Abstract
Tissue adhesive sealants have been used as substitutes for sutures in ophthalmic surgery in recent years since the latter may cause irritation, inflammation and infection. Tissue adhesives were developed as suture adjuncts and alternatives for sealing wounded tissues. They are gaining popularity for their ease of use and postoperative comfort. Two broad classes of tissue adhesives, synthetic and biological, have been reported in previous studies. Cyanoacrylate-based synthetic tissue adhesives have been conventionally used for corneal perforation surgeries. Fibrin glue is a bioadhesive developed from blood plasma. Aside from these surface sealants, a new class of compounds termed biodendrimers has also found a use in ophthalmic surgery. Other adhesives in development include acrylic-based adhesives, polyethylene glycol hydrogels, chondroitin sulfate, riboflavin–fibrinogen compounds, photoactivated serum albumin solder and photo-polymerized hyaluronic acid compounds. This article aims to cover the therapeutic uses and application techniques of the aforementioned tissue adhesives in ophthalmology.
Medscape: Continuing Medical Education Online
This activity has been planned and implemented in accordance with the Essential Areas and policies of the Accreditation Council for Continuing Medical Education through the joint sponsorship of Medscape, LLC and Expert Reviews. Medscape, LLC is accredited by the ACCME to provide continuing medical education for physicians.
Medscape, LLC designates this Journal-based CME activity for a maximum of 1 AMA PRA Category 1 Credit(s)™. Physicians should claim only the credit commensurate with the extent of their participation in the activity.
All other clinicians completing this activity will be issued a certificate of participation. To participate in this journal CME activity: (1) review the learning objectives and author disclosures; (2) study the education content; (3) take the post-test with a 70% minimum passing score and complete the evaluation at www.medscape.org/journals/expertophth; (4) view/print certificate.
Release date: November 21, 2011; Expiration date: November 21, 2012
Learning objectives
Upon completion of this activity, participants will be able to:
• Distinguish the most important intervention to improve wound healing after an eye injury or surgery
• Compare cyanoacrylate and fibrin glues in ocular surgeries
• Evaluate novel tissue adhesives under investigation for use in ocular surgeries
• Analyze laser welding and laser soldering in ocular surgeries
Financial & competing interests disclosure
EDITOR
Elisa Manzotti
Editorial Director, Future Science Group, London, UK.
Disclosure:Elisa Manzotti has disclosed no relevant financial relationships.
CME AUTHOR
Charles P Vega, MD
Health Sciences Clinical Professor; Residency Director, Department of Family Medicine, University of California, Irvine, CA, USA.
Disclosure:Charles P Vega, MD, has disclosed no relevant financial relationships.
AUTHORS AND CREDENTIALS
Heyjin C Park
Tissue Engineering and Stem Cell Group, Singapore Eye Research Institute, Singapore.
Disclosure:Heyjin C. Park has disclosed no relevant financial relationships.
Ravi Champakalakshmi
Tissue Engineering and Stem Cell Group, Singapore Eye Research Institute, Singapore.
Disclosure:Ravi Champakalakshmi has disclosed no relevant financial relationships.
Pradeep P Panengad
Tissue Engineering and Stem Cell Group, Singapore Eye Research Institute; Department of Bioengineering, Faculty of Engineering, National University of Singapore, Singapore.
Disclosure:Pradeep P Panengad has disclosed no relevant financial relationships.
Michael Raghunath, MD, PhD
Department of Bioengineering, Faculty of Engineering, National University of Singapore; Department of Biochemistry, Yong Loo Lin School of Medicine, National University of Singapore, Singapore.
Disclosure:Michael Raghunath, MD, PhD, has disclosed no relevant financial relationships.
Jodhbir S Mehta, FRCS (Ed)
Tissue Engineering and Stem Cell Group, Singapore Eye Research Institute; Singapore National Eye Centre; Department of Ophthalmology, Yong Loo Lin School of Medicine, National University of Singapore; Department of Clinical Sciences, Duke-NUS Graduate Medical School, Singapore.
Disclosure:Jodhbir S Mehta, FRCS (Ed), has disclosed no relevant financial relationships.
(A) Full-thickness stellate corneal laceration. (B) Sealed with cyanoacrylate glue without sutures.
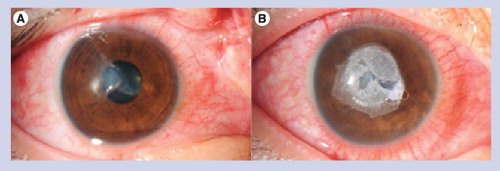
(A) Before operation; (B) 1 day after operation; (C) 1 week after operation; (D) 3 months after operation.
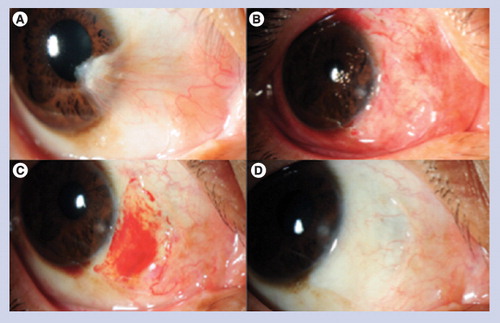
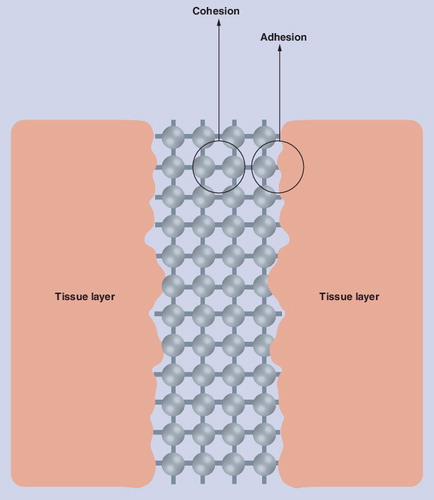
(A) Endogenous transglutaminase (TGase) incorporates biotinylated cadaverine in the presence of calcium at pH 7.4 into the tissue. Made visible using streptavidin-dichlorotriazinylaminofluorescein (green fluorescence), the epithelial activity can be attributed to TGase-1 and -3, while the stromal activity can be assigned to TGase-2. (B) Exogenously added TGase-2 crosslinks the tagged peptide into all the layers of the cornea with preference for the stroma, indicating the presence of abundant enzyme substrate sites for this enzyme here. (A´) and (B´): Calcium chelation with ethylene glycol tetra-acetic acid abolishes enzymatic activity.
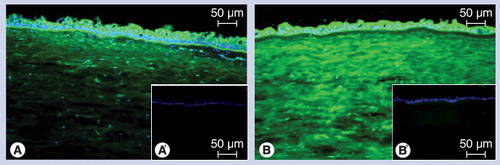
The application of tissue adhesives in ophthalmology started as early as the 19th century Citation[1]. The first surgical application of tissue adhesive was described in sutureless ocular surgery on rabbits using methyl-2-cyanoacrylate Citation[2]. Later, from the start of the 20th century, various other tissue adhesives were invented and used in ophthalmology. The drive towards the development of an adhesive comes from the complications associated with suturing. These include postoperative discomfort, prolonged healing time, risk of infection as well as prolongation of surgical time, and scarring.
Properties of ideal tissue adhesives include postoperative comfort, cost–effectiveness, rapid setting time and transparency, high tensile strength by creating a strong bridge between wound margins, easy application, biodegradable and biocompatible. Currently there are two main classes of tissue adhesives: synthetic (e.g., cyanoacrylate and acrylic-based polymers), and biological (e.g., fibrin glue, biodendrimers and riboflavin–fibrinogen compounds). Newly modified adhesives (e.g., chondroitin sulfate [CS] polymer and laser-activated serum albumin adhesive) have been described more recently in ophthalmology. Each of these adhesives has their own advantages and disadvantages. In this article, we shall discuss the uses and applications of the most commonly used tissue adhesives (i.e., cyanoacrylate and fibrin glue) and the recent developments with regard to newly described bioadhesives.
Cyanoacrylate & acrylic-based tissue adhesives
Characteristics
Cyanoacrylate glues are the most prominent and oldest glues to be used in ophthalmic surgeries. ‘Super glues’ or ‘Krazy glues’, as these synthetic glues are called, have many applications in various surgical procedures including wound repair and lacerations Citation[3], closure of pulmonary leaks in thoracic surgery Citation[4], and repair of nerves in neurosurgery Citation[4].
Cyanoacrylates are esters of cyanoacrylic acid, formed through the condensation of cyanoacetate with formaldehyde in the presence of a chemical catalyst Citation[5]. The polymerization of the glue on the surface of any tissue is achieved via a hydroxylation reaction where the cyanoacrylate monomers rapidly polymerize (10 s to 1 min) in the presence of water or weak bases present on the tissue surface Citation[5,6]. The toxicity of the glue depends on the length of alkyl side chains. Shorter side chains (e.g., methyl or ethyl cyanoacrylate) have greater toxic effects on the tissue, either directly or in the form of degraded products, whereas longer side-chain adhesives (e.g., octyl or butyl cyanoacrylate) have a slower degradation rate as well as lower toxicity Citation[6,7].
The bonding strength of various cyanoacrylate derivatives was reported by Refojo et al. The strength of the glue was tested in both dry and wet conditions. Bonding strength was found to be greater in dry conditions. They also found that tensile strength decreased with an increase in alkyl side chains (i.e., butyl-cyanoacrylate had greater bonding strength than octyl-cyanoacrylate) Citation[8,9]. The tensile strength of wounds created during cataract surgery were compared between self-sealing (sutureless) and cyanoacrylate adhesive closure at 4 and 28 days after surgery. It was reported that the tensile strength of the wound instilled with cyanoacrylate was greater than self-sealing sutureless wounds. Tensile strength of glue on the surface of the wound (after wound closure) was less than the one applied directly into the wound, indicating that cyanoacrylate acts as a barrier for natural wound-healing processes Citation[10]. The mechanical strength of octyl-cyanoacrylate in strabismus surgery of rabbits was studied by Ricci et al.Citation[11]. The bond strength of the octyl derivatives of the adhesive was found to be better than the normal cyanoacrylate adhesives, and the tensile strength doubled 45 days postoperation.
The bacteriostatic and bactericidal activities of cyanoacrylate derivatives have been reported since the 1980s. Eiferman et al. showed the bacteriostatic effects of butyl-2-cyanoacrylate against Gram-positive microorganisms (Staphylococcus aureus and Streptococcus pneumoniae) both in vitro and in vivoCitation[12]. Antibacterial analysis of ethyl–cyanoacrylate showed bacteriostatic and bactericidal nature against Gram-positive microorganisms (S. aureus, Streptococcus pyogenes and S. pneumoniae) and Gram-negative organisms (Escherichia coli and Enterococcus faecalis) Citation[13]. A comparative study of two most commonly used derivatives – N-butyl cyanoacrylate and methoxypropyl cyanoacrylate – showed bacteriostatic activity against S. aureus, S. pneumoniae and Mycobacterium chelonae, but the latter had a greater inhibitory effect than the former Citation[14]. It was also found that antibacterial effects were comparatively higher in polymerizing adhesive than the already set glue. Hence, the polymerization process is implicated to have a direct antibacterial reaction, and this effect may be harnessed to promote the healing of corneal ulcers caused by Gram-positive organisms Citation[15,16]. Prior application of cyanoacrylate adhesive was also shown to impede the effects of corneal stromal melting Citation[16,17].
Clinical uses
Cornea
Corneal perforations
Cyanoacrylate has been used for the treatment of corneal perforations and thinning Citation[18]. Small corneal perforations or impending perforations can be avoided by early application of cyanoacrylate glue combined with application of topical antibiotics or a bandage contact lens to avoid microbial infections. This has reduced the rate of enucleation (6 vs 19%) following perforation and also the need for conjunctival flap surgery or penetrating keratoplasty (PKP) Citation[6,19].
Sharma et al. compared the effectiveness of fibrin glue and N-cyanoacrylate for treating small corneal perforations of 3 mm diameter Citation[20]. Both adhesives resulted in effective closure of corneal perforations <3 mm in diameter. The investigators demonstrated that adhesive plug formation with cyanoacrylate was faster than that of fibrin glue. However, fibrin glue-assisted corneal perforation closure resulted in faster healing and less vascularization.
The combination of cyanoacrylate glue with a bandage contact lens and antibiotics can reduce the risk of infection, although corneal melting and reapplication of glue can be problematic in cases of large perforations Citation[21].
Sealing corneal cataract wounds
Cyanoacrylate adhesives have been used in small-incision cataract surgery and have been found to be a safe and effective alternative to sutures without induced astigmatism Citation[22]. Hermel used cyanoacrylate in combination with human fibrin glue in sealing cataract microsurgery and found that this combination was very effective in developing anatomical resistance within 20 days after surgery Citation[23].
In an experimental laboratory model of a human eye, cyanoacrylate has been shown to act as a barrier to extraocular fluids following corneal cataract incisions. When applied on the wound edges, 2-octyl-cyanoacrylate creates a strong barrier to the influx of India ink, thus confirming no variations in intraocular pressure (IOP) following external pressure Citation[24]. This model was further tested in vivo on patients after cataract surgery. The case study proved the safety and efficacy of 2-octyl-cyanoacrylate as a watertight sealant for wound closure after cataract incision Citation[25].
Glaucoma surgery & bleb leaks
A case report has demonstrated the use of cyanoacrylate for sealing leaking blebs postglaucoma surgery Citation[26,27]. It has also been reported to be used for sealing conjunctival button holing during glaucoma surgery Citation[28] and repair of conjunctival fistula during glaucoma drainage surgery Citation[29].
Retina
Retinal detachment surgery
Cyanoacrylate glue has been shown to seal retinal breaks in pediatric vitreoretinal surgery for retinal detachment. The sealing effect was found to be effective when applied either on the wound edges or when used as an adhesive plug for retinal breaks Citation[30]. Cyanoacrylate glue delivery was accurate even in the presence of silicone oil during vitreous surgery for retinal detachment Citation[31]. Attachment of polymethylmethacrylate loops or silicone band (scleral buckling) to sclera during retinal detachment surgery has been reported by Sternberg et al. and Ricci et al.Citation[32,33]. A novel experimental technique to treat retinal breaks was reported by Gilbert et al., where a patch comprising a substrate (i.e., lens capsule and synthetic membranes) and tissue adhesive were applied on the inner area surrounding the break. However, this led to cellular proliferation and toxicity when cultured with bovine retinal epithelial cells Citation[34].
Macular hole surgery
Transvitreal application of cyanoacrylate tissue glue directly onto the macular hole may reduce the risk of hole progression thus reducing the need for vitreous surgery through gas tamponade or macular buckling Citation[35].
Other ophthalmic applications
Harvesting of corneal limbal stem cell allografts for limbal stem cell deficiency has been aided with the use of cyanoacrylate tissue adhesive. This synthetic adhesive was found to provide great stability to the limbal tissue during dissection and harvesting; moreover, histological studies also indicated no trace of glue and cellular toxicity Citation[36].
Octyl-2-cyanoacrylate tissue adhesive has been used for the attachment of muscles to porous orbital implants and the tensile strength of the glued fixation was found to be similar to sutured attachments Citation[37]. Octyl-2 cyanoacrylate was found to have greater adhesive and tensile strength than conventional cyanoacrylate glue when tested in muscle reattachment surgery in patients having thin sclera. The tensile strength of the glue was found to increase four times within 45 days after the surgery Citation[11]. Cyanoacrylate has also been used in total socket reconstruction surgery to stabilize the mucous membrane graft Citation[38] and in eyelid repositioning surgery for patients suffering from epiphora Citation[39].
Application techniques
Standard application techniques for descemetoceles or impending perforations have been followed for several years. The quantity of cyanoacrylate used during surgery should be carefully selected since the glue expands in volume during polymerization and hence even a minute amount would be enough to seal small corneal perforations of 1–2 mm in size Citation[40]. Cyanoacrylate-based tissue adhesive can be applied directly through squeezable vials or with the help of an applicator, which can be a simple syringe attached to the vial Citation[41], 23-gauge catheter Citation[42] or plastic micropipette Citation[40,43]. The corneal patch technique is advantageous in that it provides a uniform surface Citation[6]. This procedure involves the preparation of tissue adhesive on a corneal plastic dressing or patch and direct attachment of this prepared patch onto the perforated site. Application of topical antibiotics before and after this procedure is needed to avoid infections. Polyethylene disk instead of plastic dressing can also be used as a patch for application of glue over the small perforation Citation[44].
Despite the ease of application and high bonding strength of cyanoacrylate glues, their high toxicity and elicitation of a strong foreign body response presents a need for the development of more biocompatible tissue adhesives.
Fibrin glue
Characteristics
Fibrin glue is the most commonly used clinical bioadhesive. Purified fibrin has been reported to treat parenchymal bleeding since the early 19th century and the combination of fibrin and thrombin has been used for skin graft adhesion Citation[45]. Since the 1980s, there have been various forms of fibrin glue available on the market. The sealer protein generally has two major components, fibrinogen and thrombin, two coagulating factors, aprotinin (fibrinolysis inhibitor) and calcium chloride. The setting time of the mixture is usually dependent on the thrombin concentration. A fast-setting mixture sets within 30 s and a slow-setting mixture sets within 1–2 min.
