Abstract
The current state-of-the-art tests for infection with Mycobacterium tuberculosis – the IFN-γ release assays – rely on accurate measurement of the cytokine IFN-γ. Many other potential biomarkers are expressed in concert with IFN-γ, and IP-10 in particular has shown promising results. IP-10 is produced in large amounts, allowing for the development of new and simplified test platforms, such as lateral flow. In this review, we summarize the results of 22 clinical studies exploring the use of IP-10 as an alternative marker to IFN-γ. The studies report that diagnostic accuracy of IP-10 is on par with IFN-γ, but also that IP-10 may be more robust in young children and in HIV-infected individuals with low CD4 cell counts. We conclude the review by presenting limitations of the published works and outline recent developments and future directions.
(A) T cells recognize Mycobacterium tuberculosis-specific peptides on the MHC molecules on the surface of the APCs, and this interaction activates the T cells and also the APCs. (B) T cells and the APCs secrete a series of proinflammatory cytokines, which further activates the APCs and leads to (C) IP-10 secretion from activated APCs.
APC: Antigen-presenting cell; IFN: Interferon; TCR: T-cell receptor.
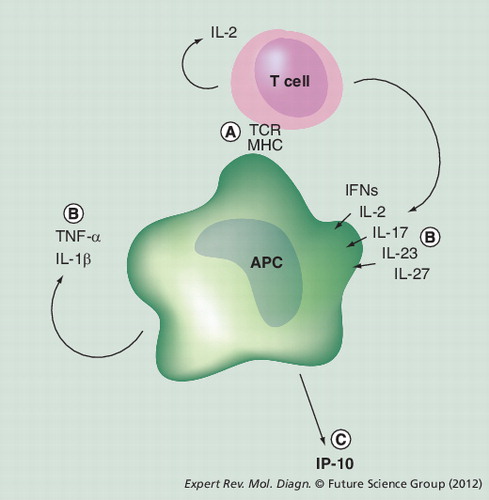
Introduction
Mycobacterium tuberculosis is a virulent intracellular pathogen that survives in macrophages, even in the presence of an intact adaptive immune response. The bacillus has coevolved with primates for thousands of years, which is probably the reason for its success Citation[1]. With approximately a third of the world’s population showing immune recognition of M. tuberculosis antigens, an estimated rate of 8 million new cases of active tuberculosis (TB) and at least 2 million TB-related deaths annually, infection with this bacillus is pandemic Citation[2]. Although the M. tuberculosis genome has been sequenced, the proteome scrutinized and many potential immunogenic proteins identified Citation[1], it has proven difficult to develop an efficient vaccine to substitute the bacillus Calmette–Guérin (BCG) vaccine Citation[3]. Consequently, the global strategy to control the disease centers on identifying and treating active cases to prevent further spread of the bacillus. In resource-rich areas screening and targeted treatment of patients at risk of developing active TB, such as recently exposed children, HIV-infected individuals and patients starting on immunosuppressive treatment, has been mandatory Citation[2,4], and in high endemic regions, chemoprophylaxis is now recommended for all exposed children <5 years of age and HIV-positive individuals Citation[301].
IFN-γ release assays
A decade ago a new generation of tests specific for M. tuberculosis infection were introduced. Two tests are currently commercially available: the whole-blood/ELISA-based QuantiFERON®-Gold In Tube test (QFT-IT; Cellestis/Qiagen, CA, USA) and the PBMC/ELISPOT based T-SPOT®.TB test (Oxford Immunotec, Abingdon, UK). These IFN-γ release assays (IGRAs) are in essence cell-mediated immune response (CMI) assays relying on measurement of T-cell responses in vitro to peptide antigens specific for M. tuberculosisCitation[5,6]. The IGRAs are based on five essential components: M. tuberculosis-specific antigens; antigen-presenting cells (APC); T cells; an incubation step; and a readout biomarker of immune recognition (Box 1).
In BCG-unvaccinated otherwise healthy individuals the IGRAs perform similarly to the tuberculin skin test (TST), but the increased specificity has proven superior in BCG-vaccinated individuals and patients with exposure to, or infection with, non-tuberculous mycobacteria (NTM) Citation[5,7–9]. Although IGRAs appear to have higher sensitivity than the TST in immunosuppressed patients, such as persons with HIV infection Citation[9,10] and in the very young and very old, IGRA sensitivity is still compromised in these patients Citation[11–15,302]. The clinical application of IGRAs is a matter of debate Citation[16,302]. New approaches are needed to improve the methods hereunder evaluation of alternative biomarkers.
The aim of this review is to provide an overview of current knowledge on the potential of IP-10 as a diagnostic biomarker for infection with M. tuberculosis and to describe the potential of IP-10 in future improvements of the IGRA.
Discovery of IP-10 as a biomarker for TB infection
In 2006, we screened a series of cytokines and chemokines for their potential as markers of CMI responses to specific M. tuberculosis antigens in QFT-IT supernatants, and found that IP-10 is expressed in very high amounts in patients with active TB, but not in unexposed controls Citation[17]. Further evidence has since substantiated this discovery and >40 additional biomarkers have been screened for diagnostic potential. Of all investigated markers, it appears that IP-10 is the most consistently expressed in response to antigen challenge, rendering it the most promising alternative marker to IFN-γ Citation[18–27] and a promising means of improving IGRAs Citation[28–34].
The immunological basis of IP-10 as a readout marker in CMI assays
IP-10 is a small chemokine expressed by APCs Citation[35,36] and a main driver of proinflammatory immune responses. IP-10 is expressed by cells infected with viruses and bacteria, but can also be induced at high levels as part of the adaptive immune response. In this case, IP-10 secretion is initiated when T cells recognize their specific peptide presented on the APC . IP-10 secretion appears to be driven by multiple signals, mainly T-cell-derived IFN-γ, but also IL-2, IFN-α, IFN-β, IL-27, IL-17, IL-23, and autocrine APC-derived TNF-α and IL-1β. TNF-α is a weak IP-10 inducer per se, but a potent synergistic inducer when acting with the interferons Citation[37–46]. Because of the many different pathways that can lead to IP-10 secretion, IP-10 can be considered a downstream marker to typical readout markers in CMI assays, such as IL-2 and IFN-γ Citation[47,48].
An important distinction of IP-10 compared with the typical readout markers is that it is expressed in much higher levels, and as IP-10 is a 2.5-times smaller molecule than IFN-γ (7.4 vs 18.8 kD), this difference is even larger at the molecular level when determined with solid-phase technologies (such as ELISA). Although the difference in magnitude is very large, the fold change between signal (antigen response) and noise (nil level) appears to be comparable between the two markers. Therefore, IP-10 is a promising alternative marker in CMI assays.
Review of published studies
Studies in active TB patients, healthy controls & exposed individuals
We identified 12 studies in adults and seven studies in children. The studies in adults comprised a total of 645 adult TB patients and 583 controls from both high- (India, Tanzania, Guinea Bissau and South Africa) and low- (Denmark, Sweden, Finland, Switzerland, Greece, Italy, Spain and USA) prevalence settings . All adult studies confirmed that IP-10 is specifically induced in response to TB-specific antigens in patients with active TB compared with controls Citation[17,19,20,22,49–55]. Seven studies set or used cutoffs for evaluation of derived IP-10 test results Citation[20,51–56], of which five reported result distribution and performed head-to-head comparisons with the QFT-IT Citation[51–55]. These five studies (two from India, one from Denmark, one from Tanzania and one multicenter study from countries in the European Union) comprise a total of 435 patients with active TB and report comparable IP-10 test and QFT-IT positivity rates (74–91 vs 79–100%, respectively) as well as good agreement between the two tests (data available for two studies only; agreement 73–89%; κ: 0.43–0.57) Citation[51–55]. Positivity rates increased significantly when IP-10 and QFT-IT test results were combined (available for four studies only; positivity rates increased to 86–93%) Citation[52–55].
