1997b]; Rajotte, [Citation1999]).
Immunomodulation
Coencapsulation of natural and genetically modified cells, which secrete growth factors and hormones, or provide local immunosuppression. For example, in vitro islet survival and insulin production are prolonged via co‐transplantation with sertoli cells (Korbutt et al., [Citation1997c]; Sanberg et al., [Citation1996], [Citation1997]; Suarez‐Pinzon et al., [Citation2000]) or with Fas‐L infected myoblasts (Lau et al., [Citation1996]).
Gene therapy. As an example the transfection of islets with immunomodulatory genes such as Fas L and CTLA4‐IG (Brady and Lew, [Citation2000]; Gainer et al., [Citation1998]) or antiapoptotic genes such as bcl‐2 (Contreras et al., [Citation2001]).
Immunoisolation
Availability of highly endotoxin‐reduced polymeric biomaterials.
Polyelectrolytes for encapsulation eliciting a minimal host response (Duvivier‐Kali et al., [Citation2001]; Schrezenmeir et al., Citation[1993]; Soon‐Shiong et al., [Citation1992]; Wang et al., [Citation1997]).
Alternative transplant sites (Ao et al., [Citation1993]), such as intraportal injections of microcapsules (Leblond et al., [Citation1999]).
Allotransplantation versus Xenotransplantation: Diabetes Case Study
The xenotransplantation debate (Bach and Fineberg, [Citation1998]) can be appreciated by using diabetes therapy as an example, since bioartificial pancreas research dates to the 1970s. Porcine‐based tissue offers scientific and ethical advantages due to the similarity of human and pig insulin, which differ by a single amino acid residue. Furthermore, given that over two hundred millions pigs are slaughtered annually in the US, societal acceptance of pigs as potential tissue banks is expected. However, there remains concern in regards to PERV (porcine‐endogenous retroviruses) with the risk of transmission to human recipient (Patience et al., [Citation1997]; van der Laan et al., [Citation2000]), although clinical evidence is still lacking (Elliott et al., [Citation2000]; Paradis et al., [Citation1999]). Moreover, phylogenetic disparities present a major immunological obstacle with exposure of isolated islets, to in vitro and in vivo blood, resulting in acute islet damage (Bennet et al., [Citation2000]). Specifically, van der Laan reported that pig islets can infect human cells in culture, providing the first evidence that PERV is transcriptionally active and infectious across species. Therefore, several advocates have recently discussed the advantages of publicly‐based allotransplantation over privately‐controlled xenotransplantation as a therapy, from a risk‐balance perspective (Hunkeler et al., [Citation1999]).
It has become clear that the fundamental concept of immunoisolation has to be re‐defined as a partial rather than complete barrier. Tissue contained within a barrier membraneFootnoteb has been shown to frequently produce an immune response in the host, albeit modified, and this is particularly true in xenotransplantation. Simply, while a porcine graft may be shielded from the host, the reverse is not true with the egress from the graft activating a response from the host. However, the fact that the immune response is attenuated provides opportunity for effective use of immunomodulation, and other maneuvers that may not be effective without the artificial barrier. The idea to combine immunomodulation, immunoisolation and more than one transplanted tissue is the basic premise of the bioartificial organ graft, as will be developed herein. Furthermore, as our knowledge of the molecular basis for immunological tolerance, and related phenomena, increases there will be the opportunity to incorporate these molecular signals on the barrier membrane surface, perhaps then achieving full protection for the graft.
A large number of public and private research organizations, world wide, have dedicated thousands of person years of time to a mostly xenografts related bioartificial pancreas as is shown in . However, there remains little evidence that porcine tissue can function clinically (Groth et al., [Citation1994]), the PERV debate aside. On the contrary, there have been excellent clinical results involving allotransplantation of islets for diabetes reversal (Ryan et al., [Citation2001]; Shapiro et al., [Citation2000]). Therefore, the xeno–allo debate centers around one alternative that offers unlimited tissue supply without the demonstration of efficacy and the second alternative, which is a clinical therapy, though restricted by the shortage in human tissue. Furthermore, in regard to the development of the bioartificial pancreas, which has been the most extensively investigated bioartificial organ, the aforementioned advances in cell sources, immunomodulation and immunoisolation have not been implemented. For example, there has been a preference for bioartificial organ transplantation into the intraperitoneum, despite recent advances which note that the optimum site for microcapsule transplantation of hormone secreting cells, such as islets, is one that drains directly into the liver (e.g. intraportal injection, omental pouch) (Ao et al., [Citation1993]).
