Abstract
The gastrointestinal (GI) tract, with its layered structure, can be imaged by ultrasound using a transabdominal approach or intraluminal high-frequency probes. New ultrasound technology can be used to characterize tissue hardness, study motility in real-time, direct diagnostic and therapeutic intervention, evaluate GI wall perfusion and tissue viability, and perform 3D imaging. Ultrasound is a safe imaging modality, and development of smaller devices has improved its application as a flexible clinical tool, which also can be used bedside. Recently, microbubbles have been injected into the blood stream loaded with contrast agents, or other diagnostic and therapeutic agents. Such bubbles can be destroyed by ultrasound waves, thus releasing their content at a given area of interest. In this article, we present a review of the GI wall anatomy and discuss currently available ultrasound technology for diagnosis and treatment of GI wall disorders.
Adapted with permission from Citation[1] © Elsevier.
![Figure 1. Overall histological organization of the digestive tube.Adapted with permission from Citation[1] © Elsevier.](/cms/asset/d9a9e1a0-10ed-4b45-a6e2-45b92365c845/ierd_a_11207343_f0001_b.jpg)
(A) The ultrasonographic five-layer and (B) nine-layer gastrointestinal wall structure. The interface echoes of the ultrasound image will appear continuous with the echo-rich wall layers. By thickening of the Mm and a visible interface echo generated between the inner and outer part of the muscularis propria, up to nine different layers can be separated by ultrasonography.
Mm: Muscularis mucosae.
Reproduced with permission from Citation[56].
![Figure 2. Relationship between histological and ultrasonographic gastrointestinal wall layers.(A) The ultrasonographic five-layer and (B) nine-layer gastrointestinal wall structure. The interface echoes of the ultrasound image will appear continuous with the echo-rich wall layers. By thickening of the Mm and a visible interface echo generated between the inner and outer part of the muscularis propria, up to nine different layers can be separated by ultrasonography.Mm: Muscularis mucosae.Reproduced with permission from Citation[56].](/cms/asset/8f19ee7c-840e-433f-9f63-4ed1071af520/ierd_a_11207343_f0002_b.jpg)
A thickened layer 2 corresponding to the gastric mucosa is seen (arrows). Reproduced with permission from Citation[56].
![Figure 3. A five-layered structure of the gastric wall showing Menetriers disease.A thickened layer 2 corresponding to the gastric mucosa is seen (arrows). Reproduced with permission from Citation[56].](/cms/asset/253c657a-9f98-41ca-b1ec-c4e4d08920d5/ierd_a_11207343_f0003_b.jpg)
(A) Elastogram in which the blue color indicates a hard and thickened anterior and posterior gastrointestinal wall. (B) Corresponding grayscale ultrasound image.
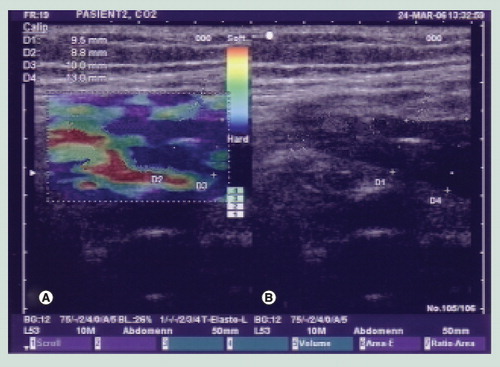
(A) An ultrasound miniprobe (p) is placed in the relaxed water-filled antral part of the stomach. (B) A contraction with thickening of the mp is seen.
m: Mucosa; mp: Muscularis propria; p: Probe; sm: Submucosa. Reproduced with permission from Citation[56].
![Figure 5. Gastric contraction demonstrated by intraluminal ultrasonography.(A) An ultrasound miniprobe (p) is placed in the relaxed water-filled antral part of the stomach. (B) A contraction with thickening of the mp is seen.m: Mucosa; mp: Muscularis propria; p: Probe; sm: Submucosa. Reproduced with permission from Citation[56].](/cms/asset/3c8fe3d5-c5e5-420e-933b-0428d8bd42fa/ierd_a_11207343_f0005_b.jpg)
(A) B-mode image of the terminal ileum where a diseased bowel loop has fused causing a sharp turn and an obstruction. (B) The corresponding image in contrast mode at the time of injection showing only interface echoes in the abdominal wall. (C) Just after the arrival of contrast in the right iliac artery and the bowel submucosa. (D) The contrast enhancement has reached maximum intensity. It is not only brighter in the submucosa but also filling the mucosa and proper muscle.
Image courtesy of K Nylund.
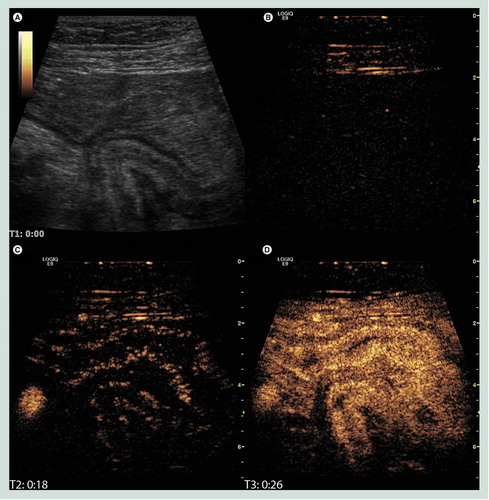
Scanning plane a goes through the ultrasound probe and is outside the data set of the tumor. The ultrasound probe is indicated by the arrow.
tu: Inflitrating tumor.
Reproduced with permission from Citation[56].