Three studies compared IP-10 test and QFT-IT performance in healthy controls Citation[52–54]. In two studies from a low-prevalence settings (Denmark and Italy), out of 187 nonexposed healthy controls, only 2% were found to be IP-10 test positive (specificity 98%) and 0% were QFT-IT positive (specificity 100%) and there was no indication of cross-reactivity with BCG vaccination Citation[52,53]. The third study, performed in India, included 100 controls and reported positive results in 52% by the IP-10 test and 48% by the QFT-IT, probably reflecting the high prevalence of latent TB infection Citation[54].
Studies of sensitivity to latent TB infection are difficult to interpret owing to the lack of a test-independent gold standard, but can be attempted by using different measures for risk of exposure. The European multicenter study reports such results for the IP-10 test and the QFT-IT in 175 patients with suspected TB but who were finally diagnosed with another disease . In this group, the IP-10 test and the QFT-IT were found to be positive in 35 and 27% of patients, respectively (p < 0.05). The increased IP-10 positivity rate could represent either superior sensitivity or impaired specificity of the IP-10 test compared with the QFT-IT. It was shown that the presence of risk factors for TB infection (exposure history, prior TB and stay in a highly endemic area) increased the adjusted odds ratios for having a positive test result from both tests, with no significant difference between tests Citation[53], and larger studies in groups with varying degrees of exposure are therefore needed to clarify this issue.
In conclusion, current evidence suggests comparable accuracy of IP-10 based tests with the QFT-IT in patients with active TB and healthy controls, as well as in TB-exposed individuals.
Children
TB is a major contributor to childhood morbidity and mortality Citation[57,58] with children <5 years of age at highest risk and 40–50% of infected infants developing disease within 1–2 years. Childhood TB is notoriously difficult to diagnose owing to its pauci-bacillary nature and the limited performance of existing tools Citation[59,60].
In contrast to adults, where the use of IGRAs in diagnosing active TB is not recommended, IGRAs may be useful in the diagnostic work-up of active TB in children with negative smear microscopy and culture results. In this case, a positive IGRA in a child indicates recent exposure, and therefore an increased risk of developing active TB.
The reported performance of IGRAs in children is not consistent with varying rates of sensitivity, specificity and proportion of indeterminate results Citation[7,8]; differences that are probably due to heterogeneity in the study population, severity of TB, malnutrition and comorbidities Citation[7,302].
Until now, seven studies comprising 1408 children aged 1–17 years from high- (Nepal, Brazil, Nigeria and Ethiopia) and low-endemic countries (USA, UK and Germany) have assessed IP-10 as a tool for the diagnosis of latent TB infection or active TB .
As in adults, the IP-10 levels after antigen stimulation were higher compared with IFN-γ levels, and IP-10 levels in unstimulated samples were generally higher in children with active or latent TB compared with children with no disease and no signs of infection with M. tuberculosis, as determined by exposure risk or a positive TST or QFT-IT test.
Antigen-stimulated IP-10 responses were higher in children with active TB and in high-risk groups. The IP-10 test positivity rate was assessed in three studies comprising 40 children with active TB (22 children from Ethiopia Citation[61] and 11 from Germany Citation[62] and seven from the USA Citation[63]). Two studies found a high rate of positive responders comparable to adult studies of 82% (18/22) Citation[61] and 91% (10/11) Citation[62], whereas Lighter et al. found a lower positivity rate of 43% (3/7) Citation[63].
In 14 children from Germany with latent TB, defined as a positive TST or QFT-IT result, the IP-10 positivity rate was 86% Citation[62]. In a study from Nigeria, the IP-10 test and the QFT-IT detected comparable proportions of positive children in high-, moderate- and low-risk groups Citation[64]. In unexposed or low-risk controls, the specificity of IP-10 varied depending on the setting; in 44 children from low-endemic countries Citation[62,63] and in 86 children with negative TST and QFT-IT from Ethiopia Citation[61], IP-10 positivity rates were low (0–5%) Citation[61–63], whereas in 23 community controls from Nigeria, the positivity rate was higher (26%) Citation[64], indicating similar specificity to the QFT-IT. Agreement of the IP-10 test and the QFT-IT was assessed in three studies and ranged from 0.50 to 0.96 Citation[25,61,62].
The IGRAs appear to perform suboptimally in children <5 years of age compared with adults and older children Citation[7,65]. As prevalence of latent TB infection increases with age, the impact of age alone on test sensitivity is difficult to interpret and studies addressing the possible influence of age on test performance are needed.
Lighter et al. found that mitogen-induced IFN-γ, but not IP-10 responses, increased with age Citation[63]. Lighter-Fischer et al. found that children <5 years of age with latent TB infection had reduced IFN-γ, but not IP-10, responses to TB antigens Citation[25]. By contrast, Ruhwald et al. found an association between age and rate of positivity rate for the IP-10 test and TST but not for QFT-IT in a group of Nigerian children with different exposure risk Citation[64]. Alsleben et al. found no effect of age on IP-10 mitogen responsiveness in 48 German children also with varying exposure Citation[62].
In children, lymphadenopathy caused by NTM is a differential diagnostic problem and in eight children with NTM infection both QFT-IT, and IP-10 tests were negative, suggesting that IP-10, as IFN-γ, discriminates between M. tuberculosis and NTM infections Citation[62].
In summary, the studies suggests that IP-10 has comparable diagnostic accuracy to QFT-IT for diagnosing TB in children, with some indication that IP-10 may be less affected by age than IFN-γ in the QFT-IT.
HIV-infected individuals
HIV-infected individuals with severe immune suppression have a high risk of progression to active TB disease and would therefore benefit from accurate diagnosis and prophylactic treatment of latent M. tuberculosis infection Citation[66]. However, many studies have shown that the IGRAs, in particular the QFT-IT, render many indeterminate and possibly also false-negative results in HIV-infected individuals with a low CD4 cell count Citation[13,14,65–72]. Therefore, alternative approaches in this group of patients are necessary, but only few studies have evaluated IP-10 for this purpose.
We identified three studies comprising a total of 143 HIV-infected TB patients, and these report rather divergent findings Citation[55,56,73]. Two small studies from India, comprising a total of 78 HIV-infected TB patients, both found a marginally higher IP-10 test positivity rate compared with the QFT-IT (86 vs 61% and 86 vs 70%, respectively) Citation[56,73]. By contrast, a study in 65 HIV-infected TB patients from Tanzania found equal positivity rates for the tests (63% for both), which was significantly lower compared with HIV uninfected individuals (82 and 80%, respectively) Citation[55].
Three studies from India included HIV-infected patients with no signs of active TB. Two studies (n = 208) both reported more positive results for the IP-10 test than the QFT-IT test (87 vs 32% Citation[56] and 45 vs 28% Citation[10], respectively), while one study (n = 50) found no positive results by either test Citation[73].