Table 1. Summary of Public and Private Bioartificial Pancreas Research (1980–2001)
The following section will define the concept of, and the agenda for, bioartificial organ grafts. Bioartifical grafts should take advantage of the progress in cell sourcing and immunomodulation/isolation while avoiding mistakes, which delayed the development of the bioartificial pancreas.
Bioartificial Organ Grafts: The Concept and Agenda
The authors believe that medical professionals and scientists, will, within two decades, have optimized essential elements in the development of bioartificial organ grafts, including:
Immortalized cell lines.
Long‐term protection and banking of primary tissues such as islets and hepatocytes, either via direct cyropreservation or cyropreservation after encapsulation.
Families of immunoprotective materials, including substitution lists for surgeons specific for various transplant sites and with tunable permeabilities and mechanical properties (Bartkowiak et al., [Citation1999]; Prokop et al., [Citation1998a], Citation[b]).
Appropriate scaled‐up technologies for the production of clinically relevant quantities of immunoisolated cells under sterile conditions (Citation[[Wang et al.]).
It is anticipated that advances in clinical cell transplantation therapy will follow an additive scheme implementing, at any given instant, the best available science, technology and medical practice. illustrates an example of such a perceived development for the treatment of diabetes via islet transplantation. At the outset it is anticipated that both immunosuppression and immunoisolation will be used in conjunction with allografted tissue. After some time it is believed that immunosuppression can be eliminated by appropriately pairing the immunoisolation material and transplant site. Ultimately, the substitution of primary allografts with human‐based genetically modified cells will take place, first with, then without immunosuppression.
Figure 2. The evolution of a clinical therapy for diabetes based on a bioartificial pancreas. The earliest strategy will involve the use of encapsulated allografted islets, under immunosuppression, with graft prolongation due to co‐encapsulation as well as genetic modifications to the islets which provide immunomodulation and vascularization Strategy 1 is basically an immunoprotected extension of the Edmonton Protocol (3) which rendered brittle diabetics insulin independent for approximately twelve months. It is based on over two decades of development. Eventually, due to optimization of islet handling and pretreatment, co‐encapsulation and in situ graft protection, the need for immunosuppression could be eliminated (Strategy 2). Given the ultimate requirement of replacing allotransplantation with cultured tissue to treat all type I diabetics, genetically modified cell lines will be developed and applied in combination with the approaches followed in Strategies 1 and 2. Within three decades genetically modified cells should replace islets, first with immunosuppression (Strategy 3) and ultimately without (Strategy 4).
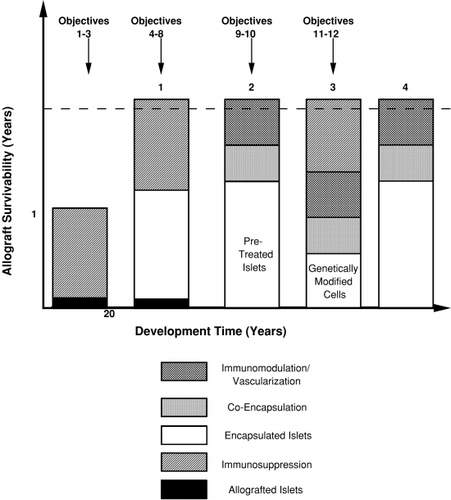
Allotransplantations could treat tens of thousands of allotransplants per year within five years (Hunkeler, [Citation1999]) based on the current availability of cadaver tissue. This will make a significant impact on those requiring organ transplantation, though it will not be sufficient to treat, for example, all diabetics. However, given the perceived and demonstrated risks involved in xenografts, the technical difficulties in immunoisolating xeno‐tissue, the recent evidence of PERV infections (van der Laan et al., [Citation2000]) will act as a, de facto, decade long moratorium on xenotransplantation. Therefore, given the enormous promise of pluripotent stem cells, and the successes in cell transplantation in replacing risky whole organ transplantation [e.g. Edmonton Protocol (Ryan et al., [Citation2001]; Shapiro et al., [Citation2000])] it seems reasonable to expect that allografts will be succeeded by genetically modified human cells without the need to pass through a xeno‐based stage. Therefore, early clinical trials will quite likely be based on primary human tissue as research on genetically modified cells advances. From a risk perspective publicly financed, and practiced, allotransplantation seems to be overwhelmingly preferred to privately controlled xenotransplantation.