![Figure 7. 3D ultrasonography of an infiltrating tumor in the cardia is seen in two scanning planes (b and c).Scanning plane a goes through the ultrasound probe and is outside the data set of the tumor. The ultrasound probe is indicated by the arrow.tu: Inflitrating tumor.Reproduced with permission from Citation[56].](/cms/asset/38a074b3-f8c1-43df-b3e5-c3dfb0ec315e/ierd_a_11207343_f0007_b.jpg)
t is seen close to a v. Fine-needle aspiration is performed through the duodenal wall. The echo of the puncture needle is seen (arrow) (C). The final diagnosis was sarcoma.
t: Tumor; v: Vessel.
Reproduced with permission from Citation[56].
![Figure 8. Ultrasound-guided fine-needle puncture. (A–C)t is seen close to a v. Fine-needle aspiration is performed through the duodenal wall. The echo of the puncture needle is seen (arrow) (C). The final diagnosis was sarcoma.t: Tumor; v: Vessel.Reproduced with permission from Citation[56].](/cms/asset/1c389fec-7368-4356-96e1-ae97a5b54cc0/ierd_a_11207343_f0008_b.jpg)
Advances in medical imaging have improved the ability to study the wall of the gastrointestinal (GI) tract. In this respect, ultrasonography (US) with its many modalities has gained a central role because of its safety, relatively low cost and ability to generate high-resolution images. The GI wall can be imaged in detail using transabdominal US or endosonography (ES), and a recognizable wall layer structure that correlates closely to layers seen on histology can be visualized. Furthermore, intramural vessels can be displayed allowing blood flow to be evaluated with Doppler US.
To achieve better and safer diagnostic and treatment procedures with fewer side effects has always been a goal for physicians. In cancer and other chronic diseases of the GI tract, new ultrasound-based technologies (e.g., Doppler US, contrast-enhanced US, sonoelastography and US-guided interventions) have improved imaging of intramural disease, such as early cancer, abnormal wall structure in inflammatory bowel disease and ischemic disorders. Furthermore, US is a suitable tool for obtaining guided biopsies and performing minimal invasive and endoscopic surgery. US can also add value to other diagnostic or therapeutic techniques, such as the emerging nanotechnology. Ultrasound can be applied to deliver certain agents or molecules into a tumor and areas of inflammation, potentially leading to improvements in the treatment of cancer and inflammatory bowel disease as well as other disorders in the near future.
Anatomy & pathology of the GI wall
General
The GI tract is a functional unit for digestion of food with well-coordinated anatomical parts that have different functions. The different steps of passage and degradation of food occur within defined time periods that are subject to rhythmic variations, ranging from minutes to hours and up to circadian periods. Whenever imaging of the GI tract is performed, normal and pathological motility aspects should also be considered.
High-frequency ultrasound imaging techniques have a resolution that can produce images reflecting even small anatomical structures. Therefore, the present article will be restricted to structural aspects that are relevant for newer and detailed US imaging Citation[1,2]. A general scheme for the structures in the GI tract in this size range is given in .
Esophagus
The esophagus is a muscular tube lined by squamous epithelium that extends down to a border zone to the stomach, consisting of 1.5–2 cm of columnar mucosal glands. The distance from the upper limit at the cricopharyngeal sphincter down to the functional sphincter at the gastrooesophageal junction is approximately 25 cm. The mucosa of the esophagus consists of nonkeratinising squamous epithelium that overlies connective tissue papillae containing blood and lymphatic vessels. Under this is a narrow muscular layer of the mucosa followed by the submucosal layer with fibrous collagen tissue. As in the rest of the GI tract there is a circular and a longitudinal muscular layer (muscularis propria) in the esophageal wall, on which the outer layer, adventitia (serosa), is situated. Besides the rhythmic muscular movements connected to the swallowing procedure, an important function of the esophagus is to prevent regurgitation of the acidic gastric content by its lower sphincter function.
Stomach
The stomach has a pear shape and is arbitrarily divided into four regions: the upper one, cardia, a 2–3 cm wide zone surrounding the esophageal opening; the fundus, projecting to the left of the esophageal junction; the body, or corpus, consisting of the middle and largest portion; and the antrum that ends with pylorus, or the exit to the upper part of the small intestines, the duodenum.
The cardia is lined by a mucosa with simple tubular or cystic glands consisting of mucus-secreting cells. In addition, there are numerous endocrine cells as well as some scattered parietal (acid-secreting) and chief (pepsinogen-secreting) cells. The mucosa of the corpus, comprising about two-thirds of the internal surface of the organ, is made up of tightly packed tubular glands. Their upper part, close to the lumen, consists of parietal cells and the lower part of chief cells. The triangular-shaped antrum is lined by branched, tortuous and less tightly packed glands than the corpus, but otherwise the same types of cells.
In general, the superficial mucosal lining of the gastric wall consists of mucous cells down to the ‘neck’ of the glands. The produced mucins are glyocproteins with high molecular mass, which in 5% aqueous solution makes an insoluble 0.1-mm thick layer forming an acid- and protease-resistant barrier on the surface cells. Among the glandular cells are also numerous neuroendocrine cells. Under the epithelial layer is a lamina propria, consisting of loose connective tissue together with lymphoid cells and small blood vessels. As in esophagus, there is a narrow muscular layer of the mucosa and thereafter a submucosa with connective tissue, blood, lymphatic vessels, nerves and ganglia. In addition, here a muscularis propria and a serosa form the outer layers.
The mucosa of a normal, contracted stomach will protrude into numerous, mainly longitudinal folds, which are reduced on dilatation. These folds can be seen with imaging techniques. Their pattern is disturbed in different pathological conditions, for example, by ulcers and tumors.