Fibrin glue is designed to mimic the last stage of blood coagulation. Factor XIIIa is a transglutaminase that catalyzes the final steps in the formation of a fibrin clot. The enzyme stabilizes the fibrin clot through crosslinking (CXL) of fibrin monomers to each other, as well as CXL of fibronectin and α2-plasmin inhibitor to fibrin Citation[46–48]. Fibrin glue clot formation starts with the activation of Factor XIII by thrombin. The activated Factor XIII then hydrolyzes prothrombin to thrombin. Thrombin converts fibrinogen into fibrin. Fibrin self-assembles into fibers to form a 3D matrix. Thrombin also activates Factor XIIIa (present in the fibrinogen component of the glue), which stabilizes the clot by CXL fibrin fibers as well as by inducing polymerization of the fibers in the presence of calcium ions. The basis of the adhesion is the presence of the Factor XIII enzyme, which causes the fibrin to crosslink and form a coagulum with great cohesive strength.
General disadvantages of fibrin glue include long preparation time for thrombin-induced activation of the enzyme, susceptibility to humidity and proteolytic degradation, and low adhesion strength. Since fibrin glue has been prepared from blood, there is a latent risk of viral transmission, although various strategies have been developed to reduce this risk.
Commercially available fibrin glue
Fibrin sealant has been available in Europe since 1978 and blood bank or laboratory-derived fibrin sealants have been used in the USA since the 1980s. However, it was not approved for commercial sale in the USA until 1998 due to cases of hepatitis transmission in early fibrinogen preparations. With increased care in donor selection, heat treatment of human fibrinogen, and the replacement of bovine thrombin by virus-inactivated human thrombin in the 1990s, the risk of virus transmission was decreased. Tisseel® (Baxter Immuno, Vienna, Austria) was the first fibrin sealant approved by the US FDA for use in the USA. Fibrin sealant is now FDA approved for use as a topical hemostat, sealant and adhesive Citation[49]. The first use of fibrinogen as an adhesive was demonstrated by Young and Medawar in 1940 for joining the cut ends of sciatic nerves in rabbit and dog models Citation[50]. Cronkite et al. used bovine thrombin mixed with fibrinogen in plasma for skin grafting Citation[51]. Rose, Dresdale and coworkers demonstrated the use of fresh–frozen plasma and bovine thrombin to create fibrin glue in 1985 Citation[52].
All fibrin sealants in use have two major ingredients, purified fibrinogen (a protein) and purified thrombin (an enzyme) derived from human or bovine blood. Many sealants have two additional ingredients, human blood Factor XIII and aprotinin, which is derived from cows’ lungs. Factor XIII is a compound that strengthens blood clots by promoting crosslinkage of fibrin strands. Aprotinin is a protein that inhibits the enzymes that break down blood clots.
There are currently four available FDA-approved commercial fibrin sealants distributed by US companies: Tisseel and Artiss (Baxter, CA, USA), Evicel™ (Johnson & Johnson, NJ, USA), Cryoseal® (Thermogenesis, CA, USA) and Vitagel™ (Orthovita, PA, USA). Tisseel and Evicel fibrin sealants are prepared from human pooled plasma. Cryoseal is prepared from individual units of plasma. Vitagel is prepared from individual units of plasma, bovine collagen, and bovine thrombin Citation[49].
In Europe, Tisseel/Tissucol™, Beriplast® (Aventis Behring) and Quixil (Johnson & Johnson Wound Management/Omrix Biopharmaceuticals) are a few of the sealants approved for use that utilize human fibrinogen and human thrombin. A comparison of Tisseel, Beriplast and Quixil has been evaluated by Tredree et al.Citation[53]. Tisseel and Beriplast both utilize aprotinin (fibrinolysis inhibitor) derived from bovine sources. Quixil on the other hand uses a synthetic fibrinolysis inhibitor (tranexamic acid) to eliminate the risk of immunological response. Bovine aprotinin is a serine protease inhibitor that also inhibits activated protein C that reduces the formation of thrombin, immune cell apoptosis and inflammation. It may inhibit other wound-healing proteases due to its nonspecific activity. In contrast, tranexamic acid is a specific plasmin/plasminogen inhibitor. Compared to tranexamic acid, bovine aprotinin is larger and diffuses at a slower rate. In addition, the clot formed with Tisseel/Tissucol becomes opaque upon formation, while the clot formed with Quixil remains transparent. While opacity gives physicians an indication of complete clot formation, transparency may be advantageous in situations when visualization of the wound is desired.
Another major difference between Quixil and other fibrin glues is in the purification method. While Tisseel and Beriplast use purified fibrinogen via a process patented by Schwartz et al.Citation[301], Quixil uses a method developed by Martinowitz and Bal to produce crosslinked fibrinogen–fibronectin multimers Citation[54]. A tissue adhesive of high bonding strength must have a large constituent of molecules (i.e., fibrinogen) capable of binding to collagen. Bar et al. found that only the fibronectin–fibrinogen ‘heteronectin copolymers’, copolymerized with Factor XIIIa to facilitate binding of fibrinogen to collagen Citation[55]. The first step to both the Schwartz et al. and Martinowitz et al. methods is cryoprecipitation. However, unlike the Martinowitz et al. method, Schwartz et al. uses a second precipitation technique to remove fibronectin and albumin. While this is effective in terms of purification, the second precipitation also removes much of the crosslinked fibrinogen–fibronectin that binds to collagen. On the other hand, the Martinowitz et al. method has been shown to increase the heteronectin copolymer to fibrinogen ratio. Bar et al. postulated that Martinowitz et al.’s purification process may increase binding of fibrinogen to collagen due to the higher concentration of the heteronectin that is left in the resulting fibrin glue.
Fibrin sealants cannot be treated alike due to differences in quality, component concentrations and source of fibrinogen, thrombin and fibrinolysis inhibitor components. In addition, indications for handling, storage, viral inactivation and removal procedures are different, affecting efficacy, preparation time, setting speed, safety and strength of the glues. Performance of the fibrin glue is dependent on the protein component, thrombin concentration and the stabilizing agent (fibrinolysis inhibitor) Citation[53]. The following sections briefly detail the most commonly used and approved commercially available fibrin glue products.
Tisseel/Tissucol fibrin sealant
Tisseel has been used in Europe for more than 25 years and has been used for over 9.5 million surgical procedures. The commercially available Tisseel (also sold under the name Tissucol in some regions) is manufactured by Baxter Immuno. It is FDA approved and is utilized in surgical procedures worldwide for hemostasis in cardiac, splenic trauma and colon anastomosis surgery. The kit contains fibrinogen, thrombin, aprotinin and CaCl2. The kit can be stored up to 6 months. Viral transmission risk is reduced by a combination of cryoprecipitation, adsorption, vapor heating and freeze–drying. Tisseel utilizes bovine aprotinin (Trasylol®, Bayer, CN, USA) to inhibit fibrinolysis. Aprotinin can elicit immunologic response and can increase the risk of renal failure and myocardial infarction Citation[56].
Evicel (Johnson & Johnson) fibrin sealant
Evicel is manufactured by Omrix Biopharmaceuticals (Ramat-Gan, Israel) and marketed by Johnson & Johnson. Evicel has been FDA approved for hemostasis in liver surgery. It does not use aprotinin or bovine derivatives. Viral disease transmission risk is reduced by cryoprecipitation, pasteurization, solvent detergent cleansing and nanofiltration. Unlike Tisseel, Evicel is plasminogen reduced and does not require an antifibrinolytic agent.
Vitagel (Orthovita) surgical hemostat
The Vitagel kit (Orthovita, Inc., PA, USA) is intended for use with plasma derived from the patient’s own blood and has been FDA approved for surgical procedures excluding neurosurgery and eye surgery. Vitagel provides a kit that includes a syringe containing a buffered solution of 20 mg/ml of bovine collagen and 300 U/ml bovine thrombin (THROMBIN-JMI®) and 40 mM calcium chloride Citation[57]. This syringe can be joined to another syringe containing autologous plasma. The extraction of fibrinogen-rich plasma is done with a device, CellPaker® Collection Device, that is not included in the Vitagel kit. The use of autologous derived fibrinogen eliminates risk of viral transmission. Bovine thrombin, however, poses a risk as it elicits elevated production of IgG antibody which interacts with human coagulation proteins leading to hemorrhaging and thromboembolism Citation[58]. Common adverse reactions associated with Vitagel are fever, pain, nausea and atelectasis Citation[401].
CryoSeal® FS system
CryoSeal (Thermogenesis, CA, USA) provides a system that enables simultaneous extraction of concentrated fibrinogen-rich cryoprecipitate and thrombin from autologous or pooled plasma Citation[59]. CryoSeal FS also includes a thrombin-processing device (TPD™) that extracts human thrombin from plasma or whole blood (7 ml thrombin from 11 ml of plasma/whole blood). Fibrinogen-rich cryoprecipitate is extracted within 60 min and thrombin extraction is complete within 25 min. The system also includes concentrated Factor VIII, Factor XIII, von Willebrand’s factor and fibronectin. Applicators come with interchangeable tips that allow the fibrin sealant to be sprayed or applied drop-wise onto target sites.
Ophthalmic applications
Fibrin glue was first used by Tidrick et al. in 1944 for skin graft fixation Citation[60]. Katzin first used it to attach corneal grafts in rabbit eyes in 1946 Citation[61]. Since then, fibrin glue has been extensively applied in research and clinical practice for a myriad of sutureless ophthalmic procedures.
Conjunctival surgery
The use of fibrin glue for conjunctival wound closure and transplantation has been found to be as effective as sutures, with the advantage of reduced operative time, postoperative discomfort and inflammation. The following sections review ophthalmic surgeries involving sutureless conjunctival surgery with fibrin glue.
Pterygium surgery
Pterygium is typically treated by surgical excision of the pterygium. However, the postoperative recurrence rate is high with simple excision and many methods have been developed to reduce this rate, including β-irradiation, application of mitomycin C, human amniotic membrane (HAM) transplantation, and autologous conjunctival grafting or limbal–conjunctival autografting Citation[62]. Conjunctival autografts have been demonstrated to yield the best results in lowering recurrence rate and in safety. Fibrin glue has been used frequently in attaching conjunctival autografts . Studies have demonstrated reduced operating time, postoperative discomfort and inflammation. Reduced recurrence rate has also been reported for conjunctival autografting in pterygium surgery with the use of fibrin glue compared with sutures. Koranyi et al. postulate that fibrin glue facilitates better adhesion of the graft with the underlying tissues, which may result in early graft vascularization and thus prevents recurrence Citation[63]. The success of autografts may also be affected by conjunctival inflammation. Sutures can cause additional trauma to the injury site and surrounding tissue, act as a nidus for infection and can usher in infectious agents along the suture tract Citation[64]. A study by Suzuki et al. found that silk and nylon sutures induced migration of Langerhans cells into the corneal epithelium, resulting in poor graft survival Citation[65].
A meta-analysis was performed by Pan et al. on seven pooled randomized control studies Citation[66–72] comparing fibrin glue and suture outcomes for limbal–conjunctival autografting following pterygium excision Citation[73]. A total of 342 participants and 366 eyes were encompassed in the seven studies. Operating time, complication rate and recurrence rate were statistically analyzed for significant differences between fibrin glue- and suture-treated data. Meta-analysis revealed statistically significant longer operating times for treatment with sutures than for fibrin glue. Fibrin glue was also found to be more effective in reducing the recurrence rate (odds ratio [OR]: 0.33; 95% CI: 0.15–0.71; p = 0.004) compared with suture. However, there were no significant differences in the complication rate between sutured and fibrin glued groups.
Studies have also demonstrated the efficacy of amniotic membrane (AM) graft fixation in conjunction with pterygium surgery as a suitable alternative to conjunctival autografting in cases of large conjunctival defects Citation[74]. Jain et al. used fibrin glue for attaching human AM grafts after primary pterygium excision Citation[75]. The study reported good results in 11 out of 12 patients in terms of successful adherence and recurrence at 1 year postoperation. Kheirkhah et al. did a comparison study of HAM with application of mitomycin C after pterygium (primary and recurrent) excision Citation[76]. However, the sample size in both of these studies was too small to provide statistically significant evidence that fibrin glue attachment of HAM in conjunction with pterygium is a superior alternative over sutures.
Strabismus surgery
Zauberman and Hemo were the first to use fibrin sealant (noncommercial) in ten cases of strabismus in 1988 Citation[77]. Biedner and Rosenthal did a comparison study between vicryl sutures and fibrin sealant on each eye of six patients after extraocular muscle surgery in 1996 Citation[78]. Erbagci and Bekir used commercially available fibrin sealant, Beriplast, on 48 conjunctival wounds of 25 patients. No cases appeared to exhibit allergic reactions, chemosis, infections, subconjunctival hemorrhage or inflammation. However, three wounds in two cases of fibrin sealant treated eyes required the reinforcement of sutures post-surgery due to a retraction of the wounds of greater than 2 mm. In total, 73% of the fibrin sealant-closed wounds remained closed after 1 day postoperation. Dadeya and Kamlesh also compared discomfort of vicryl sutures and fibrin sealant for conjunctival wound closure. No cases in which fibrin sealant was used experienced postoperative discomfort while discomfort was reported for 42% of the eyes closed with vicryl sutures Citation[79].
Fibrin sealant is particularly recommended over sutures for conjunctival wound closure for infants and children, where suture removal may be impossible. The main advantage of fibrin sealant is the lack of postoperative discomfort. Mohan et al. reported success in using fibrin sealant for conjunctival wound closure in children Citation[80]. However, one disadvantage of fibrin glue for strabismus surgery is that the bonding strength of fibrin glue is insufficient against the contractive strength of muscles in recession, demonstrated by Tonelli et al. in rabbit models Citation[81]. Krzizok also noted that the use of fibrin sealant in children may be more difficult due to the tightness of their Tenon’s capsule Citation[82].
Corneal surgery
One major use of fibrin glue is in plugging ulcer-induced perforations Citation[19]. Lagouette et al. used fibrin sealant to close perforated corneal ulcers and reported a 93% success rate Citation[83]. Sharma et al. discussed the discrepancy in outcomes between chronic and acute perforations utilizing tissue adhesives. In their study, the success rate for perforation closure was 85.7% for acute and 60% for chronic perforations treated with fibrin glue Citation[20].
Repair of corneal ulcer perforations has also been treated by human amniotic membrane transplantation (AMT). Duchesne et al. reported success in the treatment of three patients who had central corneal perforations (2 mm diameter) with fibrin glue-assisted AMT Citation[84]. Anterior chamber depth was restored with sodium hyaluronate viscoelastic material and removal of necrotic tissue. Human fibrin glue (Tisseel) was then used to fill and plug the perforation, and then sealed with AM. No sutures were required in any of the three patients. Complications included ulcer recurrence but complete epithelialization (14 or 17 days postoperation) was reported. Application of prophylactic topical ofloxacin antibiotic may have played a significant role in lack of recurrence.
Corneal defects that are 3 mm or larger have been successfully treated utilizing fibrin glue-assisted corneal patch or AM grafting. Gupta et al. demonstrated fibrin glue sealing (Realiseal; Reliance Life Sciences, Mumbai, India) of larger paracentral corneal melts with a full-thickness corneal patch graft in two patients Citation[85]. When corneal grafts are not available as in emergency cases, AMT has been demonstrated to be a suitable alternative therapy for penetrating corneal ulcers. Chen et al. utilized AMT to treat acute fungal keratitis Citation[86]. Kim and Park explored fibrin glue closure in conjunction with AMT for the treatment of large corneal perforations (>2 mm in diameter) in ten patients Citation[87]. They used fibrin glue to produce a five-to-seven-layered AM (augmented AM). The augmented AM was then secured to the perforation with 10–0 nylon sutures.
AMT
Sutureless AMT with fibrin glue has also been demonstrated in numerous other surgical procedures. Kheirkhah and coinvestigators utilized fibrin glue-assisted AMT in patients with partial limbal cell deficiency and conjunctivochalasis. They were able to circumvent limbal epithelial stem cell transplantation, demonstrating the efficacy of fibrin glue-assisted AMT in successfully inducing corneal epithelial growth, leading to complete coverage of the AM Citation[88]. In the conjunctivochalasis study, they reported effective attachment of the AM to bare sclera and development of a smooth conjunctival surface Citation[89]. However, development of focal conjunctival inflammation in four out of 25 eyes and pyogenic granuloma in one eye was presented at postoperative follow-up. Duchesne et al. demonstrated the use of AMT in three patients with 2 mm diameter central corneal perforations Citation[84]. Hick et al. used AMT on 33 eyes from 32 patients and reported a success rate of 92.9% of fibrin glue affixed AM Citation[90].
Sekiyama et al. performed sutureless AMT with fibrin glue for ocular reconstruction of rabbit eyes Citation[91]. They prepared a freeze–dried (FD) AM that was coated with fibrin glue (Tisseel). A low concentration of the fibrin glue mixture (10 µl/cm2) was applied to the entire FD-AM chorionic surface and left for 30 min. Afterwards, the fibrin glue-coated FD-AM was dried under low pressure and then vacuum packed. This method significantly reduced the amount of fibrin glue needed to secure FD AM when it was applied to tissue instead of the AM. Thus, inflammatory reactions from fibrinogen were minimized. The biocompatibility of the glue coated FD-AM was tested by transplanting it onto the bare sclera. The FD-AM was reported to adhere to the bare sclera immediately. The fibrinogen biodegraded within 2 weeks and the FD-AM remained for at least 12 weeks. Complete epithelialization was achieved in 2 weeks and normal cell differentiation was verified.
