Abstract
Calcium silicate nanostructured materials are promising drug carriers owing to their excellent biocompatibility, good bioactivity and high drug-loading capacity. In recent years, studies have been carried out on the synthesis of calcium silicate hydrate (CSH) nanostructured materials with desirable sizes and morphologies and their applications in drug delivery, where very interesting results and important insights have been documented. This editorial is not intended to offer a comprehensive review on the research on CSH nanostructured materials as drug carriers; rather, it presents representative examples: i) mesoporous microspheres; ii) ultrathin nanosheets; iii) iron oxide/CSH core/shell nanocomposites; and iv) CSH/block copolymer nanocomposites, and important results obtained in the study of CSH drug delivery systems for ibuprofen (IBU) as a model drug. These results show that the nanostructured CSH materials with specially designed architectures as IBU carriers have ultrahigh drug-loading capacity and sustainable drug release properties; thus, they are promising drug carriers for IBU. In addition, a new drug release kinetics has been found in the nanostructured CSH drug delivery systems. Most recently, new insight has been gained by tracking the behavior of these drug delivery systems on the molecular level using synchrotron-based X-ray spectroscopy.
Calcium silicates have many different forms with a suite of chemical compositions; the most common forms are CaSiO3, Ca2SiO4, Ca3SiO5, etc. Calcium silicates usually occur in hydrated form containing various percentages of water of crystallization. Calcium silicate hydrate (CSH) possesses a remarkable level of structural complexity with various Ca/Si ratios, and more than 30 crystalline CSH phases are known. CSH materials prepared under ambient conditions exhibit structures ranging from semicrystalline to nearly amorphous Citation[1,2]. Despite research on CSH materials for decades, the exact structures of various CSH phases are still under debate due to their structural complexity and poor crystallinity.
The story of CSH mesoporous microspheres in drug delivery began with a report of Zhu et al. Citation[3]; they prepared hierarchically nanostructured CSH mesoporous microspheres (HNMS-CSH) by the self-assembly of nanosheets as building blocks using the low-cost, surfactant-free sonochemical method. The CSH mesoporous microspheres thus obtained are relatively uniform with the sizes of approximately 1 μm and the nanosheet building blocks have thicknesses of approximately 30 nm. The CSH mesoporous microspheres are composed of poorly crystallized Ca3Si2O7·xH2O, where x ∼ 6. Their performance with different weight ratios of ibuprofen (IBU) to CSH carrier (n/100) is illustrated in .
Figure 1. (A) N2-adsorption–desorption isotherms and pore size distribution curves of HNMS-CSH and their ibuprofen drug delivery systems (IBU–HNMS-CSHn), where n/100 is the feeding weight ratio of IBU to HNMS-CSH; (B) TG curves of HNMS-CSH and IBU–HNMS-CSHn; (C) IBU-release profiles of the IBU–HNMS-CSHn drug delivery systems in SBF and (D) the cumulative IBU-release percentage versus natural logarithm of release time plots for IBU–HNMS-CSHn drug delivery systems (n = 200, 267 and 400).
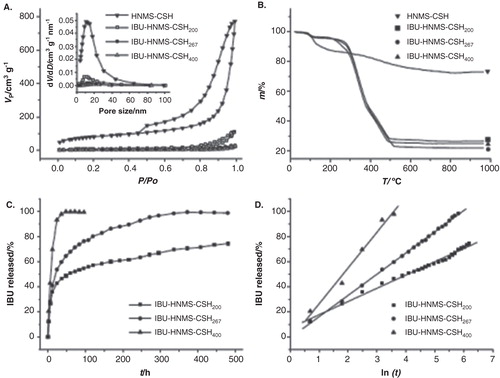
The average pore size of CSH mesoporous microspheres is 10.8 nm (), with a BET specific surface area and a cumulative pore volume of 290 m2 g–1 and 1.20 cm3 g–1, respectively (). The structural features of HNMS-CSH are highly desirable for drug delivery because they provide a large specific surface area, mesopores and interconnected nanochannels for drug loading and release. IBU has been commonly chosen as a model drug for the research on drug loading and release. IBU belongs to a class of drugs called nonsteroidal anti-inflammatory drugs. IBU is used for the management of mild to moderate pain, fever and inflammation.
Table 1. The experimental results for the IBU–HNMS-CSHn drug delivery systems.
