Abstract
The introduction of routine infant vaccination against pneumococcal disease has resulted in a decreased overall invasive pneumococcal disease incidence in adults but also a change in invasive pneumococcal disease serotypes. This study aimed to assess the cost–effectiveness of 23-valent pneumococcal polysaccharide vaccine (PPV23) in Germany in this context. A population-based Markov model was developed. A cohort of adults currently eligible for vaccination was followed until death. Adult vaccination with PPV23 was associated with an incremental cost–effectiveness ratio of €17,065/quality-adjusted life years gained from the third-party payer’s perspective. Univariate sensitivity analyses showed that the incremental cost–effectiveness ratio was below €50,000/quality-adjusted life years gained in most test scenarios. The model suggests that adult PPV23 vaccination is cost effective in Germany, due to its broad serotype coverage. This is despite epidemiological changes in Streptococcus pneumoniae serotypes caused by wider use of pneumococcal conjugate vaccines during childhood.
IPD: Invasive pneumococcal disease; PCV: Pneumococcal conjugate vaccine; PPV: Pneumococcal polysaccharide vaccine.
Data taken from Citation[11,25].
![Figure 2. Observed and modeled seroepidemiological changes in the incidence of pneumococcal serotypes causing invasive pneumococcal disease over time from 2005 to 2021 in Germany.IPD: Invasive pneumococcal disease; PCV: Pneumococcal conjugate vaccine; PPV: Pneumococcal polysaccharide vaccine.Data taken from Citation[11,25].](/cms/asset/3c59fe36-3cd8-45d8-af52-290f484558ab/ierp_a_11215585_f0002_b.jpg)
Attribution factor refers to the proportion of nonbacteremic pneumonia having pneumococcal origin.
†The maximum value obtained in the sensitivity analysis was €118,382 per quality-adjusted life-year gained, when vaccine effectiveness of 23-valent pneumococcal polysaccharide vaccine against NBPP was assumed to be 0%. The incremental cost–effectiveness ratio was €75,664, €41,789 and €22,848 per quality-adjusted life-year gained, when vaccine effectiveness of 23-valent pneumococcal polysaccharide vaccine against NBPP was assumed to be 5, 15 and 30% in the elderly and at-risk immunocompetent adults, respectively.
HR: Hazard ratio; IPD: Invasive pneumococcal disease; i.s.: Immunosuppressed; NBPP: Nonbacteremic pneumococcal pneumonia; PCV: Pneumococcal conjugate vaccine; VE: Vaccine effectiveness.
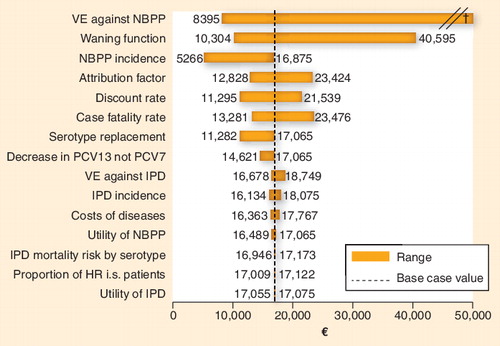
Pneumococcal diseases
Streptococcus pneumoniae bacteria are lancet-shaped, Gram-positive, facultative anaerobic organisms. They typically present in pairs (diplococci) but may also occur singularly or in short chains. Some pneumococci are encapsulated, their surfaces composed of complex polysaccharides and it is these organisms that are pathogenic for humans, whereas organisms without capsular polysaccharides are nonpathogenic. Indeed, the polysaccharide capsule acts as a virulence factor for the bacterium and it is antigenic, forming the basis for classifying pneumococci by serotypes. More than 90 different serotypes have been identified and these differ by virulence, prevalence and extent of drug resistance Citation[101]. S. pneumoniae can lead to noninvasive pneumonia (i.e., nonbacteremic pneumonia, which accounts for approximately 80% of all pneumococcal pneumonia) as well as invasive pneumonia (i.e., bacteremic pneumonia, which accounts for 80–90% of all invasive disease) and other invasive diseases (such as meningitis and bacteremia Citation[1–3]), and it is estimated to cause 1.6 million deaths annually worldwide Citation[4]. Children, the elderly and people with predisposing factors, including immunodeficiency and chronic diseases (cardiovascular, pulmonary, renal and hepatic) are particularly vulnerable to invasive pneumococcal disease (IPD) Citation[1–5]. It has been reported that in Germany alone, IPD accounts for approximately 1200 deaths each year Citation[6,102].
Vaccination
Vaccination remains the only public health strategy that can reduce the burden of IPD Citation[2]. Two types of vaccines are currently available in Germany.
