Figure 1. (A) Preoperative 18F-fluorodeoxyglucose (FDG) PET/CT maximum intensity projection and (B) cross sectional fused 18F-FDG PET/CT images of a colorectal cancer patient demonstrating a solitary hypermetabolic focus in the sigmoid colon. (C) Postoperative 18F-FDG PET/CT maximum intensity projection of the lower abdomen and pelvis demonstrates no evidence of hypermetabolism in the region of the previously resected sigmoid mass.
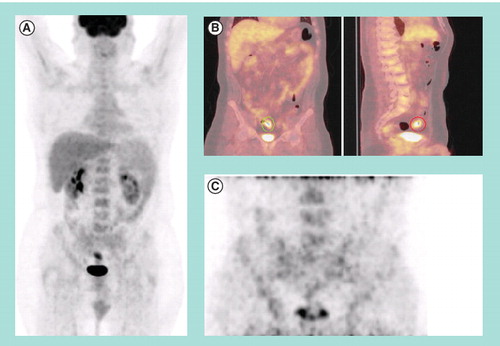
Figure 2. (A) Digital photograph of a resected sigmoid colon specimen with magnified region of interest. Suture placed at suspected tumor site based on intraoperative palpation and γ-probe detection. (B) Fused 18F-fluorodeoxyglucose PET/CT image of the resected sigmoid colon specimen. (C) Micro-PET/CT image of the specimen illustrates intense hypermetabolic activity at the tumor site and with only diffuse tracer uptake in the surrounding normal colon tissue.
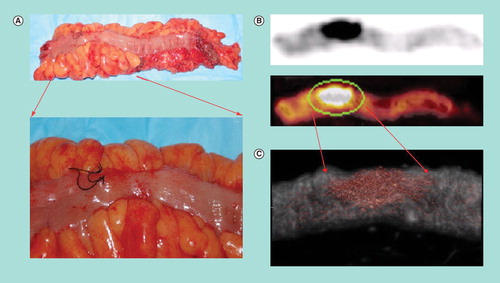
Figure 3. (A) Digital photograph of cut section of a portion of a resected liver tumor after preoperative injection of 18F-fluorodeoxyglucose. (B) Corresponding PET imaging of the same portion of the resected liver tumor, with dark spot representing resected liver tumor and surrounding lighter area representing normal liver tissue. Digital photograph (C), PET image (D), CT image (E) and fused PET/CT image (F) of the same portion of the resected liver tumor after it was divided into multiple small pieces. The metabolic activity quantified in the specimen pieces correlates well with the tumor burden.
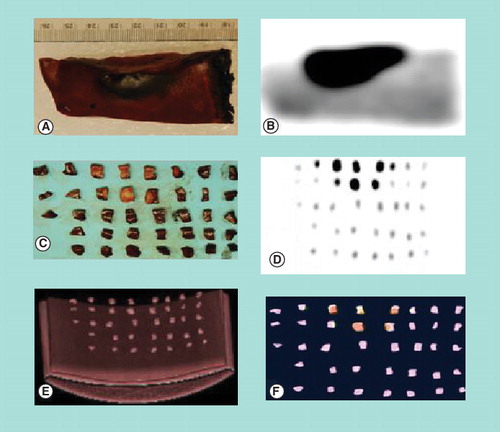
Medical imaging technology plays a critical role in the management and care of cancer patients. Diagnostic imaging is currently used for screening, diagnosis, staging and restaging of disease, as well as monitoring response to therapy. Historically, morphologic imaging strategies, such as computed tomography (CT), have provided useful information on size and extent of disease, as well as tumor size measurements for assessing response to therapy Citation[1]. More recently, functional imaging strategies, such as combined PET/CT using 18F-fluorodeoxyglucose (FDG), have undergone rapid development and are being explored as molecular imaging biomarkers for a wide variety of malignancies Citation[2–5].