Small intestine
The main functions of the small intestine are enzymatic digestion together with absorption of nutrients. The mucosa has an enormous surface because of the formation of villi protruding from the surface. Each villus is lined by epithelial cells, which have their surface covered by microvilli, which further increase the surface where absorption can occur. In addition to villi, the mucosa is organized into crypts. Among the absorptive epithelial cells are scattered goblet cells in addition to endocrine cells producing a wide variety of peptide hormones with local regulatory effects. At the base of the crypts there are cells containing lysosome-rich granules, the so-called Paneth cells, which may have growth and differentiation controlling functions on neighboring, local stem cells. In addition, the duodenal submucosa contains groups of alkaline mucus-secreting acini, Brunner’s glands, which are essential for protection of the mucosa against acid degradation from gastric fluid. The next part of the small intestine is jejunum, followed by the ileum, which ends in the large intestine. Villi may be less prominent in the distal parts of the small intestine and Brunner’s glands are not present.
An important feature of the small intestine is the lymphoid tissue in the lamina propria, consisting of prominent lymphatics, blood capillaries and lymphocytes, plasma cells, granulocytes and mast cells infiltrating the loose connective tissue. In addition, there are many lymph follicles, being most numerous in the terminal ileum, called Peyer’s patches. Altogether, the lymphoid cells are responsible for the mucosa-associated lymphoid tissue. Thus, the lamina propria in both the small and large intestine forms the mucosal immune system. Enlarged Peyer’s patches can be visualized with imaging techniques.
Large intestine (colon) & rectum
The large intestine (colon) and rectum have a length of approximately 1.5 m out of a total of 5–7 m for the entire small and large intestine and are responsible for storage, further passage and elimination of food residues. The colon also has an important role in maintaining the fluid and electrolyte balance. Vast numbers of bacteria are involved in the degradation of complex carbohydrates and other nutrients. The large intestine comprises altogether five different anatomical parts: coecum, ascending colon, transverse colon, sigmoideum and rectum. The mucosa only has perpendicular crypts going from the relatively flat surface and down to muscularis mucosae, consisting of ordinary columnar epithelial cells and an abundance of mucous-producing goblet cells. In between is a lamina propria with local immune functions as in the small intestine.
As in the rest of the GI tract, the colon and rectum walls consist of a muscularis mucosa, a submucosal layer and a muscularis propria with one longitudinal and one circular muscular layer. The outermost part is a serosal layer with loose connective tissue that is partly covered by a mesothelial cell layer of the peritoneum .
It should be noted that the whole GI tract has a complex nerve and ganglial network consisting of autonomic and sensory neurones in addition to a separate enteric nervous system. Altogether, this amounts to approximately a fifth of the size of the CNS in man Citation[1,2].
Some pathological conditions
During chronic inflammation a remodeling of the tissues may occur. A chronic ulcer is in principle an unstable equilibrium between noxious influences degrading the surface of the mucosa and local regenerative processes. If the mucosa is unable to regenerate, granulation tissue is formed instead, including inflammatory cells and ingrowth of capillaries. Underneath, a fibrotic tissue with contractile myofibroblasts may be formed, leading to the strengthening of the wall and gradual reduction of the size of the ulcer. Depending on the extent and depth of the ulcer, the regeneration may lead to increased thickness of the gut wall, where collagen fibrous tissue may give altered echoic properties. Both ulcers of the stomach and in the small and large intestine may be accompanied by such tissue remodeling. However, the type of inflammation may vary. In Crohn’s disease, in the small or large intestine, deep ulcer clefts surrounded by extensive inflammation with granulation tissue and fibrosis are common, leading to increased thickness of the whole wall. By contrast, ulcerative colitis is accompanied by more superficial and broader ulcers together with hyperplasia of the adjacent mucosa. Remaining mucosa near to ulcers may protrude as so-called pseudopolyps. In addition, lymphoid hyperplasia is often present in the submucosa. In the small intestine, a wall thickness above 3 mm and in the colon from 2 to 3.5 mm in the proximal and distal end, respectively, are considered as pathologic Citation[3].
Tumor diagnostics is another important area for imaging. For example in colon cancer formation, approximately half of the cases occur through different sizes of polyps or adenomas with increasing degrees of atypia and genetic aberrations, ending with an invasive tumor. In the colon, the invasive process is considered to be slow, whereby early diagnostics may be highly profitable. By contrast, adenocarcinomas of the lower esophagus and gastric wall tend to invade more rapidly and metastasize early. In addition, these tumors often show diffuse infiltration of tumor cells with general thickening of the wall, whereby a localized tumor may be difficult to discern.
A particularly high diagnostic potential is provided when imaging techniques are combined with site-directed biopsies where morphological diagnostics are used together with immunohistological and molecular methods, which only require small amounts of tissue.
Ultrasound imaging of the GI wall
The GI wall has long been overlooked during abdominal US. In fact, the GI tract is often looked on as a nuisance by ultrasonographers because air within the lumen obscures ultrasound imaging of other intra-abdominal organs.
Early sonographers observed that the wall of the GI tract can be seen with transabdominal US in some situations. For example, in a thin patient with a fluid-filled stomach, a layered structure can be imaged in the healthy stomach and in patients with peptic ulcer disease Citation[4]. Water can even be introduced in to the colon by enema to facilitate transabdominal US delineation of pathological thickening as seen in ulcerative colitis or colorectal cancer Citation[5–7]. A layered structure can also be visualized and is helpful in the diagnosis of acute appendicitis Citation[8,9].
The advent of endoscopic US (EUS) in the mid-1980s brought new attention to the use of ultrasound to image the GI tract wall. By taking the US transducer inside the body, and therefore close to the GI wall, higher frequency EUS transducers with their attendant-improved resolution led to a more detailed delineation of the GI wall.
Interpretation of GI ultrasound images
Early endosonographers noted that the GI wall usually demonstrated five layers, when examined under ideal circumstances with 7.5- to 12-MHz US transducers. The anatomic correlates of these layers were demonstrated by careful comparison of US images of excised human GI tissue to the histology of the exact area of tissue Citation[10].