Pneumococcal conjugate vaccines
A seven-valent pneumococcal conjugate vaccine (PCV7) obtained European market authorization for use in children aged between 2 months and 5 years in 2001. This was later replaced by a 13-valent vaccine (PCV13) in 2009. The latter was indicated for children aged between 6 weeks and 5 years Citation[103,104]. A ten-valent vaccine (PCV10) was licensed for children aged between 6 weeks and 2 years in 2009 and it was extended up to 5 years in 2011 Citation[105]. The efficacy and safety of PCV7 have been demonstrated in clinical trials in the pediatric population Citation[7,8] and evidence also supports the immunogenicity and tolerability of PCV10 and PCV13 in children Citation[9,106]. The efficacy of PCV vaccines has not yet been demonstrated in adults. In Germany, PCV vaccination in children under the age of 24 months has been recommended since 2006 Citation[10]. Before the introduction of the vaccines, serotypes associated with PCV7 accounted for 57.7% of cases of IPD in children (2001/2002), whereas the additional six serotypes included in PCV13 covered a further 24.9% of IPD cases Citation[11]. After the introduction of PCV vaccines (2009/2010), the serotypes included in PCV7 covered only 16.5% of all IPD cases in children and the six additional serotypes in PCV13 covered a further 54.7% cases Citation[11]. In Germany, the indication of PCV13 has recently been extended to include adults aged 50 years and older to prevent IPD Citation[107].
At the present time, comparisons between PCV vaccines and pneumococcal polysaccharide vaccines (PPVs) are limited to immunogenicity studies Citation[12–21] and no link between immune response and clinical effectiveness has been established Citation[22].
Pneumococcal polysaccharide vaccine
A 23-valent vaccine (PPV23) is indicated for children in at-risk populations from the age of 2 years. It covers 80–90% of serotypes of IPD in Europe Citation[23–25]. Recent German data (2009/2010) from the National Reference Center for Streptococci show that it covers 82.3% of serotypes causing IPD Citation[11] and 86.5% of serotypes causing bacteremic pneumonia in adults Citation[26]. The vaccine was introduced in 1982 in Germany. In the same year, it was recommended for use in at-risk adults Citation[27], and the recommendation to vaccinate older people, aged 60 years or more, was issued in 1998 Citation[28]. Efficacy and effectiveness of PPV23 have been demonstrated against IPD in immunocompetent adults, as well as in the immunosuppressed population (e.g., in patients infected with HIV Citation[4,29,30]). Primary vaccination and revaccination after 5 years with PPV23 is generally well tolerated Citation[4,22,31,32] and provides a sustainable immune response Citation[33]. Over the past 10 years, many studies have shown that PPV23 vaccination of at-risk adults and the elderly is a cost-effective strategy in Germany, as well as in other European and American countries Citation[22,Citation34–42].
The impact of vaccinating children
The introduction of universal PCV7 vaccination in children in industrialized countries has dramatically changed the epidemiology of IPD. Reduction in the incidence of IPD associated with PCV7 serotypes in vaccinated children was observed in Europe and the USA Citation[43,108]. As a result, the heaviest burden of IPD is now borne by the elderly and at-risk adults in these countries Citation[43,108].
Following routine childhood vaccination, the reduction of IPD incidence and its effects on herd protection, has been witnessed not only in unvaccinated children but also in unvaccinated adults (e.g., in the USA, the UK and Spain Citation[44–46]). This has been explained as a consequence of decreased nasopharyngeal carriage of PCV7 serotypes Citation[22]. However, concerns have been raised with regard to the increased incidence of IPD associated with non-PCV serotypes, known as a serotype replacement effect, which has been reported in several countries Citation[46–49]. Such changes could offset some of the benefits of vaccination as a result of reduction in herd protection Citation[50].
The recent switch from PCV7 to PCV13 in children is expected to accentuate the changes in the epidemiology of pneumococcal diseases in both children and adults, as the incidence of IPD associated with the additional six serotypes in PCV13 is expected to decrease in both children and adults while the incidence associated with serotypes not covered by the additional six serotypes is expected to increase. The increase in the incidence of IPD associated with non-PCV13 serotypes may be less than that associated with non-PCV7 serotypes observed following the introduction of PCV7 in children, since the additional six serotypes covered by PCV13 accounted for less than half of IPD cases caused by PCV7 serotypes in the period before the introduction of PCV vaccination Citation[11]. Nevertheless, the cost–effectiveness profile of PPV23 vaccination in adults against the pneumococcal diseases may be impacted.
