Abstract
Accompanied with the worsening of the pulmonary tuberculosis bacterium (TB) epidemic, the incidence of spinal TB has increased in recent years. Spinal reconstruction and stabilisation, and bone defect repair play a crucial role in the surgical treatment of spinal TB. Unfortunately, the existing materials have not completely met the requirements for spinal TB reconstruction due to their diverse deficiencies. Therefore, there is an urgent need to develop novel reconstructing implants. Poly-DL-lactide (PDLLA) and nano-hydroxyapatite (nHA) are two promising drug delivery systems (DDS) and materials for bone repair, which could help us to overcome the difficulties in spinal TB reconstruction in the future. In this article, we discuss the properties of PDLLA and nHA, two potential drug delivering and bone repair materials for spinal TB reconstruction. We also presented two alternatives for spinal TB in future. Two strategies have the potential for treating spinal TB in the future. One such strategy consists of mixing anti-TB drugs, PDLLA with nHA to fabricate a novel three-dimensional (3D) porous scaffold via 3D printing (3DP) technology. Another is preparing a novel titanium mesh implant coated with drugs/PDLLA/nHA composites by solvent evaporation and low-temperature drying technology. These two hypotheses have recently been tested in a laboratory setting by our team.
Keywords::
1. Introduction
Over the past 20 years, approximately 2 billion people have contracted tuberculosis bacterium (TB) infection and it is estimated that 300 million people will be newly affected in the next decade. Approximately 8.6 million people fell ill with TB in 2012, including 1.1 million cases among people living with HIV Citation[1]. Owing to the increase in prevalence TB, the incidence of global spinal TB and drug-resistant spinal TB has also increased in recent years. It is the highest cause of extra-pulmonary TB, accounting for approximately 3 – 5% of the total incidence of TB Citation[2]. Accompanied by the development of surgical techniques and advanced biomaterials, the curative ratio of spinal TB has been significantly improved. However, the postoperative non-union and recurrence rate were still high at 13 – 26% accounting for faulty operative time, resistant strains emergence, non-rational reconstruction materials, poor compliance and irregular chemotherapy Citation[3,4]. It is very hard for the anti-TB drugs to reach the lesion in bone tissue via oral administration. In contrast, liver and kidney dysfunctions were very common in patients who had a history of long-term anti-TB drug use Citation[5].
Autogenous bone grafts are considered to be the gold standard for bone reconstruction and regeneration Citation[6]. However, it is hard to obtain sufficient autogenous bone tissue in practice. Pain and infection related to the donor site are very common. In addition, a small amount of autogenous bone is easily absorbed by an organism. In addition, the reject reaction triggered by the immunogenicity of allogeneic bone would cause no fusion when using allogeneic bone for reconstruction during surgical operation. In recent years, novel biomaterial for spinal TB treatment has been a hot research area for clinicians and biomaterials scientists in the field of hard tissue repair. In 12 patients with TB spondylitis, it has been shown that titanium cage surgery can demonstrate good clinical efficacy for spinal reconstruction and stabilisation Citation[7]. Another study demonstrated that a patient with thoracic vertebral tuberculosis recovered satisfactorily with no complications when treated using an antibiotic crescent-shaped polymethylmethacrylate (PMMA) strut for spinal reconstruction and fusion Citation[8]. However, titanium mesh and PMMA are not ideal biomaterials to replace autogenous bone. Although titanium mesh could supply good mechanical support strength, it lacks both osteoconductive and osteoinductive properties Citation[9]. The intersurface of titanium and vertebral body were mechanically combined but not osseointegrated. Owing to this, subsidence or loosening often occur with the titanium mesh. This in turn causes the corrected kyphosis angle to be lost when reconstructing with the titanium cage Citation[10]. PMMA released lots of heat during the cure time, enabling damage to surrounding tissues. The PMMA monomer cytotoxicity also inhibited local macrophage's phagocytic and bactericidal effect Citation[11]. PMMA also triggered adverse reactions, such as a transient decrease/elevation of blood pressure, and even sudden cardiac arrest. Improper handling in operation would cause iatrogenic paraplegia as a result of spinal cord or nerve compression. Therefore, there is an urgent need to develop a novel reconstructing biomaterial for spinal TB to solve the problems stated above.