Lamellar keratoplasty
Rostron et al. was able to perform fibrin glue attachment of lenticules in half the time it took to suture lenticules Citation[92]. Kim et al. performed lamellar keratoplasties on 24 rabbit eyes with fibrin glue and found that 71% of the grafts remained in place Citation[93]. Minimal inflammatory response was reported with no inflammatory cells at the lenticule–host interface; the adhesive was also resorbed by three weeks. Rosenthal et al. also tested fibrin glue on lamellar keratoplasty autotransplants on rabbit eyes. They reported 50% success with fibrin glue alone, with adhesion being maintained for 4–6 days Citation[94]. No inflammation was observed and there was no hindrance with epithelialization. Hashemi and Dadgostar tested automated lamellar keratoplasty with fibrin glue on ten eyes of nine patients Citation[95]. The fibrin glue (Tisseel) was applied onto the corneal bed and a lenticule from donor cornea. No inflammation or rejection was reported, and the donor cornea and donor–recipient interface remained clear. Other cases for securing lamellar grafts with fibrin glue alone have been reported Citation[96,97]. Deep lamellar keratoplasty was achieved by Narendran et al. using both fibrin glue and sutures. They recommended that fibrin glue be used only in cases where donor buttons fit well in the recipient stromal bed Citation[98]. Oberg et al. addressed the challenge of applying the thick and viscous Tisseel glue into a uniform thin layer Citation[99]. They modified the application method to facilitate the attachment of donor graft to recipient tissue in posterior lamellar keratoplasty in rabbit eyes. They dusted dry cryoprecitated fibrinogen onto the lamellar surfaces of 30 enucleated donor corneal buttons and then injected thrombin solution into a submersion well on top of the recipient corneal bed before placing the donor button onto the bed. No fibrinolysis inhibitor solution (aprotinin) was used and there was no reported reduction in adhesive strength. The tensile strength was only slightly lower than that measured of complete Tisseel fibrin glue attachment.
Por et al. demonstrated the use of fibrin glue as an effective sealant in a method that allows deep anterior lamellar keratoplasty in patients with large corneal perforations (2–4 mm in maximal dimension) Citation[100]. The study involved three patients with pre-existing or intraoperative corneal macroperforations. Before keratoplasty, Tisseel fibrin sealant was delivered into the anterior chamber with a cannula or needle from the limbus, placing the tip under the corneal perforation site to create an underlying fibrin plug. Deep anterior lamellar keratoplasty was then performed, and the fibrin sealant plugs successfully maintained the fully reformed anterior chambers. Cases in this study did not present postoperative complications and successfully prevented a persistent double anterior chamber.
Penetrating keratoplasty
Bahar et al. performed PKP combining fibrin glue with eight or 16 sutures for wound closure in top hat PKP on enucleated human corneas Citation[101]. Wound-bursting pressures for fibrin plus suture-closed top hat PKP (144.6 mmHg with 6–8 sutures and >158 mmHg with 16 sutures) were compared with those of traditional PKPs closed with sutures (25.2 mmHg with eight sutures, 59.1 mmHg with 16 sutures), and top hat PKPs closed with sutures (57.6 mmHg with eight sutures, 103.8 mmHg with 16 sutures). The study demonstrated the efficacy of top hat PKP wound closure with sutures reinforced with fibrin glue.
Limbal cell transplantation
Fibrin glue has also been used to secure keratolimbal allografts to treat limbal stem cell deficiency in patients. Allografts have been obtained from conjunctival limbal allografts Citation[102], corneoscleral rims of cadaveric eyes Citation[103] and limbo–amnion composite grafts Citation[104]. These studies reported no graft rejection or dislocation associated with attachment with fibrin glue alone. Pfister et al. used fibrin glue to attach corneal epithelial stem cell transplants to the limbal niche Citation[105]. Higa et al. also used fibrin glue to create transplantable corneal epithelial sheets Citation[106].
Epithelial ingrowth & other laser-assisted in situ keratomileusis complications
The major use of fibrin glue is as a sealant. As part of this function, fibrin glue has been used to prevent epithelial ingrowth by functioning as a mechanical barrier. Postoperative complications of laser-assisted in situ keratomileusis (LASIK) surgery include flap dislocation, epithelial ingrowth, interface fluid syndrome and flap necrosis. Treatment includes mechanical debridement and flap reattachment. Successful cases of flap attachment has been reported with no recurrence of epithelial ingrowth or fluid accumulation in the interface Citation[107–110]. Azar et al. treated recurrent epithelial ingrowth after LASIK by attaching an annular AM graft with fibrin glue Citation[111]. The advantage of fibrin glue is that it will degrade over time. One disadvantage of utilizing fibrin glue for flap reattachment is the resulting opacity of the glue that makes it difficult to determine inflammatory response.
Temporary basement membrane
Bonatti proposed the use of fibrin as a temporary basal membrane on nonperforated corneas for photorefractive keratectomy and non-infected autoimmune corneal ulcers Citation[112]. The advantage of the fibrin temporary basal membrane is in its ability to facilitate epithelial migration and inhibition of fibrinolysis by the plasmin inhibitor tranexamic acid, which prevents recurrence of the ulcers. Neither in vitro nor in vivo testing has been performed to demonstrate the potential efficacy of this proposal yet.
Glaucoma surgery
Trabeculectomy is the most common glaucoma surgery performed and fibrin glue has been utilized for wound closure after trabeculectomy Citation[113]. Fibrin glue has been effectively applied to treat bleb dysfunction/leaking Citation[114–118] and securing conjunctival flaps Citation[119].
Bahar et al. performed an animal study on eight rabbit eyes to test the efficacy of fibrin glue (Quixil) for tissue attachment after conjunctival peritomy, scleral flap, and penetrating trabeculectomy Citation[120]. They investigated retention of fibrin adhesive, capillary congestion, inflammation, scar formation, IOP, conjunctival chemosis, anterior chamber reaction, and presence of filtering bleb and wound leakage. An acute inflammatory reaction was observed for up to 14 days, which progressed into chronic inflammation, collagen deposition and scar formation. One eye exhibited wound dehiscence. Investigators concluded fibrin glue attached cases did not exhibit additional adverse effects compared with sutured cases.
Fibrin glue has also been used to secure glaucoma drainage devices Citation[121]. Kahook et al. used fibrin glue to close the conjunctiva, secure a pericardium patch graft, as well as to secure the glaucoma drainage tube to the sclera Citation[122]. Postoperative conjunctival inflammation was found to be lower in cases treated with fibrin glue than with sutures and the time of surgery was significantly shorter. Valimaki used fibrin glue to seal peritubular leakage by applying it over the sclera flaps Citation[123].
Lens surgery
Fibrin glue has been used in numerous studies for sealing cataract wounds Citation[124–129]. Fibrin glue has also been used to assist in posterior chamber intraocular lens implantation in eyes with capsular support deficiency with good visual acuity outcome and no postoperative complications Citation[130,131]. Buschman et al. also reported success in using fibrin glue for closing anterior lens capsule wounds Citation[132].
Vitreoretinal surgery
Pooled fibrinogen from autologous plasma combined with bovine thrombin was applied over macular holes and large retinal tears by Coleman et al. The glue was injected subretinally under retinal detachments in rabbit eyes Citation[133]. Postoperative evaluation showed chorioretinal adhesions at the injection site. Coleman also created retinal holes in rabbit eyes with the tip of a needle and a fibrin glue mixture was injected intravitreally over the retinal hole. The autologous fibrin glue mixture was also used on the macular holes and retinal tears of patients. They found the retinal tear edges sealed earlier than that of tears which were not treated with fibrin glue. Adhesion between retina and choroid at the injection site was observed and the chorioretinal adhesion showed evidence of a fibrin clot 1–2 days after injection. Inflammatory reactions and proliferative tendencies were not observed. One eye with subretinally injected adhesive exhibited anterior chamber and vitreous flare after operation. Seven out of ten eyes showed slightly elevated scars at the injection site. Fibrin glue has also been applied for assisting implantation of keratoprosthetic devices during vitreoretinal surgeries by Uhlig et al.Citation[134].
Application techniques
The two components of fibrin glue, fibrinogen and thrombin, can either be applied simultaneously or sequentially, depending on the physician’s preference. When simultaneous application is preferred, both the components are loaded into two syringes with tips forming a common port (e.g., Duploject® syringe). When injected, the two components are mixed in equal volumes at the point of delivery. The setting time is dependent on the concentration of the thrombin component Citation[135]. The thrombin converts the fibrinogen to fibrin by enzymatic action at a rate determined by the concentration of thrombin. The more concentrated thrombin solution, thrombin 500, produces a fibrin clot in approximately 10 s and the more dilute thrombin solution, thrombin 4, results in a clot in approximately 60 s after glue application to the surgical field.
For sequential application, thrombin is first applied on to the area of interest, followed by a thin layer of fibrinogen. In 1–2 min coagulation begins, and by 2–3 min polymerization is complete. Alternatively, when apposition is required between opposing surfaces, thrombin solution may be applied to one and fibrinogen to the other surface. In all of these cases, prior to application of the glue, the surgical field must be dried meticulously. After application, the tissue is pressed gently over the glue for 3 min for firm adhesion. At the end of the procedure, a pad and a bandage are applied after application of antibiotic drops.
Spray applicators comprising of gas-pressurized devices (e.g., the Tisseel EasySpray system) have also been developed for fibrin glue application. Mehta and coinvestigators compared the adhesive strength of fibrin glue applied drop-wise versus via a spray applicator for securing conjunctival grafts onto rabbit cornea. There was no significant difference in adhesive strength between either application system, proving the efficacy of the spray delivery system for ophthalmic surgery Citation[136].
Safety of fibrin glue
The greatest hazard of fibrin glue is the risk of viral transmission in formulations comprising fibrinogen extracted from multiple donors. Risks of viral transmissions are well documented Citation[137]. Hino et al. reported three cases of parvovirus B19 transmission from the use of commercial fibrin sealants Citation[138]. This demonstrated that dry heat viral inactivation was not an effective means to eradicate the parvovirus. Methods have been developed to minimize this risk. Solvent or detergent treatment can inactivate most but not all viruses. Additional means of reducing viral transmission risk are a combination of γ-radiation, cryoprecipitation, adsorption, vapor heating, pasteurization and nanofiltration. Further insurance can be attained by testing viral markers from donors for 6 months to ensure sources are virus free. However, the safest preparation is obtained from the patient’s own blood.
The biological activity of fibrinogen itself also poses a risk. Fibrinogen has been determined to be involved in the acute inflammatory response phase, activating neutrophil markers that cause an increase in phagocytosis, cellular cytotoxicity and apoptosis delay Citation[139]. Therefore, reduced amounts of fibrinogen for surgical procedures are recommended to prevent additional inflammatory reactions.
Fibrin glue formulations that use thrombin derived from animal sources carry a risk of adverse immunological response. Cases in which patients developed antibodies against bovine thrombin leading to strong immunological response have been reported Citation[140,141].
Despite the risks of fibrin glue, it is extensively used in ophthalmic procedures because it has been shown to cause minimal inflammation in most cases, it is able to set in a moist environment, it causes significantly less postoperative discomfort compared with sutures and cyanoacrylate glues, and is antibacterial, biodegradable and nontoxic. The main disadvantages of fibrin glue are low adhesion strength, risk of viral transmission and high cost.
Investigatory adhesives
The drawbacks of sutures and approved commercially available, adhesives, cyanoacrylate and fibrin glue for ophthalmic wound sealing and healing have spurred the efforts to develop novel adhesives to address these issues. Risks of the existing options include astigmatic scarring, tissue necrosis, cytotoxicity, viral-disease transmission and low bonding strength. There is a strong need for novel ophthalmic tissue adhesives that eliminate these issues. The following text is a review of investigations of novel bioadhesives that have not yet been approved for clinical use but show promise as sutureless alternatives to existing ophthalmic wound treatment options.
Biodendrimers
There is a lot of interest in developing biodendrimers composed of biocompatible and biodegradable materials for medical and clinical applications. This interest is due to the numerous peripheral functional group dendrimers that can be modified and tailored chemically and structurally for a multitude of broad applications such as drug delivery, imaging, ligation chemistry and CXL. The latter application has been harnessed to produce novel biocompatible adhesives endowed with chemical-, thermal- and photo-activated CXL capabilities. The added advantages of mechanical and degradation rate tunability make dendrimers attractive candidates for ophthalmic adhesives. The following studies focus on dendritic bioadhesives used in ocular surgical procedures.
Photo-activated dendritic polymers
Carnahan, Grinstaff and coworkers have developed and explored numerous first- to fourth-generation dendrimers composed of polyethylene glycol, succinic acid and glycerol of numerous moieties for use as photocrosslinkable corneal adhesives Citation[142]. They have also demonstrated the use of the dendritic bioadhesive ([G1]-PGLSA-MA)2-PEG in numerous ocular applications including central corneal lacerations Citation[143], clear corneal cataract incisions Citation[144,145], flaps from LASIK surgery Citation[146], scleral incisions Citation[147] and 8-mm penetrating keratoplasties Citation[148]. These in vitro studies tested the capability of this sealant to withstand high IOP (mean leakage pressure of 78.7 mmHg in lacerated cornea) Citation[149].
Berdahl et al. tested the ([G1]-PGLSA-MA)2-PEG biodendrimer polymer adhesive in an in vivo chicken model to evaluate clinical and histological healing of corneal lacerations. A 4.1 mm keratome was used to make central full-thickness linear corneal wounds in white leghorn chicken eyes. Crosslinking of this biodendrimer polymer is activated by an argon ion laser. The ([G1]-PGLSA-MA)2-PEG is mixed with 0.5% ethyl eosin in 1-vinyl-2-pyrrolidinone (photoinitiator) and triethylaluminum (co-catalyst). Application was directly applied to the wound site with a 30-gauge needle attached to a 1 ml syringe. The polymer was then irradiated (λmax= 514 nm; 200 mW) with a handheld probe 2.5 cm from the adhesive. Complete gelation is indicated by a change in color from pink to clear. Duration of irradiation was not reported, but adhesive repair time was reported to be 1 min compared with 5 min with sutures. Corneal lacerations were also sealed with sutures for comparison. Slitlamp evaluation of wound integrity, corneal clarity, Seidel positivity and anterior chamber inflammation was performed at 6 h, and at 1, 2, 3, 4, 5, 6, 7, 14, 21 and 28 days following surgery. Immediately after closure, Seidel testing showed leakage on one of the adhesive-treated eyes and none on sutured eyes. After 1 day, all eyes were shown to be Seidel negative and a similar amount of inflammation was present in both adhesive and suture models. All corneas treated with the adhesive appeared clear while most of the sutured corneas exhibited scarring on day 28. Histological evaluation was also performed to observe inflammation and extent of epithelial, stromal and endothelial healing at 1, 3, 7 and 28 days after surgery. Degradation of the adhesion was observed within 14 days. Adhesive-treated wounds were found to have clean wound edges, exhibited minimal stromal inflammation and better wound edge approximation than that of suture-treated models. However, more hyperplastic epithelium plugging the wound anteriorly, and fibrinous/proteinaceous exudates plugging the wound posteriorly, was observed in adhesive-treated wounds. Although adhesive-treated wounds had wider scars than the sutured group, multiple prominent scars were observed at suture sites. Sutured wounds also exhibited a more irregular anterior surface and more stromal inflammation, supporting the slit-lamp observations.
Advantages of the photo-activated biodendritic adhesive include control of polymerization for easy application of the glue, faster repair time, transparency, minimal inflammation and elasticity. The wide scar formation is probably due to the limit of application of the adhesive at the surface of the wound. This may be addressed by developing an adhesive that is suitable for application at not only the surface but also epithelial, stromal and endothelial wound edges. However, disadvantages include the added time it takes for photopolymerization and the additional equipment required.
Chemical CXL (two-component) dendritic polymers
Grinstaff, Carnahan and coworkers also developed self-sealing dendritic polymers that polymerize upon mixing of two CXL components Citation[149]. One such adhesive developed consists of lysine-based peptide dendrons with N-terminal cysteine conjugates that react with poly-(ethylene glycol dialdehyde). This formulation was successfully applied for the repair of 3 mm clear corneal incisions Citation[145] and sealing of LASIK flap edges Citation[150] in enucleated eyes.
A novel chemically derived bioadhesive (CDB) composed of aldehyded dextrans and ε-poly(L-lysine) solution was developed by Araki et al. to address the reported failure of fibrin glue to prevent air leakage from pulmonary parenchymal defects during surgery and recurrent postoperative leakage Citation[151]. CDB was explored as a stronger alternative sealant. The novel glue forms a biodegradable hydrogel adhesive upon the mixture of two aqueous solutions: aldehyded dextran and; ε-poly(L-lysine) plus acetic anhydride. The mechanism of gelation of the glue is based on a Schiff base formation. The glue is applied through a mini-dual syringe (Plas-Pak Industries Inc., CN, USA). The CDB is reported to have fourfold the bonding strength than that of a commercial fibrin glue (Bolheal™) Citation[152].
To test the efficacy of the glue as a sealant, the mean bursting pressure was measured after application to 30 × 30 mm pleuroparenchymal defects in beagle dogs by Araki and coinvestigators. Fibrin glue was used as a comparison, and the burst pressures were 38.4 ± 4.6 cm H2O (p = 0.02) for the new glue and 32.1 ± 4.5 cm H2O for fibrin glue (p = 0.02). Degradation rate was slower compared with that of fibrin glue with biodegradation within 3 months, and complete disappearance by 6 months. Histological studies showed complete fibrous tissue coverage at 4 weeks, and minimal inflammatory reaction after 3 months.