An understanding of these high-frequency US images requires a basic knowledge of what causes US echoes. Most of these echoes are produced by the interaction of the US beam with the tissue itself. For example, collagen and fat are very reflective of the US beam and therefore produce bright echoes on images. This is why the submucosa and subserosal areas of the GI wall are so echoic. The other source of echoes is at the boundaries between tissues of different acoustical properties, the so-called interface echoes. This thin line of echoes at interfaces adds or detracts thickness caused by the echoes produced by the tissue itself.
The first layer seen on imaging from within the GI tract using ultrasound endoscopes is echoic and is caused by the interface between water in the lumen and the mucosal surface. This layer is less visible when the US transducer, or water-filled balloon around the transducer, is placed directly against the mucosa. The next layer of the GI wall is echo poor or hypoechoic and corresponds to the rest of the mucosa. The middle layer of the five-layered GI wall is echoic, mostly due to the submucosa. The fourth layer is hypoechoic, mostly due to the muscularis propria. The outer or fifth layer is echoic, due to subserosal or, in the esophagus, adventitial fat ( & ).
When precise measurements of the histologic layers were compared with measurements of the layers on US images, it was found that the third layer on US images was slightly thicker than the corresponding histologic submucosa and that the fourth layer on US images was slightly thinner than the histologic muscularis propria. This is due to the interface echo stripe from the boundary between the submucosa and the muscularis propria that adds thickness to the submucosal layer on the images and detracts from the thickness of the muscularis propria layer. The thickness of this interface echo is related to the axial resolution of the US transducer and is only approximately 300 µ with the high-frequency transducers used in ES Citation[10]. The muscularis mucosae also adds thickness to the third US layer, as the normal muscularis mucosae is thinner than the interface echoes and therefore is obscured by this interface echo between the lamina propria and the muscularis mucosae, which then blends with the echoes from the underlying submucosa Citation[11]. These small discrepancies from histology have not proved to be clinically important in the interpretation of EUS images of the GI wall.
When even higher frequency transducers such as 20-MHz US probes are used, additional layers can be seen in the normal GI wall. An additional echoic line is sometimes seen in the middle of the fourth hypoechoic layer. This is caused by fibrous tissue that may be present between the inner circular and outer longitudinal components of the muscularis propria or from an interface echo between these two muscle layers.
The muscularis mucosae can also be distinguished as a separate layer when it is thickened and when high-frequency transducers are used. In this situation, there are actually two additional layers at the bottom of the mucosal layer. There is an echoic line at the bottom of the mucosa due to the interface of the lamina propria and the muscularis mucosae, and then another hypoechoic layer deep to this interface echo from the muscularis mucosae itself Citation[11,12]. This additional set of layers from the muscularis mucosae has not yet been shown to aid in clinical diagnosis or cancer staging.
New US probes operating with high frequencies have improved the ability for transabdominal imaging of the GI wall. Nylund et al. recently imaged the GI tract and measured GI wall thickness in healthy subjects using 8- and 12-MHz transducers Citation[13]. The mean wall thickness ranged from 0.9 to 1.2 mm in different areas from the jejunum to the sigmoid colon. The thickness of the gastric antrum and duodenum was 2.1 and 1.6 mm, respectively. Imaging and measurement of individual GI wall layers is also possible. With an anterior access, however, the posterior wall of the GI tract is often obscured by bowel content and may thus be difficult to visualize.
Clinical applications of GI ultrasound imaging
The ability of high-frequency US to image the different histologic layers of the GI tract has helped the GI endoscopist in the differential diagnosis of intramural masses in the GI tract and also in the staging of GI cancer.
The echo pattern and the location of intramural GI masses contribute to a differential diagnosis of the cause of the mass Citation[14,15]. Some findings are sufficient for diagnosis, such as an anechoic cyst or echoic third layer lipoma, whereas other findings only narrow the differential diagnosis. For example, hypoechoic third or fourth layer masses can be from a number of underlying causes with varying clinical significance. The accuracy of EUS imaging alone is only approximately 50% in diagnosing these masses, indicating the need for tissue sampling to confirm the diagnosis Citation[16]. The various causes of intramural GI masses and their location and echo pattern are listed in .
The T stage of the tumor node metastasis cancer staging for GI cancers refers to the depth of invasion of the cancer into the GI wall. The ability of ultrasound to image the GI wall has made ES the most accurate imaging modality for T staging of esophageal, gastric and rectal cancer Citation[17–19]. Numerous clinical studies have shown that EUS is approximately 90% accurate in the T staging of GI cancers.
Sonoelastography
A major challenge when diagnosing lesions in the GI wall, as in many other organs, is to distinguish between malignancy and benign conditions, such as adenomas, myomas, ulcers or inflammatory changes. In general, tissue sampling is necessary to prove malignancy in cases of nonadvanced neoplasms. Recently, new ultrasound-based elastography methods have been introduced with the potential to map distribution of tissue elasticity thus revealing harder or softer portions within a lesion or in relation to adjacent unaffected reference tissue. Two different algorithms are currently available, one quantitative shear wave tracking system and a qualitative or semiquantitative method to map strain distribution Citation[20–23]. Strain imaging is based on changes in radiofrequency signals reflecting strain or deformation when stress is applied on the tissue. Malignancy is generally supposed to increase tissue hardness owing to a fibrogenic infiltration process called desmoplasia Citation[24]. In commercially available elastography facilities, the elastogram can be superimposed on B-mode US images, thus indicating areas with increased suspicion of malignancy. The first clinical experience with elastography is promising, but its relevance and accuracy in diagnosing GI wall lesions has to be further evaluated .