Poly-DL-lactide (PDLLA) and nano-hydroxyapatite (nHA) are considered to be two promising orthopaedic reconstruction materials because of their favourable biodegradable abilities, nontoxic side effects and non-immunogenicity Citation[12,13]. Meanwhile they are also commonly used as drug delivery system (DDS) owing to their beneficial drug release characteristics Citation[14,15]. With the development of research and exploration on these two materials, they hold the potential to be utilised to solve the existing dilemma in spinal TB in the future.
2. Current evidence
2.1 PDLLA
2.1.1 Application in bone repair
PDLLA presents in an amorphous state with low intensity and degrades rapidly. The average degradation time is approximately 12 – 18 months. The mechanical strength of PDLLA could last for 3 – 6 months in vivo, which is beneficial for maintaining the early fixation and non-weight-bearing area for bone repair Citation[16]. Histological results have shown that the surface modified three-dimensional (3D) chitosan/PDLLA scaffolds possess significantly higher bone formation potential and better biocompatibility than pure porous PDLLA scaffold in a rabbit radialis defect model in vivo Citation[17]. After being implanted in vivo, the osteocalcin production in the modified PDLLA/chitosan scaffold group was significantly higher (p < 0.05) than in the control group. In order to improve the bone repair performance, PDLLA was also added in order to adjust the mechanical properties for other bone repair scaffolds. In the present study, the mechanical properties of two scaffolds with porosities of 77 and 81% β-tri-calcium phosphate (HA/β-TCP) were significantly reinforced by adding 12 wt% PDLLA Citation[18]. The 77% HA/β-TCP/PDLLA showed similar mechanical properties to human cancellous bone and was significantly stronger than the HA/β-TCP without PDLLA. The scaffold successfully healed the cylindrical critical size defects at distal femoral condyles bilaterally in sheep.
PDLLA has demonstrated many good characteristics for bone repair; there are, however, still many deficiencies that should be improved, such as poor cell adhesion ability caused by the insufficient hydrophilic capacity, negative effect on cell growth caused by the acidic degradation products, maintenance of only short-term mechanical strength and the inconformity of degradation speed with bone repair Citation[19].
2.1.2 Application on DDS in orthopaedics
Intramedullary tibial nails preloaded with PDLLA/gentamicin have already obtained the qualification of manufacture after being Communauté Européenne (CE) certified in Europe and Canada in 2006 Citation[20]. A previous study with rats testified that the PDLLA/gentamicin coating was a promising approach for prophylaxis of implant-associated infection Citation[21]. The drug releasing experiment in vitro and in vivo in female Sprague–Dawley rats showed the titanium implants coated with PDLLA/gentamicin released rapidly with an initial burst, and then entered into a slow release stage. Slower release seems to be dependent on diffusion and degradation of the PDLLA coating. The bacterial adhesion experiment showed the coat successfully prevented bacterial adhesion to the antimicrobial modified composites. Meanwhile, neither the polymer PDLLA nor the gentamicin released from the coating had a negative effect on osteoblasts in a cellular experiment. PDLLA improved the release performance when used in combination with other DDS. PDLLA/clindamycin-containing calcium phosphate powders and clindamycin/calcium phosphate nanoparticulate powders both demonstrated a good antibacterial performance against Staphylococcus aureus in vitro. However, the former exhibited longer sustained drug release than the latter accompanied with the degradation of the carriers Citation[22]. Based on the evidence of an in vitro experiment, no cytotoxic effects have been detected within the two antibiotic-containing powders. They also increased the expression of osteogenic markers, such as osteocalcin, osteopontin, Runx2 and protocollagen type I, without any affection by the release of clindamycin.
2.2 nHA
2.2.1 Applied in bone repair
The artificial nHA has been considered to be the most promising artificial tooth and bone substitute material because of its excellent biocompatibility, good bioactivity, good torsional modulus, good tensile modulus and tensile strength, and it is fatigue resistant Citation[19]. In virtue of its small size, nHA possesses high solubility, large specific surface area (SSA) and surface energy. It can chemically bond with natural bone to enhance bone healing. However, nHA cannot be individually used as an ideal bone substitute owing to its insufficient mechanical strength and impact toughness. Many nHA composites have been successfully fabricated for tissue engineering scaffolds, such as nHA/collagen/poly (vinyl alcohol) composites Citation[23], nHA/polycaprolactone composites Citation[24], and nHA/collagen composites Citation[25]. The layer structure of chitosan/nHA composites prepared via in situ hybridisation had principally met the bone substitute materials needs for mechanical properties Citation[26]. The flexural strength is up to 86 MPa, which is nearly 3 – 4 times higher than cancellous bone and is equivalent to half of compact bone. The scaffold composed of biodegradable poly diphenyl alanine ethyl ester phosphazene and 100 nm nHA has also shown an excellent bone repair ability Citation[27]. The range of hole size is 86 – 145 nm, which is suitable for adsorption and proliferation for osteoblast cells. The compression modulus range is 46 – 81 MPa, which was similar to natural bone.