The same CDB glue was also tested in various ocular surgical procedures. In 2008 Takaoka et al. applied the CDB formulation with slightly lower concentrations of aldehyded dextran and ε-poly(L-lysine) to sutureless transplantation of AM onto the ocular surface of rabbit eyes Citation[153]. An in vitro biocompatibility assay demonstrated low cytotoxicity for both the aldehyded dextran and ε-poly(L-lysine) components. CDB biocompatibility with the ocular surface was also tested by injecting 0.1 ml of the adhesive into the subconjunctival space of rabbit eyes. Hematoxylin staining was reported to show no inflammation or scarring and immunohistochemical labeling demonstrated positive expression of keratin 4, keratin 13 and mucin type 5AC typically expressed in conjunctival epithelial cells, hence suggesting no toxicity. Transplantation of 4 × 4 cm denuded AM onto bare sclera was performed with CDB and sutures for comparison. AM fixation onto the sclera was reported to occur within 3 min. Biodegradation was observed within 4 weeks. Epithelialization time was evaluated for sclera with and without an AM graft. While sclera without an AM failed to epithelialize within 2 weeks, surfaces with AM transplantation showed complete coverage at 2 weeks. Biocompatibility of the CDB in vivo 12 weeks after transplantation was evaluated through histological examination. No significant difference was reported between inflammatory cells beneath the AM between CDB and sutured models. Morphology and desmosomal cell junctions of the conjunctival epithelial cells were characteristic of normal cells under electron microscopy evaluation, and displayed good hemidesmosomal contacts with the adhesive.
Takaoka et al. also demonstrated the use of CDB glue in sutureless keratoplasty in 2009 Citation[154]. Automated lamellar therapeutic keratoplasty was performed on two rabbit eyes whereby corneal buttons were exchanged between two eyes of each rabbit. The grafts were secured with either nylon sutures or CDB. CDB was applied directly to the entire corneal bed upon which the grafts were placed. The corneal buttons were found to affix after 3 min postplacement onto the CDB-covered bed and remained fixed without loss or dislocation until epithelialization. No significant difference was observed in corneal clarity, epithelialization, and inflammatory response between sutureless and suture models. No epithelial cell growth into the interface was observed. Histological evaluation showed well-stratified and differentiated epithelium and immunohistochemistry showed normal expression of cytokeratins (3, 4, 12 and 13) and cell junction-related proteins (ZO-1, desmoplakin, collagen VII, and integrin α6). Morphology and desmosomal cell junctions of the corneal epithelial cells were characteristic of normal cells under electron microscopy evaluation, and displayed good hemidesmosomal contacts with the adhesive. In summary, CDB has the advantages of a relatively strong bonding strength compared with fibrin glue, easy handling owing to gradual gelation, no need for additional equipment, biocompatibility, and minimal influence on surrounding cells and tissues. With these attributes, CDB has great potential as a superior alternative to sutures, cyanoacrylate and fibrin glue for ocular surgical applications.
Chemical CXL linear polymer adhesives
CS–aldehyde adhesive
CS is a naturally occurring linear polysaccharide found in the extracellular matrix of tissues and thus postulated to pose low risk of immune response. CS has also been demonstrated to have wound-healing benefits Citation[155]. Reyes et al. modified CS with aldehyde groups and developed a self-polymerizing two-component glue Citation[156]. The two components are CS–aldehyde and polyvinyl alcohol that crosslink upon mixture via a Schiff base reaction. Leakage was not observed in any of the glued models with the highest IOP possible with their experimental setup. The mean maximum IOP attained in glued models was 101.4 ± 3.2 mm Hg. Leakage was achieved with the one suture model at a mean pressure of 26.4 ± 6.0 mm Hg, and with the 3 suture models at a mean pressure of 44.3 ± 8.3 mm Hg The study thus demonstrated the superior sealing strength of the CS–aldehyde adhesive over sutures. Although this study shows the potential of CS–aldehyde as a suture alternative for corneal wound sealing, additional in vitro and in vivo biocompatibility studies need to be demonstrated for further development of the adhesive for clinical application.
Gelatin resorcinol
Gelatin–resorcinol–aldehyde is a two-component glue comprised of gelatin and resorcinol and aldehydes (typically formaldehyde, glutaraldehyde, or both). The combination of the two components spurs polymerization via nucleophilic attack upon bonding of aldehydes to activated positions of resorcinol. Although studies have demonstrated that its bonding strength to tissues is higher than that of fibrin glue Citation[157], the major deterrence to using gelatin–resorcinol–formaldehyde in ophthalmic surgery is the high toxicity of formaldehyde.
Although extensive documentation and use of gelatin resorcinol-based adhesive has been reported for cardiovascular surgery, it has only been explored in one study in ophthalmology. Tonelli et al. reported a higher inflammatory response of gelatin-resorcinol-formaldehyde-glutaraldehyde (Colagel, Cirumedica SA, São Paulo, Brazil) in rabbit eyes than either cyanoacrylate or fibrin glue Citation[81]. Concerns about formaldehyde’s mutagenicity and carcinogenicity led Ennker and coinvestigators to replace the formaldehyde component of the glue and combine gelatin instead with less toxic aldehydes such as glutaraldehyde and glyoxal (gelatine–dialdehyde), pentanedial and ethanedial (GR-DIAL), and other CXL agents Citation[158]. The gelatine–dialdehyde was tested on aortic tissue of pigs Citation[159] and the GR-DIAL was tested on lung incisions in rabbits Citation[160], as well as on lung parenchyma defects of rats Citation[161]. These modified gelatin-based adhesives were reported to be resorbed in vivo without interfering with the healing process, and was shown to have no carcinogenic, mutagenic or toxic effects.
Albumin–glutaraldehyde adhesive
The most common formulation of serum albumin and glutaraldehyde is a commercially available product, BioGlue (Cryoline Inc., GA, USA). It was approved by the FDA in 1999 as an adjunct to sutures for aortic dissections. Once the two components mix, glutaraldehyde causes lysine molecules of albumin and extracellular matrix proteins and cell surfaces to form covalent bonds. Bonding strength reaches its maximum at 2 min. In vivo and ex vivo tensile and shear strength studies have been performed to show excellent bonding strength to tissues Citation[162]. BioGlue has been used extensively to aid hemostasis in cardiovascular surgery Citation[163]. It is mainly utilized as a sealant. In a single case reported by Engel et al. BioGlue was applied in addition to sutures to a jagged scleral rupture to effectively stem fluid leakage through the wound Citation[164].
Although BioGlue has been approved by the FDA, it comes with warning statements that BioGlue may cause serious nerve injury Citation[165], coagulation necrosis and mineralization Citation[402]. Use of BioGlue in ophthalmic surgeries has only been minimal possibly due to the safety concerns with glutaraldehyde. Unreacted, volatile glutaraldehyde is a risk factor for those involved in the preparation and application of BioGlue. Since serum albumin is derived from bovine products, allergic reaction, risk of spongiform encephalopathy and hepatitis is a safety concern. Furthermore, warnings have been raised against its use in pediatric patients, and inflammatory responses were reported Citation[165].
Photocrosslinkable adhesives
Bovine serum albumin-based bioadhesives
Khadem et al. has developed a laser-activated biological tissue glue comprising covalent albumin-chlorin e6 (ce6) conjugate (bovine serum albumin [BSA]–ce6), and a mixture of BSA and Janus Green. The application of this BSA-based adhesive has been demonstrated for the treatment of corneal incisions Citation[166] and perforating corneal wounds Citation[167]. Histological results showed greater epithelial filling, less stromal edema and fewer inflammatory cells over 7 weeks postoperation. These results imply that this novel adhesive is not only effective and biocompatible but also enhances corneal wound healing.
Bloom et al. has developed a novel scaffold-enhanced, light-activated bioadhesive system for ophthalmic surgery application. A poly(L-lactic-co-glycolic acid) porous scaffold was mixed with BSA and indocyanine green (ICG). Crosslinking is activated with a diode laser at 808 nm wavelength. This adhesive system was demonstrated as a suture alternative for strabismus surgery. The adhesive was applied to extraocular muscle-to-muscle, sclera-to-sclera, and extraocular rectus muscle-to-sclera and its bonding strength was evaluated with tensile strength testing. The mean tensile strength of the adhesive muscle-to-muscle was found to be 81% (433 ± 70 g) of that of the native muscle’s tensile strength (494 ± 73 g). Sclera-to-sclera mean strength was determined to be 295 ± 38 g and extraocular muscle-to-sclera adhesions were measured at a mean of 309 ± 37 g. A comparison of the tensile strength of this adhesive to that of sutures, cyanoacrylate glue, and fibrin glue, would further validate the potential of this novel adhesive system as a sutureless alternative. In vitro and in vivo biocompatibility studies have yet to be evaluated Citation[168,169].
Riboflavin-based bioadhesive
Riboflavin photosensitizer is used to increase UVA absorption in the cornea to induce collagen CXL. CXL has been demonstrated to increase the biomechanical stiffness of the cornea by increasing intrafibrillar crosslinks. CXL has also been found to flatten the cornea and reduce spherical aberration. Upon UVA irradiation, riboflavin generates singlet oxygen and superoxide anion radicals that are speculated to photoxidize certain amino acids that may contribute to collagen aggregation. This CXL effect has been found to effectively treat keratoconus, post-LASIK ectasia, infectious keratitis and bullous keratopathy Citation[170]. Wollensak et al. utilized riboflavin/UVA-assisted CXL to stop the progression of keratoconus in patients Citation[171]. The study reported success in halting keratoconus progression in all 23 eyes treated as well as a 70% regression, a decrease in maximal keratometry (K) readings (mean decrease of 2.01 D) and a decrease refractive error (mean decrease of 1.14 D) over 23 months follow-up, and an improvement in visual acuity in 65% of eyes. Corneal and lens transparency, endothelial cell density, and IOP readings were reported to be unaffected by the CXL treatment. Raiskup-Wolf et al. performed a large cohort study on 480 keratoconus-afflicted patient eyes with a follow-up of 6 years reporting continued improvement in maximum K for up to 3 years after CXL treatment Citation[172]. Recently, CXL has also been used in the treatment of keratectasia Citation[173]. Hafezi et al. demonstrated the efficacy of riboflavin/UVA-assisted CXL in ten patients with keratectasia after LASIK with halted progression in all patients and regression in 50% of the patients Citation[174].
Safety risks associated with riboflavin/UVA treatment include endothelial cytotoxicity and infection. However, these risks have been minimized with optimization of treatment settings and methods. The current parameters used prevent damage to corneal endothelium and is below the threshold level for endothelial cytotoxicity for human corneas thicker than 400 µm Citation[175]. Epithelial debridement is typically performed to allow penetration of riboflavin into the corneal stroma. However, this can cause postoperative discomfort and infectious keratitis. Alternative methods to deliver riboflavin have been tested, including using femtosecond laser-created pockets or through intact epithelium Citation[176]. Chan et al. demonstrated the effectiveness of riboflavin/UVA induced CXL on patients whose eyes had their epithelium left intact. The eyes were soaked in riboflavin (0.1% riboflavin-5-phosphate and dextran) for 5 min and penetration reached half of the entire corneal depth Citation[177]. The study reported significant success in reducing the severity of keratoconus.
Hyaluronic acid-based photocatalytic glue
Miki et al. developed a photocrosslinkable adhesive composed of hyaluronic acid modified with methacrylate groups (HA-MA) with ethyl eosin as photo-initiator and triethanolamine as co-catalyst Citation[178]. The solution was applied on corneal lacerations in an in vivo study using rabbits. Upon irradiation with a low-intensity argon laser beam (diffuse beam: 30 s; emission maximum: 514 nm; 200 mW), the viscous polymer solution turns into a flexible transparent hydrophilic hydrogel. The hydrogel effectively sealed the wounds and minimal inflammation was reported. Histochemical studies demonstrated that the sealant did not hinder the proliferation of stromal cells and extracellular matrix regeneration at the application site. Wound edges formed adhesion 4–7 days after surgery. A degradation in vitro study was performed on the HA-MA sealant with hyaluronidase (HAase), which is present in the eye. Dextran entrapped in the HA-MA was dyed blue as an indicator of release, and reported no release of dextran for more than 30 days. The adhesion strength of HA-MA to rabbit cornea was 2.2 g/cm2 before argon ion laser irradiation, which was found sufficient to adhere the HA-MA to the cornea for subsequent photo-CXL.
Laser welding & soldering
Laser welding
Laser welding utilizes photochemical or thermal reactions to induce tissue fusion. Laser welding of tissues has been proposed as an alternative to sutures and staples for wound closures in many surgical fields. Successful welding has demonstrated significant advantages over sutures, including faster operation time, immediate closure of wounds and resistance to high leakage pressures, minimal foreign body and inflammatory response, and faster healing rate Citation[179,180]. In the context of corneal surgery, laser welding reduces secondary trauma, inflammation and scarring, which can lead to astigmatism and opacity. The first use of the laser for tissue welding was for the treatment of retinal detachment Citation[181]. Studies have demonstrated that thermal energy absorbance causes unraveling of the collagen triple helix, which allows collagen to form new noncovalent bonds between the polypeptide strands. Various methods for laser-induced tissue bonding have been developed that can be categorized into the following: laser welding using water as an endogenous chromophore; exogenous chromophore-assisted laser welding and laser soldering. These techniques require the use of different lasers and parameters such as wavelengths, laser power and angles of irradiation delivery.
First attempts at laser welding in the 1970s were unsuccessful when low concentrations of ICG were applied even at very high laser powers, resulting in no welding and strong thermal damage to the tissues. Timberlake et al. applied a solution of 0.1% ICG to partial thickness corneal incisions on human cadaveric eyes and attempted to weld the wounds with laser irradiances of 40–400 W/cm2Citation[182]. They attributed the lack of success to shrinkage of the incision, lack of compression of the cut during heating, or insufficient ICG or irradiation exposure. In later studies however, other groups were able to achieve success of tissue welding with much higher concentrations of applied ICG. Advanced developments of lasers facilitated the achievements starting in the 1990s.
Many different types of lasers have been tested to induce tissue welding. Infrared laser wavelengths, such as those emitted by CO2 (10.6 µm), Er:YAG (2.9 µm), Ho:YAG (2.1 µm) and diode lasers around 1.9 µm use water as the main chromophore because of its the high optical absorption in these spectral regions. However, the use of water yields poor results due to the difficulty in controlling dosimetry, temperature rise, localization to the wound site, and high irradiation power required to effectively induce photothermal activation of collagen. Since laser irradiation is absorbed by water, penetration of the laser is shallow and thus is not able to weld the whole thickness of wounds. The use of chromophores, however, allow the effect of the laser irradiation to deeply penetrate. By directing the beam at a tilted angle in respect to tissue surface, the whole thickness of the tissue can be welded Citation[183].
The greatest challenge of the laser-induced tissue fusion technique is the precise temperature control required. Insufficient heat results in weak bonds and failure to fuse tissue. Heat overdose results in the desiccation of the collagen fibers, irreversible denaturation of other proteins and tissue shrinkage in surrounding areas. The heat generation is not only dependent on the parameters of the laser beam (i.e., spot size, power, beam activation time, wavelength), but it is also highly dependent on the density and CXL of the tissue. The temperature required to denature collagen is between 60 and 80°C, depending on the type of collagen and degree of CXL.
Measures have been taken to increase control and precision of heat generated in the tissue. Noguera et al. set up a thermocouple device to monitor the temperature at the tissue interface. Other groups have used pulsed delivery of laser energy as opposed to continuous wave laser delivery, and it has been suggested that pulsed delivery minimizes collateral thermal damage Citation[184]. Other groups have used photo-enhancing dyes and laser wavelengths specific to the chromophore’s peak absorption to localize heat at applied areas. These chromophores also allow the fusion of tissues at a much lower irradiation power, minimizing heat damage. One common criteria for exogenous chromophores used for laser welding is an optical absorbance that is transparent to tissue. Chromophores effectively localize thermal effects and prevent collateral heat damage to surrounding tissues.
The most common chromophore used for ophthalmic procedures has been ICG. Its peak optical absorption is at wavelength 800–810 nm Citation[185], at which the corneal stroma is transparent, and the extensive documentation of its toxicity makes ICG a popular chromophore for tissue laser welding. The chromophore allows selective optical absorption of laser radiation, which enhances photothermal activation of the stromal collagen so that much lower laser power is required than that of direct tissue irradiation without chromophores. Near infrared (NIR) lasers such as Nd:YAG typically operate at approximately 800 nm and are utilized in conjunction with exogenous chromophores. At NIR, absorption by tissue is minimal and chromophores applied on wound site allow selective and more controllable welding. Ideally, laser irradiation should be confined as it can cause collagen shrinkage, denaturation, photocoagulation and tissue necrosis Citation[185,186].
Matteini and coworkers were able to successfully weld corneal incisions in porcine eyes with the application of a much higher concentration of ICG (12% w/w). In addition, they were able to achieve successful tissue welding at a very low laser power (12–16 W/cm2). ICG-tained corneal cuts were irradiated with an 810 nm laser at low power, which induces a localized heating of the stroma in the range of 55–66°C Citation[187]. Evaluation of the microscopic structural modifications induced by laser welding was performed with light and electron microscopy. Semi-thin histological sections of laser-welded stroma were stained with toluidine blue and viewed under light microscopy. Light microscopy showed collagen structures crossing the wound edges. No coagulation of the collagen was observed. Ultra-thin sections of ICG-treated and untreated samples were embedded in epoxy resin for transmission electron microscopy evaluation. The laser-welded samples showed variance in collagen orientation when compared with control samples. This effect appeared to be localized to the ICG-stained area and no heat damage was reported at the low power range. The study by Matteini et al. shows immediate sealing of the incisions via realignment and interweaving of endogenous collagen fibrils Citation[187].