Interestingly, all of the aforementioned studies in HIV-infected individuals with active TB found that IP-10 positivity rates were less dependent on CD4 cell count than QFT-IT positivity rates were. One large study in 170 otherwise healthy HIV-infected individuals from a TB-endemic setting, found that very low IP-10 responses to mitogens (<200 pg/ml) only occurred in individuals with CD4 cell count <100 cells/µl Citation[55]. However, comparisons of indeterminate results are difficult to make due to the lack of a validated cutoff for an indeterminate IP-10 response (see below). The immunological mechanisms underlying these suggested differences between IFN-γ and IP-10 in HIV-infected patients are as yet unknown, but are probably due to a combination of both the higher magnitude of response and the broader induction profile of IP-10 compared with IFN-γ Citation[37–46]. Sutherland and colleagues recently demonstrated that coincident with the loss of total CD4 count, the TB antigen-specific immune response changed from a polyfunctional CD4 response to a monofunctional IFN-γ or TNF-α CD8 T-cell response Citation[74]. As IP-10 is inducible by a wide panel of CD4 and CD8 T-cell cytokines (particularly IFN-γ and TNF-α Citation[75]), it could be that IP-10 picks up and combines these weaker cytokine responses from the monofunctional cells in a stronger common downstream signal.
In conclusion, the IP-10 test in HIV-infected patients with TB performs slightly better or comparable to QFT-IT, and both tests are negatively affected by advanced HIV infection. In HIV-positive but otherwise healthy high-risk individuals more IP-10 results are positive, a finding that may reflect that the IP-10 test is either more sensitive or less specific in this population. Therefore, although the IP-10 test performance does seem to be impaired in HIV-infected individuals, it may be more robust than the QFT-IT in individuals with low CD4 cell counts. However, current evidence is limited and larger studies are needed.
Immunosuppressive therapy
The use of IGRAs and TST in screening for latent TB infection before initiation of immunosuppression with, for example, TNF-α inhibitors, and also that IGRAs are more specific and in some instances more sensitive than TST in this vulnerable group of patients is well recognized Citation[76]. However, false-negative IGRA results occur, and test performance is impaired, in patients treated with prednisolone and possibly also other drugs used to treat inflammatory diseases Citation[77].
So far, only one study has evaluated IP-10 for diagnosing latent TB infection in patients receiving immunosuppressive treatment Citation[78]. In this study, 52 patients from Taiwan with rheumatoid arthritis (RA) were treated with methotrexate and one or more of the immunosuppressive drugs used for RA; of these, 24 had latent TB (defined by a positive TST). In addition, 18 patients with active TB were included. The study reported that, in contrast to IFN-γ, unstimulated and antigen-dependent IP-10 levels were significantly higher in TST-positive compared with TST-negative results in RA patients, and higher in those with a positive compared with a negative QFT-G result.
Interestingly one patient developed active TB despite chemoprophylaxis, and in this case, unstimulated levels of IP-10 increased until the diagnosis of active TB and declined again during effective treatment Citation[78].
The area under the curve (AUC) for detecting active TB using IP-10 was 0.71 and 0.79 for ESAT-6 and CFP-10 respectively. The AUC for detecting latent TB infection (TST positive) was 0.88 and 0.78, respectively. Interestingly, baseline IP-10 had similarly discriminatory power. IP-10 may be useful for diagnosing active and latent TB in patients receiving immunosuppressive treatment; however, the current data are very limited and further studies are needed.
Limitations of the current studies & technical aspects
One limitation of the studies evaluating diagnostic tests for latent TB infection is the lack of a gold standard. In some of the clinical studies, TST and/or QFT-IT have been used as gold standard, but in order to compare diagnostic accuracy between tests, a gold standard independent of the test under evaluation must be used. For example, if a TB patient has a positive IP-10 but a negative QFT-IT result, the IP-10 response will be deemed false positive if QFT-IT is the gold standard.
Another important issue is the need for a proper measure of indeterminate test. One study defined an arbitrary cutoff for an indeterminate IP-10 test Citation[52], which has also been applied in other studies Citation[10,53,55]. The rates of indeterminate results were, in general, comparable with the IGRAs, but there seemed to be a tendency towards fewer indeterminate and more false-negative results for the IP-10 test, suggesting a need for an adjustment of the cutoff.
The IGRAs utilize phytohemagglutinin (PHA) as mitogen positive control. However, PHA may not be the optimal choice for an IP-10 test. PHA is a powerful inducer of IFN-γ, whereas IP-10 is induced at relatively lower levels. The difference is probably due to the fact that PHA acts directly on the T cells but not on the APCs. PHA-induced IP-10 responses are indirectly induced via T-cell cytokines released into the cell-culture supernatant, leading to APC activation. A more suitable positive control mitogen for an IP-10-based test could be the lectin concavalin A Citation[79], which leads to IP-10 induction through direct activation of the monocytes Citation[80].
A future cutoff for indeterminate test should ideally be based on a cost-effective assessment of the risk of progression in false-negative patients with latent TB and weigh in the required resources needed to retest, as well as counseling of patients with persistently indeterminate tests. Such cutoffs are likely to be setting specific.
A major limitation in this field relates to the technical aspects of the assays used for IP-10 detection. In the 22 clinical studies investigating the diagnostic potential of an IP-10 release assay included in this review, IP-10 has been measured with a variety of assays: from different brands of multiplexing assays to commercial or in-house ELISA kits . Furthermore, samples have been measured in different dilutions that might not have been optimal for the dynamic range of the standard curve . Cutoffs vary from study to study, and as cutoffs are linked both to the readout platform and the sample dilution, a more stringent approach is needed before studies can be compared. Future studies should measure and report IP-10 results in ways that allow comparison between studies. Adherence to Standards for Reporting of Diagnostic Accuracy criteria, and thorough descriptions of sample assay ranges and dilutions, will accelerate the field, as cutoffs will be identified and applied between studies.
Hot topics & unsolved questions
Improving the IGRA using IP-10
The role of IGRAs in diagnosing and managing TB infection and disease is still controversial, and all of these concerns will also need to be addressed for a potential IP-10 test Citation[9,81,82]. Two of the greatest obstacles for the IGRAs are the inability to discriminate between active and latent TB infection, as well as suboptimal sensitivity Citation[83]. In immunocompromised individuals and children, who have the greatest risk of progression to active disease and would benefit the most from an accurate diagnosis of latent TB infection, the IGRA performance is compromised.
Several approaches have been attempted to overcome the shortcomings of the current IGRAs. IP-10 has been shown to be specifically induced, not only in response to the TB-specific antigens used in the QFT-IT and QFT-G Citation[78] tests, but also in response to purified protein derivative Citation[25] and alternative TB antigens, including selected region of difference 1-specific peptides Citation[21,50,51,56], peptides spanning the mycobacterial protein TB10.4 Citation[49], whole M. tuberculosis sonicate Citation[21], cytomegalovirus, HIV Citation[84] and peptides specific for Mycobacterium lepraeCitation[85]. Alternative or additional antigens appear to have the potential for improving IGRA sensitivity Citation[86,87] and enable discrimination between active and latent TB Citation[88–91]; in this context, IP-10 may be a favorable biomarker for measuring responses to novel antigens with limited immunogenicity because of the high levels of biomarker produced Citation[51].
Several studies have demonstrated that the discordance between IP-10 and IFN-γ test results can be utilized by a combination of tests to increase diagnostic sensitivity, apparently without a compromise in specificity Citation[52–55]. Other cytokines and chemokines (including IL-2, TNF-α, MIP-1b and MCP-1) have shown promise as diagnostic markers, but the potential added benefit of these has not yet been systematically assessed. We have studied MCP-2 and found a lower sensitivity of 71% for active TB at 97% specificity in healthy controls, but no significant added effect by combination with either IP-10 or IFN-γ Citation[52]. In a subsequent evaluation in HIV-infected patients with confirmed TB, we observed significant lower sensitivity of MCP-2 compared with IFN-γ and IP-10, and found no added effect by combination [Aabye MG, Ruhwald M, Unpublished Data].
