Abstract
Nociceptive inputs reach the CNS by means of small myelinated and unmyelinated fibers. Owing to this, conventional nerve conduction studies and electromyography are less likely to demonstrate abnormalities in neuropathies affecting nociceptive fibers than in those involving large myelinated fibers. Therefore, to characterize painful neuropathies, clinicians evaluate the features of the lesion that cause pain rather than the feeling of pain itself. Clinical neurophysiological assessment of painful neuropathies still relies on conventional nerve conduction studies but slightly more sophisticated techniques bring more specific information. These are the nociceptive-evoked potentials, microneurography or autonomic nervous system functional tests. Neurophysiological techniques can also add information to quantitative sensory testing by recording autonomic responses such as the sudomotor skin response or the voluntary reaction. Functional magnetic resonance should also be considered as a neurophysiological technique, which allows for mapping the areas of the brain involved in nociceptive sensation and pain control.
Medscape: Continuing Medical Education Online
This activity has been planned and implemented in accordance with the Essential Areas and policies of the Accreditation Council for Continuing Medical Education through the joint sponsorship of Medscape, LLC and Expert Reviews Ltd. Medscape, LLC is accredited by the ACCME to provide continuing medical education for physicians.
Medscape, LLC designates this Journal-based CME activity for a maximum of 1 AMA PRA Category 1 Credit(s)™. Physicians should claim only the credit commensurate with the extent of their participation in the activity.
All other clinicians completing this activity will be issued a certificate of participation. To participate in this journal CME activity: (1) review the learning objectives and author disclosures; (2) study the education content; (3) take the post-test with a 70% minimum passing score and complete the evaluation at www.medscape.org/journal/expertimmunology; (4) view/print certificate.
Release date: 12 December 2012; Expiration date: 12 December 2013
Learning objectives
Upon completion of this activity, participants will be able to:
• Describe common causes and associated features of small-fiber neuropathies associated with pain
• Describe use of neurophysiological techniques in the characterization of painful neuropathies
• Describe use of psychophysical tests and functional MRI (fMRI) in the characterization of painful neuropathies
Financial & competing interests disclosure
Editor
Elisa Manzotti
Publisher, Future Science Group, London, UK
Disclosure: Elisa Manzotti has disclosed no relevant financial relationships.
CME Author
Laurie Barclay
Freelance writer and reviewer, Medscape, LLC
Disclosure: Laurie Barclay, MD, has disclosed no relevant financial relationships.
Authors and Credentials
Gonzalo Barraza-Sandoval, MD
Neurology Department, Electromyography, Neuropathic Pain and Motor Control Unit, Hospital Clínic, Institut d'Investigació August Pi i Sunyer, Universidad de Barcelona, Spain
Disclosure: Gonzalo Barraza-Sandoval, MD, has disclosed no relevant financial relationships.
Jordi Casanova-Mollá, MD
Neurology Department, Electromyography, Neuropathic Pain and Motor Control Unit, Hospital Clínic, Institut d'Investigació August Pi i Sunyer, Universidad de Barcelona, Spain
Disclosure: Jordi Casanova-Mollá, MD, has disclosed no relevant financial relationships.
Josep Valls-Solé, MD, PhD
Neurology Department, Electromyography, Neuropathic Pain and Motor Control Unit, Hospital Clínic, Institut d'Investigació August Pi i Sunyer, Universidad de Barcelona, Spain
Disclosure: Josep Valls-Solé, MD, PhD, has disclosed no relevant financial relationships.
(A) High-frequency discharge. It usually results from an ectopic focus generating activity of neighboring hyperexcitable muscle fibers. (B) Myokymic discharge. Action potentials of a relatively simple shape, either isolated or repeating in groups at a stable frequency. (C) Neuromyotonic discharge. Complex action potentials that repeat with no variation, sometimes at a very high frequency. †Calibration is 10 ms × 0.2 mV.‡Calibration is 50 ms × 0.5 mV.§Calibration is 20 ms × 0.1 mV.
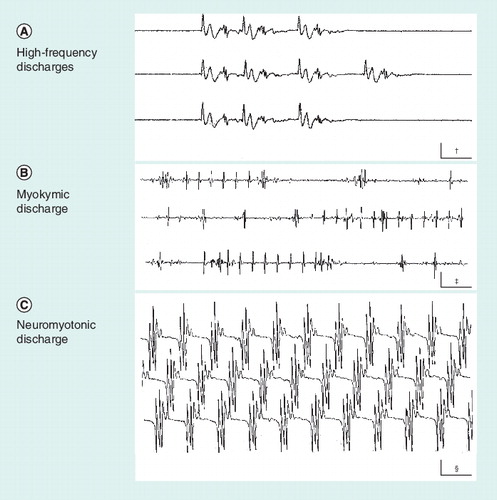
The top traces show the variation in latency of the second QRS complex with respect to the first and the bottom traces show the same recordings superimposed to better appreciate the ‘jitter’ of the second QRS complex (horizontal arrows) and the differences between (A) a healthy subject and (B) a patient with autonomic dysfunction in the context of Chagas disease.
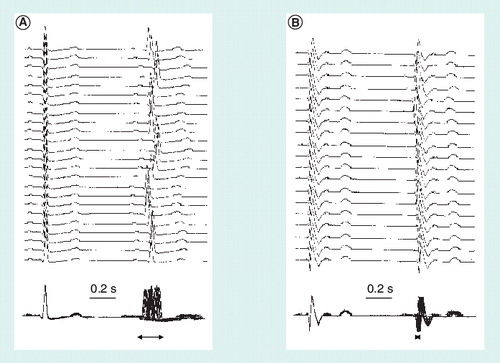
(A) In a healthy subject and (B) in a patient with axonal distal polyneuropathy. Numbers below each waveform express the peak latency in miliseconds.
CHEP: Contact heat-evoked potential; LEP: Laser-evoked potential.
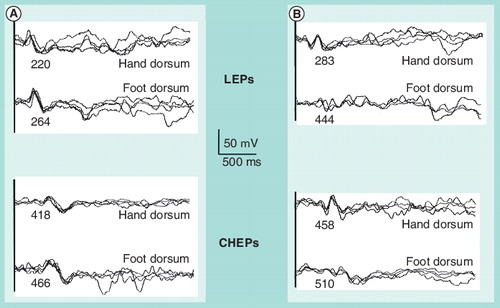
Subjects are requested to reproduce their sensations when a slow rise in temperature is applied to hands or feet. The upper graph is the temperature profile and the bottom graph the subjective reproduction of the sensations. Typically, patients with polyneuropathy show a delay in perception of warm and pain, remain marking pain for longer time than healthy subjects and do not mark any decrease in temperature below neutral (the temperature at onset), in the descent phase after the stimulus. (A) Healthy subject and (B) patient with mild-to-moderate diabetic polyneuropathy.VAS: Visual analogue scale.
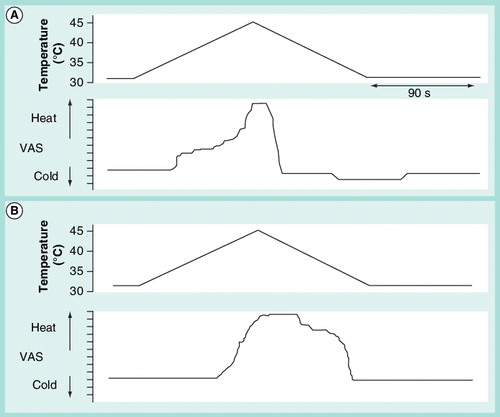
Pain is the most distressing symptom in some types of peripheral neuropathies, such as focal sensory nerve lesions and small-fiber polyneuropathies (painful neuropathies). Patients who suffer from neuropathic pain due to neuropathy may use a specific terminology to describe their symptoms and may show signs of a neuropathic lesion at physical examination that may allow for clinical diagnosis of a painful neuropathy, according to appropriate guidelines Citation[1–3]. Painful neuropathies are those peripheral nerve diseases in which the predominant manifestation is pain, including mononeuropathies, such as entrapment syndromes, traumatic lesions or radiculopathies, multifocal neuropathies, such as vasculitic lesions, and polyneuropathies, such as in metabolic, autoimmune or toxic disorders. An expert in neuropathic pain is fully aware that pain cannot be measured in an objective way. Therefore, clinical skills are still the most important asset for the assessment of painful neuropathies. A complete clinical evaluation should not only include a thorough anamnesis and physical neurological examination but also the use of visual analog scales or numerical rating scales for quantitation of symptom severity, and psychophysical testing to document sensory thresholds.