Menabuoni et al. evaluated the same ICG formulation in clinical studies of diode (805 nm) laser-welded corneal wounds. Cataract surgery was performed on 20 patients with a preoperative visual acuity worse than 20 out of 200 Citation[188]. Ten patients had phacoemulsification surgery and ten had extracapsular cataract extraction (ECCE). For this study, the patients were split into four groups: group 1: phacoemulsification cataract surgery with a 3.2-mm incision; group 2: phacoemulsification cataract surgery with a 5.5 mm incision; group 3: ECCE with a 12-mm incision and three interrupted 10–0 nylon sutures; and group 4: ECCE with a 12-mm incision and one interrupted 10–0 nylon suture. The study demonstrated minimization of postoperative astigmatism. The chromophore ICG was diluted in water (10% w/w) and was applied to the inside of the corneal incisions with a 30-gauge anterior chamber cannula. After 2 min, the excess was washed out of the wound. If the walls of the incision were not homogenously stained with ICG, the ICG solution was reapplied. An aluminum gallium arsenide diode laser attached to a fiber optic device with an irradiation wavelength of 810 nm was used to induce tissue welding. Set at a power density of 12 W/cm2, laser irradiation was applied at an angle with respect to the corneal surface to allow the laser beam to be homogenous and to have deep penetration into the stroma. The handheld optical fiber was kept in continuous movement. Complete welding was indicated by a slight whitening of stained walls associated with the bleaching of ICG. Welding times for phacoemulsification incisions ranged from 25–45 s, and for ECCE approximately 80 s.
Ophthalmological examination included recording of manifest reaction, inflammation, Seidel testing, manual K, astigmatism via vector analysis, and endothelial cell counts. All eyes showed no significant inflammation. Endothelial cell count was comparable to that of outcomes with sutured procedures. The chromophore was absorbed by tissues within 7 days after surgery. Astigmatism and Seidel testing was carried out on days 1, 3, 7, 30 and 90. Astigmatism variations were not significant except in one patient in group 3 (ECCE with three stitches). A comparison with astigmatism in this study was not compared with that of cases with the traditional suture procedure. However, the Seidel was negative in all cases at each postoperative visit. No heat damage was reported to adjacent tissue, but no data was given to support this.
In a retrospective study, Menabuoni et al. performed an in vivo study on 20 rabbit eyes to evaluate the effect of laser welding on the healing process Citation[189]. The rabbits underwent sutureless laser-assisted and conventional PKP. No shrinkage of the stroma adjacent to the wound walls was observed. There was minimal homogenization of the collagen. On postoperative day 2, complete epithelialization was reported. Fibroblast activation was not seen. On postoperative day 7, the wound was repaired by fibroblast proliferation and no scar shrinkage around the wound was detected. Wound edges were almost indiscernible except at the Descemet’s membrane. By contrast, wound edges were very evident in eyes subject to conventional PKP on postoperative day 7. Conventional PKP samples also showed an increased collagen homogenization and fibroblast activation in comparison to laser-assisted PKP samples. This histological study indicates that diode laser-assisted corneal welding accelerates the wound-healing process in comparison to suture procedures.
Laser soldering
Compared to tissue welding, laser soldering can provide greater bonding strength and less collateral damage. Laser soldering is a bonding technique that uses biological mixture of proteins or polymers that coagulate upon heating. Heat denatures proteins and produces noncovalent bonds between the solder and links sterically with collagen fibers. Protein solders are composed of in vivo absorbable material. Soldering essentially mimics the physiological process of clotting. The solder is applied to wound edges and used in conjunction with laser irradiation to weld the wound. Laser wavelengths are matched to the absorbance of the solder. Upon irradiation, the solder and chromophore mixture activates clotting and effectively seals the wound. The clot is typically composed of a material able to be resorbed in vivo. Solders developed have included various proteins such as BSA Citation[190], fibrinogen Citation[191] and purified collagen Citation[192]. The first albumin solder was successfully developed and used to seal tissue against fluid leakage in urethral reconstruction Citation[193].
There are several advantages of utilizing solders over direct tissue welding depending on the application. Solders can direct thermal absorption not only at the edges of a wound, but can also be applied beyond the edges to form adherent sleeves, which can significantly enhance the overall bonding strength Citation[194]. The solder can also be used as an effective sealant or hemostatic agent. Furthermore, solders can incorporate pharmacological agents such as anti-inflammatory agents, growth factors, antibiotics and anticoagulants to enhance the healing process. Eaton et al. tested dye-enhanced laser-activated biocompatible tissue glues composed of human albumin, hyaluronic acid, CS and ICG. Two solder mixtures were tested:
A. 1 cc of 25% human albumin, 1 cc of 1% hyaluronic acid, and 10 mg of ICG;
B. 1 cc of 25% human albumin, 1 cc of 3% hyaluronic acid/4% CS, and 10 mg of ICG.
They performed 7–10 mm scleral tunnel incisions and applied a thin layer of each solder to the inside walls of the wounds with a 27-gauge needle. A diode laser at 808 nm wavelength with a continuous power output of 300–450 mW, 2-mm spot size, power density 7–11 W/cm2 and exposure time of 30–60 s. The wound-bursting pressure with mixture A yielded a mean pressure of 69.9 ± 17.1 (p < 0.01), and the mean pressure with mixture B was 42.9 ± 9.4 (p < 0.05).
Further reduction of thermal damage from laser soldering is achieved with the incorporation of chromophores into the solder mixture. Noguera et al. used a telopeptide-free type I collagen preparation (atelocollagen) from porcine or bovine dermis, modified with glutaric anhydride Citation[195]. The modified collagen was concentrated then FD. The chemical modification (degree of 30%) and collagen concentration (55–60% weight/volume) were prepared in such a way that the product exhibited thermal transition at the 40–45°C temperature range, as well as having sufficient carboxyl groups for CXL to tissue. The laser diode used had a wavelength of 1.45 µm, and was set at a 1W output power and temperature of 40°C. The temperature of the tissue/solder interface was monitored and modulated with a thermocouple thermometer to maintain the temperature at 40°C. An ex vivo study comparing nylon sutures and laser soldering was performed on 20 enucleated rabbit eyes. A 3.0-mm wide uniplanar non-self-sealing peripheral oblique corneal wound was made parallel to the iris with a 45° angle keratome in ten rabbit eyes. A straight keratome, 2.85-mm wide, rounded-tip crescent knife was used to create a central perpendicular corneal wound in the other ten eyes. Solder was inserted along and in the wound to cover the incision in the 3 mm wide uniplanar non-self-sealing peripheral oblique corneal wound, while a patch of solder was placed on the 2.85 mm wide central perpendicular corneal wound to completely cover the incision. The diode laser was applied to the solder for 5–7 s and then allowed to cool and cure for 5 min. Evaluation of the wound stability was performed by maximum leakage pressure. Mean maximum IOP of globes treated with laser soldering was 125.15 mmHg (SD: 9.85). Mean maximum IOP of globes with ten nylon sutures was 33.44 mmHg (SD: 9.38). This ex vivo study shows the potential of this collagen-based laser soldering technique to be a good alternative to sutures. However, performance of the solder in vivo needs to be demonstrated.
Gold nanoparticles as NIR chromophores for assisting laser welding
Recently, research groups have started using gold nanoparticles as NIR chromophores for assisting laser welding. Gobin et al. were the first investigators to include gold nanoparticles in solder filling to assist with laser activated bonding of tissue. NIR-resonant gold nanoshells were mixed into an albumin comprised of solder and applied muscular and cutaneous wounds Citation[195,196]. Ratto et al. prepared gold nanorods with an aspect ratio of 4:1 and diameters of 10–15 nm Citation[197]. At this aspect ratio, gold nanorods exhibit a sharp peak absorbance at a wavelength around 810 nm. Suspensions of the gold nanorods, were concentrated to approximately 10 nM by centrifugation and redispersed into aqueous solutions with a low collagen content (10%), used as bridging molecules. Gold nanorod-assisted laser welding was tested on the attachment of an anterior capsule to the anterior capsule bags of enucleated porcine eyes. The excised anterior capsule was dipped into the solution of colloidal gold nanorods, and after the excess was washed off, placed onto the recipient capsule to completely cover the rhexis. The eye lens was then irradiated by contiguous laser spots emitted by a 300 micron core fiber at a wavelength of 810 nm and fluences of 100–140 J/cm2. Mechanical load-resistance tests were performed with laser-welded anterior lens capsule tissues. The ultimate tensile strength of the biopolymeric patch (n = 10) was 2.28 ± 0.18 MPa, while the ultimate tensile strength of intact porcine anterior lens capsule bag was found to be 2.15 ± 0.14 MPa.
At the wound edges where gold nanorods were applied, vertical shrinkage (53 ± 4% of the original thickness) of the anterior lens was observed. It was determined that the photothermal effects extended by 200–220 µm from the center point of irradiation. In tissue areas without gold nanorods, no shrinkage or adverse effects to thermal damage was detected and Ratto et al. confirmed that the range of energies applied was insufficient to produce any detectable effect in lens capsules with no gold nanorods. This was expected as the anterior lens capsule is transparent to 800 nm wavelength irradiation.
Rossi and coinvestigators incorporated NIR resonant gold nanorods (GNRs) into chitosan patches for closing severed anterior lens capsule bags Citation[198]. GNRs generate localized plasmon resonances when irradiated at the NR wavelength that produce a strong photothermal effect. Chitosan was chosen for its biodegradability, biocompatibility, antimicrobial and wound healing attributes, which are well documented. GNR-embedded chitosan were fashioned into flexible and easy-to-handle films and tested on enucleated porcine eyes on which capsulorhexis was performed. The lens was removed and the patch was put onto the capsule bag and welded with a diode laser (810 nm; 200 µm core diameter optical fiber). They demonstrated immediate welding of the capsular tissue with high bonding strength. Owing to the elasticity and thinness of the capsular bag, conventional suturing is not possible. This study reports that laser soldering has been performed successfully on 37 patients at the time of writing and may prove a good contender for procedures such as these where suturing is not an option. Mechanical strength of the GNR-loaded chitosan film was reported to be comparable to that of the lens capsule bag. Adhesion was performed with minimal heat damage with single laser pulses delivered at 200 J/cm2 per pulse.
Gold nanoparticles may offer advantages over photoenhancing dyes such as ICG. Gold nanoshells and nanorods theoretically have a photoenhancing effect of orders of magnitude higher than that of ICG Citation[199]. Steric hindrance of nanorods and larger diameters of spherical nanoparticles can reduce diffusion when localization is desired. Gold nanoparticles can also be easily functionalized for specific targeting. Furthermore, gold is stable under physiological conditions and high laser irradiance whereas ICG is hydrolytically sensitive and susceptible to photobleaching. The future of gold nanoparticles for clinical use will depend on the outcome of future cytotoxicity studies and preclinical in vivo results in experimental animals.
Microstructural effect of laser welding on collagen
The laser-induced alteration of the collagen substructure at the microstructural level was analyzed by Schober et al.Citation[200]. They studied the effect of the irradiation from a Nd:YAG laser operating at a wavelength of 1.319 µm on collagen in welded tissues. Laser parameters were set to 12 W and 0.1 s exposure and applied to lesions in carotid arteries of adult albino rats. Light and electron microscopy showed that the legions were bridged. Transmission electron microscopy showed that the collagen fibrils in the welded area had lost their characteristic periodicity, had increased diameters, and were split into its fibrillar substructures. In addition, the collagen fibrils interdigitated with each other in contrast to fibrils in control samples that were segregated. While previous studies utilizing 1.06 µm wavelength lasers reported carbonaceous deposits under the same experimental conditions, this study demonstrated the potential of laser welding without deposit production and foreign body reaction with appropriate laser parameters.
Timberlake et al. investigated the effect of laser heating of collagenous tissue and fibrils in human cornea Citation[201]. They determined that heating corneal samples to 63°C or higher resulted in bulk tissue shrinkage. Transmission electron microscopy analysis showed collagen fibrils were expanded, no longer segregated from adjacent fibers and were split into their fibrillar substructures in samples that showed tissue shrinkage. Below 63°C, collagen fibrils appeared unaltered. Thermal shrinkage and swelling of collagen fibers is a result of the uncoiling of the collagen triple helix Citation[202,203].
The success of laser tissue welding or soldering depends on a high level of precision with regard to temperature, concentration of chromophores, and the handling of the components involved in solders. Ideally, lasers generate heat that cause unraveling of collagen fibril ends, which re-entwine upon cooling that then allows them to recombine to form noncovalent bonds. However, too little heat results in weak bonds and too much heat generation desiccates the fibers.
It is imperative that laser parameters are within safe limits. These parameters vary upon the chromophores used, on the solder (if one is applied), the concentration of the chromophore applied, the tissue geometry and the application. Although far-infrared laser light using water as an endogenous chromophore is considered to be safe, this method is limited to those applications that do not require penetration depth beyond the wound surface. On the other hand, deep penetration of NIR diode lasers (805 nm) may cause damage to other ocular tissues if operated at a high laser output power and dwelling time. The use of a high concentration of exogenous chromophores minimizes collateral damage and lowers the required power and exposure time needed for effective welding.
The form of solders used can affect the ease of handling and thus the effectiveness of laser soldering. Liquid or semi-solid solders can be diluted during surgery, resulting in decreased mechanical strength. On the other hand, stronger solders are often brittle and stiff. The use of semi-solid or solid-form solders may also present difficulties in handling. Strips can move during application requiring repositioning and strips may also shrink when exposed to the laser energy. Liquid tissue glues such as albumin and fibrinogen are difficult to prevent from running off wound margins, and applied areas need to be free of moisture to prevent dilution of the glue and leakage into unwanted areas such as the anterior chamber. Insufficient drying of the wound surface, as well as glue applied too thickly, renders tissue soldering ineffective. The incorporation of pseudoplastic (characterized by low viscosity at high shear rates and its high viscosity at low shear rates) materials, such as hyaluronic acid into laser solders are proposed to improve handling difficulties. Ideally, this would allow the formulation to be delivered through gauge needles, as well as stay in place on applied areas before welding.
Tissue transglutaminase glue
The transglutaminases are a family of enzymes that catalyze an R-glutaminyl-peptide, amine-γ-glutamyl transferase reaction between proteins. The resulting γ-glutamyl-ε-lysine isopeptide bond facilitates the assembly of crosslinked supramolecular structures Citation[204]. Eight different transglutaminase genes have been characterized in mammals thus far, and each gene specializes in the CXL of proteins in different biological processes. Only six enzymes have been isolated and characterized at the protein level Citation[205]. The function of plasma Factor XIII transglutaminase in the formation of insoluble fibrin clots has been well documented and the use of the enzyme as a CXL agent in adhesives for numerous medical applications is applied worldwide. Tissue transglutaminase (TGase)-2, on the other hand, is implicated to have a more ubiquitous role and more studies are needed to fully characterize the functions, localization and target substrates of this thrombin-independent enzyme. However, documentation so far has already determined the capability of TGase-2 to crosslink numerous tissue substrates far beyond the capability of Factor XIII. Thus there is interest in TGase-2 as an alternative enzyme-based adhesive with bonding strength potentially superseding that of fibrin glue.
Bonding effectiveness of an adhesive is determined by the combination of adhesion and cohesion strength. Cohesion is defined as the attraction of constituents with the adhesive that holds the adhesive material together. Adhesion strength is defined as the bonding strength of one material to another (i.e., bioadhesive to tissue). Both adhesion and cohesion strength increases with greater degree of chemical bonds between the two materials.
An ideal adhesive is one that is capable of generating the strongest interactions in both adhesion and cohesion zones. This means it must not only be capable of CXL its internal components, but also capable of CXL adhesive to tissue (i.e., adhesion; ). This criterion can only be met by the tissue adhesive with an enzyme capable of CXL a significant number of extracellular matrix (ECM) substrates.
Although fibrin glue produces a sticky layer of fibrin that adheres to the tissue surface within a few minutes, it is held primarily by weak interactions. Furthermore, the fibrin adhesive is susceptible to proteolytic digestion by plasmin and inflammatory cells. Jürgensen et al. have also compared the force-versus-time plots of fibrin glue and tissue TGase glue of adhesion of cartilage to cartilage, which supports the implication that adhesion to tissue with fibrin glue is primarily of weak interactions Citation[206].
Plasma TGase has been shown to crosslink a limited number of ECM substrate proteins including fibrin, fibronectin, laminin and von Willebrand factor. In addition to fibrin and fibronectin, tissue TGase has also been found to crosslink collagen III, V and VII Citation[207], collagen XI, osteonectin Citation[208] and osteopontin Citation[8]. Localization of TGases has also been explored in ocular tissues Citation[209–211].
In light of the wide extent of target ECM substrates, tissue TGase has great potential as a basis for a strong, durable tissue adhesive. Although testing of TGase-2 adhesive has not been tested in in vivo models yet, preliminary in vitro investigations have demonstrated its potential as a strong and durable tissue adhesive. Jürgensen et al. was the first to apply a tissue TGase-based glue for the treatment of lesions in articular cartilage in 1997 Citation[206]. To test the bonding strength of tissue TGase derived from guinea pig liver at the cartilage–cartilage interface, the enzyme was applied to full-thickness cartilage from adult bovine shoulder joints cut into cylinders. The tissue TGase enzyme was applied at various concentrations (0.25–2.75 mg/ml) to the freshly cut surface of one cylinder, and calcium chloride solution to the surface of the other cylinder. After application of an 80-g weight for a set time at 37°C, a transverse force was applied to the upper cylinder until displacement from the lower fixed cylinder occurred. Results demonstrated the bonding strength was linearly and positively dependent on the concentration of TGase-2 enzyme applied and the time of incubation. Application of 2.75 mg/ml concentration of TGase-2 after 10 min incubation was 0.43 N per 12 mm2. With a 1 mg/ml concentration of enzyme, the adhesive strength reached a value of 0.61 N per 12 mm2 with 30 min incubation. Relative humidity (30–100%) was found to have negligible effect on adhesive strength.