Tweaking of the incubation step, for example by increasing incubation temperature Citation[49], prolonging the incubation period Citation[23,92,93], improving culture conditions with nutrients Citation[201] or addition of proinflammatory cytokines (e.g., IL-12 Citation[85]), blocking of anti-inflammatory cytokines (e.g., IL-10 Citation[49,94]) or adding ‘survival’ cytokines (e.g., IL-7 Citation[95,96]), have also shown potential for augmentation of the antigen-specific immune response.
IGRAs offer some practical advantages compared with the TST. IGRAs can be performed as a day-to-day assay with no need for the patients to return and IGRAs have an objective readout. However, IGRAs do require direct access to a laboratory that can perform the ELISA or a cold chain for transporting samples to the laboratory, which may limit their use, for example, in field studies or in low-resource settings. In this regard, IP-10 offers a significant advantage. The high levels of IP-10 secreted allow for the use of smaller blood volumes, less sample material, as well as simpler readout systems. We have developed a method using dried plasma or whole blood in filter paper [Aabye MG, Ruhwald M, Unpublished Data]. Biomarkers are stable in dried blood and plasma at ambient temperature Citation[97], therefore the filter paper technology enables cheap and simple sample transport over long distances by normal mail, and centralized analysis vis à vis the neonatal screening for metabolic diseases Citation[84].
Recently, two independent groups demonstrated CMI assays based on a finger-prick volume of blood (50 µl) using quantitative PCR detection of IP-10 mRNA Citation[98,99]. Berit Lange and coworkers have explored the potential of a simple field-friendly lateral flow rapid test for IP-10 detection in QFT-IT supernatants at point of incubation Citation[100]. IP-10 is thus a top candidate biomarker for a point-of-care test for detection of TB infection.
Other potential uses of IP-10 in TB management
Blood levels of IP-10 have potential as a marker of inflammation vis à vis C-reactive protein. A series of papers have demonstrated that unstimulated IP-10 levels in blood or plasma are increased with infection and disease, such as TB Citation[101–104], hepatitis C viral infection Citation[105–107] and bacteremia Citation[108–110]. IP-10 is produced at the site of inflammation and the levels in the blood appear to correlate with the extent of the inflammatory process. Compared with the high increase seen after in vitro antigen stimulation, blood levels in sick patients are lower.
Interestingly, IP-10 levels in blood and urine appear to decline with successful treatment Citation[104,110–116], and increase in patients where there is disease relapse or progression to active disease Citation[78,104]. IP-10 levels also remain consistent in a whole-blood sample for several days at 37°C, for example in the nil tube of the QFT-IT test Citation[53] [Aabye MG, Ruhwald M, Unpublished Data]. Therefore, measuring the level of IP-10 in the unstimulated sample appears to contribute additional information about the extent of infection, such as severity of disease and risk of progression, and could potentially also be used as a measure of treatment efficacy. This potential added benefit of IP-10 calls for exploration in prospective studies.
Conclusion
In conclusion, IP-10-based tests appear to perform comparably to the QFT-IT in most patient groups, but may provide some additional information, for example in young children and HIV-infected individuals with low CD4 cell count. However, more studies with a broader consensus on several technical aspects in IP-10 assay methodology and using validated cutoffs, as well as studies in exposed individuals, are needed before conclusions can be drawn.
New technologies are emerging, and a new generation of rapid and user-friendly tests appears eminent. If proven reliable for large-scale use, these new tests will probably be combined with a novel generation of M. tuberculosis-specific antigens with higher sensitivity, and antigens that are able to discriminate between patients with active TB and latent TB infection. In addition, several other uses of IP-10 measurements could be studied more thoroughly, such as the potential of the acute-phase reactant properties of plasma IP-10 in monitoring treatment responses, and predicting mortality and morbidity. Finally, dynamic patterns (conversions and reversions) of immune responses to TB antigens might provide useful information and need to be better understood.
Expert commentary
The IGRAs were introduced in the early 2000s as a new generation of very specific tests for infection with M. tuberculosis. It has been established that the IGRAs’ performance is superior to that of the TST, but sensitivity is still compromised in high-risk individuals, including HIV-infected individuals, children and patients treated with immunosuppressive drugs. New measures are needed to improve the IGRA method, for example the use of alternative biomarkers. From studies screening >40 alternative biomarkers, it appears that IP-10 is the most consistently expressed in response to TB antigens, rendering it the most promising alternative marker to IFN-γ in TB diagnosis.
Until now, 22 articles including authors from 22 countries have explored the diagnostic potential of IP-10. The studies conclude that IP-10 is a specific marker for TB infection expressed in high magnitude compared with IFN-γ. IP-10 appears to perform comparably to the QFT-IT in diagnosing active TB and several studies suggest that IP-10 is less influenced by advanced HIV infection and this is possibly also the case for young age. The majority of studies use supernatants from the QFT-IT; however, IP-10 has been measured with different ELISA and multiplexing assays, and interpreted using different cutoff points and very diverging study designs. This complicates accumulation of knowledge and direct comparison of the performance of an IP-10 test between studies. Therefore, there is a need for consensus in the field.
Some of the major problems of the IGRAs also seem to apply to the IP-10 test. However, particularly from the technical aspect, IP-10 might offer advantages over IFN-γ and, several innovative developments point towards a new generation of smaller, simpler and cheaper diagnostics tests based on IP-10. Further to this, IP-10 is a top candidate biomarker for a point-of-care test.
When the technical issues are resolved and the best candidate test has been identified, larger prospective studies investigating the predictive value of a positive IP-10 test should be carried out.
Five-year view
The IGRAs have set the stage for a move from the unspecific TST toward specific and objective tests for infection with M. tuberculosis. The current commercially available IGRAs show very high specificity, but have insufficient sensitivity. IP-10 is quantitative and qualitatively different to the currently used marker, IFN-γ, which opens the way for assay optimization and simplification.
In terms of improved accuracy, we believe that the intense search for efficient TB vaccine antigen candidates will also lead to the discovery of novel diagnostic antigens. Such diagnostic antigens are expected to both complement the currently known antigens for higher sensitivity, but also aid in differentiation between latent TB infection and active TB. These novel antigens will probably be less immunogenic than those such as ESAT6, wherein IP-10 is probably a more robust readout marker for such a diagnostic test.
In terms of improved ease of use we expect a panel of recently suggested low- and high-tech platforms to be optimized and evaluated for IP-10 detection. Recent work from our own group demonstrated that antigen-stimulated blood can be dried on filter paper and sent by regular mail to large centralized laboratories vis à vis neonatal screening for metabolic disorders. This method also has potential to markedly alleviate IGRA research in low-resource settings and can probably be put to use very soon.
In the future, IP-10 will possibly be quantified using a lateral-flow device, therefore enabling rapid diagnosis in places with little infrastructure, or as suggested by others in a microassay based on IP-10 mRNA detection with quantitative PCR following antigen stimulation. In addition, integration of the incubation step with the IP-10 readout step could lead to novel fully integrated fast and accurate lab-on-a-chip diagnostic platforms.
We therefore believe it is realistic that a new generation of IP-10 release assays will be designed within the next 5 years, and we expected that these have higher sensitivity, increased robustness and much greater ease of use.
Table 1. Selected studies assessing the performance of IP-10 in patients with confirmed or clinically assessed active tuberculosis.
Table 2. Selected studies assessing the specificity of IP-10 in unexposed healthy controls.
Table 3. Selected studies assessing the performance of IP-10 in children.
Table 4. Selected studies assessing the performance of IP-10 in immunosuppressed patients with active tuberculosis or tuberculosis exposure.
Table 5. Overview of assays used to detect IP-10 and potential cutoffs linked to the assays.