A series of drug delivery systems based on CSH mesoporous microspheres, named IBU–HNMS–CSHn, where n/100 is the feeding weight ratio of IBU to HNMS-CSH, has been recently investigated for IBU loading and release. For the experimental procedure used for the IBU loading, please refer to Citation[3]. Upon loading of the IBU into CSH mesoporous microspheres, the peak of the pore size distribution curves decreases drastically (the inset in ), and this is accompanied by a significant drop in specific surface area and pore volume. The drug-loading capacities (the weight of loaded IBU in the carrier/the weight of CSH carrier × 100%) and IBU-loading efficiencies are listed in . It is apparent from that the IBU drug-loading capacities in these drug delivery systems are ultrahigh and that the IBU–HNMS-CSH267 system exhibits the highest drug-loading capacity (229 wt%). To the best of our knowledge, this is the highest drug-loading capacity for IBU reported in the literature.
The drug release profiles of the IBU–HNMS-CSHn drug delivery systems in simulated body fluid (SBF) are shown in , where one can see that there are obvious differences in the drug release rates among different drug delivery systems. For the IBU–HNMS-CSH200 drug delivery system, approximately 75% of loaded IBU is released in approximately 480 h. For the IBU–HNMS-CSH267 drug delivery system, the drug release is nearly complete in approximately 372 h. These two systems show a sustained release of IBU with a long period of drug release time, which can prolong the therapeutic effect of the drug. On the other hand, the drug delivery systems of IBU–HNMS-CSH200 and IBU–HNMS-CSH267 display relatively high drug release rates at the early stage, with approximately 36 and 41% of loaded IBU released, respectively, in the first 12 h. The rapid drug release at the early stage is attributable to IBU molecules adsorbed on the external surfaces and in the nanopores. This relatively rapid drug release at the early stage is necessary for achieving the desired level of the drug concentration required for therapeutic treatment in a short period of time. However, the IBU–HNMS-CSH400 system displays an initial rapid drug release, by which approximately 43% of the loaded drug is released in the first 6 h and the release is nearly completed in 36 h. This type of drug release profile can be utilized when an immediate high dosage of the drug is required, for instance, for an acute infection or inflammation. The chemical composition of the CSH drug carrier transforms to hydroxyapatite after the drug release in SBF for 480 h, exhibiting good bioactivity of the CSH mesoporous microspheres. The slow drug release at the later stage of IBU–HNMS-CSH200 and IBU–HNMS-CSH267 is associated with the formation of hydroxyapatite, which gradually coats the pore channels. The IBU–HNMS-CSH400 system initially displays a relatively rapid drug release effect in which the loaded drug is almost completely released before the pores are capped by hydroxyapatite.
The kinetics of drug release from carriers can be usually described using the Higuchi model (C = k • t1/2) Citation[4-6] with a linear relationship between the cumulative amount of released drug, C, and the square root of release time, t1/2, where k is a constant, and drug release is governed by a diffusion process. However, Zhu et al. Citation[3] have found a new drug release kinetics in the drug delivery systems of IBU–HNMS-CSHn (), in which, in contrast to the Higuchi model, the cumulative amount of released drug (C) has a linear relationship with the natural logarithm of release time (t):
C = k • ln(t),
where k is a constant. For IBU–HNMS-CSH200, IBU–HNMS-CSH267 and IBU–HNMS-CSH400, k is determined to be 10.6, 16.9 and 28.6, respectively. The constant k is an empirical constant, which relates to the drug release rate. The bigger k value stands for the higher drug release rate. This new drug release kinetics has also been found in nanostructured calcium phosphate-based drug delivery systems Citation[7-9].
Another work on ultrathin CSH nanosheets with a thickness of approximately 2.8 nm and an ultrahigh specific surface area is noteworthy Citation[10]. The specific surface area of the CSH ultrathin nanosheets can reach as high as 505 m2 g−1, and to the best of our knowledge, this is the highest value reported for calcium silicate materials in the literature. This system exhibits little cytotoxicity and becomes anhydrous upon heat treatment. The highest IBU drug adsorption efficiency is 94 wt%, and the highest adsorption capacity for IBU is as high as 220 wt%, which is similar to that of mesoporous CSH microspheres. It has been found that the IBU adsorption on the CSH ultrathin nanosheets in the hexane system is based on the chemical interaction between –COOH groups from the IBU molecules and Ca2+ cations from the CSH nanocarrier Citation[10].
Multifunctional drug delivery systems are increasingly popular in the medical diagnosis and therapy. The core/shell-structured mesoporous nanocomposite consisting of magnetic iron oxide nanoparticles as the core and CSH as the shell has been recently synthesized using a two-liquid–phase system by ultrasound irradiation, in which the hydrophobic phase is composed of hydrophobic Fe3O4 nanoparticles and tetraethyl orthosilicate, and the aqueous phase consists of Ca(NO3)2, NaOH and water Citation[11]. The hollow mesoporous nanocomposite consisting of magnetic iron oxide nanoparticles and CSH is obtained by adding inert hydrophobic solvent isooctane in the reaction system. The as-prepared nanocomposite exhibits a superparamagnetic behavior with a high specific surface area (474 m2 g−1), high pore volume (2.75 cm3 g−1) and high drug-loading capacity for IBU (103 wt%).