Neuropathic pain, as currently defined by the International Association for the Study of Pain, implies a lesion in the somatosensory system Citation[4]. Assessment of such a lesion may require clinical skills and appropriate diagnostic tests to support the clinical suspicion and document the nerve impairment. Electrodiagnostic tests are probably the complementary examinations that are most frequently used for the diagnosis of all kinds of neuropathies. However, they have important limitations for the study of painful neuropathies. Nerve conduction studies are expressing solely or predominantly the responses of large fibers in the peripheral nerves, thus allowing for very little insight on the function of small fibers. Moreover, the assessment is limited to loss of function but does not assess irritative or positive signs, which are the ones that usually lead to the patient’s complaints in neuropathic pain syndromes. Since conventional electrodiagnostic tests may provide no evidence for dysfunction, specific tests should be considered for correct clinical–electrophysiological evaluation of patients with painful neuropathies Citation[5]. This does not mean that conventional electrophysiological studies are not useful in the diagnostic workout of patients with neuropathic pain. On the contrary, the first approach in the study of a patient with neuropathic pain due to peripheral neuropathies should be to assess the somatosensory function using clinical and electrophysiological methods. In this review, the authors discuss the neurophysiological methods that are most useful for the assessment of painful neuropathies, with emphasis on small-fiber polyneuropathies as one of the most common expressions of painful neuropathies. However, it should be made clear that although the authors were able to objectively quantify the degree of the damage in small fibers, there is currently no method that allows for an objective assessment of pain and, therefore, the only distinction between painful and nonpainful neuropathies is currently the subjective complain.
Conventional clinical neurophysiological assessment
There is a great deal of information gathered from needle electromyography, conventional nerve conduction studies and late responses, mainly in traumatic focal nerve lesions and axonal polyneuropathies. Needle electromyography may inform on the presence of denervated muscle fibers. The most typical manifestation is fibrillation potentials or positive sharp waves at rest and functional loss of motor units during voluntary contraction. Fibrillation potentials and positive sharp waves result from the depolarization of denervated muscle fibers that are hyperexcitable after having lost their nerve input. Functional loss of motor units during muscle contraction is evidenced by the remaining active motor units firing at a higher frequency than would be normally seen at any level of contraction. The axonal neurochemical dysfunction responsible for axonal damage in certain peripheral neuropathies may also lead to hyperactivity owing to self-excitation phenomena or ectopic impulse generation. The most striking forms of activity picked up with needle electromyography are neuromyotonic, myokymic or high-frequency discharges Citation[6]. Such forms of abnormal electromyographic activity in motor fibers could have a correlate in sensory fibers where similar ectopic foci may be present. Even though they are not easily recognized, ectopic discharges in sensory fibers could underlie clinical symptoms such as paresthesias or other forms of abnormal sensations, including pain Citation[7]. Hyperactivity discharges are sometimes the only sign suggesting axonal dysfunction as the underlying cause of pain in peripheral neuropathies. The discharges of high-frequency action potentials can perpetuate in reverberating circuits due to ephaptic phenomena. The muscle fibers and terminal axons generating the hyperactivity discharges may, in some cases, be disconnected from the motoneuron because of axonal block and, therefore, they remain unaccessible to voluntary inputs or other forms of motor unit activation .
Nerve conduction studies may help in the topographic diagnosis of the lesion and contribute to recognizing the nature of the disorder and its prognosis. An electrical stimulus applied to a peripheral nerve gives rise to an action potential that propagates along the nerve and can be recorded with appropriate electrodes in another nerve site (sensory or mixed nerve action potentials), in the supplied muscle (compound motor action potential [CMAP]), or along the sensory pathway to the brain (somatosensory evoked potentials [SSEPs]). Motor or sensory nerve conduction velocity is usually calculated between two consecutive points of stimulation or between the stimulation and recording sites. With supramaximal stimulation, the CMAP or the sensory nerve action potential reflect the amount of excitable motor axons. Functional or structural axonal damage would lead to loss of action potential amplitude while demyelination would lead to slowing of conduction velocity. For the assessment of proximal nerve lesions (i.e., those occurring in the plexus or in the roots), late responses have been used to the electrical stimuli applied directly over mixed nerves. In motor fibers, the orthodromic propagation will give rise to the CMAP in the muscle innervated by that nerve, while the antidromic propagation will reach the motoneuron and induce a reverberating response, known as ‘F wave’, if the motoneuron is quiescent. In sensory fibers, the orthodromic input will reach the CNS and eventually generate a cerebral-evoked potential, while the antidromic volley will reach the distal end of the sensory fibers with no known consequences. A few relevant points in the interpretation of the neurophysiological findings gathered after a complete conventional electrophysiological study in patients with peripheral neuropathy are described in Box 1.
Certain stimuli are suitable for triggering a specific reflex response. Electrical stimuli of low intensity are likely to activate only the largest fibers in a nerve trunk, the Ia afferent fibers from the muscle spindles, which are known to connect to the a motoneuron to mediate the monosynaptic tendon jerk. Therefore, low-intensity electrical stimuli applied to the posterior tibial nerve at the popliteal fossa should lead in a healthy subject to the H reflex in the soleus muscle, a reflex response that is considered similar to the Achilles tendon jerk for clinical studies. The main benefit of the study of reflex responses in patients with painful neuropathies is the eventual localization of the site of the lesion. Other reflex responses issuing from more complex circuits generated in cutaneous inputs Citation[8] may be abnormal in painful neuropathies. One test that specifically involves small afferent fibers is the silent period induced by cutaneous afferents Citation[9]. However, the clinical applicability of these tests to the evaluation of painful neuropathies is still scarce.
Nerve conduction studies and needle electromyography, together with the study of late responses, should indicate the site and extent of the lesion; however, we can only speculate on the pathophysiological mechanisms leading to pain. If the lesion involves the small nerve fibers from nociceptive receptors, pain may result from ectopic discharges and other forms of hyperactivity in sensory axons Citation[10–12]. Apart from axonal irritation due to the inadequate interstitial environment, axonal growth after axonotmesis (either in traumatic nerve lesions or in systemic neuropathies) may lead to hyperexcitability. The likely reinnervation errors may also contribute to the disorder. Abnormal reinnervation involves misdirection of growing axons to targets different from those that were previously innervating, multiple unnecessary branching of axons Citation[13], axonal growth in distant nerves, compensatory hyperactivity in nondamaged territories and increase in excitability of the parent neurons Citation[14]. All of these abnormalities imply unstable axons capable of generating ectopic discharges or autoexcitation after the passage of a normal impulse. In lesions involving other nerve fibers, pain may generate if there is deafferentation of second-order sensory neurons. Some of them receive synaptic inputs from afferents of different sensory modalities (wide dynamic range neurons). These neurons may undergo rearrangement of synaptic connections in peripheral nerve lesions Citation[15], a theory that has been used to explain many pain syndromes Citation[16–19].
Activation of afferents from cutaneous nerves can be used as a tool to investigate individual responses to experimental pain. For this, trains of three to five stimuli at a rather high frequency (200 Hz) are applied to the sural or posterior tibial nerves and responses are recorded from the lateral head of the biceps femoris. The response can be considered a surrogate of the withdrawal reflex, with paradigmatic RII and RIII reflex responses appearing at pain threshold at approximately 90–130 ms Citation[20]. The main interest of this response is that its size correlates positively with the sensation of pain, measured with a numerical rating scale score and, therefore, it has been often used as a test for efficacy of certain treatments for pain Citation[21].
Stimulus-induced sensory volleys reaching the CNS may cause neuronal activity that can be recorded with electrodes attached to the skin in the skull, the SSEPs. These are appropriate for the assessment of lesions in the somatosensory pathways for tactile and proprioceptive systems (i.e., the dorsal columns) but they are normal in patients with selective lesions of the spinothalamic tract and in syndromes presenting with dissociated sensory loss (i.e., impairment of pain and temperature sensitivity). This limitation means that a number of lesions of the somatosensory system may not be detectable by the SSEPs, which has made it necessary to develop tools for testing the nociceptive-evoked potentials.