Box 1. The five essential components in the IFN-γ release assays test.
• Antigens: the specificity of the IFN-γ release assays is primarily linked to the antigens. The commercial tests use overlapping 16–26-mer peptides of the Mycobacterium tuberculosis proteins ESAT-6, CFP-10 and TB7.7. Several promising antigen alternatives are emerging.
• Antigen presenting cells (APCs): APCs express MHC molecules with specificity for the antigens to T cells. If the T-cell receptor recognizes the peptide antigen on the MHC, both the T cell and the APC become activated. If the person tested has a poor fit between HLA genotype and antigens used in the test, the IFN-γ release assays are expected to perform poorly Citation[117].
• T cells: a high number of central memory T cells specific for the peptides presented on the APCs ensure a strong immune activation in vitro; the presence of regulatory T cells dampens this response Citation[118,119].
• Incubation: for immune activation to occur, the cells need time and heat, so the commercial tests incubate for 16–24 h at 37°C.
• A readout biomarker: the commercial tests monitor the degree of immune activation by measuring the release of IFN-γ. If the release is higher than a cutoff, the test is deemed positive; if there is little or no response, the test is deemed negative Citation[5,30]. As in any immune response, several cytokines and chemokines are expressed as a result of the immune activation, including the chemokine IP-10 Citation[19,20].
Key issues
• Tuberculosis (TB) is one of the most important public health problems, with over 8 million new cases and 2 million deaths annually.
• Current focus is on identifying and treating active TB, but more accurate and cheaper tests for latent TB would enable early detection and targeted treatment of infected individuals at risk of developing active TB.
• The IFN-γ release assays have operational and diagnostic qualities for latent TB, which makes them superior to the century-old tuberculin skin test; however, there is room for improvement, especially in terms of increased sensitivity, ease of use and cost.
• IP-10 is an alternative diagnostic biomarker expressed at very high levels compared with the current marker, IFN-γ.
• The studies included in this review suggest that IP-10 performs at least on par with IFN-γ, and is unaffected by bacillus Calmette–Guérin vaccination and non-tuberculous mycobacteria infection.
• IP-10 has qualities that open the way for further developments, not available for IFN-γ, and several innovations have shown potential.
Financial & competing interests disclosure
M Ruhwald holds a research grant from the Danish National Advanced Technology Foundation, MG Aabye holds a Career PhD Scholarship from Copenhagen University and P Ravn is supported by The Danish Council for Independent Research – Medical Sciences. M Ruhwald, P Ravn and MG Aabye are registered as inventors on pending and issued patents disclosing the use of IP-10 as diagnostic biomarker for infection with Mycobacterium tuberculosis. The authors have no other relevant affiliations or financial involvement with any organization or entity with a financial interest in or financial conflict with the subject matter or materials discussed in the manuscript apart from those disclosed.
No writing assistance was utilized in the production of this manuscript.
References
- Dorhoi A, Reece ST, Kaufmann SHE. For better or for worse: the immune response against Mycobacterium tuberculosis balances pathology and protection. Immunol. Rev.240(1), 235–251 (2011).
- Lawn SD, Zumla AI. Tuberculosis. Lancet378(9785), 57–72 (2011).
- Kaufmann SHE. Fact and fiction in tuberculosis vaccine research: 10 years later. Lancet Infect. Dis.11(8), 633–640 (2011).
- Grant AD, Charalambous S, Fielding KL et al. Effect of routine isoniazid preventive therapy on tuberculosis incidence among HIV-infected men in South Africa: a novel randomized incremental recruitment study. JAMA293(22), 2719–2725 (2005).
- Andersen P, Munk ME, Pollock JM, Doherty TM. Specific immune-based diagnosis of tuberculosis. Lancet356(9235), 1099–1104 (2000).
- Lalvani A, Pareek M. A 100 year update on diagnosis of tuberculosis infection. Br. Med. Bull.93, 69–84 (2010).
- Mandalakas AM, Detjen AK, Hesseling AC, Benedetti A, Menzies D. Interferon-γ release assays and childhood tuberculosis: systematic review and meta-analysis. Int. J. Tuberc. Lung Dis.15(8), 1018–1032 (2011).
- Sester M, Sotgiu G, Lange C et al. Interferon-γ release assays for the diagnosis of active tuberculosis: a systematic review and meta-analysis. Eur. Respir. J.37, 100–111 (2011).
- Diel R, Goletti D, Ferrara G et al. Interferon-γ release assays for the diagnosis of latent Mycobacterium tuberculosis infection: a systematic review and meta-analysis. Eur. Respir. J.37(1), 88–99 (2011).
- Kabeer BSA, Sikhamani R, Raja A. Comparison of interferon γ-inducible protein-10 and interferon γ-based QuantiFERON TB Gold assays with tuberculin skin test in HIV-infected subjects. Diagn. Microbiol. Infect. Dis.71(3), 236–243 (2011).
- Cattamanchi A, Smith R, Steingart KR et al. Interferon-γ release assays for the diagnosis of latent tuberculosis infection in HIV-infected individuals: a systematic review and meta-analysis. J. Acquired Immune Defic. Syndromes56(3), 230–238 (2011).
- Bélard E, Semb S, Ruhwald M et al. Prednisolone treatment affects the performance of the QuantiFERON gold in-tube test and the tuberculin skin test in patients with autoimmune disorders screened for latent tuberculosis infection. Inflamm. Bowel Dis.17(11), 2340–2349 (2011).
- Aabye MG, Ravn P, PrayGod G et al. The impact of HIV infection and CD4 cell count on the performance of an interferon γ release assay in patients with pulmonary tuberculosis. PLoS One4(1), e4220 (2009).
- Aichelburg MC, Rieger A, Breitenecker F et al. Detection and prediction of active tuberculosis disease by a whole-blood interferon-γ release assay in HIV-1-infected individuals. Clin. Infect. Dis.48(7), 954–962 (2009).
- Søborg B, Andersen AB, Melbye M et al. Risk factors for Mycobacterium tuberculosis infection among children in Greenland. Bull. WHO89(10), 741E–748E (2011).
- Lange C, Rieder HL. Intention to test is intention to treat. Am. J. Respir. Crit. Care Med.183(1), 3–4 (2011).
- Ruhwald M, Bjerregaard-Andersen M, Rabna P, Kofoed K, Eugen-Olsen J, Ravn P. IP-10/CXCL10 release is induced by incubation of whole blood from tuberculosis patients with ESAT-6, CFP10 and TB7.7. Microbes Infect.9(7), 806–812 (2007).
- Bibova I, Linhartova I, Stanek O et al. Detection of immune cell response to M. tuberculosis-specific antigens by quantitative polymerase chain reaction. Diagn. Microbiol. Infect. Dis.72(1), 68–72 (2012).
- Chegou N, Black G, Kidd M, van Helden P, Walzl G. Host markers in QuantiFERON supernatants differentiate active TB from latent TB infection: preliminary report. BMC Pulmonary Med.9(1), 21 (2009).
- Frahm M, Goswami ND, Owzar K et al. Discriminating between latent and active tuberculosis with multiple biomarker responses. Tuberculosis91(3), 250–256 (2011).
- Hasan Z, Rao N, Salahuddin N et al.M. tuberculosis sonicate induced IFNγ, CXCL10 and IL10 can differentiate severity in tuberculosis. Scand. J. Immunol. doi:10.1111/j.1365-3083.2011.02642.x (2011) (Epub ahead of print).
