Abstract
Pulmonary drug delivery requires particles with a fine and uniform size distribution, 1 – 5 µ hydrodynamic diameter, for targeted delivery and higher uptake within the pulmonary tract. The antisolvent vapor precipitation method is a new alternative method to generate particles with such characteristics. Development so far has shown that the technique is applicable to disaccharides (lactose), magnesium sulfate, hydrophobic and protein particles. The method may also be extended to generate fine encapsulation particles and to produce disaccharides (lactose) and protein particles blend in a single step.
1. Introduction
This editorial describes developments of a new method to make ultrafine and uniform particles for pulmonary drug delivery. Particle size and uniformity are very important characteristics in the delivery of drugs via the pulmonary route Citation[1-3]. Uniform particle size ensures that the aerodynamically transported drug particles are more targeted. This is because the behavior of the particles in following or traversing the inhalation airflow (resulting in deposition) is highly affected by their size and density, which is described by the aerodynamic diameter. Therefore, particle size uniformity will ensure that particles in a blend experiences similar air flow interaction resulting in similar trajectory within the airways. In addition to the need for uniform particle sizes, smaller particles will also enhance the dissolution or absorption rate of the pulmonary delivered drugs Citation[4]. This serves to overcome the mucous layer in the lungs so that the drug materials are absorbed into the bloodstream before being expelled. This aspect has been the subject of many studies, particularly in the delivery of protein or poorly water-soluble drugs via the pulmonary tract. In many cases, reducing the size of the drug particle might be an important option in increasing the uptake of these drug particles.
One common method to generate drug particles for pulmonary delivery, or as a general particle production process in the pharmaceutical industry, is the spray drying technology Citation[5]. Although this process has been the work horse in particle production for the industry, one challenge is in producing uniform particles in economical quantity. This is because conventional nozzle or rotating atomizers typically generate a wide distribution of droplets. In conventional spray dryers, each droplet will dehydrate into a single particle. Therefore, the particle size produced will follow that of the initially atomized droplet. The relatively large initial droplet size is also closely linked with the size and throughput of the atomizer. Although there may be variations in atomizer design and operating conditions, in general, the size distribution may be reduced by using smaller atomizers, which also reduce the overall particle size. This strategy has been utilized in commercial laboratory scale equipments Citation[6]. However, the throughput is relatively low and it might not be economical for routine production purpose. There are other strategies used such as including surfactants and additives which are highly material-specific. This editorial focuses on a strategy to alleviate these aforementioned factors in the spray drying process. The key is in replacing the hot convective drying air with ethanol vapor (antisolvent vapor) as the drying medium.
2. Ethanol vapor as the convective drying medium
The premise of this concept lies in the absorption of ethanol vapor into the droplet during the convective drying process Citation[7]. As a result, particles are then formed by the precipitation process from within the droplet, due to the presence of ethanol, rather than by progressive solidification due to moisture removal by hot air. illustrates the difference between this new concept and the conventional spray drying. Typical spray drying produces a single particle from each droplet, whereas with the antisolvent vapor precipitation, hundreds of smaller particles can potentially be produced from a single droplet. This concept, so far, has been developed using the modified single-droplet drying technique in which a single droplet of 1 – 2 mm in diameter is statically suspended exposed to a convective stream of ethanol-nitrogen medium, mimicking a droplet within a spray dryer Citation[7]. Initial developmental work was undertaken with lactose as a model sugar pharmaceutical. Uniform spherical micron-sized amorphous particles or pollen-like crystalline particles were produced ( and ). The difference in morphology is due to different ethanol vapor concentrations. Although the exact detailed mechanism is still far from certain at the moment, there was an apparent critical ethanol vapor concentration from which a higher concentration resulted in the precipitation of the spherical particles Citation[8]. Investigation on the detailed mechanism of the process is still in progress. The hundreds of lactose microspheres in displayed high uniformity with a mean diameter of 2 µ with a span of ±1 µ. This initial observation illustrated the potential of this concept to produce uniform fine particles. Surprisingly, the concept also produced amorphous lactose particles which were not normally obtained by precipitating lactose from ethanol-water mixtures Citation[9]. We believe this is due to the unique mass transfer behavior of ethanol into the droplet, which sets it apart from the usual liquid-based antisolvent technique from which the liquid antisolvents are added to the formulation resulting in particle precipitation.
Figure 1. Comparison between conventional spray drying and the proposed antisolvent vapor precipitation approach is given.