Amorphous CSH/block copolymer monomethoxy-(polyethyleneglycol)-poly (lactide-co-glycolide) (CSHP) nanoparticles have been prepared in aqueous solution via a facile co-precipitation route at room temperature Citation[12]. The IBU drug-loading capacity of the CSHP nanoparticles is ultrahigh (∼ 190 wt%), and the IBU loading efficiency reaches as high as approximately 100%. The loaded IBU in CSHP nanoparticles can be released in SBF for a long period of time (about 300 h), during which the CSHP nanocarrier completely transforms to hydroxyapatite, exhibiting good bioactivity.
X-ray absorption near-edge spectroscopy (XANES) is an element and site-specific core-level absorption spectroscopic technique, which allows for tracking the chemical environment of the absorbing atom. In XANES, X-ray with energies in the vicinity and above the threshold excites the core electron to the unoccupied bound states, quasi-bound states and the continuum and probes the local environment in terms of bonding with neighboring atoms and local coordination and symmetry; the excited electron brings back these information in the form of a modulated absorption coefficient above the monotonic atomic background. A recent XANES study of CSH–IBU interaction clearly shows the interaction of Ca2+ of CSH with the carboxyl group of IBU and the effect on the rest of the system, the Si and the O site, for example Citation[13]. This technique has also been applied to track the behavior of Fe3O4 particles in connection with drug delivery Citation[14]. Using a nano X-ray beam, an individual microsphere has been examined with spectromicroscopy. These techniques will play an increasingly significant role in understanding the drug–carrier interaction in the loading and unloading processes; hence, the kinetics Guo X et al. (in preparation). shows the Ca K-edge XANES where Ca2+–IBU interaction is apparent in the first derivative of the XANES. Synchrotron studies already underway using a 30 nm X-ray beam confirm the CSH–IBU interaction on an individual microsphere and the formation of hydroxyapatite. The activation energy for the processes clearly lies in the IBU–CSH interaction in the drug-loading process and IBU–CSH–SBF interaction in the unloading process. However, more research is needed to clarify the exact mechanism behind the new drug release kinetics.
Expert opinion
Several important factors influence the drug-loading and-release properties of the drug carriers: these include chemical composition, structure, size, morphology, porosity, etc. Appropriate materials, high specific surface area, large pore volume and well-defined microstructures with interconnected nanoporous networks of the drug carriers are vital factors for achieving high drug-loading capacities and favorable drug release behaviors. CSH materials have promising applications in many biomedical fields because of their excellent biocompatibility, good bioactivity, biodegradability, high drug-loading capacity and favorable drug release behaviors, as demonstrated in the examples described above. Although high specific surface area and large pore volume of the drug carrier are vital factors for high drug-loading capacity, the intrinsic nature of carrier materials is crucial for high drug-loading capacity. IBU molecules can form the linkage to CSH carrier by the electrostatic interaction between Ca2+ cations and carboxyl groups under basic circumstances, and the linkage will break under acidic conditions. Coincidentally, CSH exhibits basicity with Ca2+-enriched surface. Therefore, the chemical interaction between IBU molecules and the CSH carrier is a vital factor for the extremely high drug-loading capacity of the CSH-based drug carriers, apart from high specific surface area and high pore volume.
The structural features of the as-prepared HNMS-CSH are advantageous for ultrahigh drug-loading capacity (229 wt%) and sustained drug release. The CSH-based nanostructured IBU drug delivery systems have very long drug release times (usually hundreds of hours), which can significantly prolong the therapeutic effect of the drug. For IBU drug delivery applications, calcium silicate nanostructured materials are promising for the implantable local drug-delivery devices that can reduce the inflammation and pain after the bone/tooth repair surgeries, with the in situ combination of hard tissue repair and drug delivery. The formulation that gives a relatively fast drug release profile is suitable for the treatment of an acute inflammation that needs a high drug dosage in a short period of time. The formulation that gives a long-time drug release profile is suitable for relieving the pain and preventing inflammation in the recovery stage that needs a long-term sustained drug dosage. Furthermore, the combined use of the fast drug-release and long-term drug-release formulations with proper ratios can meet different demands for the clinical hard tissue repair applications with drug delivery function.
For the first time, a new drug release kinetics has been found in the drug delivery systems of HNMS-CSH, in which there is a linear relationship between the cumulative amount of drug released and the natural logarithm of the release time. Furthermore, CSH nanocarrier can entirely transform to hydroxyapatite after drug release in SBF, implying its good bioactivity. Currently, the detailed mechanisms for this drug release kinetics are still not fully understood, and more research is needed to clarify this issue. Preliminary experimental results obtained in the drug delivery systems based on nanostructured CSH and calcium phosphate carriers where this new drug release kinetics is applicable indicate that the crystal phase transformation from the CSH or amorphous calcium phosphate to hydroxyapatite occurs. We propose that the combined effects of several factors such as the crystal phase transformation, desorption of drug molecules from the carrier and diffusion of drug molecules into solution are responsible for this drug release kinetics.