Objectives
This study aimed to assess the cost–effectiveness of PPV23 in the elderly and at-risk adults in Germany, and it takes into account the decreased incidence of IPD induced by universal vaccination of children with PCV7/13.
Modeling approach
Target population & model structure
A population-based Markov model was developed to analyze the cost–effectiveness of PPV23 vaccination compared with no vaccination as no alternative to PPV23 existed with clinical effectiveness data at the time of this study. The model accounted for future changes in the epidemiology of IPD in adults induced by the universal PCV vaccination of children. The corresponding approach is detailed in the following section. The model tracked a cohort of adults (aged 18 years and older) until death or 100 years of age.
The target population consisted of German adults eligible for PPV23 vaccination and who would receive the vaccination in 2011. It consisted of individuals younger than 60 years of age who were at higher risk of developing IPD diseases (‘at-risk adults’) and individuals aged 60 years or older (‘the elderly’). A further distinction was made in the at-risk population such that the population was split between immunocompetent: those with chronic respiratory, cardiovascular conditions and/or diabetes; and immunosuppressed: those who were infected with HIV, had received immunosuppressant therapies (e.g., chemotherapy or high-dose corticosteroids) or had received a transplantation.
Five health states were considered in the Markov structure: no pneumococcal disease, nonbacteremic pneumococcal pneumonia (NBPP), IPD (including pneumococcal meningitis and bacteremic pneumococcal pneumonia), postmeningitis sequelae (PMS) and death . Individuals entered the model in the no pneumococcal disease state, and they risked developing IPD or NBPP. Patients with IPD or NBPP faced a higher risk of death than individuals without pneumococcal disease. Following IPD and/or NBPP, patients could recover and return to the no pneumococcal disease health state, and a proportion of patients with pneumococcal meningitis could develop PMS. It was assumed that following PMS, patients do not develop another episode of IPD or NBPP, a situation estimated as rare (less than one in a million Citation[51]).
The cycle length of the model was 1 year so that the seasonality of pneumococcal diseases did not have to be reflected in the model Citation[52]. The model was developed in compliance with guidelines published by the German health technology assessment body Institut für Qualität und Wirtschaftlichkeit im Gesundheitswesen Citation[109] and the principles of good practice for modeling by the International Society for Pharmacoeconomics and Outcomes Research Citation[53].
Given the lack of evidence of indirect protection against pneumococcal diseases obtained through the vaccination of adults with PPV23, such an effect was not included in the model. This approach was conservative as it underestimates the effect of the vaccine (by ignoring the potential herd protection from vaccinating adults).
Natural history of IPD & future epidemiology
The change in the incidence of IPD in adults induced by the vaccination of children (decreased incidence of vaccine serotypes and serotype replacement) was modeled through time-dependent probabilities of developing IPD. Due to a lack of data, a stable incidence of IPD was assumed between 2003 and 2005. This was based on findings from a prospective laboratory-based surveillance study conducted in the largest federal state, North-Rhine Westphalia, in Germany between 2001 and 2003 Citation[51].
The recommendation of PCV7 in Germany since 2006 led to a change in the epidemiology of disease Citation[11,25]. As the German data were not sufficient to simulate epidemiological trends, the changes were estimated based on US data. In the USA, it has been observed that increased PCV7 vaccine uptake in children is associated with a decreased incidence of IPD in adults caused by PCV7 serotypes Citation[9] while the incidence of IPD associated with other serotypes has increased Citation[44,110].
Based on US data observed during the 7 years following introduction of the PCV7 vaccine in children, the change in the incidence of IPD associated with PCV7 in adults was found to be related to cumulative vaccine uptake in children Citation[11]. A parametric function estimating the change in IPD incidence as a function of cumulative vaccine coverage rate in children was therefore estimated using the US data. A cumulative gamma distribution was selected for its goodness of fit. The cumulative coverage rates observed in German children were then used in order to estimate the change in IPD incidence from 2005. A stable incidence was assumed beyond 2012 (7 years after introduction of the vaccine in children) due to a lack of longer term data. A similar approach, based on the same US data, was selected to predict the expected increase in the incidence of IPD cases not associated with serotypes included in the vaccine used in children.
The model considered the effect of introducing universal PCV13 vaccination in children, which protects against six additional serotypes than PCV7. Due to a lack of data, it was assumed that the association between the corresponding incidence of IPD in adults and cumulative coverage of the additional six serotypes in children followed the same pattern as the PCV7 serotypes.