Specific tests for the study of small (unmyelinated or thinly myelinated) fibers
Microneurography
Microneurography allows for the assessment of conduction and other properties of thin nerve fibers Citation[22]. In expert hands, the technique may be the only one to inform directly on axonal conduction abnormalities in patients with painful neuropathies but the collaboration of the patient is of paramount importance. It requires the insertion of a fine needle recording electrode with a tip diameter of 1–5 µm into an accessible peripheral nerve to directly record the action potentials from axons. Once the needle is positioned in such a way that action potentials can be obtained to cutaneous stimulation, the behavior of such potentials to changes in frequency or quality of the stimulus are the ones defining the type of fiber Citation[23,24]. The action potentials recorded with microneurography are relatively stable with stimuli of rather low frequency but may be blocked with higher frequencies. The behavior of these potentials to protocolized stimuli parameters helps to identify the existence of axonal dysfunction Citation[24,25]. The main drawback of the technique is that it requires lengthy examinations and the cooperation of the patient in sustaining the discomfort of the test while remaining still. Although repeated nerve insertions with the needle electrode has been shown to cause minor lesions in rats Citation[12,26], no overt or persistent nerve damage has been found in prospective studies in humans. Therefore microneurography is considered a safe technique Citation[27,28].
Several studies with microneurography have provided useful information in patients with small-fiber neuropathies. Ochoa et al. showed that patients with painful polyneuropathy have hyperexcitable polymodal and mechanical insensitive C nociceptors that could explain allodynia and hyperalgesia Citation[29]. Liguori et al. demonstrated the involvement of autonomic nerve sympathetic fibers in patients with small-fiber neuropathy Citation[30]. Schmidt et al. showed that multiple firing of C nociceptors is a mechanism related to neuropathic pain rather than to the neuropathy itself Citation[31]. Microneurography is currently the only method allowing for investigation of how peripheral nerve axons discharge under natural conditions Citation[32]. As such, it offers the well-trained person the opportunity of gathering precious information on many physiological and pathophysiological aspects of patients with painful neuropathies. It is noteworthy the fact that it can record positive sensory phenomena mediated by small myelinated and unmyelinated fibers Citation[33]. Peripheral nociceptors may undergo abnormal activation, with spontaneous ectopic discharges and repeated firing to isolated inputs that are most likely the primary cause of spontaneous and evoked pain in patients with peripheral neuropathies Citation[12,25,34].
Neurophysiological study of autonomic nervous system functions
The fibers of the autonomic nervous system are small unmyelinated nerve fibers, and therefore they are often involved in small-fiber neuropathies. Autonomic functions available for electrophysiological evaluation are sudomotor activity and the regulation of heartbeat frequency Citation[35], even though the most relevant disorders in patients with small-fiber neuropathy involve gastrointestinal functions, sexual functions and orthostatic hypotension.
The preferred neurophysiological method for recording the sudomotor activity is by means of surface electrodes in the palm and dorsum of the hand. Any stimulus that induces an emotional reaction, such as fear, stress or surprise, may generate changes in the galvanic resistance of the skin, which can be recorded as the electrodermal response to those stimuli. A synchronized activation of sudomotor glands also occurs after electrical stimuli, which is recorded as the sympathetic sudomotor skin response (SSSR). The SSSR tests somatosympathetic reflexes Citation[36]. One of the advantages of the SSSR is its availability to electrophysiological recording with a simple electromyograph Citation[4]. The disadvantages are that the response habituates quickly and varies widely with stimulus type and frequency, patient age, skin temperature and mental stress Citation[37]. The generation of the SSSR has been better understood since the studies of Mitani et al. Citation[38] and Toyokura and Takeda Citation[39]. After the electrical volley reaches the sweat gland, ions of Na+ and Cl− are pushed to the outside at the gland ductus. Na+ is actively reabsorbed and, owing to that, the outer end of the ductus becomes negative with respect to the inner part, giving rise to a negative deviation of the isoelectric line. When sweating is produced, Na+ ions accumulate at the exterior, changing the polarity difference between the outer and the inner part of the gland. Therefore, in view of its physiological mechanisms, the SSSRs can be divided into predominantly negative type, which derives from a sensory input of tolerable intensity and a predominantly positive type, which results from a relatively large intensity stimulus Citation[40,41]. A stimulus of similar intensity may elicit a SSSR of different shapes in different subjects according to the relative intensity of the perceived stimulus. In other words, the SSSR may be bigger and of predominantly positive shape in patients with hypervigilance.
The SSSR can be elicited by nociceptive stimuli Citation[42]. In this case, response latency is slightly longer than with other types of stimuli, due to the slowness of conduction in the small afferent fibers. Typically, this response habituates fast in healthy subjects. Therefore, the study of habituation could reveal the degree at which the subject has control of his or her emotional response to repeated sensory inputs. This could be an interesting method for the study of some syndromes presenting with pain. For instance, Schestatsky et al. Citation[43] studied patients with Parkinson’s disease who reported pain as one of their nonmotor signs Citation[44,45] with no known cause (no peripheral nerve lesions or soft tissue damage). These authors found that while the SSSR habituated fast in healthy subjects, it did not do so in patients with Parkinson’s disease, remaining with relatively high amplitude along a series of stimuli.
The variability of cardiac frequency is studied by recording the QRS complex from any thoracic site. Many electromyographs are supplied nowadays with the appropriate software for quantitation of the main variables derived from heartbeat frequency evaluation. Typically, the maneuvres requested to assess heartbeat frequency regulation (parasympathetic innervation) are as follows: heart rate (HR) response to normal and deep breathing, Valsalva ratio and HR response to standing (30:15 ratio). In the normal breathing test, the software calculates the ratio between the maximum and the minimum RR intervals along some 3 min or so, whereas in the deep breathing test, a 1-min baseline is recorded, which is immediately followed by eight consecutive breathing cycles. The subject breathes maximally at a rate of six breaths/min (inspiratory and expiratory cycles of 5 s each). The end point is the maximal HR variability. Valsalva ratio is derived from the shortest RR interval (the tachycardia) during or after Phase II of the maneuvre to the longest RR interval (the bradycardia) in Phase IV of the maneuvre occurring within 30 s of the peak HR. In the HR response to standing, the initial HR responses to standing consist of an initial tachycardia followed by a slight bradycardia, which are reflexly mediated through baroreceptors except for the initial part that may be part of the efferent command. The typical measure in the standing test is the 30:15 ratio (RR interval at beat 30)/(RR interval at beat 15), which is considered an index of cardiovagal function. In patients, the range of variability of the RR interval during these maneuvres is often reduced Citation[46]. A simple superimposition of a series of traces recorded with a delay line can be useful to measure the ‘jitter’. The number of recorded traces should allow for a meaningful result in epochs of 1–2 s . Usually, there is good correlation between the abnormalities in the control of heartbeat frequency and the abnormalities in maintenance of blood pressure in the same maneuvres, leading to orthostatic hypotension in postural changes Citation[47].
More complex measures have been used by some authors. Usually, the RR interval is proportional to the QT interval. This correlation may become abnormal in patients with autonomic dysfunction Citation[48]. In healthy subjects, any stimulus that causes an emotional reaction should trigger a change in the RR interval, with an initial enhancement of the heartbeat frequency. However, in CNS dysfunctions of autonomic regulation, a strong stimulus capable of inducing a muscle reaction in the context of a startle does not trigger the expected change in cardiac frequency Citation[49].
Nociceptive-evoked potentials mediated by radiant heat & contact heat stimuli
Electrical stimuli can be of an intensity high enough to induce pain; however, even if such a high-intensity stimulus is applied, the evoked potentials obtained would not be related to activation of pain fibers but to the same afferent fibers as those being activated with stimuli of low intensity (i.e., large diameter fibers). This is because of the rule known as ‘the first come first served’ Citation[50], indicating that the first volley arriving to the brain activates the centers leading to the evoked potential. The second volley would find refractory pathways and would not be able to lead to another sufficiently salient cerebral-evoked potential within a certain time period. Technical advances have brought up the possibility to use stimuli that selectively activate thermoalgesic receptors to elicit nociceptive-evoked potentials. Mor and Carmon were the first to use radiant heat to stimulate the skin through a laser beam to induce the laser-evoked potentials (LEPs) Citation[51]. However, the most relevant contributions of this method for clinical practice in the analysis of pain did not take place until 20 years later Citation[52,53]. There are many types of laser stimulators, but the one that has been most used so far is the CO2 laser stimulator. The authors use a prototype CO2 laser (AGM, Barcelona), which emits a nonspread beam of 2 mm2 diameter, with a power of up to 15 W and a duration that can vary between 5 and 100 ms. Therefore, the stimulus intensity is changed by modifying the duration. Suitable stimulus intensity is approximately 40 mJ/mm2.