2.2.2 Applied as DDS in orthopaedics
Diffusion is the principal way for HA to exert its drug releasing effect Citation[28]. When the materials loaded with drugs were implanted in vivo, the body fluids infiltrated into the porous scaffold to dissolve drugs. The drug then diffused from areas of high concentration to the lower concentration environment because of drug concentration differences. The release speed was determined by pore size, porosity and additive components. The external microwave or ultrasound could also have been used to adjust the delivery ratio and release speed in the experiment Citation[29].
Radioactive elements are easy to insert into nHA during the manufacturing process, which enables targeting in order to inactivate tumour cells Citation[30]. It was also testified that nHA could deliver macromolecular drugs in large quantities, due to excellent medicine absorbability by bio-adhesion or bio-binding Citation[31]. nHA was an excellent carrier for vancomycin to treat chronic osteomyelitis and bone defects caused by MRSA strains Citation[32]. The concentration of vancomycin could remain effectively in osseous and soft tissues up to 12 weeks. In the in vivo experiment using rabbits, all rabbits in the experimental group recovered and the bone defects were partially repaired within 3 months, whereas the infection and bone defects persisted in the control and blank groups. The research of nHA in DDS is just merely at exploratory stage. The delivery mechanism, control of release profile and the targeting of delivery still need to be defined and require further attention and research in the future.
2.3 PDLLA/nHA composites
2.3.1 Applied in bone repair
On account of their own strengths and weaknesses in bone reconstruction, many research groups have made great efforts on PDLLA/nHA composites to enhance bone repair ability. The addition of nHA could neutralise the acid environment caused by the acidic degradation products from PDLLA, which will be propitious to cell growth and proliferation. It has been demonstrated that the osteoconductive and osteoinductive properties of porous HA/PDLLA scaffold were significantly superior to porous PDLLA scaffold Citation[33]. In the present study, the PDLLA/HA composite- and PDLLA-coated implants (50/50 vol%) were implanted bilaterally in the proximal part of humeri with a 2-mm peri-implant gap in 10 sheep Citation[34]. After 12 weeks, the remains of the coatings were present on 20.3 and 19.8% of the two implants, respectively. The newly formed bone (39.3%) and fibrous tissue (58.3%) had replaced the PDLLA/HA coat, whereas pure PDLLA coat was replaced almost completely by fibrous tissue (96.2%). The former implant fixed much more firmly than the latter. The results revealed that the addition of nHA/PDLLA coating on titanium implants significantly improved osseointegration. Recently, the porous 3D PDLLA/nHA scaffolds have been successfully fabricated by stereolithography Citation[35]. The results showed these scaffolds possess an excellent 3D structure for reproduction and good mechanical properties. It was confirmed there was no cytotoxicity with human marrow mesenchymal cells (hMSC). The addition of nHA significantly increased the mechanical properties and osteoconductive potential.
2.3.2 Applied as DDS in orthopaedics
Owing to their different and excellent drug releasing properties, PDLLA and nHA are often used together as drug transport vehicles for improving drug release performance experimentally. PDLLA/HA/vancomycin composites have shown excellent and sustained antibacterial effects on MRSA for treating osteomyelitis Citation[36]. The PDLLA scaffold with a 28-µm HA fibre coating had steadily released only 23% after 28 days. The drug release experiment on dexamethasone/PDLLA/nHA composites showed that dexamethasone release profiles includes an initial burst stage (in the first 10 h) and a slow release stage (in the following 790 h) Citation[37]. The presence of the initial burst stage is on account of the free drug located near to the surface of the drug-coated layer. The releasing rate of the scaffolds was highly correlated with the concentration of the nHA during the first 30 h. It was considered that the advantage of the drug loading capacity of the scaffolds can be attributed to hydrophilic nHA incorporation. Besides of the amount of the nHA, the pore size and porosity level of the scaffolds could also effectively control the drug release behaviour of the PDLLA/nHA scaffold Citation[38].