The utility of functional imaging, specifically 18F-FDG PET/CT diagnostic imaging, has been widely demonstrated for diagnosis, staging and restaging, as well as monitoring response to therapy for a variety of cancer types Citation[6]. These advances have significantly improved the physician’s ability to determine the most appropriate therapeutic approach for cancer patients and may ultimately lead to a reduction in morbidity to cancer patients by avoiding unnecessary invasive procedures and pharmacologic therapies that may not be of real benefit. Despite the rapid growth of evidence-based medicine surrounding functional imaging technology, there are relatively few data describing the utilization of functional imaging for guiding real-time therapy decision-making. For example, while 18F-FDG PET/CT diagnostic imaging is extensively used for preoperative planning, we have not seen evidence of this functional imaging being utilized in a real-time fashion, within the operating room, for making critical surgical treatment decisions. However, we are now beginning to see this technology utilized in such a fashion.
The primary goal of cancer surgery is complete resection of all known disease. Postoperatively, the most frequently asked question by both patients and their families is “Did you get it all?”. If the surgeon knows that there is tumor left behind in the patient, then the answer is simply “No”. Commonly, the honest answer would be “I think so” or “I hope so”, but less commonly can the surgeon definitely answer “Yes”. Too often, days after the operation has been performed, the final pathology report will describe the finding of a positive margin on the surgically resected specimen. In many of these cases, it is evident that the patient underwent an invasive surgical procedure which may not have provided maximal benefit. If, however, surgeons had access to intraoperative real-time information regarding the precise location of all disease and had a real-time assessment of surgical resection margins, they would be able to intervene immediately to accomplish a complete resection without subjecting the patient to subsequent additional surgical procedures. In addition to these advantages, tangible confirmation of complete tumor resection on the day of surgery would be of potential psychological benefit to patients and their families. Therefore, it is imperative to utilize our current medical imaging technologies and design future imaging technological approaches for intraoperative real-time applications.
While it is well-established that 18F-FDG PET/CT diagnostic imaging is a powerful tool for assisting in the diagnosis as well as in the staging/restaging and monitoring of therapeutic response for a variety of solid malignancies, one of its main limitations relates to the standard practice of utilizing preoperative image acquisition at the time of the initial evaluation of cancer patients for the surgical planning of such patients. Such a preoperative image acquisition approach does not adequately translate into real-time information that is available within the operating room, biopsy suite or pathology department. It is frequently very difficult for the surgeon to intraoperatively localize 18F-FDG-avid abnormalities that were previously evident on preoperative images acquired at the time of the initial work-up. As a consequence, this may result in the inability to detect occult sites of disease and may lead to incomplete surgical resection of tumor at the time of definitive cancer surgery. Furthermore, similar limitations also exist with respect to the localization of suspicious tissue for diagnostic biopsy, as well as to localize malignant cells within a surgically resected specimen or a biopsy specimen once it has been obtained. All of these limiting variables may ultimately be responsible for delay in diagnosis, disease progression or disease recurrence, thus impacting negatively upon long-term patient outcomes. Thus, providing the operating surgeon, the physician performing a diagnostic biopsy and the pathologist access to imaging and metabolic information at the time of their intervention has great potential to significantly improve overall success of the management of these cancer patients and may ultimately impact positively upon long-term patient outcomes.
Early detection is an important variable in the successful management of all malignancies. Two confounding factors with regard to accurately making the correct diagnosis early on are the potential inaccuracy in tissue sampling technique (i.e., sampling the correct lymph node or the metabolically active portion of a heterogeneous tumor) and, subsequently, the potential sampling error induced during pathologic evaluation (i.e., a normal section of a specimen is analyzed while the tumor cells exist in another section of the specimen). Incorporation of radioguided molecular imaging and detection techniques has the potential for guiding biopsy localization as well as pathologic tissue sampling, and could ultimately have an enormous impact on our ability to diagnose cancer at an earlier stage than ever before.