CO2 laser stimuli applied to hairy skin excite exclusively mechano-thermal nociceptors, from which inputs are conveyed by Ad- and C-fibers in the peripheral nerves and via the spinothalamic tracts in the spinal cord. They connect to several centers in the brainstem before reaching the relay thalamic nuclei that lead to somatosensory perception areas of the brain Citation[54,55]. Scalp surface electrodes (active: vertex; reference: linked earlobes) record a late potential after a single stimulus to the dorsum of the hand; latency varies as a function of the subject’s height, age, attention and arousal, among other factors. In our department, the mean latency of the N2 peak was 217.1 ± 35 ms in 12 healthy young volunteers Citation[56]. Averaging some traces helps in obtaining a larger potential by improving the signal-to-noise ratio. Many contributions from various authors have characterized the physiology of LEPs Citation[57–59]. The potential recorded in the vertex (Cz) is probably generated in deep cortical layers (anterior cingulate cortex and insula) and is termed the N2/P2 complex, while the potential recorded in the parieto–temporal areas probably corresponds to the secondary sensory cortex and is termed the N1 potential. The N1 potential is somehow phase reversed with respect to the N2 potential. Both LEP waveforms (N1 and N2) result from the synchronization of activity in a group of neurons receiving the inputs from nociceptive afferents. However, they are not just indicating the arrival of the input. Instead, they reflect the integration of the signal in cortical sensory circuits in such a way that, in healthy subjects, the subjective sensation of pain correlates with the amplitude of cortical LEPs rather than with the physical stimulus intensity. This can be demonstrated by the fact that when attention is directed to the laser stimulus, the size of the LEP increases together with the subjective pain sensation and, on the opposite, both of them decrease when attention is diverted away from the stimulus Citation[60,61]. In its afferent path, the nociceptive volley induced by laser stimuli will activate many structures and circuits at the spinal cord, brainstem and encephalon. Even though LEPs are the best known event, nociceptive stimuli will also produce responses or reactions that can be evaluated if appropriate recording methods are used. The blink reflex can be obtained after facial laser stimulation Citation[62] and may be useful for the assessment of trigeminal small-fiber function. Moreover, the laser stimulus applied to the hand dorsum can be used as a prepulse to inhibit the blink reflex Citation[63]. The SSSR can be recorded from the palm of the hand and is useful for the assessment of autonomic reactions to nociceptive stimuli Citation[42]. Reaction time can be monitored with appropriate electromyography or movement recording Citation[64] and reflects the sensorimotor integrative processes after laser stimulation. Using a scanner-controlled large area of stimulation, Mørch et al. were able to elicit a withdrawal reaction in healthy subjects, offering a new tool for testing nociceptive reflexes and the modulation of trigemino–facial reflexes Citation[65]. Finally, a method based on Libet’s clock Citation[66] can be used to evaluate the conscious perception of the stimulus, which seems to be correlated with the evoked potential amplitude Citation[56].
LEPs have demonstrated their ability to detect lesions in peripheral and central pain pathways, including small-fiber neuropathies and CNS lesions in a series of research studies Citation[52,53,58,67]. LEPs are particularly suitable for unilateral lesions that allow for comparison between sides Citation[68,69]. In order to show symmetrical abnormalities in distal small-fiber neuropathies, comparison with age and sex-matched healthy individuals is necessary Citation[53,58,70,71]. Usually, LEPs are absent to stimuli applied to the feet in metabolic or toxic polyneuropathies. However, unfortunately, not all healthy subjects have consistent LEPs to feet stimuli and the observation cannot always be considered significant. In some patients with distal axonal polyneuropathy, LEPs can be obtained to stimuli applied to relatively proximal sites in the lower limbs in the absence of feet stimulation, but this is not a consistent finding either, since the density of nociceptors is not the same in different skin sites.
Another method has appeared more recently as an alternative to laser stimuli. This is contact heat stimulation, induced by a thermofoil thermode that can increase the surface temperature at a very fast rate, which is felt as a pinprick. This induces contact heat-evoked potentials (CHEPs). The main advantage of this method is the absence of the potential skin damage produced by laser stimuli. Contact heat stimuli are based on early methods used for quantitative thermal testing Citation[72]. Technology has made it possible to increase the temperature of the thermode at a very fast rate (up to 70°C/s). This takes approximately 300°ms for a thermode at 32°C to reach a peak temperature of 52°C. The sensation is more of pricking pain than of burning and it is similar to the one induced by laser stimuli, inducing similar evoked potentials Citation[73]. Certain differences can be appreciated between LEPs and CHEPs. The obvious difference is that with contact heat stimuli the activation of pain fibers occurs while there is a simultaneous skin contact and therefore some activation of pressure receptors. However, keeping the pressure of the thermode over the skin constant diminishes the possible interference between the two types of stimuli. The area of stimulation is also different. With the contact heat, the stimulation area is that of a circumference of approximately 3-cm diameter, while with the radiant heat, the stimulus is of a few millimeters in diameter. Therefore, the number of receptors activated with contact heat is bigger than with the laser, leading to different mechanisms of spatial and temporal summation. Finally, the duration of the stimulus is also different. With contact heat, the stimulus temperature rises continuously within a range of 20–25°C for approximately 300 ms, while with laser, the temperature reaches a very high value for a very short time. There are so far not many studies comparing the results of the two techniques in healthy subjects or patients. In healthy subjects, Iannetti et al. reported similar differences between stimuli to the dorsum and to the palm of the hand in CHEPs and LEPs Citation[74]; Valls-Solé et al. found no differences between the two types of stimuli in the conscious awareness of stimulus perception Citation[56]. In patients with small-fiber polyneuropathies, Casanova-Mollá et al. found that LEPs were more sensitive than CHEPs to the axonal damage, while CHEPs were better correlated than LEPs with the density of intraepidermal fibers Citation[71]. shows nociceptive-evoked potentials to hand and feet stimulation in a healthy volunteer and in a patient with painful polyneuropathy.
Other techniques have been developed for the study of nociceptive-evoked potentials. A promising technique is the one using small electrical stimuli delivered with a special concentric planar electrode Citation[75,76]. The tip of the electrode is inserted into the skin but, owing to its very short length, it remains at epidermic level. The high impedance of the electrode, with reference to the disc above the skin, is able to produce a very focused stimulation. The small cathode–anode distance results in a high current density with a small current intensity. Thus, the depolarization is limited to the epidermis and superficial layers of the dermis containing Ad- and C-fibers Citation[77]. The system allows for adjusting the intensity according to the individual pain threshold and site of stimulation. The concentric electrode evoked potentials have a similar waveform as those produced by laser stimulation Citation[78] and also have been correlated with the epidermal nerve fiber density in patients with peripheral neuropathies, mainly HIV-related neuropathy Citation[77,79]. However, concentric electrode evoked potentials latency is significantly shorter than LEPs latency Citation[80], suggesting that some coactivation of Ad and Ab fibers takes place with the concentric electrode stimuli. This should be taken into account in further studies in evaluating the nociceptive system.
Although reference values of amplitude and latency for the main components of LEPs and CHEPs in healthy subjects are available, no study has been specifically performed yet to assess the normative range for clinical use. Some authors have reported an effect of age on the CHEPs amplitude and of gender on CHEPs latency Citation[81]. However, further studies are warranted to establish the reliability of the nociceptive evoked potentials for diagnostic and as a measure of outcome for prospective studies, especially in small-fiber neuropathies.
Other tests using a neurophysiological approach
Chronic pain, whether neuropathic or nociceptive in origin, is a subjective feeling that has to be regarded as a very personal condition. At present, there are no methods appropriate for quantifying pain. However, there are systems that allow for recognizing the neuropathic deficit and simultaneously provide information on how subjects perceive the sensory abnormalities derived from such deficit. The authors present data about two of them: psychophysical tests and functional magnetic resonance.
Psychophysical testing
Behavioral studies of patients with neuropathic pain should be an important part of their evaluation. Apart from psychological tests, psychophysical exams are also appropriate for the assessment. The simplest technique is known as quantitative sensory testing, which is used to determine the thresholds to standard stimuli of various sensory modalities Citation[82]. There are two main methods to measure the threshold to a given sensation: the method of levels and the method of limits Citation[72]. In the method of levels, subjects are given one stimulus and are asked to report whether they have felt it once the stimulus is over. If they report not feeling it, the intensity is increased and if they felt the stimulus the intensity is decreased in such a way that a level of sensation is determined after several trials. In the method of limits, subjects are requested to be ready to indicate when they feel the sensation by pressing a button or reacting in some other form. This is a faster method that has the small inconvenience that the reaction time of the subject counts as a time in which the stimulus intensity continues growing.
