Abstract
Isolated perfusion of the rat kidney causes hypoxic damage in the cells of the thick ascending limb of the loop of Henle. The cell damage is driven by active solute transport, which generates an imbalance of oxygen supply and demand. This injury is paradoxically prevented by adding the mitochondrial electron transport inhibitors rotenone or antimycin to the perfusion media. The present study shows that rotenone and antimycin decrease production of hydrogen peroxide in the thick ascending limb during perfusion. The findings support the hypothesis that the injury in this model is dependent on mitochondrial electron flow and suggest that mitochondrial electron flow, driven by the work of active solute transport in the presence of limited oxygen availability, may result in the generation of toxic oxygen metabolites.
INTRODUCTION
The isolated perfused rat kidney has been extensively studied as a model of hypoxic injury. Perfusion of the kidney in vitro with a cell free buffered salt solution consistently causes epithelial cell damage in the thick ascending limb of the loop of Henle (TAL).Citation[[1]], Citation[[2]] The isolated perfused kidney (IPK) model has received some attention for its potential implications for the pathophysiology of hypoperfusion mediated acute renal failureCitation[[3]] and also for its more general implications for understanding cellular defense strategies against hypoxia.Citation[[4]], Citation[[5]] The injury is known to be caused by the combination of a low oxygen tension in the renal medulla and the oxygen demand imposed by active ion transport in the TAL. Improving the oxygen delivery in the IPK by adding red blood cells or hemoglobin to the perfusate prevents the TAL damageCitation[[2]] as does decreasing solute transport work by inhibition of the sodium pump with ouabain.Citation[[6]], Citation[[7]]
One paradoxical finding in this model is that TAL damage is not worsened or reproduced by blockade of mitochondrial electron transport. In fact, electron transport blockade with agents such as rotenone (inhibitor at complex I of the electron transport chain) or antimycin (a blocker at complex III) actually prevents the hypoxic damage usually seen in this model.Citation[[8]] This protection from injury occurs despite the marked ATP depletion that results from exposure of kidneys to these metabolic toxins. Consideration of this remarkable feature of the hypoxic damage in the TAL led to the proposal that the injury may be dependent on mitochondrial electron transport activity itself during relative oxygen deprivation.Citation[[9]], Citation[[10]] The mechanism of such a “mitochondrial electron flow dependent” cell injury is not known. One hypothesis involves the possibility that potentially toxic oxygen metabolites might be generated by mitochondrial activity during relative oxygen deprivation. More specifically, mitochondrial electron flow, in the presence of inadequate terminal oxygenation of the electron transport chain, results in reduction of the electron transport intermediates. The reduced electron transporters might then interact with the limited oxygen available resulting in partial reduction of the oxygen to superoxide, hydrogen peroxide and hydroxyl radical.Citation[[11]], Citation[[12]]
The present study examines this hypothesis by determining the effects of rotenone and antimycin on hydrogen peroxide production in the TAL during isolated perfusion of the kidney.