In at-risk adults, the incidence of IPD was adjusted for risk group using the following sources. The relative risks of developing IPD by risk group were based on data observed in the USA Citation[54], and the proportion of patients in each risk group was derived from German sources Citation[55]. The incidence of IPD by serotypes for this period was estimated according to the nationwide surveillance from Germany Citation[25]. The proportion of IPD cases by serotype was compared with German data reported by the National Reference Center for Streptococci between 2004 and 2009 as part of the model validation Citation[11,25]. Due to a lack of German data, the IPD case–fatality rate was estimated from a retrospective surveillance study covering approximately 25% of the Dutch population, which was conducted between 2004 and 2006 Citation[56]. As the case–fatality rate varies across serotypes, mortality by serotype was estimated based on a Danish nationwide population-based study, which followed 18,858 IPD patients between 1977 and 2007 Citation[57].
Natural history of IPD complications & other pneumococcal diseases
Few studies reporting the incidence of NBPP in Germany were identified. Ewig et al. reported the incidence of community-acquired pneumonia requiring hospitalization by age group based on the German program for quality in healthcare in 2005–2006 Citation[58]. It was estimated that approximately 40% of community-acquired pneumonia cases were attributable to pneumococcal infection, based on the German Competence Network for Community-Acquired Pneumonia study Citation[59]. Case–fatality rates also came from the Competence Network for Community-Acquired Pneumonia study Citation[59]. Due to a lack of data, a similar incidence of NBPP was assumed across risk groups.
Following an episode of pneumococcal meningitis, patients may develop PMS, consisting of hearing loss and other neurological sequelae Citation[60,61]. As noted in a review by des Portes (2009 Citation[62]), 30% of hospitalized adult patients develop moderate to severe neurological sequelae, which lead to an inability to work. Furthermore, 22% of surviving adult patients experience hearing loss. The prevalence rates were applied in the model to estimate the number of patients with PMS. No excess mortality was assumed for the PMS health state, due to a lack of data.
Demography
The size of the German population by age in 2011 was obtained from the 12th Coordinated Population Projection for Germany (12. Bevölkerungsvorausberechnungfür Deutschland) from DESTATIS (Statistisches Bundesamt Deutschland) Citation[111]. Linear interpolation between the two available data points were used where needed Citation[Emmerling D, Pers. Comm.]. The split by risk for the influenza vaccination in the German population aged between 5 and 49 years was applied to all age groups in the model due to the lack of data in the population aged 50 years and above (at-risk immunocompetent [with chronic cardiovascular or respiratory disease or diabetes] was 12.8%; and at-risk immunosuppressed [with HIV/AIDS or transplantations] was 0.2% Citation[55]).
It was estimated that 3% of the eligible German population would receive PPV23 vaccination in 2011, leading to a target population size of 833,890 individuals. This was based on sales data for PPV23 Citation[11], due to a lack of publicly available information on the vaccination coverage rate in Germany.
Vaccine effectiveness & revaccination
Vaccine effectiveness differs between the three risk groups (Table 2). In immunocompetent individuals, vaccine effectiveness against IPD was obtained from a recent Cochrane systematic literature review and meta-analysis of ten prospective clinical trials Citation[29], whereas for at-risk immunosuppressed individuals, vaccine effectiveness against IPD was retrieved from a prospective trial conducted on HIV-infected patients in the USA Citation[30].
Vaccine effectiveness against NBPP was taken from a prospective cohort study conducted between 2002 and 2005 in Spain of 11,241 individuals aged 65 years and older Citation[63]. It was assumed that the vaccine did not protect the immunosuppressed patients against NBPP.
In the model, the same vaccine effectiveness was applied to all age groups due to the lack of data. It was assumed that vaccinated individuals were protected from the time of vaccination and were no longer protected after 8 years from the initial vaccination Citation[64,65]. As per German recommendations, the model assumed that only people younger than 60 years at initial vaccination would receive a second dose of PPV23 after 5 years of the initial vaccination Citation[66]. Based on the results from several observational studies, revaccination was assumed to provide the same vaccine effectiveness as the initial vaccination Citation[31,67,68]. A total of 3% of vaccinated people were expected to receive revaccination, according to PPV23 sales data Citation[11].
Resource utilization & costs
The resource utilization and cost analysis took the perspective of the German third-party payer (TPP, i.e., sickness funds), as well as a societal perspective in order to account for the inability of patients with PMS to work (Table 3). All costs were estimated in 2010 Euros and the Harmonized Indices of Consumer Prices inflator for healthcare services was used when necessary Citation[112]. Costs were discounted at 3%, following German guidelines on economic evaluations Citation[109].