- Ruhwald M, Bjerregaard-Andersen M, Rabna P, Eugen-Olsen J, Ravn P. IP-10, MCP-1, MCP-2, MCP-3, and IL-1RA hold promise as biomarkers for infection with M. tuberculosis in a whole blood based T-cell assay. BMC Res. Notes2(1), 19 (2009).
- Sutherland JS, de Jong BC, Jeffries DJ, Adetifa IM, Ota MOC. Production of TNF-α, IL-12(p40) and IL-17 can discriminate between active TB disease and latent infection in a West African cohort. PLoS One5(8), e12365 (2010).
- Kellar KL, Gehrke J, Weis SE et al. Multiple cytokines are released when blood from patients with tuberculosis is stimulated with mycobacterium tuberculosis antigens. PLoS One6(11), e26545 (2011).
- Lighter-Fisher J, Peng CH, Tse DB. Cytokine responses to QuantiFERON® peptides, purified protein derivative and recombinant ESAT-6 in children with tuberculosis. Int. J. Tuberc. Lung Dis.14(12), 1548–1555 (2010).
- Nemeth J, Winkler HM, Boeck L et al. Specific cytokine patterns of pulmonary tuberculosis in Central Africa. Clin. Immunol.138(1), 50–59 (2011).
- Li L, Qiao D, Fu X, Lao S, Zhang X, Wu C. Identification of Mycobacterium tuberculosis-specific Th1, Th17 and Th22 cells using the expression of CD40L in tuberculous pleurisy. PLoS One6(5), e20165 (2011).
- Chegou NN, Hoek KGP, Kriel M, Warren RM, Victor TC, Walzl G. Tuberculosis assays: past, present and future. Expert Rev. Anti Infect. Ther.9(4), 457–469 (2011).
- Lalvani A, Millington KA. T-cell interferon-γ release assays: can we do better? Eur. Respir. J.32(6), 1428–1430 (2008).
- Lalvani A, Pareek M. Interferon γ release assays: principles and practice. Enferm. Infecc. Microbiol. Clin.28(4), 245–252 (2010).
- Lange C, Mori T. Advances in the diagnosis of tuberculosis. Respirology15(2), 220–240 (2010).
- Walzl G, Ronacher K, Hanekom W, Scriba TJ, Zumla A. Immunological biomarkers of tuberculosis. Nat. Rev. Immunol.11(5), 343–354 (2011).
- van der Werf MJ, van Leth F. Latent tuberculosis infection and interferon-γ release assays: what new knowledge did we gain through the Journal in 2009? Int. J. Tuberc. Lung Dis.14(12), 1525–1529 (2010).
- Kasprowicz VO, Churchyard G, Lawn SD, Squire SB, Lalvani A. Diagnosing latent tuberculosis in high-risk individuals: rising to the challenge in high-burden areas. J. Infect. Dis.204(Suppl. 4), S1168–S1178 (2011).
- Dhillon NK, Peng F, Ransohoff RM, Buch S. PDGF Synergistically enhances IFN-γ-induced expression of CXCL10 in blood-derived macrophages: implications for HIV dementia. J. Immunol.179(5), 2722–2730 (2007).
- Moser B, Loetscher P. Lymphocyte traffic control by chemokines. Nat. Immunol.2(2), 123–128 (2001).
- Groom JR, Luster AD. CXCR3 ligands: redundant, collaborative and antagonistic functions. Immunol. Cell Biol.89(2), 207–205 (2011).
- Farber JM. Mig and IP-10: CXC chemokines that target lymphocytes. J. Leukoc. Biol.61(3), 246–257 (1997).
- Narumi S, Finke JH, Hamilton TA. Interferon γ and interleukin 2 synergize to induce selective monokine expression in murine peritoneal macrophages. J. Biol. Chem.265(12), 7036–7041 (1990).
- Sauty A, Dziejman M, Taha RA et al. The T cell-specific CXC chemokines IP-10, Mig, and I-TAC are expressed by activated human bronchial epithelial cells. J. Immunol.162(6), 3549–3558 (1999).
- Karonitsch T, Dalwigk K, Steiner CW et al. Interferon (IFN) signals and monocytic sensitization of the IFNγ signaling pathway in peripheral blood of rheumatoid arthritis patients. Arthritis Rheum. doi:10.1002/art.33347 (2011) (Epub ahead of print).
- Ohmori Y, Wyner L, Narumi S, Armstrong D, Stoler M, Hamilton TA. Tumor necrosis factor-α induces cell type and tissue-specific expression of chemoattractant cytokines in vivo. Am. J. Pathol.142(3), 861–870 (1993).
- Guzzo C, Che Mat NF, Gee K. Interleukin-27 induces a STAT1/3- and NF-κB-dependent proinflammatory cytokine profile in human monocytes. J. Biol. Chem.285(32), 24404–24411 (2010).
- Rudner XL, Happel KI, Young EA, Shellito JE. Interleukin-23 (IL-23)-IL-17 cytokine axis in murine Pneumocystis carinii infection. Infect. Immun.75(6), 3055–3061 (2007).
- Khader SA, Bell GK, Pearl JE et al. IL-23 and IL-17 in the establishment of protective pulmonary CD4+ T cell responses after vaccination and during Mycobacterium tuberculosis challenge. Nat. Immunol.8(4), 369–377 (2007).
- Qian C, An H, Yu Y, Liu S, Cao X. TLR agonists induce regulatory dendritic cells to recruit Th1 cells via preferential IP-10 secretion and inhibit Th1 proliferation. Blood109(8), 3308–3315 (2007).
- Chen J, Liu X. The role of interferon γ in regulation of CD4+ T-cells and its clinical implications. Cell Immunol.254(2), 85–90 (2009).
- Farrar MA, Schreiber RD. The molecular cell biology of interferon-γ and its receptor. Annu. Rev. Immunol.11, 571–611 (1993).
- Aabye MG, Ravn P, Johansen IS, Eugen-Olsen J, Ruhwald M. Incubation of whole blood at 39°C augments IP-10 and IFN-γ responses to M. tuberculosis antigens. Clin. Vaccine Immunol.18(7), 1150–1156 (2011).
- Goletti D, Raja A, Kabeer BSA et al. IFN-[γ], but not IP-10, MCP-2 or IL-2 response to RD1 selected peptides associates to active tuberculosis. J. Infect.61(2), 133–143 (2010).
- Kabeer BSA, Raja A, Raman B et al. IP-10 response to RD1 antigens might be a useful biomarker for monitoring tuberculosis therapy. BMC Infect. Dis.11, 135 (2011).
- Ruhwald M, Bodmer T, Maier C et al. Evaluating the potential of IP-10 and MCP-2 as biomarkers for the diagnosis of tuberculosis. Eur. Respir. J.32(6), 1607–1615 (2008).
- Ruhwald M, Dominguez J, Latorre I et al. A multicentre evaluation of the accuracy and performance of IP-10 for the diagnosis of infection with M. tuberculosis. Tuberculosis91(3), 260–267 (2011).
- Kabeer BSA, Raman B, Thomas A, Perumal V, Raja A. Role of QuantiFERON-TB gold, interferon γ inducible protein-10 and tuberculin skin test in active tuberculosis diagnosis. PLoS One5(2), e9051 (2010).
- Aabye MG, Ruhwald M, Praygod G et al. Potential of interferon-{γ}-inducible protein 10 in improving tuberculosis diagnosis in HIV-infected patients. Eur. Respir. J.36(6), 1488–1490 (2010).
- Goletti D, Raja A, Kabeer BSA et al. Is IP-10 an accurate marker for detecting M. tuberculosis-specific response in HIV-infected persons? PLoS One5(9), e12577 (2010).