DESIGN AND METHODS
Catalase is irreversibly inhibited by 3-amino-1,2,4-triazole (AMTZ) in the presence of hydrogen peroxide.Citation[[13]] Since hydrogen peroxide is required for catalase inactivation by AMTZ, exposure of cells to AMTZ provides a sensitive method for detecting cellular production of H2O2. In the present experiments, AMTZ was added to the perfusion medium in the isolated perfused kidney and catalase activity was measured in the inner stripe of the outer medulla after short perfusions under various experimental conditions. The inner stripe of the outer medulla is the zone of the kidney where the TAL tubules are concentrated and constitute the bulk of epithelial cell mass. Experimental groups for which outer medullary catalase activity was measured were as follows:
In vivo, untreated and flushed with KPO4 buffer for baseline catalase activity (n = 9)
Standard perfusion for 10 min without aminotriazole (n = 7)
Standard perfusion for 10 min with AMTZ (n = 8)
Rotenone perfusion for 10 min with AMTZ (n = 5)
Antimycin perfusion for 10 min with AMTZ (n = 3)
Standard perfusion for 20 min with AMTZ added at 10 min (n = 4)
Rotenone perfusion for 20 min with AMTZ added at 10 min (n = 3)
Perfusion of rat kidneys was as previously described.Citation[[14]] The recirculating perfusion medium was Krebs-Henseleit-albumin solution at pH 7.4 with 5 mM glucose as substrate and no amino acids added. Temperature was maintained at 37°C and perfusion pressure was 100 mm Hg at the cannula tip. The bovine serum albumin (BSA) was at 6.7 g%. Rotenone and antimycin were at 10−5M in the standard perfusate from the start of the experiments where they were used. Aminotriazole was added to the perfusate and perfusions were carried out for a total of 10 min after addition of the AMTZ in all cases. The dose of aminotriazole used was determined in a preliminary dose-response analysis where from 50 to 1000 mg AMTZ were added to exactly 125 cc of perfusion medium. It was found that 200 mg AMTZ (19 mM) was adequate for maximal catalase inactivation in the outer medulla of the isolated perfused kidney under standard conditions (data not shown). Following the 10 min of perfusion in the presence of AMTZ, the reaction was stopped and perfusate was flushed out of the kidney by introduction of 15 cc KPO4 buffer (5 mM, pH 7.8) into the arterial line via 3-way stopcock. The kidney was then removed from the perfusion circuit and the inner stripe of the outer medulla was dissected from the remaining tissue and snap frozen in liquid nitrogen. The frozen tissue was weighed and then homogenized in 50 mM KPO4 buffer (5 µL/mg tissue). The homogenate was centrifuged at 20,000 RPM for 20 min at 4°C and catalase activity was determined on the supernatant as described in detail by Brown et al.Citation[[15]] In brief, the change in absorbance per min at 240 nm was measured spectrophotometrically after addition of supernatant to a H2O2/KPO4 solution and the enzyme activity was determined from a standard curve.
In selected experiments, perfusions under standard conditions or with rotenone were for 60 min and aminotriazole was omitted. Following these perfusions the kidneys were fixed by perfusion with glutaraldehyde and prepared for examination by transmission electron microscopy as previously described.Citation[[16]] The ultrastructure shown represents typical TAL epithelial cell morphology in the various experimental groups. Quantitative aspects of the TAL lesions under standard perfusion conditions and with mitochondrial electron transport inhibitors have been described elsewhere.Citation[[8]]
RESULTS
Perfusion of the isolated rat kidney for 60 min under standard conditions results in extensive fragmentation of epithelial cells in the thick ascending limb of the loop of Henle and addition of rotenone to the perfusate prevents this damage (). Compared to the standard perfusion, the TAL in the rotenone perfused kidneys have an intact smooth luminal plasma membrane and do not exhibit nuclear pyknosis. There is some peripheral nuclear chromatin clumping in the rotenone perfused TAL and some of the mitochondria appear to be in a condensed state.
Figure 1. Electron micrographs of thick ascending limb of loop of Henle after perfusion fixation in vivo (A), following 60 min of standard perfusion (B) and after 60 min of perfusion with addition of rotenone to the standard perfusate (C). Standard perfusion causes hypoxic cell death in the tubules. Nuclei are pyknotic and cells are fragmented. These lesions are prevented by the addition of rotenone.
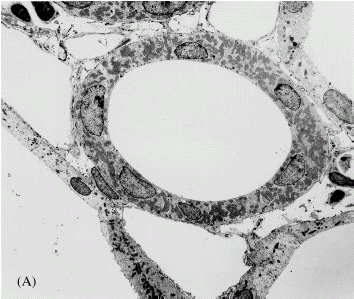
When aminotriazole is added to the standard perfusion media, 10 min of kidney perfusion results in a marked inhibition of catalase activity in the outer medulla. Addition of rotenone or antimycin to the perfusion media containing aminotriazole prevents the inactivation of catalase. The data in show this for perfusions lasting a total of 10 min where aminotriazole is present from the onset (groups 2–5). In a second series of experiments, aminotriazole was added after a 10 min equilibration period and the perfusions carried out for a total of 20 min (groups 6 and 7). Again, the addition of rotenone prevented catalase inactivation by AMTZ. In this second series of experiments catalase activity under standard conditions was 2.07 ± 0.36 U/mg tissue and with rotenone added catalase activity was 6.97 ± 1.56 U/mg tissue (p<0.02 by t-test).