3. Expert opinion
Based on the understanding of the current situation of spinal TB, current application progress and material characteristics on PDLLA and nHA in orthopaedics, we present two novel treatment strategies for spinal TB (). The first one is combining anti-tuberculosis drug with PDLLA and nHA using an appropriate technique to generate a novel 3D and porous scaffold biomaterial for reconstructing. The scaffold should possess certain mechanical properties, good osteoconductive and osteoinductive properties, and especially good anti-TB delivering performance. It could be used individually or in combination with titanium mesh to reconstruct spinal stabilisation and repair bone defect in surgery. The second strategy is coating the anti-TB drugs/PDLLA/nHA composites on the surface of titanium mesh to create a novel bioactive implant, which could be used individually or in combination with a few autogenous bone grafts in operation.
Figure 1. Two novel reconstruction strategies for spinal tuberculosis bacterium (TB) in surgery. One is combining anti-TB drugs, poly-DL-lactide (PDLLA) and nano-hydroxyapatite (nHA) to fabricate a novel three-dimensional porous scaffold via three-dimensional printing (3DP) technology; and the other is preparing a novel titanium mesh coated with drugs/PDLLA/nHA composites by solvent evaporation and low-temperature drying technology.
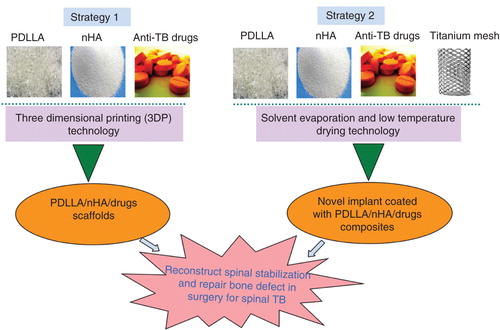
The newly developed three dimensional printing (3DP) technology is one of the most exciting technologies in area of rapid prototyping, 3DP was a safe and flexible approach for fabricating tissue engineering scaffolds. It could pre-design ideal pore size, porosity and 3D structure based on the needs of cell growth and mechanical property requirements. It had been widely used in the research on tissue engineering scaffold formation and implantable DDS preparation Citation[39,40]. The scaffold with villi features made via indirect 3DP was testified to be suitable for intestinal epithelial cells 6 (IEC6) attached uniformly in vitro Citation[41]. Scanning electron microscope (SEM) showed the scaffold presented to be highly open, well interconnected and show uniform pore architecture (100 – 150 μm). An anatomically shaped zygoma scaffold with 300 – 500 μm interconnected pores were also successfully produced and characterised. An implantable DDS for levofloxacin manufactured via 3DP could maintain a slow steady release for 80 days after an initial short-term burst release Citation[42]. This outstanding technology could be an excellent manufacture method for the first strategy. The novel porous titanium implants coated with PDLLA/nHA composites had been successfully fabricated by means of solvent evaporation and low-temperature drying technology and was successfully applied for bone repair in a sheep model Citation[34]. However, the multiple PDLLA/nHA/medicines coats with titanium alloy should be further investigated so as to work with the drug delivery and releasing profile. Maybe we could make use of this way to accomplish the second strategy. The two programs are currently being investigated in our laboratory.
We believe that the two novel strategies would open a new era for spinal TB reconstruction if the hypotheses proved to be practical. The composites composed of PDLLA and nHA would give full play of two materials' advantages and make up for the shortcomings of each other. The mechanical properties, bone repair ability, degradation characteristic, anti-TB activity of the composites are interrelated and interact on each other (). PDLLA would facilitate the shaping and supply a certain mechanical strength for nHA. The addition of PDLLA would remedy a defect of nHA in mechanical strength and improve the drug releasing capability. nHA would neutralise the acidic environment caused by the degradation products of PDLLA to promote new cell growth. Compared with conventional reconstruction, these two techniques would in theory significantly enhance the cell growth for defect repair and promote the interface osseointegration between titanium mesh and bone surface. The subsidence and loosening rate would reduce significantly. The post-operative fusion rate would be significantly improved. More importantly, the quantity demanded of autologous bone in practice would greatly reduce in the future. Meanwhile, if we could adjust the content of nHA, porous structure and porosity to an ideal range in order to achieve their full potential in adhesive attraction and wraparound effect with anti-TB drugs, the composites would maintain an excellent slow drug releasing performance for post-operative anti-TB treatment.