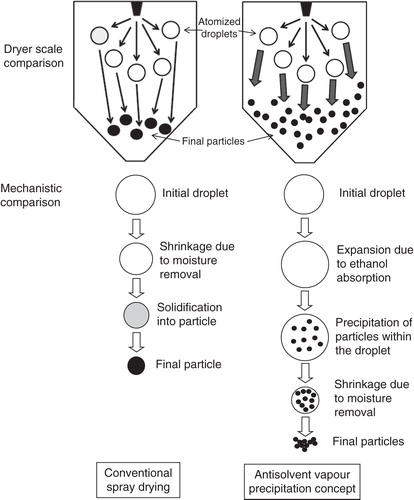
Figure 2. Antisolvent vapor precipitated particles: (A) lactose microspheres; (B) lactose micro crystals; (C) lactose + fish oil; (D) magnesium sulfate; (E) lactose + magnesium sulfate; (F) whey protein isolate microspheres and (G) whey protein isolate + lactose segregated particles are shown.
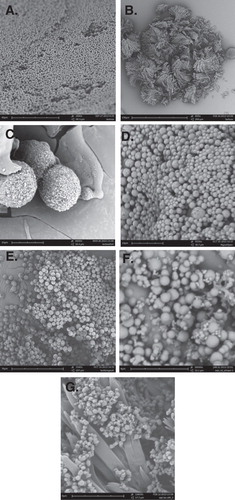
In view of the capability to produce uniform microsphere, the possibility of extending this concept to make micro-encapsulates for hydrophobic materials was further explored. Most encapsulates dealing with dispersed hydrophobic materials tend to form a continuous solid matrix as a large particle. Exploratory experiments undertaken showed that mini-encapsulates can be formed within a single droplet with the encapsulating materials Citation[10], in this case lactose, forming fibrous spheres directly onto the emulsified oil material (). This illustrates the potential of the technique to be an alternative to form micron-sized encapsulate particles. At the moment, however, the size of the micro-encapsulate is still relatively large and it was difficult to control the size of the distribution as it was highly linked with the size of the emulsified hydrophobic materials. In treating acute asthma, magnesium sulfate can be used as drugs Citation[11,12]. With the antisolvent vapor precipitation technique, micron-sized uniform magnesium sulfate particles can be produced albeit with slightly wrinkled surface morphology (). Combining the magnesium sulfate with dissolved lactose produced similar uniform particles but exhibiting a smoother surface morphology (). It will be interesting to further extend the technique to produce other pulmonary drug particles.
Further developmental work was undertaken for protein-based particles using whey proteins Citation[13]. Uniform particles of even smaller sizes were produced, down to the scale of hundreds of fine particles with a mean size of 200 nm (). A different strategy was required for producing such protein particles which involved the adjustment of the initial pH of the droplet. Although the complete dissolution of these particles is not fully assessed in the preliminary exploratory work, dispersion of the protein particles was observed from the single droplet dissolution study. From the experimental evidence, we believe that the technique can be used in producing protein-based particles for pulmonary delivery. The capability to generate submicron (nanoscale particles) will offer a useful avenue for improving the dissolution and uptake of potential protein-based pharmaceutics Citation[14,15]. It is noteworthy that the uniformity, however, is relative to the length scale of the particles produced. In the micrometer length scale, as observed for the lactose microspheres, the variation of ±1 µ in size can be considered relatively ‘uniform’, when compared to typical spray-dried particles. On the other hand, in the submicron length scale, particle sizes produced can vary by hundreds of nanometers as illustrated in . It is unclear, at the moment, if optimization of the process can narrow the size distribution of the particles in the submicron scale.
Another question then arises: What happens if we mix protein- and sugar-based excipient material into the initial droplet? Significant segregation can then occur as illustrated in . Such particles resemble a combination of protein particles embedded in a matrix of crystalline lactose. If further broken down, one possible method is via the ultrasonic technique; such morphology will resemble blended excipients and protein particles. Although not shown here, both the segregated materials can also be produced into spherical microspheres by adjustment of the antisolvent vapor concentration. Therefore, although the direct control in the formation of such morphologies is still far from certain, there is potential to generate such blends in a one-step approach during the particle formation process.
3. Conclusion
This editorial discussed the development of the antisolvent vapor precipitation technique for potentially producing particles for pulmonary drug delivery. The idea is to replace the hot air in a spray dryer, a common process used to produce drug particles, with ethanol (antisolvent) vapor. High uniformity and the fine size of the particles produced will certainly be attractive for pulmonary drug delivery application. From the lactose experiments, amorphous or crystalline morphology can be produced with the former being capable of enhancing dissolution of the delivered particles. The concept can also be extended to make micro-encapsulates for hydrophobic materials and was effective in producing magnesium sulfate microspheres – the latter is used in the treatment of acute asthma. Even finer submicron particles can be produced for protein-based materials which can potentially enhance the uptake of these materials in the pulmonary tract. There is possibility to use this approach as a one-step process to produce sugar-based protein particle blends.