Motility & biomechanics
Abnormal GI motility may cause symptoms that are difficult to diagnose and characterize. Manometry has been widely used in the examination of GI contractions, but this method may give unreliable registrations because incomplete contractions sometimes remain undetected. However, real-time ultrasound can provide information about GI tract motility by visualizing contractions and transport of bowel content. Thus, both transabdominal and intraluminal US have gained increasing interest for studying biomechanical function of the GI tract using established, as well as some new, experimental ultrasound modalities . Thus, gastroduodenal motility and gastric emptying have been studied using both 2D and 3D B-mode US and Doppler techniques. Comparison with intragastric manometry has demonstrated that ultrasound gives more detailed information about gastric contractions and can simultaneously also display the appearance of the individual wall layers Citation[25–27]. A multimodal device that can be inserted into the esophageus combining bag distension, manometry, high-frequency intraluminal ultrasound, laser Doppler flowmetry and symptom registration has been developed. This device was applied in examining circulatory aspects and simultaneous registration with biomechanics for investigating pain on bag distension in the esophagus. Pain elicited by bag distension correlated significantly with biomechanical parameters of passive wall stress and strain Citation[28].
Endosonography allows detailed information about GI wall motility to be obtained by the application of high-frequency ultrasound Citation[29]. Taniguchi et al. recorded changes occurring in the layers of the sheep esophageal wall during swallowing using a 20-MHz M-mode US probe and could demonstrate structural changes occurring separately in the esophageal wall layers during peristalsis Citation[30]. Ultrasound registrations reflecting changes in the thickness of the individual esophageal wall layers were compared simultaneously with corresponding changes in intraluminal pressure. Miller and coworkers combined manometry with a 20-MHz ultrasound transducer and were able to correlate wall thickness, luminal diameter and contractive activity in healthy volunteers Citation[31].
High-frequency intraluminal US also has the potential to monitor wall thickening and other changes such as contractions of the gut in real time in response to provocation with food allergens. Arslan et al. investigated duodenum with high-frequency US to study wall layers and motility during allergen provocation Citation[32]. A 20-MHz miniature ultrasound probe was inserted through a nasoduodenal tube and ultrasound examination was performed before and after provocation. Clinical symptoms were recorded, and the thickness of mucosa, submucosa and muscularis propria was measured directly on ultrasonographic images before and after allergen provocation. Endosonographic changes were observed in 15 of the 20 patients. Mucosal thickening, changes in the number of wall layers and sustained contractions of duodenum were observed in some patients after provocation.
The GI wall echogenicity and layer structure can be changed with increasing pressure generated by an ultrasound transducer. This may be particularly important in ES where the probe is often placed in direct contact with the GI wall Citation[33].
Strain rate imaging (SRI) is a Doppler ultrasound method that so far has mainly been used in cardiology to assess the contractility of the myocardium. Recently, this technique has also gained increasing interest in examination of gastric motor function and may be a promising technology in studying active deformation of the GI wall. However, SRI is challenged by several factors as the GI wall is very thin, has a layered structure with individual elastic properties and the contraction activity is relatively slow. Nevertheless, studies in patients suffering from functional GI disorders, for example functional dyspepsia, have shown that SRI has the potential to becom a method, that can help in the evaluation of symptoms caused by disturbed GI motor function Citation[34,35].
Contrast-enhanced US
High-frequency, high-resolution US allows imaging of the bowel wall and small intramural vessels (diameter <0.5 mm), and Doppler US is a widely used technique that can provide important information about GI wall vascularity Citation[36–38]. The evaluation of vascularity is of major importance in estimating the viability of tissue, characterizing inflammation, for follow-up during treatment and to define tumor-induced angiogenesis.
Ultrasound can be used to evaluate inflammatory bowel disease, and if new ultrasound-based techniques are applied, additional information about mural perfusion can be obtained . It has been shown that there is a difference between normal and inflamed bowel wall regarding several perfusion parameters Citation[39]. Contrast harmonic imaging with quantitative time intensity curve analysis has shown promise in monitoring patients with active Crohn’s disease Citation[40]. Furthermore, harmonic flash echo imaging, which is an intermittent scanning technique using contrast for the evaluation of GI wall microperfusion, may be helpful in predicting the need for surgery if loss of GI wall stratification and rich perfusion are seen Citation[41]. When ultrasound contrast agents are injected into the blood stream, imaging of tissue perfusion is facilitated. The new generation microbubble contrast agents (<6 µm) that stay in the bloodstream for minutes can depict GI wall perfusion and thus have the ability to discriminate between acute and chronic inflammation Citation[42].
In general, the ultrasound contrast agents are very safe but should not be used in pregnancy. Anaphylactoid reactions are rare and no nephrotoxic effects have been encountered Citation[43].
3D & 4D US
3D US images are assumed to be easier to understand and communicate than the mental reconstruction of several 2D images . 3D techniques are currently under development for most imaging modalities and 3D US may be applied for improved recognition of the GI anatomy and pathological lesions. Advanced software programs containing different rendering or other reconstruction algorithms are used for postprocessing and display of 3D ultrasound data, which can be studied using anyplane slicing, segmentation and volume calculation Citation[44,45]. Increased data power and reduced processing time allow 3D imaging in real time (4D) making dynamic display of the individual GI wall layers and gut motility possible.
US-guided interventions in the GI wall
High-frequency US can image soft tissue on a submillimeter resolution level and is theoretically well suited for detailed preoperative diagnosis of lesions to be treated through the endoscope or by minimal invasive surgery. Hence, US can guide interventions indirectly by providing detailed diagnostic information. GI tract interventions can also be guided directly by US as puncture needles or other equipment can be monitored in real time when operated in the US scanning plane. Interventional procedures in the GI wall can be performed with support from external US probes, but procedures targeted at small wall lesions are most often carried out through the endoscope using EUS. Dedicated EUS instruments have been developed for this purpose with a longitudinally directed scanning plane in the prolongation of the biopsy channel.