The following plan itemizes objectives, which will likely contribute, in three largely sequential stages, to the routine clinical implementation of an immunosuppression‐free bioartificial organ graft by 2020.
Stage I: 2001–6
Cell‐Based Research
Developmental biology for identifying cell‐specific markers of stem cells and progenitor cells of the different target cells.
Exploration of the potential use of human embryonic or adult stem cells in the formation of human cell lines.
Understanding the key signals, signaling pathway components and transcription factors that regulate the fate of parenchymal cells and their regeneration.
The role of interactions between differentiating cells and extracellular matrices in the morphogenesis of organs, the development and culture of surrogate cells, which could permanently retain the characteristics of the target cells suitable for implantation in man.
Materials‐Based Research
The microtechnology to produce clinical quantities of sterile bioartificial organ grafts requires further development. Laboratory‐ and commercial‐prototypes will be needed to move the bioartificial organs presently in clinical trials through the regulatoryFootnotec pipeline.
A risk balance between transplant volume and nutrient/oxygen supply will also have to be completed.
At the end of Stage‐I, it is anticipated that bioartificial organs grafts, based on primary allotissue, will be in the clinic for the treatment of several hormone deficient diseases (e.g. diabetes, Parkinson disease) as well as some cancers (). Bioartificial organ graphs are forecasted to be commercialized within this time for as little as 3 cents per capsule. This is an expense, which pharmacoeconomically, the insurance firms will likely advocate relative to other therapies, which have large up‐front and continuing expenses, associated with complicated surgery and morbidity. Therefore, it is anticipated that, like many medical advances including the heart–lung machine, the life cycle perspectives in implementing bioartificial organ graphs, once a certain durability is met, will force their wide spread implementation. We seem close to this threshold for immunoisolated allograft based tissue, which is why the present committee of authors is advocating the bioartificial organ graft agenda.
Stage II: 2006–20
Biomedical Research
Genetic modification of the different cell lineages permitting the elimination of immunosuppression is a mid‐term goal.
Transplant site selection including the evaluation of immuneprivleged sites versus traditional internal organs, intraperitoneal and subcutaneous sites, an omental pouch or multiple sites simultaneously.
Host modification using different approaches of tolerance induction.
Materials‐Based Research
The evaluation of the “site–size hypothesis” which postulates that the geometry, and chemistry of a bioartificial organ graft, will be dependent on the transplant site requires investigation. Different characteristics are anticipated for microcapsules used in internal organs (e.g. intraportal injection) and in the intraperitoneum (Angelova and Hunkeler, [Citation1999]). Capsule seeding (e.g. with albumin or oxygen to enhance cell viability), has been demonstrated, though the optimization of concentration gradients in immunoprotective devices remains to be achieved.
Many of the aforementioned milestones are under investigation with developments in medicine, biology, microtechnology and materials chemistry all contributing towards the sequential implementation of these new therapies. By the end of stage II, the authors expect that bioartificial organ grafts based on genetically modified tissues could be implemented.
Stage III: Long Term Research (2020 and Beyond) Patients Specific Grafts
Cell source development will likely focus on immortalized and genetically modified cell lines based on the patient's own cells. Those “autografts” tailored for the individual patients are aimed at, ultimately, displacing the transplantation of foreign tissue. If the autograft would require the co‐transplantation of different cell types (bioartificial organ graft) than immunoisolation devices could be considered as containment vehicles with relatively open pores so as to not restrict ingress and egress.