For many malignancies, the risk of disease recurrence remains deceptively high despite presumption of complete resection at the time of initial surgical management. Therefore, incomplete resection of disease at the time of surgery may be the main contributor to high recurrence rates and resultant increased mortality. The availability of intraoperative radioguided molecular imaging and detection to verify complete tumor resection and detection of occult disease would be of great utility in improving the ability of the surgeon to achieve complete extirpation of tumor at the time of the operative procedure.
Our group at The Ohio State University (OH, USA) has published promising preliminary data with 18F-FDG for attempting to improve the care of cancer patients by utilizing an integrated multimodal approach of radioguided molecular imaging and detection technologies, as applied to colorectal cancer Citation[7], breast cancer Citation[8], melanoma Citation[9], ovarian cancer Citation[10], lung cancer Citation[11] and thyroid cancer Citation[12]. This integrated multimodal approach involves administration of a radioactive tracer, specifically 18F-FDG, prior to the surgical procedure. Perioperative 18F-FDG PET/CT diagnostic imaging is generally performed just prior to the surgical procedure and then again after the surgical resection, based on the same 18F-FDG dose administered prior to surgery . Intraoperatively, detection and resection of 18F-FDG-avid tissue is guided by a handheld γ-detection probe. The utilization of the intraoperative γ-detection probe in radioimmunoguided surgery has been previously and extensively investigated with the non-positron emitting radiopharmaceutical 125I for a variety of malignancies, including colorectal, gastric, ovarian and breast Citation[13–16]. More recently, positron emitting radiopharmaceuticals, such as 124I in radioimmunoguided surgery for renal cell cancer Citation[17] and 18F-FDG in 18F-FDG-directed surgery for colorectal cancer, breast cancer, melanoma, ovarian cancer, lung cancer and thyroid cancer have been investigated Citation[7–12]. The safety of these 18F-FDG-directed surgical procedures, with respect to occupational radiation exposure to all involved intraoperative and perioperative personnel, has been well characterized by our group at The Ohio State University Citation[18].
The comprehensive approach employed in our investigations is novel and unique, and addresses many of the specific limitations that are encountered with the current management of cancer patients, including:
• The limited ability of imaging procedures to detect microscopic metastatic disease;
• The limited ability to accurately sample tissue identified by molecular imaging techniques (which may be morphologically normal);
• The inaccurate correlation of preoperative imaging findings with the actual anatomy of the patient within the operating room;
• The limited ability of the surgeon to localize tumor and metastatic deposits intraoperatively based on the preoperative evaluation and imaging;
• The lack of intraoperative real-time verification of complete resection of disease;
• The inaccurate and noncomprehensive assessment of tumor margins in real-time during the operative procedure.
The potential benefits of exploring and integrating these functional imaging approaches include the ability to address the logistical difficulties of completely and comprehensively evaluating a surgical resection specimen for evidence of tumor based on limited time and resources, as well as overcoming potential sampling error issues based on restrictions in the ability to evaluate only a limited portion of the entire submitted surgical resection specimen. Resolving these issues may lead to more accurate intraoperative real-time assessment of surgical resection margins, especially when the final pathology report can take days to be completed. Developing these innovative and integrated multimodal approaches for radioguided molecular imaging and detection, and for surgical resection specimen evaluation in a real-time environment may ultimately revolutionize diagnosis, operative management and pathologic evaluation involved in the treatment of cancer patients. Such integrated radioguided molecular imaging and detection techniques could improve complete response rates and prolong disease-free survival for a wide variety of malignancies. The downstream effects of this could translate into better quality of life and significant reduction in the costs associated with the healthcare of cancer patients. Molecular imaging guidance allows for more directed pathological evaluation of tissue specimens and could improve the accuracy of appropriate tissue sampling in patients with known or suspected malignancies. This technology requires development of detection devices that would work in conjunction with biopsy instrumentation. Development and implementation of this technology would improve the ability to sample the most appropriate lymph nodes, as well as sample the most appropriate portions of soft tissue masses that have the highest likelihood of containing tumor cells versus other portions that may only contain necrotic tissue.