Exact staging and tumor delineation may be wanted prior to endoscopic mucosal resection of early GI cancer or other intramural tumors in the GI tract as well as snare polypectomy. EUS has been introduced as a useful tool in this regard, but its role in endoscopic mucosal resection has been disputed, as well as in endoscopic treatment of Barrett’s esophagus with high-grade dysplasia, because of limited accuracy (generally less than 70%) in defining submucosal tumor infiltration Citation[46,47].
Most US probes, including electronic EUS instruments, have Doppler facilities allowing exact identification and localization of vessels, thus improving safety during endoscopic interventions in the GI tract. Doppler US may provide important information on tissue vascularization and help to differentiate between cystic and vascular structures Citation[48]. Doppler US can also be helpful in the diagnosis and evaluation after endoscopic treatment of varices and vascular malformations in the GI wall Citation[49,50].
EUS-guided fine-needle aspiration (EUS-FNA) is the most common US-guided intervention in the GI tract. As the needle can be monitored in real time, a short, safe and effective puncture route can be established for sampling of cells or fluid . For larger lesions, tissue samples for histological examination can be accomplished by the application of EUS-guided Trucut biopsy. Most frequently, these interventions are aimed at structures outside the visceral tract, such as the pancreas or mediastinal lymph nodes, but they can also be targeted at intramural lesions, such as tumors or cysts. Early studies on EUS-guided fine-needle aspiration indicated that the diagnostic yield from intramural lesions was lower when compared with extravisceral tumors and lymph nodes Citation[51].
If a therapeutic agent is delivered directly to a target area the local concentration of a drug can be increased by reducing systemic toxicity Citation[52]. Ultrasound can be applied to guide and achieve such deposition of small elements carried in small bubbles in the blood. A shell of microbubbles must be chemically and mechanically designed making specific treatment possible, for example delivering genes, drugs or other therapeutic agents to a specific area Citation[53]. If the area is exposed to ultrasound with sufficient energy, the cells may allow transmembrane uptake of microbubbles and the therapeutic molecules can be released Citation[54,55]. Thus, the cellular uptake of a molecule is facilitated by ‘opening up’ the cell membrane for a short period (sonoporation).
Expert commentary
Transabdominal and intraluminal high-frequency US can provide a detailed image of the GI wall, visualizing wall layers and small intramural structures. Thus, GI wall pathology can be imaged in great detail and detected at an early stage, and follow-up during treatment is possible using a patient-friendly technique. US-guided interventions (e.g., biopsy or endomucosal resection), biomechanics and motility studies, 3D US, contrast-enhanced US, SRI, sonoelastography and combination with molecular imaging and nanotechnology are some of the improvements and new techniques with a promising future in the diagnosis and treatment of disorders of the GI wall.
Five-year view
Several imaging techniques (e.g., US, computed tomography, MRI and PET) are currently available for imaging of the GI wall. High-frequency US, with the safety and patient-friendly characteristics of ultrasound, can resolve small details in the GI wall allowing a detailed evaluation of small lesions of the GI wall in a clinical setting. Technical improvements of hardware and continuous software development have enabled imaging of small structures and even cellular structures. The combination of endoscopy and high-frequency ultrasound (EUS) has given new possibilities in imaging of the GI mucosal surface and the subepithelial GI wall. Real-time 3D imaging, functional imaging using Doppler technology or other methods for tissue characterization, ultrasound-guided minimal invasive treatment and integration with other imaging modalities are expected to evolve in the time to come. Combined monitoring and imaging techniques have been developed to study GI motility including dynamics in individual GI wall layers. Improved tissue characterization using imaging techniques can possibly limit the need for biopsies in the future. Sonoelastography is a new technique that can visualize changes in tissue hardness, but its ability to differentiate between benign and malignant lesions has yet to be established. Another new technology not yet established is haptic imaging (virtual ultrasound) where ultrasound images are translated into a tactile system allowing manual virtual palpation of imaged structures. Ultrasound can be combined with emerging microbubble techniques allowing transport of diagnostic (e.g., contrast-enhanced ultrasound) as well as therapeutic (nanoparticles) agents to defined areas of interest. In conclusion, ultrasound techniques are continuously being improved and developed and will remain an important clinical imaging tool for the diagnosis and treatment of diseases of the GI tract.
Table 1. Ultrasonography layer location and echogenicity of gastrointestinal intramural masses.
Key issues
• Ultrasound is a safe modality that can image details of the gastrointestinal wall with high spatial resolution in real time.
• It is well suited to guide interventional procedures in the gastrointestinal tract.
• Current development of ultrasound techniques includes high-frequency and 3D imaging, Doppler facilities, air-bubbled contrast agents, tissue characterization methods and dynamic studies.
Financial & competing interests disclosure
This work was generously financially supported by a grant from Olav Raagholt and Gerd Meidel Raagholts Research Foundation, Norway (to S Ødegaard and LB Nesje). The authors have no other relevant affiliations or financial involvement with any organization or entity with a financial interest in or financial conflict with the subject matter or materials discussed in the manuscript apart from those disclosed.
No writing assistance was utilized in the production of this manuscript.
References
- Kierszenbaum AL, Tres L. Histology and Cell Biology. An Introduction to Pathology (3rd Edition). Elsevier Saunders, PA, USA (2011).
- Underwood JCE, Cross SS, Britton R. General and Systematic Pathology (5th Edition). Churchill Livingstone, Edinburgh, UK (2009).
- Fraquelli M, Colli A, Casazza G et al. Role of US in detection of Crohn disease: meta-analysis. Radiology 236(1), 95–101 (2005).
- Joharjy IA, Mustafa MA, Zaidi AJ. Fluid-aided sonography of the stomach and duodenum in the diagnosis of peptic ulcer disease in adult patients. J. Ultrasound Med. 9(2), 77–84 (1990).