Conclusions
Given the high cost for the treatment of endocrine disorders, the increasing incidence of the disease, and the overall lack of advancement in the transplantation of encapsulated single cell lines, bioartificial organ grafts, and its multiple cell approach, seems to be the most viable path. The stepwise implementation that the authors advocate herein, using the best available medical practice, materials, tissue and technology seems prudent. Simply, the need for therapies to treat the hormone deficient diseases is sufficiently large that we cannot wait until for two or more decades until a perfect solution, including infinite cell availability, is upon us prior to beginning. There are sufficient encouraging advances in allografts to warrant a beginning based on primary human tissue, and it is this starting point, which the authors recommend for the bioartificial organ graft concept.
Clinical testing of the bioartificial organ grafts can proceed very soon (within 24 months) in an allograft model. This would be, initially, directed to patients who have already had a whole organ transplant and are on immunosuppressive therapy, yet suffer from other hormone deficient diseases. Although limited by the number of brain‐dead human donors, the allotransplantation solution can provide, in a few years, cures to tens of thousands of patients though‐out the world.
The hypothesis of bioartificial organ grafts is that cell–cell communication is an essential element in bioartificial organ durability. Therapies to treat specific diseases such as diabetes (bioartificial pancreas) and fulminant liver failure (bioartificial liver) should not be discontinued while awaiting xenotransplantation as the all in one solution. Rather they should be complimented with research, which aims at a more supple duplication of cellular function. The authors are recommending funding of both allograft‐based cell transplantation therapy and the development of genetically modified cells. That is, cell transplantation requires sufficient short term funding to bring it to the clinic, with long‐term support for second generation solutions. Given the promise of cell transplantation, the authors close by calling for an international bioartificial organ graft initiative, which can coordinate the necessary developments and provide staged clinical solutions.
Notes
aBioartificial Organ (Synonym: Biohybrid Artificial Organ): A transplantable device providing a mechanically stable biocompatible, non‐degradable host to living cells. Examples include macro‐ and microencapsulated cells used for immunoisolation such as the bioartificial pancreas (islets), liver (hepatocytes), and parathyroid (thyroid). Applications generally involve allo‐ and xenografts of exocrine tissues, as well as the treatment of neurological disorders and hormone deficient diseases. Human tissue is derived from cadavers with the potential of immortalized cells lines based on pluripotent stem cell technology at the forefront of biomedical research and ethical debates. Bioartificial cartilage, skin and bone belong to a second category of bioartificial organs where cells are seeded to an often biodegradable substrate. The latter class of bioartificial organs can be considered to be part of the general area of Tissue Engineering. Overall, bioartificial organs comprised are comprised of cells immunoisolated by elastic polymers or semi‐permeable membranes derived from synthetic and naturally occurring products such as polysaccharides.
bSuch barrier membranes can be on the exterior of microcapsules (Ramiya et al., [Citation2000]), in sheets (Kobayashi et al., [Citation2000b]) or in tubular constructs (Rayat et al., [Citation1999]).
cIt is speculated, by some, that bioartificial organs will be regulated, by the FDA, as a biologic and a drug.