Molecular imaging of surgical specimens provides another unique opportunity for the utilization of state-of-the-art imaging technology to improve the treatment of cancer patients. Assessment of surgical resection specimens with radioguided molecular imaging and detection techniques, in both a perioperative and intraoperative fashion, will complement the patient imaging techniques described previously, as well as provide new mechanisms for assisting the pathologist in performing directed pathology for the most accurate sampling and evaluation of these surgical resection specimens. Such surgical specimens, removed from the patient by the standard surgical procedures, can be imaged with PET/CT (either by a standard clinical scanner unit or by a micro-PET/CT scanner unit). These microimaging systems have the potential for being easily transported into the operating arena, allowing for the possibility of surgical resection specimen scanning in the operating room at the time of resection. This concept of specimen scanning would allow for immediate evaluation of the entire surgical resection specimen. Such a technique, as illustrated by , would allow for complete evaluation of the surgical resection margins before the operative procedure is completed, and potentially overcome some of the limitations of the current standard frozen section analysis for evaluating the surgical resection margins at the time of surgery. The benefits of utilizing molecular imaging technology to evaluate surgical resection specimens includes assessment of the success of the surgical procedure by verifying tumor resection and negative surgical margins, assisting pathology in accurate sampling of surgical specimens to increase sensitivity and accuracy for locating tumor cells within a specimen and assessing tumor margins, and potentially assisting pathology in evaluation of lymph nodes resected during surgery by identifying lymph nodes that have the highest probability of containing tumor cells. An example of the imaging schema for the assessment of the surgical resection margin for a resected liver tumor specimen is shown in .
The integration of molecular imaging and intraoperative radioguided detection into a multimodal technologic approach has the potential to significantly improve and revolutionize the practice of surgical oncology for the care of cancer patients. 18F-FDG PET/CT imaging has demonstrated significant promise in assisting the surgeon for intraoperatively localizing and completely resecting primary and recurrent tumors, as well as occult disease. If medical imaging technology is further advanced with the development of intraoperative imaging devices for imaging both the patient as well as the resected surgical specimens, then postresectional intraoperative imaging could provide immediate information to the surgeon regarding any tumor that may remain within the patient and will provide the opportunity for immediate and further surgical intervention and complete resection of disease prior to the patient leaving the operating room. This intraoperative real-time feedback will improve the ability of the surgeon to accomplish complete surgical extirpation of disease at the initial time of definitive cancer surgery. Therefore, it is clear that the further development of medical imaging technology and detection strategies that can be integrated in the operating room environment, as well as development of more cancer-specific radioactive tracers, will have a great potential for significantly improving the sensitivity for detecting and resecting primary/recurrent tumors and identifying occult metastatic disease and may ultimately translate into better care and improved outcomes for cancer patients.
Financial & competing interests disclosure
The authors have no relevant affiliations or financial involvement with any organization or entity with a financial interest in or financial conflict with the subject matter or materials discussed in the manuscript. This includes employment, consultancies, honoraria, stock ownership or options, expert testimony, grants or patents received or pending, or royalties.
No writing assistance was utilized in the production of this manuscript.
References
- Jaffe CC. Measures of response: RECIST, WHO, and new alternatives. J. Clin. Oncol.24(20), 324–3251 (2006).
- Multiagency effort to focus on PET as biomarker. J. Nucl. Med.47(4), 11N (2006).
- Dehdashti F, Mortimer JE, Trinkaus K et al. PET-based estradiol challenge as a predictive biomarker of response to endocrine therapy in women with estrogen-receptor-positive breast cancer. Breast Cancer Res. Treat. DOI 10.1007/s10549-008-9953-0 (2008) (Epub ahead of print).