Declaration of interest
This project was financially supported by the 973 project of China (No. 2012CB933601) and National “Twelfth Five-Year” science and technology support program (2012BAI17B02).
Bibliography
- WHO. World Health Organization, Global tuberculosis report 2013. World Health Organization; Geneva, Switzerland; 2013
- Garg RK, Somvanshi DS. Spinal tuberculosis: a review. J Spinal Cord Med 2011;34(5):440-54
- Jain AK, Dhammi IK. Tuberculosis of the spine: a review. Clin Orthop Relat Res 2007;460:39-49
- Suárez-García I, Noguerado A. Drug treatment of multidrug-resistant osteoarticular tuberculosis: a systematic literature review. Int J Infect Dis 2012;16(11):e774-8
- Wang Z, Ge Z, Jin W, et al. Treatment of spinal tuberculosis with ultrashort-course chemotherapy in conjunction with partial excision of pathologic vertebrae. Spine J 2007;7(6):671-81
- Weisz RD, Errico TJ. Spinal infections. Diagnosis and treatment. Bull Hosp Jt Dis 2000;59(1):40-6
- Christodoulou AG, Givissis P, Karataglis D, et al. Treatment of tuberculous spondylitis with anterior stabilization and titanium cage. Clin Orthop Relat Res 2006;444:60-5
- Jun W, Yi-Jun K, Fei C, et al. Application of an antibiotic crescent-shaped polymethylmethacrylate strut in thoracic vertebral tuberculosis. Turk Neurosurg 2013;23(1):107-12
- Korovessis P, Petsinis G, Koureas G, et al. Anterior surgery with insertion of titanium mesh cage and posterior instrumented fusion performed sequentially on the same day under one anesthesia for septic spondylitis of thoracolumbar spine: is the use of titanium mesh cages safe? Spine (Phila Pa 1976) 2006;31(9):1014-19
- Kanayama M, Cunningham BW, Haggerty CJ, et al. In vitro biomechanical investigation of the stability and stress-shielding effect of lumbar interbody fusion devices. J Neurosurg 2000;93(2 Suppl):259-65
- Bettencourt A, Almeida AJ. Poly(methyl methacrylate) particulate carriers in drug delivery. J Microencapsul 2012;29(4):353-67
- Tang J, Hu JF, Guo WC, et al. Research and application of absorbable screw in orthopedics: a clinical review comparing PDLLA screw with metal screw in patients with simple medial malleolus fracture. Chin J Traumatol 2013;16(1):27-30
- Nazhat SN, Kellomäki M, Törmälä P, et al. Dynamic mechaniacal characterization of biodegradable composites of hydroxyapatite and polylactides. J Biomed Mater Res 2001;58(4):335-43
- Bahna P, Dvorak T, Hanna H, et al. Orthopaedic metal devices coated with a novel antisepticdye for the prevention of bacterial infections. Int J Antimicrob Agents 2007;29:593-6
- Norowski PA Jr, Bumgardner JD. Biomaterial and antibiotic strategies for peri-implantitis: a review. J Biomed Mater Res B Appl Biomater 2009;88(2):530-43
- Agarwal S, Gupta A, Grevious M, et al. Use of resorbable implants for mandibular fixation: a systematic review. J Craniofac Surg 2009;20(2):331-9
- Cai K, Yao K, Yang Z, et al. Histological study of surface modified three dimensional poly (D, L-lactic acid) scaffolds with chitosan in vivo. J Mater Sci Mater Med 2007;18(10):2017-24
- Ding M, Røjskjaer J, Cheng L, et al. The effects of a novel-reinforced bone substitute and Colloss®E on bone defect healing in sheep. J Biomed Mater Res B Appl Biomater 2012;100(7):1826-35
- Navarro M, Michiardi A, Castaño O, et al. Biomaterials in orthopaedics. J R Soc Interface 2008;5(27):1137-58
- Schmidmaier G, Lucke M, Wildemann B, et al. Prophylaxis and treatment of implant-related infections by antibiotic-coated implants: a review. Injury 2006;37(2):105-12
- Vester H, Wildemann B, Schmidmaier G, et al. Gentamycin delivered from a PDLLA coating of metallic implants: in vivo and in vitro characterisation for local prophylaxis of implant-related osteomyelitis. Injury 2010;41(10):1053-9