References
- Angelova N., Hunkeler D. Rationalizing the design of polymeric biomaterials. Trends Biotechnol. 1999; 17: 409–421
- Ao Z., Matayoshi K., Lakey J. R., Rajotte R. V., Warnock G. L. Survival and function of purified islets in the omental pouch site of outbred dogs. Transplantation 1993; 56: 524–529
- Arkadopoulos N., Detry O., Rozga J., Demetriou A. A. Liver assist systems: state of the art. Int. J. Artif. Organs 1998; 21: 781–787
- Bach F. H., Fineberg H. V. Call for moratorium on xenotransplants. Nature 1998; 391: 326
- Bartkowiak A., Hunkeler D. New multicomponent capsules for immunoisolation. Ann. N. Y. Acad. Sci. 1999; 875: 135–145
- Bennet W., Surberg B., Lundgren T., Tibell A., Groth C. G., Richards A., White D. J., Elgue G., Larsson R., Nilsson B., Korsgren O. Damage to porcine islets of Langerhans after exposure to human blood in vitro, or after intraportal transplantation to cynomologus monkeys: protective effects of sCR1 and heparin. Transplantation 2000; 69: 711–719
- Brady J. L., Lew A. M. Additive efficacy of CTLA4Ig and OX40Ig secreted by genetically modified grafts. Transplantation 2000; 69: 724–730
- Chang T. M. Semipermeable microcapsules. Science 1964; 146: 524
- Contreras J. L., Bilbao G., Smyth C. A., Jiang X. L., Eckhoff D. E., Jenkins S. M., Thomas F. T., Curiel D. T., Thomas J. M. Cytoprotection of pancreatic islets before and soon after transplantation by gene transfer of the anti‐apoptotic Bcl‐2 gene. Transplantation 2001; 71: 1015–1023
- Duvivier‐Kali V. F., Omer A., Parent R. J., O'Neil J. J., Weir G. C. Complete protection of islets against allorejection and autoimmunity by a simple barium–alginate membrane. Diabetes 2001; 50: 1698–1705
- Elliott R. B., Escobar L., Garkavenko O., Croxson M. C., Schroeder B. A., McGregor M., Ferguson G., Beckman N., Ferguson S. No evidence of infection with porcine endogenous retrovirus in recipients of encapsulated porcine islet xenografts. Cell Transplant 2000; 9: 895–901
- EURODIAB ACE Study Group. Variation and trends in incidence of childhood diabetes in Europe. Lancet 2000; 355: 873–876
- Freed C. R., Greene P. E., Breeze R. E., Tsai W. Y., DuMouchel W., Kao R., Dillon S., Winfield H., Culver S., Trojanowski J. Q., Eidelberg D., Fahn S. Transplantation of embryonic dopamine neurons for severe Parkinson's disease. N. Engl. J. Med. 2001; 344: 710–719
- Freeman T. B., Cicchetti F., Hauser R. A., Deacon T. W., Li X. J., Hersch S. M., Nauert G. M., Sanberj P. R., Kordower J. H., Saporta S., Isacson O. Transplanted fetal striatum in Huntington's disease: phenotypic development and lack of pathology. Proc. Natl. Acad. Sci. U. S. A. 2000; 97: 13877–13882
- Gainer A. L., Suarez‐Pinzon W. L., Min W. P., Swiston J. R., Hancock‐friesen C., Korbutt G. S., Rajotte R. V., Warnock G. L., Elliott J. F. Improved survival of biolistically transfected mouse islet allografts expressing CTLA4‐Ig or soluble Fas ligand. Transplantation 1998; 66: 194–199
- Groth C. G., Korsgren O., Tibell A., Tollemar J., Moller E., Bolinder J., Ostman J., Reinholt F. P., Hellerstrom C., Andersson A. Transplantation of porcine fetal pancreas to diabetic patients. Lancet 1994; 344: 1402–1404
- Hering B. J., Ricordi C. Islet transplantation for patients with type 1 diabetes. Graft 1999; 2: 12–27
- Hollander D. A., Soranzo C., Falk S., Windolf J. Extensive traumatic soft tissue loss: reconstruction in severly injured patients using cultured hyleron‐based three‐dimensional dermal and epidermal autografts. J. Trauma 2001; 50: 1125–1136
- Humes H. D., Buffington D. A., MacKay S. M., Funke A. J., Weitzel W. F. Replacement of renal function in uremic animals with a tissue‐engineered kidney. Nat. Biotechnol. 1999; 17: 451–455