In the same study, Jürgensen et al. also conducted a comparison of TGase 2 with Tissucol Citation[206]. Its adhesive strength was shown to exhibit the same linear and positive dependence on incubation time on the articular cartilage surfaces. Unlike the tissue TGase adhesion, the adhesive strength showed significant decrease with increasing relative humidity <80%. The adhesive strength of TGase-2 was reported to be 62% greater than that of Tissucol at the maximum concentration of TGase-2. However, it was not reported whether the adhesive strength of Tissucol was compared with that of TGase-2 at an equivalent concentration or activity of the Factor XIII enzyme.
Furthermore, a comparison of shear force-versus-time plots indicated difference in interactions at the cartilage–cartilage interface between the two adhesives. While Tissucol showed a slow and variable decrease in force post-failure, the force curve for tissue TGase exhibited a sharp decrease. This indicates that Tissucol produces both covalent and noncovalent interactions with the cartilage interface, while tissue TGase produces predominantly covalent bonds.
TGase-2-based glue for ocular applications has not been demonstrated yet. However, the potential for the enzyme to produce molecular bonds with an extensive number of extracellular matrix structures in ocular tissue has been investigated. Raghunath et al. showed the distribution of endogenous TGase in the cornea, conjunctiva, sclera, and iris of the human eye Citation[211]. Immunolocalization of TGase-2 demonstrated its predominant presence over other TGases (-1, -3 and Factor XIIIa). TGase-2 was detected in connective tissue fibers of the ciliary stroma and endothelia within the ciliary body. TGase-2 was also expressed extracellularly in fibers of the ciliary body stroma. TGase-2 was also detected in the iris on the endothelium and singe cells in the iris stroma. Zonule fibrils were also determined to possess abundant substrate sites for commercially available purified guinea pig TGase-2 although endogenous activity of the enzyme was not able to be detected through immunohistochemistry Citation[209].
Panengad et al. were the first to propse a TGase-2 based biological glue for the treatment of ocular surgical wounds. Immunohistochemistry demonstrated an abundance of TGase-2 substrates distributed throughout the cornea tissue layers Citation[212]. Future studies testing ex vivo models of burst pressure and in vivo biocompatibility testing of the recombinant human TGase-2-based biological tissue glue will be pursued.
Expert commentary
Tissue adhesives are becoming more common in surgical wound closure as an alternative or adjunct to sutures especially in ophthalmology. Although currently approved adhesives have been shown to be effective for many ophthalmic procedures, there is still a need for a tissue adhesive that has both high bonding strength and biocompatibility. This is clearly indicated by the growing number of studies applying novel tissue adhesives in both ophthalmology and thoracic treatments. Preliminary studies of novel adhesives and bonding techniques demonstrate their potential to fill this need, but in many cases further extended studies are required for approval from both scientists and clinicians.
According to most studies covered here, immediate sealing and adhesion of injured tissue is indicated as the most effective and significant factor for rapid wound healing and reduced recurrence rate. Thus, highest priority should be put on developing adhesives with high a bonding strength and rapid sealing techniques. Furthermore, with increasing popularity of sutureless treatment by both clinicians and patients, there is a great need for improved standardization of preparation, bonding strength measurement protocol, and application techniques for both existing and emerging adhesives in the market.
Five-year view
Numerous efforts are underway to find better alternatives for sutures, cyanoacrylates and fibrin glue. The drawbacks of sutures and the great need for better tissue adhesive alternatives will probably spur the emergence of many novel bioadhesives into the market in the near future. Many of these novel adhesives show potential to address the risks and drawbacks of existing options which are critical particularly in ocular procedures. The number of different ophthalmic procedures treated by fibrin glue is becoming more commonplace and with the emergence of better tissue adhesives, sutureless ocular wound treatment may well become the standard within the next 5 years. The acceptance of these developing adhesives by clinicians, however, will depend largely on the results of large long-term cohort studies.
Key issues
• Properties of ideal tissue adhesives include: postoperative comfort, cost–effectiveness, rapid setting time and transparency, high tensile strength by creating a strong bridge between wound margins, easy application, biodegradability and biocompatibility.
• Despite the ease of application and high bonding strength of cyanoacrylate glues, their high toxicity and elicitation of strong foreign body responses present a need for the development of more biocompatible tissue adhesives.
• The main disadvantages of fibrin glue are low adhesion strength, risk of viral transmission and high cost. Despite these drawbacks, fibrin glue is extensively used in ophthalmic procedures because it has been shown to cause minimal inflammation in most cases, is able to set in moist environments, causes significantly less postoperative discomfort compared with sutures and cyanoacrylate glues, and is antibacterial, biodegradable and nontoxic.
• In many studies, immediate sealing and adhesion of injured tissue is indicated as the most effective and significant factor for rapid wound healing and reduced recurrence rate.
• There is a lot of interest in developing biodendrimers composed of biocompatible and biodegradable materials due to numerous modifiable peripheral functional groups, which can be tailored chemically and structurally for a multitude of broad applications such as drug delivery, imaging agents, ligation chemistry and crosslinking. The added advantages of mechanical and degradation rate tunability make dendrimers attractive candidates for ophthalmic adhesives.
• Advantages of the photoactivated biodendritic adhesive include control of polymerization for easy application of the glue, faster repair time, transparency, minimal inflammation and elasticity.
• Two-component polymer adhesives such as chemically derived bioadhesive have the advantages of relatively strong bonding strength compared with fibrin glue, easy handling due to controllable gelation time, minimal equipment, biocompatibility, and low influence on surrounding cells and tissues.
• Biological adhesives comprising of proteins and enzymes carry the risk of immunological response and disease transmission. Human recombinant enzymes and proteins are now being developed and provide the means to eliminate these risks.
• A tissue adhesive with high bonding strength must simultaneously exhibit both good cohesion and adhesion. Good adhesion to tissue requires covalent binding to a large number of extracellular matrix substrates.
References
- Coover H Jr, Joyner FB, Shearer NH Jr, Wicker TH Jr. Chemistry and performance of cyanoacrylate adhesives. SPE Tech. Papers5, 92 (1959).
- Ellis RA, Levin AM. Experimental sutureless ocular surgery. Am. J. Ophthalmol.55, 733 (1963).
- Applebaum JS, Zalut T, Applebaum D. The use of tissue adhesion for traumatic laceration repair in the emergency department. Ann. Emerg. Med.22, 1190–1192 (1993).
- Pineros-Fernandez A, Rodeheaver PF, Rodeheaver GT. Octyl-2-cyanoacrylate for repair of peripheral nerve. Ann. Plast. Surg.55, 185–195 (2005).
- Kilpikari J, Lapinsuo M, Tormala P, Patiala H, Rokkanen P. Bonding strength of alkyl-2-cyanoacrylates to bone in vitro. J. Biomed. Mater. Res.20, 1095–1102 (1986).
- Vote BJT, Fraco EMJ. Cyanoacrylate glue for corneal perforations: a description of a surgical technique and a review of the literature. Clin. Exp. Opthal.28, 437–442 (2000).
- Leggat PA, Smith DR, Kedjarune U. Surgical applications of cyanoacrylate adhesives: a review of toxicity. ANZ J. Surg.77, 209–213 (2007).
- Refojo MF, Dohlman CH, Ahmad B, Caroll JM, Allen JC. Evaluation of adhesives for corneal surgery. Arch. Ophthalmol.80, 645–656 (1968).
- Refojo MF, Dohlman CH. The tensile strength of adhesive joints between eye tissues and alloplastic materials. Am. J. Ophthalmol.68, 248–255 (1960).
- Shigemitsu T, Majima Y. The utilization of biological adhesive for wound treatment: comparison of suture, self-sealing sutureless and cyanoacrylate closure in the tensile strength test. Int. Ophthalmol.20, 323–328 (1997).
- Ricci B, Ricci F, Bianchi PE. Octyl 2-cyanoacrylate in sutureless surgery of extraocular muscles: an experimental study in the rabbit model. Graefe’s Arc. Clin. Exp. Ophthalmol.238, 454–458 (2000).
- Eiferman RA, Snyder JW. Antibacterial effect of cyanoacrylate glue. Arch. Ophthalmol.101, 958–960 (1983).
- de Almeida Manzano RP, Naufal FC, Hida RY et al. Antibacterial analysis in vitro of ethyl-cyanoacrylate against ocular pathogens. Cornea25, 350–351 (2006).
- Wei-Lin C, Chung-Tien L, Chia-Yun H, I-Hua T, Willie YW, Fung-Rong H. Comparison of the bacteriostatic effects, corneal cytotoxicity, and the ability to seal corneal incisions among three different tissue adhesives. Cornea26, 1228–1234 (2007).
- Romero IL, Malta JB, Silva CB, Mimica LM, Soong KH, Hida RY. Antibacterial properties of cyanoacrylate tissue adhesive: does the polymerization reaction play a role? Indian J. Ophthalmol.57(5), 341–344 (2009).
- Prause JU, Jensen OA. Studies on human corneal ulcers treated with histoacrylic glue. Morphological studies of a successful and unsuccessful membrane. Acta Ophthalmol. (Copenh.)60, 547–556 (1982).
- Fogle JA, Kenyon KR, Foster CS. Tissue adhesive arrests stromal melting in the human cornea. Am. J. Ophthalmol.89, 795 (1980).
- Webster RG Jr, Slansky HH, Refojo MF et al. The use of adhesive for the closure of corneal perforations. Report of two cases. Arch. Ophthalmol.80, 705–709 (1968).
- Weiss JL, Williams P, Lindstrom RL, Doughman DJ. The use of tissue adhesive in corneal perforations. Ophthalmology90(6), 610–615 (1983).
- Sharma A, Kaur R, Kumar S et al. Fibrin glue versus N-butyl-2-cyanoacrylate in corneal perforations. Ophthalmology110(2), 291–298 (2003).
- Singh R, Umapathy T, Abedin A, Eatamadi H, Maharajan S, Dua HS. Choroidal detachment in perforated corneal ulcers: frequency and management. Br. J. Ophthalmol.90, 1111–1114 (2006).
- Alio JL, Mulet ME, Garcia JC. Use of cyanoacrylate tissue adhesive in small-incision cataract surgery. Ophthalmic. Surg. Lasers27(4), 270–274 (1996).
- Hermel B. Studies on the use of tissue adhesives in cataract microsurgery. Polim. Med.10(1), 3–18 (1980).
- Ritterband CD, Meskin WS, Shapiro ED, Kusmierczyk J, Seedor A, Koplin SR. Laboratory model of tissue adhesive (2-octyl cyanoacrylate) in sealing clear corneal cataract wounds. Am. J. Ophthalmol.140(6), 1039–1043 (2005).
- Meskin WS, Ritterband CD, Shapiro ED et al. Bandage (2-octyl-cyanoacrylate) as a temporary wound barrier in clear corneal cataract surgery. Ophthalmology112(11), 2015–2021 (2005).
- Zalta HA, Weider HR. Closure of leaking filtering blebs with cyanoacrylate tissue adhesive. Br. J. Ophthalmol.75, 170–173 (1991).
- Weber PA, Baker ND. The use of cyanoacrylate adhesive with a collagen shield in leaking filtering blebs. Ophthal. Surg.20(4), 284–285 (1998).
- Grady FJ, Forbes M. Tissue adhesive for repair of conjunctival buttonhole in glaucoma surgery. Am. J. Ophthalmol.68, 656–658 (1969).
- Awan KJ, Spaeth PG. Use of isobutyl 2-cyanoacrylate tissue adhesive in the repair of conjunctival fistula in filtering procedures of glaucoma. Ann. Ophthalmol.6, 851–853 (1974).
- Hartnett ME, Hirose T. Cyanoacrylate glue in the repair of retinal detachment associated with posterior retinal breaks in infants and children. Retina18(2), 125–129 (1998).
- Sheta SM, Hida T, McCuen BW. Experimental transvitreal cyanoacrylate retinopexy through silicone oil. Am. J. Ophthalmol.102(6), 717–722 (1986).
- Sternberg P, Tiedeman J, Prensky JG. Sutureless scleral buckle for retinal detachment with thick sclera. Retina8(4), 247–249 (1988).
- Ricci B, Ricci F. Octyl-2-cyanoacrylate tissue adhesive in experimental scleral buckling. Acta Ophthalmol. Scand.78, 506–508 (2001).
- Gilbert CE, Grierson I, McLeod D. Retinal patching: a new approach to the management of selected retinal breaks. Eye (Lond.)3(1), 19–26 (1989).
- Sheta SM, Hida T, McCuen BW. Cyanoacrylate tissue adhesive in the management of recurrent retinal detachment caused by macular hole. Am. J. Ophthalmol.109(1), 28–32 (1990).
- Lim LT, Bhatt PR, Ramaesh K. Harvesting keratolimbal allografts from corneoscleral buttons: a novel application of cyanoacrylate adhesive. Br. J. Ophthalmol.92, 1550–1551 (2008).
- Gupta KB, Edward D, Duffy TM. 2-Octyl cyanoacrylate tissue adhesive and muscle attachment to porous anophthalmic orbital implants. Ophthal. Plast. Reconstr. Surg.17, 264–269 (2001).
- Gallwmore RP, Green J, Shorr N, Goldberg RA. Use of isobutyl cyanoacrylate tissue adhesive to stabilize mucous membrane grafts in total socket reconstruction. Ophthal. Plast. Reconstr. Surg.15(3), 210–212 (1999).
- Shepler TR, Stuart R. Use of isobutyl cyanoacrylate tissue adhesive to stabilize external eyelid weights in temporary treatment of facial palsies. Ophthal. Plast. Reconstr. Surg.17(3), 169–173 (2001).
- Bhatia SS. Ocular surface sealants and adhesives. Ocul. Surf.4(3), 146–154 (2006).
- Vanathi M, Sharma N, Titiyal JS. Tectonic grafts for corneal thinning and perforations. Cornea21, 792–797 (2002).
- Foster CS. Tissue adhesives. In: Smolin and Toft’s The Cornea: Scientific Foundations and Clinical Practice (4th Edition). Lippincott Williams and Wilkins, PA, USA, 437–442 (2005).
- Wessels IF, McNeill JI. Applicator for cyanoacrylate tissue adhesive. Ophthalmic Surg.20, 211–214 (1989).
- Boruchoff SA, Donshik PC. Medical and surgical management of corneal thinnings and perforations. Int. Ophthalmol. Clin.15, 111–123 (1975).
- Jackson MR, MacPhee MJ, Drohan WN, Alving BM. Fibrin sealant: current and potential clinical applications. Blood Coagul. Fibrinolysis7, 737–746 (1996).
- Chung SI. Comparative studies on tissue transglutaminase and Factor XIII. Ann. NY Acad. Sci.202, 240–255 (1972).
- Iwanaga S, Suzuki K, Hashimoto S. Bovine plasma cold-insoluble globulin: gross structure and function. Ann. NY Acad. Sci.31256–31273 (1978).
- Sakata Y, Aoki N. Significance of cross-linking of α2-plasmin inhibitor to fibrin in inhibition of fibrinolysis and in hemostasis. J. Clin. Invest.69(3), 536–542 (1982).
- Spotnitz WD. Fibrin sealant: past, present, and future: a brief review. World J. Surg.34(4), 632–634 (2010).
- Young J, Medawar P. Fibrin suture of peripheral nerves. Lancet1(7), 77 (1943).
- Cronkite EP, Lozner EL, Deaver J. Use of thrombin and fibrinogen in skin grafting. JAMA124, 976–978 (1944).
- Dresdale A, Rose EA, Jeevanandam V. Preparation of fibrin glue from single-donor fresh-frozen plasma. Surgery97, 750–755 (1985)
- Tredree R, Beierlein W, Debrix I et al. Evaluating the differences between fibrin sealants: recommendations from an international advisory panel of hospital pharmacists. Eur. J. Hosp. Pharm. Sci.12(1), 3–9 (2006).
- Martinowtiz U, Bal F. Improved tissue glue prepared by using cyroprecitpitate. EP (application) Assigned to Octapharma AG Patent 534, 178 (1993).
- Bar L, Malka O, Naboichenko E, Nur I, The binding of fibrin sealant to collagen is influenced by the method of purification and the cross-linked fibrinogen–fibronectin (heteronectin) content of the ‘fibrinogen’ component. Blood Coagul. Fibrinolysis16(2), 111–117 (2005).
- Mangano DT, Tudor IC, Dietzel C. The risk associated with aprotinin in cardiac surgery. N. Engl. J. Med.354(4), 353–365 (2006).
- Nhunsberger, Microsoft Word – 5332–0002SCD_Vitagel Hemostat IFU – US_Rev.01_SCD.doc. 21 June 2007 (2010).
- Ortel TL, Mercer MC, Thames EH, Moore KD, Lawson JH. Immunologic impact and clinical outcomes after surgical exposure to bovine thrombin. Ann. Surg.233(1), 88–96 (2001).
- Rock G, Berger R, Lange J, Tokessy M, Palmer,DS, Giulivi A. A novel, automated method of temperature cycling to produce cryoprecipitate. Transfusion41(2), 232–235 (2001).