The most useful psychophysical tests are those used for the quantitation of thermal sensation, based on the use of thermodes. These are composed of a thin highly conductive plate, refrigerated by water, which can reach the desired temperature at a rate up to 4°C/s Citation[83]. The experimenter triggers the change in temperature, and the subject is requested to stop the ongoing change at perception. Typically, the threshold for warm perception, measured with the method of limits, shows a slight tendency to decrease, while the threshold for pain shows a tendency to increase, with repetition of stimuli in healthy subjects. Many patterns can be seen in patients Citation[84], but the most characteristic in distal polyneuropathies is the decrease in warm perception, with a narrow separation between perception of warm (hypesthesia) and perception of pain (hyperalgesia). A strategy to examine patients with suspected small-fiber polyneuropathy is to test distal and proximal points to identify the distal predominance of the involvement.
A slightly more sophisticated method is to reproduce the sensation using an electronic visual analogue scale score system, present the stimuli with a very slow change in temperature (0.5°C/s) and request that subjects describe continuously their perceived sensation for the duration of the test. This dynamic psychophysical testing Citation[85] allows for the observation of the time the subjects take to perceive the temperature change, a parameter that depends on factors such as adaptation, refractoriness, sensitization and others, qualities attributed to different neural structures along the pain pathway. The slow rising of temperature is usually marked by healthy subjects up to a certain temperature level (usually between 37 and 40°C), where they stop marking an increase for some 2–4°C, to resume again to rise the sensation up to pain levels . If the recording of the electrodermal activity is included in the test, the experimenter would obtain information on the subject’s emotional reactivity to the perception of the stimulus Citation[86]. If more than one stimulus is presented with variable interstimulus interval, it is also possible to measure the influence of one stimulus to the response to a subsequent stimulus of the same characteristics Citation[85]. Finally, if subjects are allowed to mark their perception in both sides out of the basal temperature (warm and cold), healthy subjects usually mark cold when the temperature descends slowly from the peak to baseline at a 0.5°C/S rate, even if temperature does not reach cold sensation level Citation[87]. This is probably due to the activation of cold receptors by temperature drop. Interestingly, this cool sensation is usually not marked by patients with small-fiber neuropathy, reflecting the possible abnormality of the fine tuning of temperature receptors in distal axonal damage . Since neural transmission for cold is mediated through specific ionic channels Citation[88], there is potential for clinical applicability in the assessment of how subjects perceive the rapid change in temperature that leads to the cool sensation in our test.
Functional magnetic resonance
While psychophysical tests may tell us about the behavior of the subject to specific stimuli, functional neuroimaging indicates the areas of the brain that are more involved in processing of pain stimuli. Functional neuroimaging techniques include functional fMRI, positron emission tomography and single photon emission computed tomography. The fMRI has the advantage in front of the PET and single photon emission computed tomography of not needing radioisotope contrast to produce a quantifiable representation of the examined function. Authors have used these techniques to investigate the spatial representation of pain in the human brain and how this is modified in pain syndromes or pain therapy, as well as in physiological experimentation Citation[89,90,91]. One of the most influential findings of functional neuroimaging of pain has been the definition of the pain matrix Citation[92], as the brain areas thought to be preferentially involved in the perception of pain. These comprise the primary and secondary sensory cortices, the insula, the anterior cingulate cortex, the thalamus and the prefrontal cortex. However, recent studies have challenged the specificity of these areas for pain perception. Mouraux et al. have investigated the activation of brain areas to stimuli of different modalities and concluded that the largest part of the fMRI responses elicited by phasic nociceptive stimuli reflects non nociceptive-specific cognitive processes that would be probably similar to those obtained using other stimulus modalities Citation[93]. Furthermore, it is possible that the pain matrix, or the allodynia network, as reported by Moisset and Bouhassira Citation[94], is different for experimentally induced acute pain in healthy subjects than for chronic neuropathic pain in patients. In spite of that, many studies have been carried out on the anatomical localization of pain processing areas in the human brain in healthy subjects and patients Citation[95,96]. Results are not always homogenous between groups studying patients with neuropathic pain. Slightly different scalp topography and methodological particularities may be responsible for intergroup differences. However, physiologically, there are reasons to lead to both, reduced signal and increased signal in patients with neuropathic pain. If there is less input because of functional loss of nociceptive fibers, the area of the brain activated in fMRI would be reduced. But the same inputs may be amplified at their arrival to perception areas and perhaps other brain areas, leading to a larger activation area. Apart from regions involved in the feeling of pain, funcitonal neuroimaging techniques allow for defining tracks or networks. Cauda et al. have used resting state and spatial independent component analysis to determine the default mode network of spontaneous pain in a group of eight diabetic patients with painful polyneuropathy Citation[97]. They found a general reduction of the default mode network but an increase in some areas related with pain. This is a promising technique for identifying the pathways of nociception in the brain.
In some studies, the authors have combined neurophysiological tests with fMRI tests Citation[98,99]. In these studies, the authors point at the similarities in the recording of the nociceptive evoked potentials with EEG during an fMRI session and those recorded in the neurophysiology laboratory. While magnetic resonance makes for an excellent spatial resolution, evoked potential and electroencephalographic recordings in general provide for an excellent temporal resolution and, therefore, the two techniques combined should facilitate in the near future our understanding on the processing of nociceptive stimuli.
Conclusion
Even if we use the most sophisticated tools available at present, we still have no way to objectively assess pain itself. It is noteworthy that an important group of nociceptive afferents establish functional connections at brainstem level where sensory volleys are integrated into circuits that are relatively poorly known and have more speculative than proven functions. This is the case with the descending noxious inhibitory control system or other circuits leading to defense or attack after an unexpectedly painful stimulus. The technological advance in clinical neurophysiology has contributed to improving our knowledge on the consequences of a lesion in the peripheral and CNS. We now know how to activate solely or mainly the nociceptive pathway and test its integrity from the receptors in the skin to the structures dealing with perception at the CNS. We can even semiquantify the sensory experiences and establish diagnostic and treatment algorithms. However, the individual’s emotional reaction to pain is still the core of the patient’s problem that all those interested on painful neuropathies face in our clinical work.
Expert commentary
Clinical neurophysiology is still the most appropriate technique for the study of lesions inducing neuropathic pain, even though the combination of neurophysiological techniques with the assessment of intraepidermal nerve fiber density after skin biopsy may add significantly to the outcome. Both, conventional and specific neurophysiological, techniques are informative for understanding of the underlying neuropathic pain mechanisms and for clinical purposes. Among the specific techniques, laser and contact heat stimuli are useful not only for the study of nociceptive evoked potentials but also for the assessment of additional responses such as the sudomotor skin response, reaction time, conscious awareness of the stimulus or others. Further works comparing the two types of stimuli are needed to find out whether the differences may open new avenues of research in the field of neuropathic pain.
The correlation between neurophysiological techniques and neuroimaging will also bring future insight on how our brain processes inputs that are considered painful. In this field, more than in others, understanding of the physiological mechanisms of pain processing should go in parallel with clinical assessment and therapeutic approaches. Many patients with inexplicable pain syndromes might well be candidates for physiological studies together with healthy subjects and patients with clear neuropathic lesions, to find out the substrate for pain sensation.
Five-year view
It would be too daring to state how the field in neurophysiological assessment of painful neuropathies would develop in 5 years time. This is one of the fields in which clinical neurophysiology is still a relevant method for the diagnosis and we foresee that it will continue in that position for many years. Technical development may help. There is need for the development of better approaches to direct assessment of small fibers in peripheral nerves. The closest one is nowadays microneurography; but this technique cannot be considered yet as of full clinical applicability because it is too time consuming and dependent on the skills of the experts. Technical research is expensive and there is only a minority of researchers devoted in the field of neurophysiology. Nevertheless, we foresee some interesting advances in this area in 5 years from now.
Combination of electroencephalography and magnetic resonance imaging is another area of growth. Neurophysiology provides good temporal resolution of events and magnetic resonance provides good spatial resolution. The combination of both techniques should lead to know better the sources of evoked potentials (nociceptive and non-nociceptive) and to better determine the meaning of this cerebral event and its relation to the feeling of pain. The same applies for the recognition of descending inhibitory pathways for nociceptive control. This is a concept born in neurophysiological studies that may find an anatomical correlate through functional magnetic resonance studies.
Finally, neurophysiology should grow in the field of pain together with other approaches from different areas. For instance, psychophysical testing may be limited to threshold determination but it increases its yield if combined with neurophysiological methods. Dynamic sensory testing allows for a more detailed subjective description of the sensations than just threshold determination. The time it takes for a normal subject to feel heat pain may differ according to context and may be that this is a distinctive aspect of patients with painful neuropathy. A very small number of publications have dealt with this aspect so far but we think it will develop further in the near future.