Figure 2. Catalase activity in the outer medulla after 10 min of isolated perfusion of the kidney under various conditions is shown. The dotted lines represent the range covered by the mean ± SEM for catalase activity in the outer medulla in vivo in untreated rats and are provided for reference. Standard kidney perfusion without aminotriazole (AMTZ) causes no statistically significant decrease in medullary tissue catalase activity from the in vivo level. The presence of AMTZ in the medium during otherwise standard perfusion results in a decrease in tissue catalase activity indicating H2O2 production during the perfusion. Addition of either rotenone or antimycin prevents the loss of catalase activity caused by the presence of AMTZ indicating inhibition of H2O2 production. ANOVA indicates p<0.001 for differences among the groups. Standard perfusions with aminotriazole (**) show statistically significant difference from all other groups by Bonferroni's method of post-test analysis. None of the other groups differ significantly from each other or from the in vivo level.
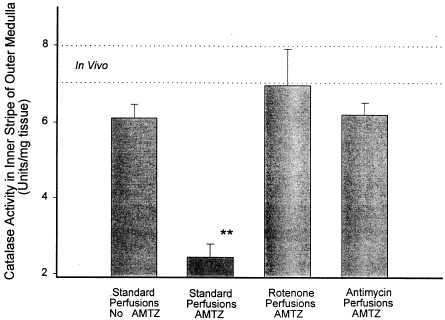
DISCUSSION
Mitochondrial electron transport blockade with rotenone or antimycin prevents hypoxic damage in the thick ascending limb of the loop of Henle in the isolated perfused kidney. This unexpected finding suggested that the injury in this system is not simply an effect of homeostasis failure from ATP depletion. The pace of injury generation in this model (with complete cell fragmentation within one hour) along with evidence that the damage was driven by cell workCitation[[6]], Citation[[7]] suggested a more active mechanism of injury and the concept of a mitochondrial electron flow dependent mechanism was proposed by Brezis.Citation[[9]], Citation[[10]] In the time since that proposal was made, more has been learned about the mechanism of this injury. Dissociation of the injury from the degree of ATP depletion in the TAL has been confirmed,Citation[[17]] calcium and pH dependence has been establishedCitation[[16]], Citation[[18]], Citation[[19]] and the possibility that activation of programmed cell death plays a role has been suggested.Citation[[20]] However, the possible role of mitochondrial activity in the mechanism of damage remains essentially unexplored.
One hypothesis possibly linking mitochondrial activity to cell injury during hypoxia involves the generation of partially reduced, toxic oxygen metabolites. Continued electron flow in the face of inadequate terminal oxygenation could result in relative reduction of electron chain intermediates. These reduced intermediates might then interact directly with available oxygen to produce potentially toxic oxygen metabolites such as superoxide, hydrogen peroxide and hydroxyl radical.Citation[[11]], Citation[[12]]
There is evidence that mitochondrial electron transport chain intermediates are relatively reduced in the thick ascending limb of Henle during isolated kidney perfusion. Epstein has shown incomplete oxidation of cytochrome oxidase in the medulla of the isolated perfused kidney by optical spectroscopy.Citation[[21]] The reduction of this cytochrome is attenuated by addition of furosemide to the perfusate, which suggests that ion transport in the TAL accounts for the partial reduction. Furthermore, mitochondrial electron transport blockade with rotenone or antimycin restores a high oxidation state to the cytochrome aa3. The latter finding indicates that adequate oxygen is available in this system for maintenance of fully oxidized cytochrome aa3 if electron flow is inhibited.