- Tidrick RT, Warner ED. Fibrin fixation of skin transplants. Surgery15(1), 90–95 (1944).
- Katzin HM. Aqueous fibrin fixation of corneal transplants in the rabbit. Arch. Ophthalmol.35, 415–420 (1945).
- Prabhasawat P, Barton, K, Burkett G, Tseng SCG. Comparison of conjunctival autografts amniotic membrane grafts, and primary closure for pterygium excision. Ophthalmology104(6), 974–985 (1997).
- Koranyi G, Seregard S, Kopp ED. The cut-and-paste method for primary pterygium surgery: long-term follow-up. Acta Ophthalmol. Scand.83(3), 298–301 (2005).
- Sridhar MS, Bansal AK, Rao GN. Surgically induced necrotizing scleritis after pterygium excision and conjunctival autograft. Cornea21, 305–307 (2002).
- Suzuki T, Sano Y, Kinoshita S. Conjunctival inflammation induces Langerhans’ cell migration into the cornea. Curr. Eye Res.21(1), 550–553 (2000).
- Koranyi G, Seregard S, Kopp ED. Cut and paste: a no suture, small incision approach to pterygium surgery. Br. J. Ophthalmol.88(7), 911–914 (2004).
- Hall RC, Logan AJ, Wells AP. Comparison of fibrin glue with sutures for pterygium excision surgery with conjunctival autografts. Clin. Exp. Ophthalmol.37(6), 584–589 (2009).
- Karalezli A, Kucukerdonmez C, Akova YA, Altan-Yaycioglu R, Borazan M. Fibrin glue versus sutures for conjunctival autografting in pterygium surgery: a prospective comparative study. Br. J. Ophthalmol.92(9), 1206–1210 (2008).
- Ratnalingam V, KeatEu AL, Ng GL, Taharin R, John E. Fibrin adhesive is better than sutures in pterygium surgery. Cornea29(5), 485–489 (2010).
- Ozdamar Y, Mutevelli S, Han U et al. A comparative study of tissue glue and vicryl suture for closing limbal–conjunctival autografts and histologic evaluation after pterygium excision. Cornea27(5), 552–558 (2008).
- Uy HS, Reyes JMG, Flores JDG et al. Comparison of fibrin glue and sutures for attaching conjunctival autografts after pterygium excision. Ophthalmology112(4), 667–671 (2005).
- Jiang J, Yang Y, Zhang M, Fu X, Bao X, Yao K. Comparison of fibrin sealant and sutures for conjunctival autograft fixation in pterygium surgery: one-year follow-up. Ophthalmologica222(2), 105–111 (2008).
- Pan HW, Zhong JX, Jing CX. Comparison of fibrin glue versus suture for conjunctival autografting in pterygium surgery: a meta-analysis. Ophthalmology118(6), 1049–1054 (2011).
- Solomon A, Pires RTF, Tseng SCG. Amniotic membrane transplantation after extensive removal of primary and recurrent pterygia. Ophthalmology108(3), 449–460 (2001).
- Jain AK, Bansal R, Sukhija J. Human amniotic membrane transplantation with fibrin glue in management of primary pterygia: a new tuck-in technique. Cornea27(1), 94–99 (2008).
- Kheirkhah A, Casas V, Sheha H, Raju VK, Tseng SC. Role of conjunctival inflammation in surgical outcome after amniotic membrane transplantation with or without fibrin glue for pterygium. Cornea27(1), 56–63 (2008).
- Zauberman H, Hemo I. Use of fibrin glue in ocular surgery. Ophthalmic Surg.19(2), 132–133 (1988).
- Biedner B, Rosenthal G. Conjunctival closure in strabismus surgery: vicryl versus fibrin glue. Opthalmic Surg.27(11), 967 (1996).
- Dadeya S, Kamlesh MS. Strabismus surgery: fibrin glue versus vicryl for conjuctival closure. Acta Ophthalmol. Scand.79(5), 515–517 (2001).
- Mohan K, KaurMalhi R, Sharma A, Kumar S. Fibrin glue for conjunctival closure in strabismus surgery. J. Pediat. Opthalmol. Strabismus40(3), 158–160 (2003).
- Tonelli E Jr, De Almeida HC, Bambirra EA. Tissue adhesives for a sutureless fadenoperation: an experimental study in a rabbit model. Invest. Ophthalmol. Visual Sci.45(12), 4340–4345 (2004).
- Krzizok T. Fibrin glue for closing conjunctival wounds in ophthalmic surgery. Ophthalmologe101(10), 1006–1010 (2004).
- Lagoutte FM, Gauthier L, Comte PRM. A fibrin sealant for perforated and preperforated corneal ulcers. Br. J. Ophthalmol.73(9), 757–761 (1989).
- Duchesne B, Tahi H, Galand A. Use of human fibrin glue and amniotic membrane transplant in corneal perforation. Cornea20(2), 230–232 (2001).
- Gupta N, Sachdev R, Tandon R. Sutureless patch graft for sterile corneal melts. Cornea29(8), 921–923 (2010).
- Chen HC, Tan HY, Hsiao CH, Huang SCM, Lin KK, Ma DHK. Amniotic membrane transplantation for persistent corneal ulcers and perforations in acute fungal keratitis. Cornea25(5), 564–572 (2006).
- Kim HK, Park HS. Fibrin glue-assisted augmented amniotic membrane transplantation for the treatment of large noninfectious corneal perforations. Cornea28(2), 170–176 (2009).
- Kheirkhah A, Casas V, Raju VK, Tseng SCG. Sutureless amniotic membrane transplantation for partial limbal stem cell deficiency. Am. J. Ophthalmol.145(5), 787–794 (2008).
- Kheirkhah A, Casas V, Blanco G. Amniotic membrane transplantation with fibrin glue for conjunctivochalasis. Am. J. Ophthalmol.144(2), 311–313 (2007).
- Hick S, Demers PE, Brunette I, La C, Mabon M, Duchesne B. Amniotic membrane transplantation and fibrin glue in the management of corneal ulcers and perforations: a review of 33 cases. Cornea24(4), 369–377 (2005).
- Sekiyama E, Nakamura T, Kurihara E et al. Novel sutureless transplantation of bioadhesive-coated, freeze-dried amniotic membrane for ocular surface reconstruction. Invest. Ophthalmol. Visual Sci.48(4), 1528–1534 (2007).
- Rostron CK, Brittain GP, Morton DB, Rees JE. Experimental epikeratophakia with biological adhesive. Arch. Ophthalmol.106(8), 1103–1106 (1988).
- Kim MS, Kim JH. Effects of tissue adhesive (Tisseel) on corneal wound healing in lamellar keratoplasty in rabbits. Korean J. Ophthalmol.3(1), 14–21 (1989).
- Rosenthal AR, Harbury C, Egbert PR, Rubenstein E. Use of a platelet fibrinogen thrombin mixture as a corneal adhesive: experiments with sutureless lamellar keratoplasty in the rabbit. Invest. Ophthalmol.14(11), 872–875 (1975).
- Hashemi H, Dadgostar A. Automated lamellar therapeutic keratoplasty with fibrin adhesive in the treatment of anterior corneal opacities. Cornea30(6), 655–659 (2011).
- Duarte MCB, Kim T. Sutureless lamellar keratoplasty: a modified approach for fibrin glue application. Cornea26(9), 1127–1128 (2007).
- Chen W, Qu J, Lu F, Zhu RY. Sutureless lamellar keratoplasty by microkeratome combined with fibrin tissue adhesive in rabbits. Chinese J. Ophthalmol.40(5), 331–336 (2004).
- Narendran N, Mohamed S, Shah S. No sutures corneal grafting – a novel use of overlay sutures and fibrin glue in deep anterior lamellar keratoplasty. Cont. Lens Ant. Eye30(3), 207–209 (2007).
- Oberg TJ, Friday JW, Ursea R, Snyder RW. Is Tisseel a viable option in posterior lamellar keratoplasty? Cornea28(6), 681–687 (2009).
- Por YM, Tan YL, Mehta JS, Tan DTH. Intracameral fibrin tissue sealant as an adjunct in tectonic lamellar keratoplasty for large corneal perforations. Cornea28(4), 451–455 (2009).
- Bahar I, Kaiserman I, Slomovic A, McAllum P, Rootman D. Fibrin glue for opposing wound edges in ‘top hat’ penetrating keratoplasty: a laboratory study. Cornea26(10), 1235–1238 (2007).
- Sonmez B, Beden Ã. Fibrin glue-assisted sutureless limbal stem cell transplantation surgery for the treatment of severe ocular chemical injury. Cornea30(3), 296–300 (2010).
- Nassiri N, Pandya HK, Djalilian AR. Limbal allograft transplantation using fibrin glue. Arch. Ophthalmol.129(2), 218–222 (2011).
- Zakaria N, Koppen C, Van Tendeloo V, Berneman Z, Hopkinson A, Tassignon MJ. Standardized limbal epithelial stem cell graft generation and transplantation. Tissue Eng. Part C Methods16(5), 921–927 (2010).
- Pfister R, Sommers CI. Fibrin sealant in corneal stem cell transplantation. Cornea24(5), 593–598 (2005).
- Higa K, Shimazaki J. Recent advances in cultivated epithelial transplantation. Cornea27(Suppl. 1), S41–S47 (2008).
- Narvaez J, Chakrabarty A, Chang K. Treatment of epithelial ingrowth after LASIK enhancement with a combined technique of mechanical debridement, flap suturing, and fibrin glue application. Cornea25(9), 1115–1117 (2006).
- Elderkin SJ, Epstein RJ, Seldomridge DL. Successful treatment of recurrent epithelial ingrowth associated with interface fluid syndrome, flap necrosis, and epithelial defects following LASIK. J. Refract. Surg.27(1), 70–73 (2011).
- Yeh DL, Bushley DM, Kim T. Treatment of traumatic LASIK flap dislocation and epithelial ingrowth with fibrin glue. Am. J. Ophthalmol.141(5), 960–962 (2006).
- Szurman P, Warga M, Grisanti S et al. Sutureless amniotic membrane fixation using fibrin glue for ocular surface reconstruction in a rabbit model. Cornea25(4), 460–466 (2006).
- Azar G, Doan S, Cochereau I, Gabison EE. Management of post-LASIK recurrent epithelial ingrowth with flap melting using annular amniotic membrane graft. J. Cataract Refract. Surg.36(12), 2207–2208 (2010).
- Bonatti JA, Bechara SJ, Dall’Col MW, Cresta FB, Carricondo PC, Kara-José N. A fibrin-related line of research and theoretical possibilities for the use of fibrin glue as a temporary basal membrane in non-perforated corneal ulcers and in photorefractive keratectomy (PRK)-operated corneas. Arq. Bras. Oftalmol.70(5), 884–889 (2007).
- O’Sullivan F, Dalton R, Rostron CK. Fibrin glue: an alternative method of wound closure in glaucoma surgery. J. Glaucoma5(6), 367–370 (1996).
- Gammon RR, Prum BE Jr, Avery N, Mintz PD. Rapid preparation of small-volume autologous fibrinogen concentrate and its same day use in bleb leaks after glaucoma filtration surgery. Ophthal. Surg. Lasers29(12), 1010–1012 (1998).
- Asrani SG, Wilensky JT. Management of bleb leaks after glaucoma filtering surgery: use of autologous fibrin tissue glue as an alternative. Ophthalmology103(2), 294–298 (1996).
- Grewing R, Mester U. Fibrin sealant in the management of complicated hypotony after trabeculectomy. Ophthal. Surg. Lasers28(2), 124–127 (1997).
- Wright MM, Brown EA, Maxwell K, Cameron JD, Walsh AW. Laser-cured fibrinogen glue to repair bleb leaks in rabbits. Arch. Ophthalmol.116(2), 199–202 (1998).
- Seligsohn A, Moster MR, Steinmann W, Fontanarosa J. Use of tisseel fibrin sealant to manage bleb leaks and hypotony: case series. J. Glaucoma13(3), 227 (2004).
- Lee GA, Holcombe DJ. Surgical revision of dysfunctional filtration blebs with bleb preservation, sliding conjunctival flap and fibrin glue. Eye24(6), 947–953 (2009).
- Bahar I, Weinberger D, Lusky M, Avisar R, Robinson A, Gaton D. Fibrin glue as a suture substitute: histological evaluation of trabeculectomy in rabbit eyes. Curr. Eye Res.31(1), 31–36 (2006).
- Freeman PD, Kahook MY, Curtis TH. Glaucoma drainage device implantation in children using fibrin glue. J. AAPOS14(2), 169–171 (2010).
- Kahook MY, Noecker RJ. Fibrin glue-assisted glaucoma drainage device surgery. Br. J. Ophthalmol.90(12), 1486–1489 (2006).
- Valimakii J. Fibrin glue for preventing immediate postoperative hypotony following glaucoma drainage implant surgery. Acta Ophthalmol. Scand.84(3), 372–374 (2006).
- Hovanesian JA, Karageozian VH. Watertight cataract incision closure using fibrin tissue adhesive. J. Cataract Refract. Surg.33(8), 1461–1463 (2007).
- Kim T, Kharod BV. Tissue adhesives in corneal cataract incisions. Curr. Opin. Ophthalmol.18(1), 39–43 (2007).
- Mulet Homs ME, Alio Y, Sanz JL, Gobby F. Efficacy of fibrinogen as bioadhesive in cataract surgery through scleral tunnel. J. Cataract Refract. Surg.72(6), 427–430 (1997).
- Grewing R, Mester U. Radial suture stabilized by fibrin glue to correct preoperative against – the-rule astigmatism during cataract surgery. Ophthal. Surg.25(7), 446–448 (1994).
- Henrick A, Kalpakian B, Gaster RN, Vanley C. Organic tissue glue in the closure of cataract incisions in rabbit eyes. J. Cataract Refract. Surg.17(5), 551–555 (1991).
- Henrick A, Gaster RN, Silverstone PJ. Organic tissue glue in the closure of cataract incisions. J. Cataract Refract. Surg.13(5), 551–553 (1987).
- Kumar DA, Agarwal A, Prakash G, Jacob S, Saravanan Y, Agarwal A. Glued posterior chamber IOL in eyes with deficient capsular support: a retrospective analysis of 1-year postoperative outcomes. Eye24(7), 1143–1148 (2010).
- Agarwal A, Kumar DA, Jacob S, Baid C, Agarwal A, Srinivasan S. Fibrin glue-assisted sutureless posterior chamber intraocular lens implantation in eyes with deficient posterior capsules. J. Cataract Refract. Surg.34(9), 1433–1438 (2008).
- Buschmann W. Microsurgical treatment of lens capsule perforations. Part II: clinical applications and results. Ophthal. Surg.18(3), 276–282 (1987).
- Coleman DJ, Lucas BC, Fleischman JA. A biologic tissue adhesive for vitreoretinal surgery. Retina8(4), 250–256 (1988).
- Uhlig CE, Gerding H. Fibrin sealing improves stability of corneal prostheses during vitreoretinal procedures. Retina23(2), 209–214 (2003).
- Goessl A, Redl H. Optimized thrombin dilution protocol for a slowly setting fibrin sealant in surgery. Acta Chirurgica Austriaca37(1), 43–51 (2005).
- Champalakshmi R, Chaurasia SS, Angunawaela RI, Tan DT, Mehta JS. Optimization and comparison of fibrin glue spray system for ocular delivery. Presented at: Asia ARVO. Singapore, 20–22 January 2011 (Poster #728).
- Wilson SM, Pell P, Donegan EA. HIV-1 transmission following the use of cryoprecipitate fibrinogen as gel/adhesive. Transfusion31, S51 (1991).
- Hino M, Ishiko O, Honda KI et al. Transmission of symptomatic parvovirus B19 infection by fibrin sealant used during surgery. Br. J. Haematol.108, 194–195 (2000).
- Rubel C, Fernandez GC, Dran G, Bompadre MB, Isturiz MA, Palermo MS. Fibrinogen promotes neutrophil activation and delays apoptosis. J. Immunol.166, 2002–2010 (2001).
- Banninger H, Hardegger T, Tobler A et al. Fibrin glue in surgery: frequent development of inhibitors of bovine thrombin and human Factor V. Br. J. Haematol.855, 528–532 (1993).
- Rapaport SI, Zivelin A, Minow R, Hunter CS, Donnelly K. Clinical significance of antibodies to bovine and human thrombin and factor V after surgical use of bovine thrombin. Am. J. Clin. Pathol.97, 84–91 (1992).
- Carnahan MA, Grinstaff MW. Synthesis and characterization of poly(glycerol-succinic acid) dendrimers. Macromolecules34(22), 7648–7655 (2001).
- Berdahl JP, Johnson CS, Proia AD, Grinstaff MW, Kim T. Comparison of sutures and dendritic polymer adhesives for corneal laceration repair in an in vivo chicken model. Arch. Ophthalmol.127(4), 442–447 (2009).
- Johnson CS, Wathier M, Grinstaff M, Kim T. In vitro sealing of clear corneal cataract incisions with a novel biodendrimer adhesive. Arch. Ophthalmol.127(4), 430–434 (2009).
- Wathier M, Jung PJ, Carnahan MA, Kim T, Grinstaff MW. Dendritic macromers as in situ polymerizing biomaterials for securing cataract incisions. J. Am. Chem. Soc.126(40), 12744–12745 (2004).
- Kang C, Carnahan MA, Wathier M, Grinstaff MW, Kim T. Novel tissue adhesives to secure laser in situ keratomileusis flaps. J. Cataract Refract. Surg.31(6), 1208–1212 (2005).