4. Expert opinion
The developments in the preceding section were undertaken with the single droplet experimental technique which uses relatively large droplets of 1 – 2 mm in diameter. A typical droplet size in an actual spray dryer ranges in the order of 101 – 102 µ. In view of this, further experiments with actual sprayed droplets in the range of 15 – 60 µ statically exposed to convective antisolvent vapor were already undertaken in a series of uniquely controlled experiments. Reports for these experiments are now currently underway, awaiting provisional patenting of the process. In our opinion, the successful production of the fine uniform particles from this latest phase of experiments is clearly a proof of concept of this new production method. The team in Monash University is currently in the midst of implementing this in an actual dryer.
Once this technological hurdle is overcome, larger quantities of powders can then be produced for aerosolization analysis via the Anderson Cascade techniques. It is envisaged that subsequent developments will then shift toward the formulation aspect for this technology. One key aspect to consider would be the required contrasting solubility of the drug material in water and ethanol. This aspect will be even more complex if multiple solutes are present in the droplet. Such a situation might arise if additives are required to stabilize the particles or to generate a blend of particles using the potential one-step approach described earlier. On top of the formulation aspect, the ethanol vapor absorption process will also determine the final morphology of the particles. In view that the technique may produce amorphous particles such as those observed for the lactose particles, stability profile of the particles will be another important consideration in using the technology.
One can question on the economics of this method. Would the usage of ethanol vapor present a less economical option, and also a more hazardous one, when compared to that of hot air? The advantage of this process is the potential to generate fine uniform particles to enhance drug particle delivery where these two parameters (size and uniformity) are important. Therefore, the economic benefit in enhancement of the delivered drug should outweigh the additional costs if it were to be economically viable. Another key aspect is that this process has the potential to make ultrafine particles without the need for very fine droplet atomization which tend to limit the throughput of the process. In addition, the technique utilizes low (close to ambient) pressure in contrast to other high-pressure techniques such as the supercritical fluid precipitation technique Citation[16]. Although lower drying temperatures will have to be used in the presence of ethanol vapor, due to the potential hazards, such a condition might even be more favorable for protein particle production in light of possible thermal denaturation and aggregation associated with typical spray drying processes. These will certainly be important considerations, particularly from the manufacturer's perspective.
Declaration of interest
Financial support was gained from the Australia Research Council under the Discovery Project (DP130104836) to develop the antisolvent vapor precipitation technique.
Bibliography
- Frijlink HW, De Boer AH. Dry powder inhalers for pulmonary drug delivery. Expert Opin Drug Deliv 2004;1:67-86
- Edwards DA. Delivery of biological agents by aerosol. AICHE J 2002;48:2-6
- Pilcer G, Amighi K. Formulation strategy and use of excipients in pulmonary drug delivery. Int J Pharm 2010;392:1-19
- Odziomek M, Sosnowski TR, Gradon L. Conception, preparation and properties of functional carrier particles for pulmonary drug delivery. Int J Pharm 2012;433:51-9
- Vehring R. Pharmaceutical particle engineering via spray drying. Pharm Res 2007;25:999-1022
- Steckel H, Brandes HG. A novel spray-drying technique to produce low density particles for pulmonary delivery. Int J Pharm 2004;278:187-95
- Mansouri S, Fu N, Woo MW, Chen XD. Uniform amorphous lactose microspheres form convective –dehydration antisolvent precipitation under normal atmospheric pressure. Langmuir 2012;28:13772-6
- Mansouri S, Chin GQ, Ching TW, et al. Precipitating smooth amorphous or pollen structured lactose microparticles. Chem Eng J 2013;226:312-18
- Crisp JL, Dann SE, Blatchford CG. Antisolvent crystallization of pharmaceutical excipients from aqueous solutions and the use of preferred orientation in phase identification by powder X-ray diffraction. Eur J Pharm Sci 2011;42:568-77
- Yue S, Mansouri S, Woo MW, Chen XD. Antisolvent vapour precipitation of droplets with multi-components: non-soluble encapsulation and simultaneous precipitation of soluble materials. Chem Eng Res Des 2013;91:1705-14
- Sellers WFS. Inhaled and intravenous treatment in acute severe and life-threatening asthma. Br J Anaesth 2013;110(2):183-90
- Nannini LJ Jr, Pendino JC, Corna RA, et al. Magnesium sulfate as a vehicle for nebulized salbutamol in acute asthma. Am J Med 2000;108:193-7
- Mansouri S, Woo MW, Chen XD. Making uniform whey, lactose and composite lactose-whey particles from the dehydration of single droplets with antisolvent vapour. Dry Technol 2013;31:1570-7
- Onoue S, Hashimoto N, Yamada S. Dry powder inhalation systems for pulmonary delivery of therapeutic peptides and proteins. Expert Opin Ther Pat 2008;18:429-42
- Soares S, Costa A, Sarmento B. Novel non-invasive method of insulin delivery. Expert Opin Drug Deliv 2012;9:1539-58
- Dube A, Lemmer Y, Hayeshi R, et al. State of the art and future directions in nanomedicine for tuberculosis. Expert Opin Drug Deliv 2013;10(12):1725-34