The present study examined the effects of inhibition of mitochondrial electron transport activity on the production of hydrogen peroxide in the inner stripe of the outer medulla in the isolated perfused kidney. The electron transport blockade was accomplished with rotenone, an inhibitor at complex I of the mitochondrial electron transport chain, or antimycin, an inhibitor in complex III. As is the case with isolated mitochondria, these agents cause a rapid decrease in oxygen utilization in the isolated perfused kidney and ATP depletion can be demonstrated in cortex and medulla.Citation[[8]]
Hydrogen peroxide production was measured as a representative of the potentially toxic oxygen metabolites. A very sensitive assay developed for measuring H2O2 production in bacteriaCitation[[13]] was adapted for kidney tissue measurement. The method is based on the inactivation of catalase by aminotriazole in the presence of small amounts of hydrogen peroxide. Thus a decrease in tissue catalase activity in the presence of added aminotriazole indicates production of H2O2 in the tissue.
The kidney was dissected after perfusions so that only the inner stripe of the outer medulla was studied since this is the region where the thick ascending limb of the loop of Henle is concentrated and where the TAL make up most of the parenchymal mass. The outer stripe tissue level of catalase activity after a perfusion in the presence of AMTZ was assumed, therefore, to reasonably estimate thick ascending limb of Henle H2O2 production.
The study showed that there was hydrogen peroxide production in the thick ascending limb of Henle during standard isolated perfusion of the kidney and that the H2O2 production was eliminated by mitochondrial electron transport blockade with addition of either rotenone or antimycin. Thus, the findings are consistent with the possibility that toxic oxygen products play a role in the cell fragmentation injury and may explain the paradoxical effects of these metabolic poisons in this model.
The effectiveness of antimycin in preventing hydrogen peroxide production in the TAL may be surprising since many studies have concluded that addition of antimycin is, in fact, an effective means of generating oxygen free radicals in isolated mitochondria.Citation[[11]] The protective effect of antimycin in this model has been consistent and impressive, however, and the discrepancy with other systems is unexplained. The findings here suggest that maintenance of the oxygenation of the terminal portion of the electron transport chain is critical to preventing partial reduction of oxygen at least in the IPK model.
The mechanism of hypoxic damage in the thick ascending limb of Henle in the isolated perfused kidney model appears to involve calcium, solute transport activity and mitochondrial electron flow in the face of inadequate terminal oxygenation. It is rapid in onset, is preventable by acidosis and perhaps involves a cell death program. Recent information concerning the mitochondrial permeability transition and its potential importance as a trigger for either necrosis or apoptosisCitation[[22]], Citation[[23]] may provide a framework for future work on this model. The known synergism between calcium mechanisms and free radical production in producing the permeability transitionCitation[[22]] makes the hypothesis attractive. One might suggest that relative hypoxia disrupts calcium homeostasis and, at the same time, sets up conditions for mitochondrial electron flow to cause reduction of electron transport chain intermediates. Cell work, such as tubular solute transport, might drive the mitochondrial electron flow. Reduced cytochromes could interact directly with the limited oxygen available and produce toxic oxygen products. These, together with abnormal calcium availability, trigger a mitochondrial permeability transition and rapid cell destruction.
ACKNOWLEDGMENT
This work was supported by Grant DK38516-03 from the National Institutes of Health. We thank Alan S. Jones for expert assistance with the electron microscopy.