- Wathier M, Johnson MS, Carnahan MA et al.In situ polymerized hydrogels for repairing scleral incisions used in pars planavitrectomy procedures. ChemMedChem1(8), 821–825 (2006).
- Degortcija L, Starck Johnson C, Wathier M, Kim T, Grinstaff MW. Photo cross-linkable biodendrimers as ophthalmic adhesives for central lacerations and penetrating keratoplasties. Invest. Ophthalmol. Visual Sci.48(5), 2037–2042 (2007).
- Velazquez AJ, Carnahan MA, Kristinsson J, Stinnett S, Grinstaff MW, Kim T. New dendritic adhesives for sutureless ophthalmic surgical procedures: in vitro studies of corneal laceration repair. Arch. Ophthalmol.122(6), 867–870 (2004).
- Kang PC, Jung PJ, Carnahan MA, Kim T, Grinstaff MW. Novel tissue adhesives to secure laser in situ keratomileusis flaps. J. Cataract Refract. Surg.31(6), 1208–1212 (2005).
- Araki M, Tao H, Nakajima N et al. Development of new biodegradable hydrogel glue for preventing alveolar air leakage. J. Thorac. Cardiovasc. Surg.134(5), 1241–1248 (2007).
- Nakajima N, Sugai H, Tsutsumi S, Hyon SH. Self-degradable bioadhesives. Key Eng. Mat.342, 713–716 (2007).
- Takaoka M, Nakamura T, Sugai H. Sutureless amniotic membrane transplantation for ocular surface reconstruction with a chemically defined bioadhesive. Biomaterials29(19), 2923–2931 (2008).
- Takaoka M, Nakamura T, Sugai H. Novel sutureless keratoplasty with a chemically defined bioadhesive. Invest. Ophthalmol. Visual Sci.50(6), 2679–2685 (2009).
- Kirker KR, Luo Y, Nielson JH, Shelby J, Prestwich GD. Glycosaminoglycan hydrogel films as bio-interactive dressings for wound healing. Biomaterials23(17), 3661–3671 (2002).
- Reyes JMG, Herretes S, Pirouzmanesh A. A modified chondroitin sulfate aldehyde adhesive for sealing corneal incisions. Invest. Ophthalmol. Visual Sci.46(4), 1247–1250 (2005).
- Braunwald NS, Gay W, Tatooles CJ. Evaluation of crosslinked gelatin as a tissue adhesive and hemostatic agent: an experimental study. Surgery59(6), 1024–1030 (1966).
- Sung HW, Huang DM, Chang WH, Huan RN, Hsu JC. Evaluation of gelatin hydrogel crosslinked with various crosslinking agents as bioadhesives: in vitro study. J. Biomed. Mat. Res.46(4), 520–530 (1999).
- Ennker J, Ennker IC, Schoon D et al. The impact of gelatin-resorcinol glue on aortic tissue: a histomorphologic evaluation. J. Vasc. Surg.20(1), 34–43 (1994).
- Ennker IC, Ennker J, Schoon D, Schoon HA, Rimpler M, Hetzer R. Formaldehyde-free collagen glue in experimental lung gluing. Ann. Thorac. Surg.57(6), 1622–1627 (1994).
- Wertzel H, Wagner B, Stricker A et al. Experimental gluing of lung parenchyma in rats. Thorac. Cardiovasc. Surgeon45(2), 83–87 (1997).
- Chao HH, Torchiana DF. BioGlue: albumin/glutaraldehyde sealant in cardiac surgery. J. Card. Surg.18(6), 500–503 (2003).
- Anghelacopoulos SE, Tagarakis GI, Pilpilidis I, Kartsounis C, Chryssafis G. Albumin–glutaraldehyde bioadhesive (‘Bioglue’) for prevention of postoperative complications after stapled hemorrhoidopexy: a randomized controlled trial. Wien. Klin. Wochenschr.118(15–16), 469–472 (2006).
- Engel HM, Chechik D, Burde RM. Repair of a traumatic scleral rupture with scleral imbrication and BioGlue. Retina27(4), 505–508 (2007).
- Furst W, Banerjee A. Release of glutaraldehyde from an albumin–glutaraldehyde tissue adhesive causes significant in vitro and in vivo toxicity. Ann. Thorac. Surg.79(5), 1522–1528 (2005).
- Khadem J, Martino M, Anatelli F, Dana MR, Hamblin MR. Healing of perforating rat corneal incisions closed with photodynamic laser-activated tissue glue. Lasers Surg. Med.35(4), 304–311 (2004).
- Khadem JJ. Dana MR. Photodynamic biologic tissue glue in perforating rabbit corneal wounds. J. Clin. Laser Med. Surg.18(3), 125–129 (2000).
- Bloom JN, Duffy MT, Davis JB, McNally-Heintzelman KM. A scaffold-enhanced laser-activated surgical adhesive technique for sutureless strabismus surgery. Invest. Ophthalmol. Visual. Sci.44 (2003) (E-Abstract 2748).
- Bloom JN, Duffy MT, Davis JB, McNally-Heintzelman KM. A light-activated surgical adhesive technique for sutureless ophthalmic surgery. Arch. Ophthalmol.121(11), 1591–1595 (2003).
- Letko E, Majmudar PA, Forstot SL, Epstein RJ, Rubinfeld RS. UVA-light and riboflavin-mediated corneal collagen cross-linking. Int. Ophthalmol. Clin.51(2), 63–76 (2011).
- Wollensak G, Spoerl E, Seiler T. Riboflavin/ultraviolet-A-induced collagen crosslinking for the treatment of keratoconus. Am. J. Ophthalmol.135(5), 620–627 (2003).
- Raiskup-Wolf F, Hoyer A, Spoerl E et al. Collagen crosslinking with riboflavin andultraviolet-A light in keratoconus: long-term results. J. Cataract Refract. Surg.347, 796–801 (2008).
- Kohlhaas M, Spoerl E, Speck A et al. [A new treatment of keratectasia after LASIK by using collagen with riboflavin/UVA light cross-linking.] Klin. Monbl. Augenheilkd.222, 430–436 (2005).
- Hafezi F, Kanellopoulos J, Wiltfang R et al. Corneal collagen crosslinking with riboflavin and ultraviolet A to treat induced keratectasia after laser in situ keratomileusis. J. Cataract Refract. Surg.33, 2035–2040 (2007).
- Wollensak G, Sparl E, Reber F, Pillunat L, Funk R. Corneal endothelial cytotoxicity of riboflavin/UVA treatment in vitro. Ophthal. Res.35(6), 324–328 (2003).
- Kissner A, Spoerl E, Jung R, Spekl K, Pillunat LE, Raiskup F. Pharmacological modification of the epithelial permeability by benzalkonium chloride in UVA/riboflavin corneal collagen cross-linking. Curr. Eye Res.35(8), 715–721 (2010).
- Chan CCK, Sharma M, Wachler BSB. Effect of inferior-segment Intacs with and without C3-R on keratoconus. J. Cataract Refract. Surg.33(1), 75–80 (2007).
- Miki D, Dastgheib K, Kim T et al. A photopolymerized sealant for corneal lacerations. Cornea21(4), 393–399 (2002).
- Rossi F, Pini R, Menabuoni L et al. Experimental study on the healing process following laser welding of the cornea. J. Biomed. Optics10(2), 1–7 (2005).
- Pini R, Rossi F, Menabuoni L et al. In diode laser welding for cornea suturing: an experimental study of the repair process. Proc. SPIE Biomed. Optics Imag.245–252 (2004).
- L’Esperance FA Jr. Argon and ruby laser photocoagulation of disciform macular disease. Trans. Am. Acad. Opthalmol. Otolaryngol.75(3), 609–628 (1971).
- Timberlake GT, Patmore A, Shallal A, Marshall J, McHugh D. Thermal and infrared-diode laser effects on indocyanine-green-treated corneal collagen. Proc. SPIE Int. Soc. Optical Eng.1882, 244–253 (1993).
- Pini R, Menabuoni L, Starnotti L. First application of laser welding in clinical transplantation of the cornea. Proc. SPIE Int. Soc. Optical Eng.2001, 266–271 (2001).
- Deckelbaum LI, Isner JM, Donaldson RF. Use of pulsed energy delivery to minimize tissue injury resulting from carbon dioxide laser irradiation of cardiovascular tissues. J. Am. Coll. Cardiol.7(4), 898–908 (1986).
- Burstein NL, Williams JM Sr, Nowicki MJ, Johnson DE, Jeffers WQ. Corneal welding using hydrogen fluoride lasers [2]. Arch. Ophthalmol.110(1), 12–13 (1992).
- Trabucchi G, Gobbi PG, Brancato R et al. Laser welding of corneal tissue: preliminary experiences using 810nm and 1950nm diode lasers, Proc. SPIE Int. Soc. Optical Eng.1996, 380–387 (1996).
- Matteini P, Rossi F, Menabouni L, Pini R. Microscopic analysis of structural changes in diode-laser-welded corneal stroma. Proc. SPIE Int. Soc. Optical Eng.6426, 14 (2007).
- Menabuoni L, Pini R, Rossi F, Lenzetti I, Yoo SH, Parel JM. Laser-assisted corneal welding in cataract surgery: retrospective study. J. Cataract Refract. Surg.33(9), 1608–1612 (2007).
- Menabuoni L, Minicione F, Minicione GP, Pini R. Laser welding to assist penetrating keratoplasty: in vivo studies. Proc. SPIE Int. Soc. Optical Eng.3195, 25 (1998).
- Kirsch AJ, Miller MI, Hensle TW. Laser tissue soldering in urinary tract reconstruction: first human experience. Urology46(2), 261–266 (1995).
- Khadem J, Truong T, Ernest JT. Photodynamic biologic tissue glue. Cornea13(5), 406–410 (1994).
- Bass LS, Treat MR. Laser tissue welding: a comprehensive review of current and future clinical applications. Lasers Surg. Med.17(4), 315–349 (1995).
- Poppas DP, Schlossberg SM, Richmond IL, Gilbert DA, Devine CJ Jr. Laser welding in urethral surgery: improved results with a protein solder. Urology139(2), 415–417 (1988).
- Noguera G, Lee WS, Castro-Combs J et al. Novel laser-activated solder for sealing corneal wounds. Invest. Ophthalmol. Visual Sci.48(3), 1038–1042 (2007).
- Gobin A, O’Neal DP, Halas NJ, West J. Nanoshells as near infrared absorbers to enhance laser tissue welding, Transactions. Presented at: 7th World Biomaterials Congress. Sydney, Australia, 17–21 May 2004.
- Gobin AM, O’Neal DP, Halas NJ, Drezek RA, West JL. Laser tissue soldering with near-infrared absorbing nanoparticles. Proc. SPIE Biomed. Optics Imag.261–266 (2005).
- Ratto F, Matteini P, Rossi F et al. Photothermal effects in connective tissues mediated by laser-activated gold nanorods. Nanomedicine5(2), 143–151 (2009).
- Rossi F, Matteini P, Ratto F, Menabuoni L, Lenzetti I, Pini R. Laser welding of chitosan-GNRs films for the closure of a capsulorhexis. Proc. SPIE Biomed. Optics Imag.7885, 78851O-78851O-4 (2011).
- Hirsch LR, Stafford RJ, Bankson JA et al. Nanoshell-mediated near-infrared thermal therapy of tumors under magnetic resonance guidance. Proc. Natl Acad. Sci. USA100(23), 13549–13554 (2003).
- Schober R, Ulrich F, Sander T. Laser-induced alteration of collagen substructure allows microsurgical tissue welding. Science232(4756), 1421–1422 (1986).
- Timberlake GT, Patmore A, Shallal A, McHugh D, Marshall J. Thermal and infrared-diode laser effects on indocyanine-green-treated corneal collagen. Proc. SPIE Int. Soc. Optical Eng.1882, 244–253 (1993).
- Allain JC, Le Lous M, Cohen-Solal L. Isometric tensions developed during the hydrothermal swelling of rat skin. Connective Tissue Res.7(3), 127–133 (1980).
- Hayashi K, Thabit IG, Bogdanske JJ, Mascio LN, Markel MD. The effect of nonablative laser energy on the ultrastructure of joint capsular collagen. Arthroscopy12(4), 474–481 (1996).
- Aeschlimann D, Thomazy V. Protein crosslinking in assembly and remodelling of extracellular matrices: the role of transglutaminases. Connective Tissue Res.41(1), 1–27 (2000).
- Griffin M, Casadio R, Bergamini CM. Transglutaminases: nature’s biological glues. Biochem. J.368(2), 377–396 (2002).
- Jürgensen K, Aeschlimann D, Cavin V, Genge M, Hunziker EB. A new biological glue for cartilage–cartilage interfaces: tissue transglutaminase. J. Bone Joint Surg. A79(2), 185–193 (1997).
- Raghunath M, Höpfner B, Aeschlimann D et al. Cross-linking of the dermo-epidermal junction of skin regenerating from keratinocyte autografts: anchoring fibrils are a target for tissue transglutaminase. J. Clin. Invest.98(5), 1174–1184 (1996).
- Kaartinen MT, Murshed M, Karsenty G, McKee MD. Osteopontin upregulation and polymerization by transglutaminase 2 in calcified arteries of matrix Gla protein-deficient mice. J. Histochem. Cytochem.55(4), 375–386 (2007).
- Samuelsson B, Stenberg P, Pandolfi M. Localization and characteristics of transglutaminase in the rabbit and human eye. Graefe’s Arch. Clin. Exp. Ophthalmol.218(5), 233–236 (1982).
- Hidasi V, Adany R, Muszbek L. Localization of transglutaminase in human lenses. J. Histochem. Cytochem.43(11), 1173–1177 (1995).
- Raghunath M, Cankay R, Kubitscheck U. Transglutaminase activity in the eye: cross-linking in epithelia and connective tissue structures. Invest. Ophthalmol. Visual Sci.40(12), 2780–2787 (1999).
- Panengad P, Salazar G, Liew M et al. Biological cornea glue – in vitro evaluation. Presented at: ARVO Annual Meeting. Fort Lauderdale, FL, USA, 4–19 May 2010.
Patent
- Schwartz O, Linnau Y, Loblich F et al.: US Patent no. 4377572 (1982).
Websites
- US FDA. Vitagel™ Surgical Hemostat – P050044. www.fda.gov/MedicalDevices/ProductsandMedicalProcedures/DeviceApprovalsandClearances/Recently-ApprovedDevices/ucm078128.htm
- US FDA. FDA’s Role. www.fda.gov/cdrh/pdf/p010003b.pdf 2001
Tissue adhesives in ocular surgery
To obtain credit, you should first read the journal article. After reading the article, you should be able to answer the following, related, multiple-choice questions. To complete the questions (with a minimum 70% passing score) and earn continuing medical education (CME) credit, please go to www.medscape.org/journals/expertophth. Credit cannot be obtained for tests completed on paper, although you may use the worksheet below to keep a record of your answers. You must be a registered user on Medscape.org. If you are not registered on Medscape.org, please click on the New Users: Free Registration link on the left hand side of the website to register. Only one answer is correct for each question. Once you successfully answer all post-test questions you will be able to view and/or print your certificate. For questions regarding the content of this activity, contact the accredited provider, [email protected]. For technical assistance, contact [email protected]. American Medical Association’s Physician’s Recognition Award (AMA PRA) credits are accepted in the US as evidence of participation in CME activities. For further information on this award, please refer to http://www.ama-assn.org/ama/pub/category/2922.html. The AMA has determined that physicians not licensed in the US who participate in this CME activity are eligible for AMA PRA Category 1 Credits™. Through agreements that the AMA has made with agencies in some countries, AMA PRA credit may be acceptable as evidence of participation in CME activities. If you are not licensed in the US, please complete the questions online, print the AMA PRA CME credit certificate and present it to your national medical association for review.
Activity Evaluation: Where 1 is strongly disagree and 5 is strongly agree
1. You are seeing a 24-year-old man with a left corneal perforation after an accident at work. As you evaluate this patient, what should you consider regarding the most important intervention to assure rapid wound healing and a reduced risk for recurrence?
□ A Use of at least 2 prophylactic antibiotics after surgical repair
□ B Immediate sealing and adhesion of injured tissue
□ C Use of chondroitin sulfate aldehyde adhesive instead of sutures
□ D The routine use of lamellar keratoplasty
2. You are deciding whether to apply cyanoacrylate glue or fibrin glue to repair this patient’s corneal perforation. What should you consider regarding these 2 treatments?
□ A Cyanoacrylate glue has less bonding strength
□ B Cyanoacrylate glue is higher in cost
□ C Fibrin glue carries a risk for viral transmission and infection
□ D Fibrin glue has a higher rate of toxicity
3. What should you consider regarding investigatory adhesives for use in ocular surgery?
□ A Photo-activated biodendritic adhesive is associated with high degrees of inflammation and corneal opacities
□ B Chemically derived bioadhesives may offer superior bonding strength compared with fibrin glue
□ C Most chemically derived bioadhesives require expensive specialized equipment
□ D Photo-activated biodendritic adhesive is associated with complications due to stiffness of the resultant scar
4. A colleague suggests that you might be welding or soldering this patient’s wound with a laser in the next few years. What should you consider regarding these procedures?
□ A Temperature control is a substantial challenge in any laser-induced tissue fusion technique
□ B The most common chromophore for use during laser welding ophthalmic procedures is a porcine collagen preparation
□ C Laser soldering produces less bonding strength compared with laser welding
□ D Laser soldering now has a fair amount of evidence supporting its use in vivo