- Limberg B. Diagnosis of acute ulcerative colitis and colonic Crohn’s disease by colonic sonography. J. Clin. Ultrasound 17(1), 25–31 (1989).
- Limberg B. Diagnosis and staging of colonic tumors by conventional abdominal sonography as compared with hydrocolonic sonography. N. Engl. J. Med. 327(2), 65–69 (1992).
- Kimmey MB, Wang KY, Haggitt RC, Mack LA, Silverstein FE. Diagnosis of inflammatory bowel disease with ultrasound. An in vitro study. Invest. Radiol. 25(10), 1085–1090 (1990).
- Schwerk WB, Wichtrup B, Rothmund M, Rüschoff J. Ultrasonography in the diagnosis of acute appendicitis: a prospective study. Gastroenterology 97(3), 630–639 (1989).
- Spear R, Kimmey MB, Wang KY, Sillery JK, Benjamin DR, Sawin RS. Appendiceal US scans: histologic correlation. Radiology 183(3), 831–834 (1992).
- Kimmey MB, Martin RW, Haggitt RC, Wang KY, Franklin DW, Silverstein FE. Histologic correlates of gastrointestinal ultrasound images. Gastroenterology 96(2 Pt 1), 433–441 (1989).
- Ødegaard S, Kimmey MB. Location of the muscularis mucosae on high frequency gastrointestinal ultrasound images. Eur. J. Ultrasound 1, 39–50 (1994).
- Nylund K, Leh S, Immervoll H et al. Crohn’s disease: comparison of in vitro ultrasonographic images and histology. Scand. J. Gastroenterol. 43(6), 719–726 (2008).
- Nylund K, Hausken T, Ødegaard S, Gilja OH. Gastrointestinal wall thickness and demographic factors in healthy subjects. Gut. 60(Suppl. 3), A293 (2011).
- Hwang JH, Kimmey MB. The incidental upper gastrointestinal subepithelial mass. Gastroenterology 126(1), 301–307 (2004).
- Hwang JH, Rulyak SD, Kimmey MB; American Gastroenterological Association Institute. American Gastroenterological Association Institute technical review on the management of gastric subepithelial masses. Gastroenterology 130(7), 2217–2228 (2006).
- Hwang JH, Saunders MD, Rulyak SJ, Shaw S, Nietsch H, Kimmey MB. A prospective study comparing endoscopy and EUS in the evaluation of GI subepithelial masses. Gastrointest. Endosc. 62(2), 202–208 (2005).
- Puli SR, Reddy JB, Bechtold ML, Antillon D, Ibdah JA, Antillon MR. Staging accuracy of esophageal cancer by endoscopic ultrasound: a meta-analysis and systematic review. World J. Gastroenterol. 14(10), 1479–1490 (2008).
- Puli SR, Reddy JB, Bechtold ML, Antillon MR, Ibdah JA. How good is endoscopic ultrasound for TNM staging of gastric cancers? World J. Gastroenterol. 14(10), 4011–4019 (2008).
- Puli SR, Bechtold ML, Reddy JB, Choudhary A, Antillon MR, Brugge WR. How good is endoscopic ultrasound in differentiating various T stages of rectal cancer? Meta-analysis and systematic review. Ann. Surg. Oncol. 16(2), 254–265 (2009).
- Ophir J, Céspedes I, Ponnekanti H, Yazdi Y, Li X. Elastography: a quantitative method for imaging the elasticity of biological tissues. Ultrason. Imaging 13(2), 111–134 (1991).
- Giovannini M, Hookey LC, Bories E, Pesenti C, Monges G, Delpero JR. Endoscopic ultrasound elastography: the first step towards virtual biopsy? Preliminary results in 49 patients. Endoscopy 38(4), 344–348 (2006).
- Saftoiu A, Vilmann P, Hassan H, Gorunescu F. Analysis of endoscopic ultrasound elastography used for characterisation and differentiation of benign and malignant lymph nodes. Ultraschall Med. 27(6), 535–542 (2006).
- Hoyt K, Castaneda B, Parker KJ. Two-dimensional sonoelastographic shear velocity imaging. Ultrasound Med. Biol. 34(2), 276–288 (2008).
- Abbas O, Mahalingam M. Desmoplasia: not always a bad thing. Histopathology 58(5), 643–659 (2011).
- Bolondi L, Bortolotti M, Santi V, Calletti T, Gaiani S, Labò G. Measurement of gastric emptying time by real-time ultrasonography. Gastroenterology 89(4), 752–759 (1985).
- Hausken T, Odegaard S, Matre K, Berstad A. Antroduodenal motility and movements of luminal contents studied by duplex sonography. Gastroenterology 102(5), 1583–1590 (1992).
- Hveem K, Gregersen H. Simultaneous recordings of gastric motility by ultrasound, scintigraphy and manometry. In: Basic and New aspects of Gastrointestinal ultrasonography. Ødegaard S, Gilja OH, Gregersen H (Eds). World Scientific Publishing, Singapore, 189–202 (2005).
- Hoff DA, Gregersen H, Odegaard S et al. A multimodal laser Doppler and endosonographic distension device for studying mechanosensation and mucosal blood flow in the esophagus. Neurogastroenterol. Motil. 18(3), 243–248 (2006).
- Odegaard S, Nesje LB, Hoff DA, Gilja OH, Gregersen H. Morphology and motor function of the gastrointestinal tract examined with endosonography. World J. Gastroenterol. 12(18), 2858–2863 (2006).
- Taniguchi DK, Martin RW, Trowers EA, Dennis MB Jr, Odegaard S, Silverstein FE. Changes in esophageal wall layers during motility: measurements with a new miniature ultrasound suction device. Gastrointest. Endosc. 39(2), 146–152 (1993).