- Hunkeler D. Bioartificial organs transplanted from research to reality. Nat. Biotechnol. 1999; 17: 335–336
- Hunkeler D., Sun A. M., Korbutt G. S., Rajotte R. V., Gill R., Calafiore R., Morel P. Bioartificial organs and acceptable risk. Nat. Biotechnol. 1999; 17: 1045
- Ibarra C., Koski J. A., Warren R. F. Tissue engineering meniscus: cells and matrix. Orthop. Clin. North Am. 2000; 31: 411–418
- Jackson D. W., Scheer M. J., Simon T. M. Cartilage substitutes: overview of basic science and treatment options. J. Am. Acad. Orthop. Surg. 2001; 9: 37–52
- Kobayashi N., Fujiwara T., Westerman K. A., Inoue Y., Sakaguchi M., Noguchi H., Miyazaki M., Cai J., Tanaka N., Fox I. J., Leboulch P. Prevention of acute liver failure in rats with reversibly immortalized human hepatocytes. Science 2000a; 287: 1258–1262
- Kobayashi N., Nogushi H., Westerman K. A., Matsumura T., Watanabe T., Totsugawa T., Fujiwara T., Leboulch P., Tanaka N. Efficient Cre/loxP site‐specific recombination in a HepG2 human liver cell line. Cell Transplant 2000b; 9: 737–742
- Korbutt G. S., Ao Z., Flashner M., Rajotte R. V. Neonatal porcine islets as a possible source of tissue for humans and microencapsulation improves the metabolic response of islet graft posttransplantation. Ann. N. Y. Acad. Sci. 1997a; 831: 294–303
- Korbutt G. S., Rayat G. R., Ezekowitz J., Rajotte R. V. Cryopreservation of rat pancreatic islets: effect of ethylene glycol on islet function and cellular composition. Transplantation 1997b; 64: 1065–1070
- Korbutt G. S., Elliott J. F., Rajotte R. V. Cotransplantation of allogeneic islets with allogeneic testicular cell aggregates allows long‐term graft survival without systemic immunosuppression. Diabetes 1997c; 46: 317–322
- Lau H. T., Yu M., Fontana A., Stoeckert C. J., Jr. Prevention of islet allograft rejection with engineered myoblasts expressing FasL in mice. Science 1996; 273: 109–112
- Leblond F. A., Simard G., Henley N., Rocheleau B., Huet P. M., Haller J. P. Studies on smaller (approximately 315 microM) microcapsules: IV. Feasibility and safety of intrahepatic implantations of small alginate poly‐l‐lysine microcapsules. Cell Transplant 1999; 8: 327–337
- Lim F., Sun A. M. Microencapsulated islets as bioartificial endocrine pancreas. Science 1980; 210: 908–910
- NIH Implementation of the Recommendations of the Congressionally Directed Diabetes Research Working Group, http://www.niddk.nih.gov/federal/dwg/DRWGREP2.htm
- Oberholzer J., Triponez F., Mage R., Andereggen E., Buhler L., Cretin N., Fournier B., Goumaz C., Lou J., Philippe J., Morel P. Human islet transplantation: lessons from 13 autologous and 13 allogeneic transplantations. Transplantation 2000; 69: 1115–1123
- Paradis K., Longford G., Long Z. F., Heneine W., Sandstrom P., Switzer W. M., Chapman L. E., Lockey C., Onions D., Otto E. Search for cross‐species transmission of porcine endogenous retrovirus in patients treated with living pig tissue. Science 1999; 285: 1236–1241
- Patience C., Takeuchi Y., Weiss R. A. Infection of human cells by an endogenous retrovirus of pigs. Nat. Med. 1997; 3: 282–286
- Prokop A., Hunkeler D., Haralson M., Dimari S., Wang T. Water soluble polymers for immunoisolation II: evaluation of multicomponent encapsulation systems. Adv. Polym. Sci. 1998a; 136: 55
- Prokop A., Hunkeler D., Haralson M., Dimari S., Wang T. Water soluble polymers for immunoisolation I: complex coacervation and cytotoxicity. Adv. Polym. Sci. 1998b; 136: 1
- Rajotte R. V. Islet cryopreservation protocols. Ann. N. Y. Acad. Sci. 1999; 875: 200–207
- Ramiya V. K., Maraist M., Arfors K. E., Schatz D. A., Peck A. B., Cornelius J. G. Reversal of insulin‐dependent diabetes using islets generated in vitro from pancreatic stem cells. Nat. Med. 2000; 6: 278–282