The ultimate goal of all studies on pain is to find a specific therapeutic agent but if we do not know well all the physiological details involved in impulse conduction, synaptic transmission and sensory perception, we will be hardly able to find an agent that modifies (improves) the function of what is wrong.
Box 1. Relevant points in conventional electrodiagnosis of peripheral nerve lesions.
• What about lesion topography?
– It is important in focal neuropathies due to compression. Sensory nerve action potentials are usually preserved in preganglionic lesions (radiculopathy) and affected in postganglionic lesionis (plexopathies)
• What about neuropathy distribution?
– The assessment of mononeuropathy, polyneuropathy or multineuritis is an important point in the clinical and electrophysiological diagnosis of peripheral nerve diseases
• Is the lesion axonal or demyelinating?
– Most of them result in presenting denervation, which usually indicates axonal damage. If it affects unmyelinated fibers, the lesion is axonal
• Degree of focal nerve lesion severity
– The important information is in the amplitude of the compound motor action potential to stimuli distal to the lesion
• What is the relevance of reinnervation signs?
– Reinnervation is a necessary requirement for recovery of function but there can be reinnervation errors leading to long-lasting dysfunctions
Key issues
• The symptom pain cannot be quantified by any available technique so far. Therefore, in neuropathic pain patients, the assessment should be done on the lesions suspected to cause pain.
• Distal axonal polyneuropathies involving small fibers are the most common causes of neuropathic pain. This can be the case with diabetic, alcoholic, toxic, immune, paraneoplastic or other kind of neuropathies.
• Clinical skills are still the most important asset for the assessment of painful neuropathies. Conventional electrophysiological studies should be complemented with techniques that specifically assess nerve conduction and function in small fibers.
• Disorders in autonomic nervous system functions may be a warning sign of small-fiber neuropathy. Orthostatic hypotension, gastrointestinal disorders or sexual dysfunction might be important cues to characterize the type of neuropathy.
• Stimuli with either radiant heat (laser) or contact heat (thermode) are the most frequently used methods to induce inputs in the nociceptive pathway. Other modalities may lead to promising results, but their clinical applicability is not yet clear.
• Psychophysical testing should be included in the assessment of small-fiber neuropathies. It provides the opportunity to quantify the deficit in thermal sensation through the subject’s own perception of such deficit.
• The combined use of neurophysiological techniques, psychophysical tests and functional magnetic resonance may bring future insight in pain processing and may eventually lead to finding the substrate for pain sensation.
Financial & competing interests disclosure
The authors have no relevant affiliations or financial involvement with any organization or entity with a financial interest in or financial conflict with the subject matter or materials discussed in the manuscript. This includes employment, consultancies, honoraria, stock ownership or options, expert testimony, grants or patents received or pending, or royalties.
No writing assistance was utilized in the production of this manuscript.
References
- Treede RD, Jensen TS, Campbell JN et al. Neuropathic pain: redefinition and a grading system for clinical and research purposes. Neurology 70(18), 1630–1635 (2008).
- Cruccu G, Sommer C, Anand P et al. EFNS guidelines on neuropathic pain assessment: revised 2009. Eur. J. Neurol. 17(8), 1010–1018 (2010).
- Jensen TS, Baron R, Haanpää M et al. A new definition of neuropathic pain. Pain 152(10), 2204–2205 (2011).
- Haanpää M, Attal N, Backonja M et al. NeuPSIG guidelines on neuropathic pain assessment. Pain 152(1), 14–27 (2011).
- Lefaucheur JP, Créange A. Neurophysiological testing correlates with clinical examination according to fibre type involvement and severity in sensory neuropathy. J. Neurol. Neurosurg. Psychiatr. 75(3), 417–422 (2004).
- Valls-Solé J, Montero J. Role of EMG evaluation in muscle hyperactivity syndromes. J. Neurol. 251(3), 251–260 (2004).
- Ochoa J, Torebjörk HE, Culp WJ, Schady W. Abnormal spontaneous activity in single sensory nerve fibers in humans. Muscle Nerve 5(9S), S74–S77 (1982).
- Deuschl G, Lücking CH. Physiology and clinical applications of hand muscle reflexes. Electroencephalogr. Clin. Neurophysiol. Suppl. 41, 84–101 (1990).
- Kofler M, Poustka K. Ipsi- and contralateral exteroceptive EMG modulation in uni- and bilaterally activated thenar muscles. Clin. Neurophysiol. 116(2), 300–307 (2005).
- Chaplan SR, Guo HQ, Lee DH et al. Neuronal hyperpolarization-activated pacemaker channels drive neuropathic pain. J. Neurosci. 23(4), 1169–1178 (2003).
- Aurilio C, Pota V, Pace MC, Passavanti MB, Barbarisi M. Ionic channels and neuropathic pain: physiopathology and applications. J. Cell. Physiol. 215(1), 8–14 (2008).
- Serra J, Bostock H, Solà R et al. Microneurographic identification of spontaneous activity in C-nociceptors in neuropathic pain states in humans and rats. Pain 153(1), 42–55 (2012).
- Agius E, Cochard P. Comparison of neurite outgrowth induced by intact and injured sciatic nerves: a confocal and functional analysis. J. Neurosci. 18(1), 328–338 (1998).
- Valls-Sole J, Castillo CD, Casanova-Mollá J, Costa J. Clinical consequences of reinnervation disorders after focal peripheral nerve lesions. Clin. Neurophysiol. 122(2), 219–228 (2011).
- Zimmermann M. Pathobiology of neuropathic pain. Eur. J. Pharmacol. 429(1–3), 23–37 (2001).
- Lee J, Dougherty PM, Antezana D, Lenz FA. Responses of neurons in the region of human thalamic principal somatic sensory nucleus to mechanical and thermal stimuli graded into the painful range. J. Comp. Neurol. 410(4), 541–555 (1999).
- Zhang H, Xie W, Xie Y. Spinal cord injury triggers sensitization of wide dynamic range dorsal horn neurons in segments rostral to the injury. Brain Res. 1055(1–2), 103–110 (2005).
- Quinn KP, Dong L, Golder FJ, Winkelstein BA. Neuronal hyperexcitability in the dorsal horn after painful facet joint injury. Pain 151(2), 414–421 (2010).
- Renn CL, Carozzi VA, Rhee P, Gallop D, Dorsey SG, Cavaletti G. Multimodal assessment of painful peripheral neuropathy induced by chronic oxaliplatin-based chemotherapy in mice. Mol. Pain 7, 29 (2011).
- Willer JC. [Clinical exploration of nociception with the use of reflexologic techniques]. Neurophysiol. Clin. 20(5), 335–356 (1990).
- Sandrini G, Arrigo A, Bono G, Nappi G. The nociceptive flexion reflex as a tool for exploring pain control systems in headache and other pain syndromes. Cephalalgia 13(1), 21–27 (1993).
- Torebjörk E. Human microneurography and intraneural microstimulation in the study of neuropathic pain. Muscle Nerve 16(10), 1063–1065 (1993).
- Bostock H, Campero M, Serra J, Ochoa JL. Temperature-dependent double spikes in C-nociceptors of neuropathic pain patients. Brain 128(Pt 9), 2154–2163 (2005).
- Serra J, Campero M, Bostock H, Ochoa J. Two types of C nociceptors in human skin and their behavior in areas of capsaicin-induced secondary hyperalgesia. J. Neurophysiol. 91(6), 2770–2781 (2004).
- Schmelz M, Forster C, Schmidt R, Ringkamp M, Handwerker HO, Torebjörk HE. Delayed responses to electrical stimuli reflect C-fiber responsiveness in human microneurography. Exp. Brain Res. 104(2), 331–336 (1995).
- Fried K, Frisén J, Mozart M. De- and regeneration of axons after minor lesions in the rat sciatic nerve. Effects of microneurography electrode penetrations. Pain 36(1), 93–102 (1989).
- Eckberg DL, Wallin BG, Fagius J, Lundberg L, Torebjörk HE. Prospective study of symptoms after human microneurography. Acta Physiol. Scand. 137(4), 567–569 (1989).
- Jørum E, Schmelz M. Chapter 29 microneurography in the assessment of neuropathic pain. Handb. Clin. Neurol. 81, 427–438 (2006).
- Ochoa JL, Campero M, Serra J, Bostock H. Hyperexcitable polymodal and insensitive nociceptors in painful human neuropathy. Muscle Nerve 32(4), 459–472 (2005).