- Larson SM, Schwartz LH. 18F-FDG PET as a candidate for “qualified biomarker”: functional assessment of treatment response in oncology. J. Nucl. Med.47(6), 901–903 (2006).
- Zheng QH, Gardner TA, Raikwar S et al. [11C] Choline as a PET biomarker for assessment of prostate cancer tumor models. Bioorg. Med. Chem.12(11), 2887–2893 (2004).
- Fletcher JW, Djulbegovic B, Soares HP et al. Recommendations on the use of 18F-FDG PET in oncology. J. Nucl. Med.49(3), 480–508 (2008).
- Sarikaya I, Povoski SP, Al-Saif OH et al. Combined use of preoperative 18F-FDG-PET imaging and intraoperative γ probe detection for accurate assessment of tumor recurrence in patients with colorectal cancer. World J. Surg. Oncol.5, 80 (2007).
- Hall NC, Povoski SP, Murrey DA, Knopp MV, Martin EW Jr. Combined approach of perioperative 18F-FDG PET/CT imaging and intraoperative 18F-FDG handheld γ-probe detection for tumor localization and verification of complete tumor resection in breast cancer. World J. Surg. Oncol.5, 143 (2007).
- Povoski SP, Hall NC, Martin EW Jr, Walker MJ. Multimodality approach of perioperative 18F-FDG PET/CT imaging, intraoperative 18F-FDG handheld γ probe detection, and intraoperative ultrasound for tumor localization and verification of resection of all sites of hypermetabolic activity in a case of occult recurrent metastatic melanoma. World J. Surg. Oncol.6, 1 (2008).
- Cohn DE, Hall NC, Povoski SP et al. Novel perioperative imaging with 18F-FDG PET/CT and intraoperative 18F-FDG detection using a handheld γ probe in recurrent ovarian cancer. Gynecol. Oncol.110(2), 152–157 (2008).
- Moffatt-Bruce SD, Povoski SP, Sharif S et al. A novel approach to positron emission tomography in lung cancer. Ann. Thorac. Surg.86(4), 1355–1357 (2008).
- Agrawal A, Hall NC, Ringel MD, Povoski SP, Martin EW Jr. Combined use of perioperative TSH-stimulated 18F-FDG PET/CT imaging and γ probe radioguided surgery to localize and verify resection of iodine scan-negative recurrent thyroid carcinoma. Laryngoscope (2008) (In Press).
- Bell J, Mojzisik C, Hinkle G Jr et al. Intraoperative radioimmunodetection of ovarian cancer using monoclonal antibody B72.3 and a portable γ-detecting probe. Obstet. Gynecol.76(4), 607–611 (1990).
- Martin EW Jr, Mojzisik CM, Hinkle GH Jr et al. Radioimmunoguided surgery using monoclonal antibody. Am. J. Surg.156(5), 386–392 (1988).
- Nieroda CA, Mojzisik C, Sardi A et al. Staging of carcinoma of the breast using a hand-held γ detecting probe and monoclonal antibody B72.3. Surg. Gynecol. Obstet.169(1), 35–40 (1989).
- Sun D, Bloomston M, Hinkle G et al. Radioimmunoguided surgery (RIGS), PET/CT image-guided surgery, and fluorescence image-guided surgery: past, present, and future. J. Surg. Oncol96(4), 297–308 (2007).
- Strong VE, Humm J, Russo P et al. A novel method to localize antibody-targeted cancer deposits intraoperatively using handheld PET β and γ probes. Surg. Endosc.22(2), 386–391 (2008).
- Povoski SP, Sarikaya I, White WC et al. Comprehensive evaluation of occupational radiation exposure to intraoperative and perioperative personnel from 18F-FDG radioguided surgical procedures. Eur. J. Nuc. Med. Mol. Imaging35(11), 2026–2034 (2008).