- Chintu C, Mudenda V, Lucas S et al. Lung diseases at necropsy in African children dying from respiratory illnesses: a descriptive necropsy study. Lancet360(9338), 985–990 (2002).
- McNally LM, Jeena PM, Gajee K et al. Effect of age, polymicrobial disease, and maternal HIV status on treatment response and cause of severe pneumonia in South African children: a prospective descriptive study. Lancet369(9571), 1440–1451 (2007).
- Marais BJ, Gie RP, Schaaf HS, Beyers N, Donald PR, Starke JR. Childhood pulmonary tuberculosis: old wisdom and new challenges. Am. J. Respir. Crit. Care Med.173(10), 1078–1090 (2006).
- Marais BJ, Pai M. New approaches and emerging technologies in the diagnosis of childhood tuberculosis. Paediatr. Respir. Rev.8(2), 124–133 (2007).
- Yassin MA, Petrucci R, Garie KT et al. Can interferon-γ or interferon-γ-induced-protein-10 differentiate tuberculosis infection and disease in children of high endemic areas? PLoS One6(9), e23733 (2011).
- Alsleben N, Ruhwald M, Rüssmann H, Marx FM, Wahn U, Magdorf K. Interferon-γ inducible protein 10 as a biomarker for active tuberculosis and latent tuberculosis infection in children: a case–control study. Scand. J. Infect. Dis. doi:10.3109/00365548.2011.632644 (2011).
- Lighter J, Rigaud M, Huie M, Peng CH, Pollack H. Chemokine IP-10: an adjunct marker for latent tuberculosis infection in children. Int. J. Tuberc. Lung Dis.13(6), 731–736 (2009).
- Ruhwald M, Petersen J, Kofoed K et al. Improving T-cell assays for the diagnosis of latent TB infection: potential of a diagnostic test based on IP-10. PLoS One3(8), e2858 (2008).
- Machingaidze S, Wiysonge CS, Gonzalez-Angulo Y et al. The utility of an interferon γ release assay for diagnosis of latent tuberculosis infection and disease in children: a systematic review and meta-analysis. Pediatr. Infect. Dis. J.30(8), 694–700 (2011).
- Akolo C, Adetifa I, Shepperd S, Volmink J. Treatment of latent tuberculosis infection in HIV infected persons. Cochrane Database Syst. Rev.1, CD000171 (2010).
- Santin M, Casas S, Saumoy M et al. Detection of latent tuberculosis by the tuberculin skin test and a whole-blood interferon-γ release assay, and the development of active tuberculosis in HIV-seropositive persons. Diagn. Microbiol. Infect. Dis.69(1), 59–65 (2011).
- Latorre I, Martínez-Lacasa X, Font R et al. IFN-γ response on T-cell based assays in HIV-infected patients for detection of tuberculosis infection. BMC Infect. Dis.10, 348 (2010).
- Leidl L, Mayanja-Kizza H, Sotgiu G et al. Relationship of immunodiagnostic assays for tuberculosis and numbers of circulating CD4+ T-cells in HIV infection. Eur. Respir. J.35(3), 619–626 (2010).
- Kabeer BSA, Sikhamani R, Swaminathan S, Perumal V, Paramasivam P, Raja A. Role of interferon γ release assay in active TB diagnosis among HIV infected individuals. PLoS One4(5), e5718 (2009).
- Raby E, Moyo M, Devendra A et al. The effects of HIV on the sensitivity of a whole blood IFN-γ release assay in Zambian adults with active tuberculosis. PLoS One3(6), e2489 (2008).
- Brock I, Ruhwald M, Lundgren B, Westh H, Mathiesen LR, Ravn P. Latent tuberculosis in HIV positive, diagnosed by the M. tuberculosis specific interferon-γ test. Respir. Res.7, 56 (2006).
- Kabeer BS, Sikhamani R, Raja A. Comparison of interferon γ and interferon γ-inducible protein-10 secretion in HIV-tuberculosis patients. AIDS24(2), 323–325 (2010).
- Sutherland JS, Young JM, Peterson KL et al. Polyfunctional CD4+ and CD8+ T cell responses to tuberculosis antigens in HIV-1-infected patients before and after anti-retroviral treatment. J. Immunol.184(11), 6537–6544 (2010).
- Qi XF, Kim DH, Yoon YS et al. Essential involvement of cross-talk between IFN-γ and TNF-α in CXCL10 production in human THP-1 monocytes. J. Cell. Physiol.220(3), 690–697 (2009).
- Solovic I, Sester M, Gomez-Reino JJ et al. The risk of tuberculosis related to tumour necrosis factor antagonist therapies: a TBNET consensus statement. Eur. Respir. J.36(5), 1185–1206 (2010).
- Bélard E, Semb S, Ruhwald M et al. Prednisolone treatment affects the performance of the QuantiFERON gold in-tube test and the tuberculin skin test in patients with autoimmune disorders screened for latent tuberculosis infection. Inflamm. Bowel Dis.17(11), 2340–2349 (2011).
- Chen DY, Shen GH, Chen YM et al. Interferon-inducible protein-10 as a marker to detect latent and active tuberculosis in rheumatoid arthritis. Int. J. Tuberc. Lung Dis.15(2), 192–200 (2011).
- Lei HY, Chang CP. Lectin of concanavalin A as an anti-hepatoma therapeutic agent. J. Biomed. Sci.16, 10 (2009).
- Itoh Y, Morita A, Nishioji K et al. Time course profile and cell-type-specific production of monokine induced by interferon-γ in concanavalin A-induced hepatic injury in mice: comparative study with interferon-inducible protein-10. Scand. J. Gastroenterol.36(12), 1344–1351 (2001).
- Denkinger CM, Dheda K, Pai M. Guidelines on interferon-γ release assays for tuberculosis infection: concordance, discordance or confusion? Clin. Microbiol. Infect.17(6), 806–814 (2011).
- Sester M, Sotgiu G, Lange C et al. Interferon-γ release assays for the diagnosis of active tuberculosis: a systematic review and meta-analysis. Eur. Respir. J.37(1), 100–111 (2011).
- Goletti D, Carrara S, Butera O et al. Accuracy of immunodiagnostic tests for active tuberculosis using single and combined results: a multicenter TBNET-Study. PLoS One3(10), e3417 (2008).
- Skogstrand K, Thorsen P, Nørgaard-Pedersen B, Schendel DE, Sørensen LC, Hougaard DM. Simultaneous measurement of 25 inflammatory markers and neurotrophins in neonatal dried blood spots by immunoassay with xMAP technology. Clin. Chem.51(10), 1854–1866 (2005).
- Geluk A, van der Ploeg-van Schip JJ, van Meijgaarden KE et al. Enhancing sensitivity of detection of immune responses to Mycobacterium leprae peptides in whole-blood assays. Clin. Vaccine Immunol.17(6), 993–1004 (2010).
- Chen J, Wang S, Zhang Y et al. Rv1985c, a promising novel antigen for diagnosis of tuberculosis infection from BCG-vaccinated controls. BMC Infect. Dis.10, 273 (2010).
- Millington KA, Fortune SM, Low J et al. Rv3615c is a highly immunodominant RD1 (region of difference 1)-dependent secreted antigen specific for Mycobacterium tuberculosis infection. Proc. Natl Acad. Sci. USA108(14), 5730–5735 (2011).
- Bertholet S, Horne DJ, Laughlin EM et al. Effect of chemotherapy on whole blood cytokine profiles to Mycobacterium tuberculosis antigens in a small cohort of pulmonary tuberculosis patients. Clin. Vaccine Immunol.18(8), 1378–1386 (2011).
- Goletti D, Butera O, Vanini V et al. Response to Rv2628 latency antigen associates with cured tuberculosis and remote infection. Eur. Respir. J.36(1), 135–142 (2010).