- Miller LS, Liu JB, Colizzo FP et al. Correlation of high-frequency esophageal ultrasonography and manometry in the study of esophageal motility. Gastroenterology 109(3), 832–837 (1995).
- Arslan G, Ødegaard S, Elsayed S, Florvaag E, Berstad A. Food allergy and intolerance: response to intestinal provocation monitored by endosonography. Eur. J. Ultrasound 15(1–2), 29–36 (2002).
- Odegaard S, Kimmey MB, Martin RW, Yee HC, Cheung AH, Silverstein FE. The effects of applied pressure on the thickness, layers, and echogenicity of gastrointestinal wall ultrasound images. Gastrointest. Endosc. 38(3), 351–356 (1992).
- Gilja OH, Heimdal A, Hausken T et al. Strain during gastric contractions can be measured using Doppler ultrasonography. Ultrasound Med. Biol. 28(11–12), 1457–1465 (2002).
- Gilja OH, Hatlebakk JG, Odegaard S et al. Advanced imaging and visualization in gastrointestinal disorders. World J. Gastroenterol. 13(9), 1408–1421 (2007).
- Ødegaard S, Kimmey MB, Cheung AHS et al. High frequency endosonography of gastrointestinal arteries: potential and limitations defined in vitro. Eur. J. Ultrasound 33, 313–319 (1995).
- Dietrich CF, Jedrzejczyk M, Ignee A. Sonographic assessment of splanchnic arteries and the bowel wall. Eur. J. Radiol. 64(2), 202–212 (2007).
- Cheung AH, Wang KY, Jiranek GC, Odegaard S, Kimmey MB, Silverstein FE. Evaluation of a 20-MHz ultrasound transducer used in diagnosing porcine small bowl ischemia. Invest. Radiol. 27(3), 217–223 (1992).
- Girlich C, Jung EM, Iesalnieks I et al. Quantitative assessment of bowel wall vascularisation in Crohn’s disease with contrast-enhanced ultrasound and perfusion analysis. Clin. Hemorheol. Microcirc. 43(1), 141–148 (2009).
- Schreyer AG, Finkenzeller T, Gössmann H et al. Microcirculation and perfusion with contrast enhanced ultrasound (CEUS) in Crohn’s disease: first results with linear contrast harmonic imaging (CHI). Clin. Hemorheol. Microcirc. 40(2), 143–155 (2008).
- Kunihiro K, Hata J, Manabe N et al. Predicting the need for surgery in Crohn’s disease with contrast harmonic ultrasound. Scand. J. Gastroenterol. 42(5), 577–585 (2007).
- Migaleddu V, Quaia E, Scano D, Virgilio G. Inflammatory activity in Crohn disease: ultrasound findings. Abdom. Imaging 33(5), 589–597 (2008).
- Piscaglia F, Nolsøe C, Dietrich CF et al. The EFSUMB Guidelines and Recommendations on the Clinical Practice of Contrast Enhanced Ultrasound (CEUS). Update 2011 on non-hepatic applications. Ultraschall. Med. 32, 1–27 (2011).
- Odegaard S, Nesje LB, Molin SO, Gilja OH, Hausken T. 3D intraluminal sonography in the evaluation of gastrointestinal diseases. Abdom. Imaging 24(5), 449–451 (1999).
- Gilja OH, Martin RW. 3D ultrasonography in gastroenterology. In: Basic and New aspects of Gastrointestinal ultrasonography. Ødegaard S, Gilja OH, Gregersen H (Eds). World Scientific Publishing, Singapore, 273–292 (2005).
- Chandrasekhara V, Ginsberg GG. Endoscopic mucosal resection: not your father’s polypectomy anymore. Gastroenterology 141(1), 42–49 (2011).
- Thomas T, Gilbert D, Kaye PV, Penman I, Aithal GP, Ragunath K. High-resolution endoscopy and endoscopic ultrasound for evaluation of early neoplasia in Barrett’s esophagus. Surg. Endosc. 24(5), 1110–1116 (2010).
- Saftoiu A, Vilmann P, Hassan H, Krag Jacobsen G. Utility of colour Doppler endoscopic ultrasound evaluation and guided therapy of submucosal tumours of the upper gastrointestinal tract. Ultraschall Med. 26(6), 487–495 (2005).
- El−Saadany M, Jalil S, Irisawa A, Shibukawa G, Ohira H, Bhutani MS. EUS for portal hypertension: a comprehensive and critical appraisal of clinical and experimental indications. Endoscopy 40, 690–696 (2008).
- Folvik G, Nesje LB, Berstad A, Odegaard S. Endosonography-guided endoscopic band ligation of Dieulafoy’s malformation: a case report. Endoscopy 33(7), 636–638 (2001).
- Wiersema MJ, Vilmann P, Giovannini M, Chang KJ, Wiersema LM. Endosonography-guided fine-needle aspiration biopsy: diagnostic accuracy and complication assessment. Gastroenterology 112(4), 1087–1095 (1997).
- Hung SH, Yeh CK, Tsai TH, Chen T, Chen RC. A simple method for quantifying ultrasound-triggered microbubble destruction. Ultrasound Med. Biol. 37(6), 949–957 (2011).
- Cavalieri F, Zhou M, Ashokkumar M. The design of multifunctional microbubbles for ultrasound image-guided cancer therapy. Curr. Top. Med. Chem. 10(12), 1198–1210 (2010).
- Voigt JU. Ultrasound molecular imaging. Methods 48(2), 92–97 (2009).
- Postema M, Gilja OH. Contrast-enhanced and targeted ultrasound. World J. Gastroenterol. 17(1), 28–41 (2011).
- Atlas of Endoscopic Ultrasonography. Ødegaard S, Nesje LB, Gilja OH (Eds). Fagbokforlaget, Bergen, Norway (2007).