The recent introduction of advanced photon techniques using synchrotron radiation has greatly enhanced the characterization capabilities in tracking drug–carrier interaction on the molecular level, which in turn help identify the nature of the interaction in both the drug-loading and the -unloading process. These techniques will play an increasingly significant role in understanding the drug–carrier interaction in the loading and unloading processes, hence the kinetics.
Future research directions of CSH nanostructured material-based drug delivery systems will mainly focus on the design, synthesis and applications in drug delivery of the nanostructured CSH drug carriers with well-defined chemical composition, structure, size, morphology and porosity, and CSH-based nanocomposites with biocompatible polymers, multifunctional CSH-based nanostructured drug carriers and, importantly, the mechanisms regarding the CSH-based drug delivery systems, drug release kinetics and the interactions between the drug and the carrier.
Declaration of interest
Financial support from the National Natural Science Foundation of China (51172260) and the Science and Technology Commission of Shanghai (11nm0506600) is gratefully acknowledged. Research conducted at the University of Western Ontario is supported by the Natural Science and Engineering Research Council of Canada, the Canada Research Chairs and the Canadian Foundation for Innovation. The authors have no other relevant affiliations or financial involvement with any organization or entity with a financial interest in or financial conflict with the subject matter or materials discussed in the manuscript apart from those disclosed.
Notes
Bibliography
- Taylor HFW. Cement chemistry. 2nd edition. Thomas Telford; London, UK: 1997
- Chen JJ, Thomas JJ, Taylor HFW, Jennings HM. Solubility and structure of calcium silicate hydrate. Cement Concr Res 2004;34:1499-519
- Wu J, Zhu YJ, Cao SW, Chen F. Hierarchically nanostructured mesoporous spheres of calcium silicate hydrate: surfactant-free sonochemical synthesis and drug-delivery system with ultrahigh drug-loading capacity. Adv Mater 2010;22:749-53
- Vallet-Regí M, Balas F, Arcos D. Mesoporous materials for drug delivery. Angew Chem Int Ed 2007;46:7548-58
- Higuchi T. Mechanism of sustained-action medication. Theoretical analysis of rate of release of solid drugs dispersed in solid matrices. J Pharm Sci 1963;52:1145-9
- Andersson J, Rosenholm J, Areva S, Lindén M. Influences of material characteristics on ibuprofen drug loading and release profiles from ordered micro- and mesoporous silica matrices. Chem Mater 2004;16:4160-7
- Chen F, Zhu YJ, Zhang KH, et al. Europium-doped amorphous calcium phosphate porous nanospheres: preparation and application as luminescent drug carriers. Nanoscale Res Lett 2011;6:67
- Tang QL, Zhu YJ, Wu J, et al. Calcium phosphate drug nanocarriers with ultrahigh and adjustable drug-loading capacity: one-step synthesis, in situ drug loading and prolonged drug release. Nanomedicine 2011;7:428-34
- Qi C, Zhu YJ, Zhao XY, et al. Highly stable amorphous calcium phosphate porous nanospheres: microwave-assisted rapid synthesis using ATP as phosphorus source and stabilizer, and their application in anticancer drug delivery. Chem Eur J 2013;19:981-7
- Wu J, Zhu YJ, Chen F. Ultrathin calcium silicate hydrate nanosheets with large specific surface areas: synthesis, crystallization, layered self-assembly and applications as excellent adsorbents for drug, protein, and metal ions. Small 2013;9(17):2911-25
- Lu BQ, Zhu YJ, Ao HY, et al. Synthesis and characterization of magnetic iron oxide/calcium silicate mesoporous nanocomposites as a promising vehicle for drug delivery. ACS Appl Mater Interfaces 2012;4:6969-74
- Wu J, Zhu YJ, Chen F, et al. Amorphous calcium silicate hydrate/block copolymer hybrid nanoparticles: synthesis and application as drug carriers. Dalton Trans 2013;42:7032-40
- Guo XX, Wu J, Yiu YM, et al. Drug–nanocarrier interaction—tracking the local structure of calcium silicate upon ibuprofen loading with X-ray absorption near edge structure (XANES). Phys Chem Chem Phys 2013;15:15033-40
- Chen L, Razavi FS, Mumin A, et al. Multifunctional nanoparticles for rapid bacterial capture, detection, and decontamination. RSC Adv 2013;3:2390-7