- Uskoković V, Hoover C, Vukomanović M, et al. Osteogenic and antimicrobial nanoparticulate calcium phosphate and poly-(D,L-lactide-co-glycolide) powders for the treatment of osteomyelitis. Mater Sci Eng C Mater Biol Appl 2013;33(6):3362-73
- Degirmenbasi N, Kalyon DM, Birinci E. Biocomposites of nanohydroxyapatite with collagen and poly(vinyl alcohol). Colloids Surf B Biointerfaces 2006;48(1):42-9
- Thomas V, Jagani S, Johnson K, et al. Electrospun bioactive nanocomposite scaffolds of polycaprolactone and nanohydroxyapatite for bone tissue engineering. J Nanosci Nanotechnol 2006;6(2):487-93
- Yunoki S, Marukawa E, Ikoma T, et al. Effect of collagen fibril formation on bioresorbability of hydroxyapatite/collagen composites. J Mater Sci Mater Med 2007;18(11):2179-83
- Hu Q, Li B, Wang M, et al. Preparation and characterization of biodegradable chitosan/hydroxyapatite nanocomposite rods via in situ hybridization: a potential material as internal fixation of bone fracture. Biomaterials 2004;25(5):779-85
- Nukavarapu SP, Kumbar SG, Brown JL, et al. Polyphosphazene/nano-hydroxyapatite composite microsphere scaffolds for bone tissue engineering. Biomacromolecules 2008;9(7):1818-25
- Bose S, Tarafder S. Calcium phosphate ceramic systems in growth factor and drug delivery for bone tissue engineering: a review. Acta Biomater 2012;8(4):1401-21
- Hassan MA, Ahmed IS, Campbell P, et al. Enhanced gene transfection using calcium phosphate co-precipitates and low-intensity pulsed ultrasound. Eur J Pharm Sci 2012;47(4):768-73
- Kolhe S, Parikh K. Application of nanotechnology in cancer: a review. Int J Bioinform Res Appl 2012;8(1-2):112-25
- Fox K, Tran PA, Tran N. Recent advances in research applications of nanophase hydroxyapatite. Chemphyschem 2012;13(10):2495-506
- Jiang JL, Li YF, Fang TL, et al. Vancomycin-loaded nano-hydroxyapatite pellets to treat MRSA-induced chronic osteomyelitis with bone defect in rabbits. Inflamm Res 2012;61(3):207-15
- Hasegawa S, Neo M, Tamura J, et al. In vivo evaluation of a porous hydroxyapatite/poly-DL-lactide composite for bone tissue engineering. J Biomed Mater Res A 2007;81(4):930-8
- Jensen T, Jakobsen T, Baas J, et al. Hydroxyapatite nanoparticles in poly-D,L-lactic acid coatings on porous titanium implants conducts bone formation. J Biomed Mater Res A 2010;95(3):665-72
- Ronca A, Ambrosio L, Grijpma DW. Design of porous three-dimensional PDLLA/nano-hap composite scaffolds using stereolithography. J Appl Biomater Funct Mater 2012;10(3):249-58
- Ravelingien M, Mullens S, Luyten J, et al. Vancomycin release from poly(D,L-lactic acid) spray-coated hydroxyapatite fibers. Eur J Pharm Biopharm 2010;76(3):366-70
- Chen L, Tang CY, Tsui CP, et al. Mechanical properties and in vitro evaluation of bioactivity and degradation of dexamethasone releasing poly-d-l-lactide/nano-hydroxyapatite composite scaffolds. J Mech Behav Biomed Mater 2013;22:41-50
- Chen L, Tang CY, Chen DZ, et al. Fabrication and characterization of poly-D-L-lactide/nano-hydroxyapatite composite scaffolds with poly (ethylene glycol) coating and dexamethasone releasing. Compos Sci Technol 2011;71(16):1842-9
- Lee JY, Choi B, Wu B, et al. Customized biomimetic scaffolds created by indirect three-dimensional printing for tissue engineering. Biofabrication 2013;5(4):045003
- Lee YB, Polio S, Lee W, et al. Bio-printing of collagen and VEGF-releasing fibrin gel scaffolds for neural stem cell culture. Exp Neurol 2010;223(2):645-52
- Lee M, Dunn JC, Wu BM. Scaffold fabrication by indirect three-dimensional printing. Biomaterials 2005;26(20):4281-9
- Huang W, Zheng Q, Sun W, et al. Levofloxacin implants with predefined microstructure fabricated by three-dimensional printing technique. Int J Pharm 2007;339(1-2):33-8