- Schuck SD, Mueller H, Kunitz F et al. Identification of T-cell antigens specific for latent Mycobacterium tuberculosis infection. PLoS One4(5), e5590 (2009).
- Chiacchio T, Petruccioli E, Vanini V et al. Higher frequency of T-cell response to M. tuberculosis latency antigen Rv2628 at the site of active tuberculosis disease than in peripheral blood. PLoS One6(11), e27539 (2011).
- Butera O, Chiacchio T, Carrara S et al. New tools for detecting latent tuberculosis infection: evaluation of RD1-specific long-term response. BMC Infect. Dis.9, 182 (2009).
- Leyten EM, Lin MY, Franken KL et al. Human T-cell responses to 25 novel antigens encoded by genes of the dormancy regulon of Mycobacterium tuberculosis. Microbes Infect.8(8), 2052–2060 (2006).
- Denis M, Wedlock DN, McCarthy AR et al. Enhancement of the sensitivity of the whole-blood γ interferon assay for diagnosis of Mycobacterium bovis infections in cattle. Clin. Vaccine Immunol.14(11), 1483–1489 (2007).
- Feske ML, Medina M, Graviss EA, Lewis DE. IL-7 addition increases spot size and number as measured by T-SPOT.TB(®). Methods Mol. Biol.792, 229–241 (2012).
- Feske M, Nudelman RJ, Medina M et al. Enhancement of human antigen-specific memory T-cell responses by interleukin-7 may improve accuracy in diagnosing tuberculosis. Clin. Vaccine Immunol.15(10), 1616–1622 (2008).
- Mei JV, Zobel SD, Hall EM, De Jesús VR, Adam BW, Hannon WH. Performance properties of filter paper devices for whole blood collection. Bioanalysis2(8), 1397–1403 (2010).
- Kasprowicz VO, Mitchell JE, Chetty S et al. A molecular assay for sensitive detection of pathogen-specific T-cells. PLoS One6(8), e20606 (2011).
- Chakera A, Bennett SC, Cornall RJ. A whole blood monokine-based reporter assay provides a sensitive and robust measurement of the antigen-specific T cell response. J. Translat. Med.9(1), 143 (2011).
- Lange B, Vavra M, Kern M, Wagner D. IP-10 measurement using a point of care test for the diagnosis of active tuberculosis. Presented at: The European Respiratory Society Annual Congress. Amsterdam, The Netherlands, 24–28 September 2011 (Abstract #1900).
- Su WL, Perng WC, Huang CH, Yang CY, Wu CP, Chen JH. Association of reduced tumor necrosis factor α, γ interferon, and interleukin-1β (IL-1β) but increased IL-10 expression with improved chest radiography in patients with pulmonary tuberculosis. Clin. Vaccine Immunol.17(2), 223–231 (2010).
- Lu C, Wu J, Wang H et al. Novel biomarkers distinguishing active tuberculosis from latent infection identified by gene expression profile of peripheral blood mononuclear cells. PLoS One6(8), e24290 (2011).
- Berry MPR, Graham CM, McNab FW et al. An interferon-inducible neutrophil-driven blood transcriptional signature in human tuberculosis. Nature466(7309), 973–977 (2010).
- Azzurri A, Sow OY, Amedei A et al. IFN-γ-inducible protein 10 and pentraxin 3 plasma levels are tools for monitoring inflammation and disease activity in Mycobacterium tuberculosis infection. Microbes Infect.7(1), 1–8 (2005).
- Casrouge A, Decalf J, Ahloulay M et al. Evidence for an antagonist form of the chemokine CXCL10 in patients chronically infected with HCV. J. Clin. Invest.121(1), 308–317 (2011).
- Zeremski M, Hooker G, Shu MA et al. Induction of CXCR3- and CCR5-associated chemokines during acute hepatitis C virus infection. J. Hepatol.55(3), 545–553 (2011).
- Askarieh G, Alsiö A, Pugnale P et al. Systemic and intrahepatic interferon-γ-inducible protein 10 kDa predicts the first-phase decline in hepatitis C virus RNA and overall viral response to therapy in chronic hepatitis C. Hepatology51(5), 1523–1530 (2010).
- Ng PC, Li K, Chui KM et al. IP-10 is an early diagnostic marker for identification of late-onset bacterial infection in preterm infants. Pediatr. Res.61(1), 93–98 (2007).
- Punyadeera C, Schneider EM, Schaffer D et al. A biomarker panel to discriminate between systemic inflammatory response syndrome and sepsis and sepsis severity. J. Emerg. Trauma Shock3(1), 26–35 (2010).
- Olszyna DP, Prins JM, Dekkers PE et al. Sequential measurements of chemokines in urosepsis and experimental endotoxemia. J. Clin. Immunol.19(6), 399–405 (1999).
- Zeremski M, Petrovic LM, Talal AH. The role of chemokines as inflammatory mediators in chronic hepatitis C virus infection. J. Viral Hepat.14(10), 675–687 (2007).
- Ng PC, Lam CWK, Li AM et al. Chemokine response in children with SARS. Arch. Dis. Child90(4), 422–423 (2005).
- Bai X, Wilson SE, Chmura K, Feldman NE, Chan ED. Morphometric analysis of Th(1) and Th(2) cytokine expression in human pulmonary tuberculosis. Tuberculosis84(6), 375–385 (2004).
- Pokkali S, Das SD, R L. Expression of CXC and CC type of chemokines and its receptors in tuberculous and non-tuberculous effusions. Cytokine41(3), 307–314 (2008).
- Dheda K, Van-Zyl Smit RN, Sechi LA et al. Clinical diagnostic utility of IP-10 and LAM antigen levels for the diagnosis of tuberculous pleural effusions in a high burden setting. PLoS One4(3), e4689 (2009).
- Cannas A, Calvo L, Chiacchio T et al. IP-10 detection in urine is associated with lung diseases. BMC Infect. Dis.10, 333 (2010).
- Hang NTL, Lien LT, Kobayashi N et al. Analysis of factors lowering sensitivity of interferon-γ release assay for tuberculosis. PLoS One6(8), e23806 (2011).
- Li L, Qiao D, Zhang X, Liu Z, Wu C. The immune responses of central and effector memory BCG-specific CD4+ T cells in BCG-vaccinated PPD+ donors were modulated by Treg cells. Immunobiology216(4), 477–484 (2011).
- Rueda CM, Marín ND, García LF, Rojas M. Characterization of CD4 and CD8 T cells producing IFN-γ in human latent and active tuberculosis. Tuberculosis90(6), 346–353 (2010).
- Petrucci R, Abu AN, Gurgel RQ et al. Interferon γ, interferon-γ-induced-protein 10, and tuberculin responses of children at high risk of tuberculosis infection. Pediatr. Infect. Dis. J.27(12), 1073–1077 (2008).
- Whittaker E, Gordon A, Kampmann B. Is IP-10 a better biomarker for active and latent tuberculosis in children than IFNγ? PLoS One3(12), e3901 (2008).
Patent
- Rothel JS, Wild S, Cosgriff A: Diagnostic assay for measuring a cell mediated immune response: US7608392 (2004).
Websites
- WHO New WHO recommendations on use of commercial TB interferon-γ release assays (IGRAs) in low- and middle-income countries.www.who.int/tb/features_archive/igra_policy24oct/en/index.html
- WHO Guidelines for intensified tuberculosis case-finding and isoniazid preventive therapy for people living with HIV in resource-constrained settings. www.who.int/tb/challenges/hiv/ICF_IPTguidelines/en/index.html