REFERENCES
- Alcorn D., Emslie K.R., Ross B.D., Ryan G.B., Tange J.D. Selective Distal Nephron Damage During Isolated Kidney Perfusion. Kidney Int. 1981; 19: 638–647
- Brezis M., Rosen S., Silva P., Epstein F.H. Selective Vulnerability of the Medullary Thick Ascending Limb to Anoxia in the Isolated Perfused Rat Kidney. J. Clin. Invest. 1984; 73: 182–190
- Brezis M., Rosen S. Hypoxia of the Renal Medulla—Its Implications for Disease. N. Engl. J. Med. 1995; 332: 647–655
- Hochachka P.W. Defense Strategies Against Hypoxia and Hypothermia. Science 1986; 231: 234–241
- Hochachka P.W., Guppy M. Metabolic Arrest and the Control of Biological Time. Harvard University Press, Cambridge, MA
- Brezis M., Rosen S., Silva P., Epstein F.H. Transport Activity Modifies Thick Ascending Limb Damage in the Isolated Perfused Kidney. Kidney Int. 1984; 25: 65–72
- Brezis M., Rosen S., Spokes K., Silva P., Epstein F.H. Transport-Dependent Anoxic Cell Injury in the Isolated Perfused Rat Kidney. Am. J. Pathol. 1984; 116: 327–341
- Brezis M., Shanley P., Silva P., Spokes K., Lear S., Epstein F.H., Rosen S. Disparate Mechanisms for Hypoxic Cell Injury in Different Nephron Segments. Studies in the Isolated Perfused Rat Kidney. J. Clin. Invest. 1985; 76: 1796–1806
- Brezis M., Rosen S., Shanley P., Spokes K., Silva P., Epstein F.H. Electron Flow Dependent Cell Damage: An Unrecognized Element of Anoxic Injury?. (Abstract) Clin. Res. 1984; 32: 442A
- Brezis M., Rosen S., Silva P., Spokes K., Epstein F.H. Mitochondrial Activity: A Possible Determinant of Anoxic Injury in Renal Medulla. Experientia 1986; 42: 570–572
- McCord J.M., Turrens J.F. Mitochondrial Injury by Ischemia and Reperfusion. Current Topics in Bioenergetics 1994; 17: 173–195
- Dawson T.L., Gores G.J., Nieminen A.L., Herman B., Lemasters J.J. Mitochondria as a Source of Reactive Oxygen Species During Reductive Stress in Rat Hepatocytes. American Journal of Physiology 1993; 264(4 Pt 1)C961–C967
- Cohen G., Somerson N.L. Catalase-Aminotriazole Method for Measuring Secretion of Hydrogen Peroxide by Microorganisms. Journal of Bacteriology 1969; 98(2)543–546
- Ross B.D., Epstein F.H., Leaf A. Sodium Reabsorption in the Perfused Rat Kidney. Am. J. Physiol. 1973; 225: 165–171
- Brown J.M., Terada L.S., Grosso M.A., Whitman G.J., Velasco S.E., Patt A., Harken A.H., Repine J.E. Xanthine Oxidase Produces Hydrogen Peroxide which Contributes to Reperfusion Injury of Ischemic Isolated Perfused Hearts. J. Clin. Invest. 1988; 81: 1297–1301
- Shanley P.F., Shapiro J.I., Chan L., Burke T.J., Johnson G.C. Acidosis and Hypoxic Medullary Injury in the Isolated Perfused Kidney. Kidney Int. 1988; 34: 791–796
- Shanley P.F., Johnson G.C. Adenine Nucleotides, Transport Activity and Hypoxic Necrosis in the Thick Ascending Limb of Henle. Kidney Int. 1989; 36: 823–830
- Brezis M., Shina A., Kidroni G., Epstein F.H., Rosen S. Calcium and Hypoxic Injury in the Renal Medulla of the Perfused Rat Kidney. Kidney Int. 1988; 34: 186–194
- Shanley P.F., Johnson G.C. Calcium and Acidosis in Renal Hypoxia. Lab. Invest. 1991; 65: 298–305
- Beeri R., Symon Z., Brezis M., Ben-Sasson S.A., Baehr P.H., Rosen S., Zager R.A. Rapid DNA Fragmentation from Hypoxia Along the Thick Ascending Limb of Rat Kidneys. Kidney Int. 1995; 47(6)1806–1810
- Epstein F.H., Balaban R.S., Ross B.D. Redox State of Cytochrome aa3 in Isolated Perfused Rat Kidney. Am. J. Physiol. 1982; 243: F356–F363
- Lemasters J.J., Nieminen A.L., Qian T., Trost L.C., Elmore S.P., Nishimura Y., Crowe R.A., Cascio W.E., Bradham C.A., Brenner D.A., Herman B. The Mitochondrial Permeability Transition in Cell Death: A Common Mechanism in Necrosis, Apoptosis and Autophagy. Biochimica et Biophysica Acta 1998; 1366(1–2)177–196
- Saikumar P., Dong Z., Weinberg J.M., Venkatachalam M.A. Mechanisms of Cell Death in Hypoxia/Reoxygenation Injury. Oncogene 1998; 17(25)3341–3349