- Rayat G. R., Rajotte R. V., Korbutt G. S. Potential application of neonatal porcine islets as treatment for type 1 diabetes: a review. Ann. N. Y. Acad. Sci. 1999; 875: 175–188
- Ronfard B., Rives J. M., Neveux Y., Carsin H., Barrandon Y. Long term regeneration of human epidermis on third degree burns transplanted with autologous cultured epithelium grown on a fibrin matrix. Transplantation 2000; 70: 1588–1598
- Ryan E. A., Lakey J. R., Shapiro A. M. Clinical outcomes and insulin secretion after islet transplantation with the Edmonton protocol. Diabetes 2001; 50: 710–719
- Salmon P., Obelholzer J., Occhiodoro T., Morel P., Lou J., Trono D. Reversible immortalization of human primary cells by lentivector‐mediated transfer of specific genes. Mol. Ther. 2000; 2: 404–414
- Sanberg P. R., Borlongan C. V., Saporta S., Cameron D. F. Testis‐derived sertoli cells survive and provide localized immunoprotection for xenografts in rat brain. Nat. Biotechnol. 1996; 14: 1692–1695
- Sanberg P. R., Borlongan C. V., Othberg A. I., Soporta S., Freeman T. B., Cameron D. F. Testis‐derived sertoli cells have a trophic effect on dopamine neurons and alleviate hemiparkinsonism in rats. Nat. Med. 1997; 3: 1129–1132
- Schrezenmeir J., Krichgessner J., Gero L., Kunz L. A., Beyer J., Muellerklieser W. Long‐term function of porcine islets and single cells embedded in barium–alginate matrix. Transplantation 1994; S7(9)1308–1314
- Shapiro A. M.J., Lakey J. R.T., Ryan E. A., Korbutt G. S., Toth E., Warnock G. L., Kneteman N. M., Rajotte R. V. Islet transplantation in seven patients with type 1 diabetes mellitus using a glucocorticoid‐free immunosuppressive regimen. N. Engl. J. Med. 2000; 343: 230–238
- Soon‐Shiong P. Treatment of type I diabetes using encapsulated islets. Adv. Drug Deliv. Rev. 1999; 35: 259–270
- Soon‐Shiong P., Feldman E., Nelson R., Komtebedde J., Smidsrod O., Skjak‐Break G., Espevik T., Heintz R., Lee M. Successful reversal of spontaneous diabetes in dogs by intraperitoneal microencapsulated islets. Transplantation 1992; 54: 769–774
- Soon‐Shiong P., Heintz R. E., Merideth N., Yao Q. X., Yao Z., Zheng T., Murphy M., Maloney M. K., Schmehl M., Harris M. Insulin independence in a type 1 diabetic patient after encapsulated islet transplantation. Lancet 1994; 343: 950–951
- Soria B., Roche E., Berna G., Leon‐Quinto T., Reig J. A., Martin F. Insulin‐secreting cells derived from embryonic stem cells normalize glycemia in streptozotocin‐induced diabetic mice. Diabetes 2000; 49: 157–162
- Suarez‐Pinzon W., Korbutt G. S., Power R., Hooton J., Rajotte R. V., Rabinovitch A. Testicular sertoli cells protect islet beta‐cells from autoimmune destruction in NOD mice by a transforming growth factor‐beta1‐dependent mechanism. Diabetes 2000; 49: 1810–1818
- Sun Y., Ma X., Zhou D., Vacek I., Sun A. M. Normalization of diabetes in spontaneously diabetic cynomologus monkeys by xenografts of microencapsulated porcine islets without immunosuppression. J. Clin. Invest. 1996; 98: 1417–1422
- Sundback C. A., Vacanti J. P. Alternatives to liver transplantation: from hepatocyte transplantation to tissue‐engineered organs. Gastroenterology 2000; 118: 438–442
- van der Laan L. J., Lockey C., Griffeth B. C., Frasier F. S., Wilson C. A., Onions D. E., Hering B. J., Long Z., Otto E., Torbett B. E., Salomon D. R. Infection by porcine endogenous retrovirus after islet xenotransplantation in SCID mice. Nature 2000; 407: 90–94
- Wang T., Lacik I., Brissova M., Anilkumar A. V., Prokop A., Hunkeler D., Green R., Shahrokhi K., Powers A. C. An encapsulation system for the immunoisolation of pancreatic islets. Nat. Biotechnol. 1997; 15: 358–362
- Wang T. G., Granett D., Akutagawa W. M., US Patent, 4, 645,442