- Liguori R, Giannoccaro MP, Di Stasi V et al. Microneurographic evaluation of sympathetic activity in small fiber neuropathy. Clin. Neurophysiol. 122(9), 1854–1859 (2011).
- Schmidt R, Kleggetveit IP, Namer B et al. Double spikes to single electrical stimulation correlates to spontaneous activity of nociceptors in painful neuropathy patients. Pain 153(2), 391–398 (2012).
- Vallbo AB, Hagbarth KE. Activity from skin mechanoreceptors recorded percutaneously in awake human subjects. Exp. Neurol. 21(3), 270–289 (1968).
- Ochoa J, Torebjörk E. Sensations evoked by intraneural microstimulation of C nociceptor fibres in human skin nerves. J. Physiol. 415, 583–599 (1989).
- Orstavik K, Jørum E. Microneurographic findings of relevance to pain in patients with erythromelalgia and patients with diabetic neuropathy. Neurosci. Lett. 470(3), 180–184 (2010).
- Dotson RM. Clinical neurophysiology laboratory tests to assess the nociceptive system in humans. J. Clin. Neurophysiol. 14(1), 32–45 (1997).
- Shahani BT, Halperin JJ, Boulu P, Cohen J. Sympathetic skin response – a method of assessing unmyelinated axon dysfunction in peripheral neuropathies. J. Neurol. Neurosurg. Psychiatr. 47(5), 536–542 (1984).
- Hoeldtke RD, Davis KM, Hshieh PB, Gaspar SR, Dworkin GE. Autonomic surface potential analysis: assessment of reproducibility and sensitivity. Muscle Nerve 15(8), 926–931 (1992).
- Mitani H, Ishiyama Y, Hashimoto I. Equivalent current dipole estimated from SSR potential distribution over the human hand. Sympathetic skin response. Clin. Neurophysiol. 114(2), 233–238 (2003).
- Toyokura M, Takeda H. Waveform of sympathetic skin response in diabetic patients. Clin. Neurophysiol. 112(7), 1229–1236 (2001).
- Toyokura M. Waveform of sympathetic skin response. Suppl. Clin. Neurophysiol. 57, 352–357 (2004).
- Toyokura M. Sympathetic skin responses: the influence of electrical stimulus intensity and habituation on the waveform. Clin. Auton. Res. 16(2), 130–135 (2006).
- Cervera A, Veciana M, Valls-Solé J. Sympathetic sudomotor skin responses induced by laser stimuli in normal human subjects. Neurosci. Lett. 334(2), 115–118 (2002).
- Schestatsky P, Ehlers JA, Rieder CR, Gomes I. Evaluation of sympathetic skin response in Parkinson’s disease. Parkinsonism Relat. Disord. 12(8), 486–491 (2006).
- Poewe W. Non-motor symptoms in Parkinson’s disease. Eur. J. Neurol. 15(Suppl. 1), 14–20 (2008).
- Chaudhuri KR, Healy DG, Schapira AH; National Institute for Clinical Excellence. Non-motor symptoms of Parkinson’s disease: diagnosis and management. Lancet Neurol. 5(3), 235–245 (2006).
- Low P, Sletten D. Laboratory evaluation of autonomic failure. In: Clinical Autonomic Disorders (3rd Edition). Low PA, Benarroch EE (Eds). Lippincott Williams & Wilkins, Philadelphia, PA, USA, 130–160 (2008).
- Goldstein DS, Tack C. Noninvasive detection of sympathetic neurocirculatory failure. Clin. Auton. Res. 10(5), 285–291 (2000).
- Parisi L, Valente G, Serrao M et al. RR interval variation, sympathetic skin reflex and QT dispersion in the assessment of autonomic function in peripheral neuropathy. Electromyogr. Clin. Neurophysiol. 39(8), 461–468 (1999).
- Valls-Solé J, Veciana M, Leon L, Valldeoriola F. Effects of a startle on heart rate in patients with multiple system atrophy. Mov. Disord. 17(3), 546–549 (2002).
- Garcia-Larrea L. Somatosensory volleys and cortical evoked potentials: ‘first come, first served’? Pain 112(1–2), 5–7 (2004).
- Mor J, Carmon A. Laser emitted radiant heat for pain research. Pain 1(3), 233–237 (1975).
- Bromm B, Treede RD. Laser-evoked cerebral potentials in the assessment of cutaneous pain sensitivity in normal subjects and patients. Rev. Neurol. 147(10), 625–643 (1991).
- Wu Q, García-Larrea L, Mertens P, Beschet A, Sindou M, Mauguière F. Hyperalgesia with reduced laser evoked potentials in neuropathic pain. Pain 80(1–2), 209–214 (1999).
- Willis WD, Westlund KN. Neuroanatomy of the pain system and of the pathways that modulate pain. J. Clin. Neurophysiol. 14(1), 2–31 (1997).
- Willis WD Jr. The somatosensory system, with emphasis on structures important for pain. Brain Res. Rev. 55(2), 297–313 (2007).
- Valls-Solé J, Castellote JM, Kofler M, Casanova-Mollá J, Kumru H, Schestatsky P. Awareness of temperature and pain sensation. J. Pain 13(7), 620–627 (2012).
- Plaghki L, Mouraux A. How do we selectively activate skin nociceptors with a high power infrared laser? Physiology and biophysics of laser stimulation. Neurophysiol. Clin. 33(6), 269–277 (2003).
- Treede RD, Lorenz J, Baumgärtner U. Clinical usefulness of laser-evoked potentials. Neurophysiol. Clin. 33(6), 303–314 (2003).
- Cruccu G, García-Larrea L. Clinical utility of pain – laser evoked potentials. Suppl. Clin. Neurophysiol. 57, 101–110 (2004).
- Liu CC, Ohara S, Franaszczuk PJ, Lenz FA. Attention to painful cutaneous laser stimuli evokes directed functional connectivity between activity recorded directly from human pain-related cortical structures. Pain 152(3), 664–675 (2011).
- Legrain V, Perchet C, García-Larrea L. Involuntary orienting of attention to nociceptive events: neural and behavioral signatures. J. Neurophysiol. 102(4), 2423–2434 (2009).
- Cruccu G, Romaniello A, Amantini A, Lombardi M, Innocenti P, Manfredi M. Assessment of trigeminal small-fiber function: brain and reflex responses evoked by CO2-laser stimulation. Muscle Nerve 22(4), 508–516 (1999).
- Valls-Solé J, Veciana M, Serra J, Cruccu G, Romaniello A. Prepulse inhibition of the blink reflex by laser stimuli in normal humans. Neurosci. Lett. 286(2), 79–82 (2000).
- Andersen OK, Jensen LM, Brennum J, Arendt-Nielsen L. Evidence for central summation of C and A delta nociceptive activity in man. Pain 59(2), 273–280 (1994).
- Mørch CD, Andersen OK, Graven-Nielsen T, Arendt-Nielsen L. Nociceptive withdrawal reflexes evoked by uniform-temperature laser heat stimulation of large skin areas in humans. J. Neurosci. Methods 160(1), 85–92 (2007).
- Libet B, Gleason CA, Wright EW, Pearl DK. Time of conscious intention to act in relation to onset of cerebral activity (readiness-potential). The unconscious initiation of a freely voluntary act. Brain 106 (Pt 3), 623–642 (1983).
- Truini A, Romaniello A, Galeotti F, Iannetti GD, Cruccu G. Laser evoked potentials for assessing sensory neuropathy in human patients. Neurosci. Lett. 361(1–3), 25–28 (2004).
- Galeotti F, Truini A, Cruccu G. Neurophysiological assessment of craniofacial pain. J. Headache Pain 7(2), 61–69 (2006).
- Veciana M, Valls-Solé J, Schestatsky P, Montero J, Casado V. Abnormal sudomotor skin responses to temperature and pain stimuli in syringomyelia. J. Neurol. 254(5), 638–645 (2007).
- Agostino R, Cruccu G, Romaniello A, Innocenti P, Inghilleri M, Manfredi M. Dysfunction of small myelinated afferents in diabetic polyneuropathy, as assessed by laser evoked potentials. Clin. Neurophysiol. 111(2), 270–276 (2000).
- Casanova-Mollá J, Grau-Junyent JM, Morales M, Valls-Solé J. On the relationship between nociceptive evoked potentials and intraepidermal nerve fiber density in painful sensory polyneuropathies. Pain 152(2), 410–418 (2011).
- Fruhstorfer H, Lindblom U, Schmidt WC. Method for quantitative estimation of thermal thresholds in patients. J. Neurol. Neurosurg. Psychiatr. 39(11), 1071–1075 (1976).
- Valeriani M, Le Pera D, Niddam D, Chen AC, Arendt-Nielsen L. Dipolar modelling of the scalp evoked potentials to painful contact heat stimulation of the human skin. Neurosci. Lett. 318(1), 44–48 (2002).
- Iannetti GD, Zambreanu L, Tracey I. Similar nociceptive afferents mediate psychophysical and electrophysiological responses to heat stimulation of glabrous and hairy skin in humans. J. Physiol. 577(Pt 1), 235–248 (2006).
- Kaube H, Katsarava Z, Käufer T, Diener H, Ellrich J. A new method to increase nociception specificity of the human blink reflex. Clin. Neurophysiol. 111(3), 413–416 (2000).
- Inui K, Kakigi R. Pain perception in humans: use of intraepidermal electrical stimulation. J. Neurol. Neurosurg. Psychiatr. 83(5), 551–556 (2012).
- Obermann M, Katsarava Z, Esser S et al. Correlation of epidermal nerve fiber density with pain-related evoked potentials in HIV neuropathy. Pain 138(1), 79–86 (2008).
- Mouraux A, Iannetti GD, Plaghki L. Low intensity intra-epidermal electrical stimulation can activate Ad-nociceptors selectively. Pain 150(1), 199–207 (2010).
- Katsarava Z, Yaldizli O, Voulkoudis C, Diener HC, Kaube H, Maschke M. Pain related potentials by electrical stimulation of skin for detection of small-fiber neuropathy in HIV. J. Neurol. 253(12), 1581–1584 (2006).
- de Tommaso M, Santostasi R, Devitofrancesco V et al. A comparative study of cortical responses evoked by transcutaneous electrical vs CO(2) laser stimulation. Clin. Neurophysiol. 122(12), 2482–2487 (2011).
- Chao CC, Hsieh ST, Chiu MJ, Tseng MT, Chang YC. Effects of aging on contact heat-evoked potentials: the physiological assessment of thermal perception. Muscle Nerve 36(1), 30–38 (2007).
- Chong PS, Cros DP. Technology literature review: quantitative sensory testing. Muscle Nerve 29(5), 734–747 (2004).
- Triplett B, Ochoa J. Contemporary techniques in assessing peripheral nervous system function. Am. J. EEG Technol. 30, 29–44 (1990).
- Verdugo R, Ochoa JL. Quantitative somatosensory thermotest. A key method for functional evaluation of small calibre afferent channels. Brain 115 (Pt 3), 893–913 (1992).
- Schestatsky P, Algaba R, Pérez D et al. Transient decrease of sensory perception after thermoalgesic stimuli for quantitative sensory testing. Muscle Nerve 36(4), 466–470 (2007).
- Schestatsky P, Callejas MA, Valls-Solé J. Abnormal modulation of electrodermal activity by thermoalgesic stimuli in patients with primary palmar hyperhidrosis. J. Neurol. Neurosurg. Psychiatr. 82(1), 92–96 (2011).
- Castillo-Hernández CD, Casanova-Mollá J, Verger J, Valls-Solé J. Cold sensation after experiencing heat pain. Differences between healthy subjects and patients with peripheral sensory neuropathy. 20th meeting of the European Neurological Society. Berlin, 19–23 June 2010.
- Viana F, de la Peña E, Belmonte C. Specificity of cold thermotransduction is determined by differential ionic channel expression. Nat. Neurosci. 5(3), 254–260 (2002).
- Geha PY, Apkarian AV. Brain imaging findings in neuropathic pain. Curr. Pain Headache Rep. 9(3), 184–188 (2005).
- Peyron R, Faillenot I, Mertens P, Laurent B, Garcia-Larrea L. Motor cortex stimulation in neuropathic pain. Correlations between analgesic effect and hemodynamic changes in the brain. A PET study. Neuroimage 34(1), 310–321 (2007).
- Witting N, Kupers RC, Svensson P, Jensen TS. A PET activation study of brush-evoked allodynia in patients with nerve injury pain. Pain 120(1–2), 145–154 (2006).
- Apkarian AV, Bushnell MC, Treede RD, Zubieta JK. Human brain mechanisms of pain perception and regulation in health and disease. Eur. J. Pain 9(4), 463–484 (2005).
- Mouraux A, Diukova A, Lee MC, Wise RG, Iannetti GD. A multisensory investigation of the functional significance of the “pain matrix”. Neuroimage 54(3), 2237–2249 (2011).
- Moisset X, Bouhassira D. Brain imaging of neuropathic pain. Neuroimage 37(Suppl. 1), S80–S88 (2007).
- Obermann M, Pleger B, de Greiff A et al. Temporal summation of trigeminal pain in human anterior cingulate cortex. Neuroimage 46(1), 193–200 (2009).
- Hatem SM, Attal N, Ducreux D et al. Clinical, functional and structural determinants of central pain in syringomyelia. Brain 133(11), 3409–3422 (2010).
- Cauda F, Sacco K, Duca S et al. Altered resting state in diabetic neuropathic pain. PLoS ONE 4(2), e4542 (2009).
- Iannetti GD, Niazy RK, Wise RG et al. Simultaneous recording of laser-evoked brain potentials and continuous, high-field functional magnetic resonance imaging in humans. Neuroimage 28(3), 708–719 (2005).
- Roberts K, Papadaki A, Gonçalves C et al. Contact heat evoked potentials using simultaneous eeg and fmri and their correlation with evoked pain. BMC Anesthesiol. 8, 8 (2008).
Neurophysiological assessment of painful neuropathies
To obtain credit, you should first read the journal article. After reading the article, you should be able to answer the following, related, multiple-choice questions. To complete the questions (with a minimum 70% passing score) and earn continuing medical education (CME) credit, please go to www.medscape.org/journal/expertimmunology. Credit cannot be obtained for tests completed on paper, although you may use the worksheet below to keep a record of your answers. You must be a registered user on Medscape.org. If you are not registered on Medscape.org, please click on the New Users: Free Registration link on the left hand side of the website to register. Only one answer is correct for each question. Once you successfully answer all post-test questions you will be able to view and/or print your certificate. For questions regarding the content of this activity, contact the accredited provider, [email protected]. For technical assistance, contact [email protected]. American Medical Association's Physician's Recognition Award (AMA PRA) credits are accepted in the US as evidence of participation in CME activities. For further information on this award, please refer to http://www.ama-assn.org/ama/pub/category/2922.html. The AMA has determined that physicians not licensed in the US who participate in this CME activity are eligible for AMA PRA Category 1 Credits™. Through agreements that the AMA has made with agencies in some countries, AMA PRA credit may be acceptable as evidence of participation in CME activities. If you are not licensed in the US, please complete the questions online, print the AMA PRA CME credit certificate and present it to your national medical association for review.
Activity Evaluation: Where 1 is strongly disagree and 5 is strongly agree
1. Based on the review by Drs. Barraza-Sandoval, Casanova-Mollá and Valls-Solé, which of the following statements about common causes and associated features of neuropathies associated with pain is most likely correct?
□ A Nociceptive inputs reach the central nervous system mostly via large myelinated fibers
□ B Distal axonal polyneuropathies include diabetic, alcoholic, toxic, immune, and paraneoplastic neuropathies
□ C The most common causes of neuropathic pain are proximal demyelinating neuropathies
□ D Autonomic nervous system dysfunction may suggest large fiber neuropathy
2. Your patient is a 67-year-old male with diabetes and painful neuropathy. Based on the review by Drs. Barraza-Sandoval, Casanova-Mollá and Valls-Solé, which of the following statements about use of neurophysiological techniques in the characterization of painful neuropathies is most likely correct?
□ A Conventional nerve conduction studies and electromyography are nearly always abnormal and offer definitive diagnosis of painful neuropathies
□ B Characterization of painful neuropathies is best done by assessing the feeling of pain itself
□ C Nociceptive evoked potentials, microneurography, and autonomic nervous system functional tests provide more specific information
□ D Cold stimuli are most frequently used to induce inputs in the nociceptive pathway
3. Based on the review by Drs. Barraza-Sandoval, Casanova-Mollá and Valls-Solé, which of the following statements about use of other testing modalities for the patient described in question 1 would most likely be correct?
□ A Psychophysical testing is useful only for evaluating large fiber neuropathies
□ B Psychophysical testing quantifies autonomic response
□ C Functional MRI of the brain has no place in assessing painful neuropathies
□ D Combined use of neurophysiological techniques, psychophysical tests, and fMRI may help to elucidate pain processing and eventually to find the substrate for pain sensation