The vaccine’s unit price (Pneumovax® 23, single dose pack; Sanofi Pasteur MSD) was taken from the LAUER-Taxe (as of 1 January 2011 Citation[113]). The treatment of pneumococcal diseases and vaccine administration costs were obtained from German sources Citation[11,114] and a German cost–effectiveness analysis conducted by Claes et al. Citation[69]. For the treatment of hearing loss due to pneumococcal meningitis, the annual costs were estimated from a German cost–effectiveness analysis of cochlear implants in children Citation[70]. The treatment costs of other neurological sequelae were estimated based on the ratio between the costs of treating hearing loss and other neurological sequelae in the study by Claes et al. Citation[69]Citation. A copayment of €7 was applied to treatment and vaccine administration costs based on the assumption that 30% of the patients were fully exempted from a copayment of €10 Citation[115,116].
The numbers of days off work in patients with IPD and NBPP were taken from the German Federal Health Monitoring (Gesundheitsberichterstattung des Bundes Citation[117]). In 2009, the average length of stay was 13.5 days in patients with pneumonia due to S. pneumoniae (ICD10: J13) aged 15 years old and above and the figure was applied to both IPD and NBPP cases. Patients with postmeningitis sequelae were assumed to cease employment completely Citation[62]. The cost of a day off was calculated from the average compensation of employees (Arbeitnehmerentgelt) in Germany Citation[118]. It was assumed that the productivity loss applied until the legal retirement age of 65 years, as the higher retirement age (67 years) is only expected to be fully implemented within two decades Citation[119].
Effectiveness
Effectiveness was measured in quality-adjusted life years (QALYs) in the model (Table 4). QALYs were discounted at 3% Citation[109]. The baseline QALY weights were obtained from a US cost–effectiveness study which reported utility values by risk group based on data from the 1990 National Health Interview Survey Citation[41]. The utility associated with the IPD health state was from the same study, which has been used in several other cost–effectiveness analyses Citation[34,71]. Due to the scarcity of data on utility in patients with NBPP, a conservative approach was used and no utility decrement was assumed for NBPP. For PMS, the utility multipliers used were from a Canadian cost–effectiveness study Citation[72].
Economic analyses
The economic analyses compared PPV23 vaccination with no vaccination. The German health technology assessment authorities do not set a fixed threshold of incremental cost–effectiveness ratio (ICER) below which a health technology is considered as ‘good value for money’ Citation[109]. In this study, an arbitrary threshold of €50,000 per QALY gained was considered as the primary threshold of cost–effectiveness, as it was commonly used in the German context Citation[73–75]. A secondary threshold of €30,000 per QALY was also considered in the analysis.
Univariate sensitivity analyses were performed to examine the impact of individual parameters on the ICER. Selected parameters in the sensitivity analyses and corresponding variability were estimated from the sources or assumed, as reported in .
A probabilistic sensitivity analysis (PSA) was also conducted to quantify the uncertainty in the ICER as a result of the uncertainty in the different model parameters. The distribution associated with each model parameter was selected based on the German guidelines on economics evaluations Citation[76] and the published literature . To account for uncertainty around the epidemiological changes in IPD incidence as a result of vaccinating children, the presence of epidemiological change was also drawn randomly when running the PSA, with a chance of 50%. The PSA was run for 1000 iterations and the cost–effectiveness acceptability curve was plotted.
Results
Model validation
The modeled epidemiological changes were compared with data observed in Germany Citation[11,25]. As can be seen in , overall, the model predictions matched the observed incidence data. However, the model underestimated the contribution of PCV13 serotypes on the incidence of IPD and overestimated the contribution of non-PCV13 serotypes. The modeled trend appears to better fit the observed data in more recent years, which correspond to the study period for the cost–effectiveness analysis. The significantly decreased incidence predicted by the model between 2009 and 2010 is explained by the switch from PCV7 to PCV13 in children when the coverage rate was already high, and the corresponding assumptions of the effect of PCV13 on the epidemiology of disease led to a switch from an increase to a decrease in IPD associated with non-PCV13 serotypes.
Base-case analysis
A cohort of 833,890 German individuals eligible to receive PPV23 vaccination was followed by the model until death or the age of 100 years. A total of 22% of them (182,358) were at-risk adults aged below 60 years at the time of initial vaccination. The detailed results are reported in . When compared with no vaccination, the ICER of PPV23 vaccination was estimated at €17,065 per QALY gained from the TPP’s perspective, and at €25,687 from the societal perspective.
Sensitivity analyses
shows the variation of the ICER according to each parameter (univariate sensitivity analysis). The ICER was sensitive to the vaccine effectiveness against NBPP, waning function and incidence of NBPP. Except when assuming no vaccine effectiveness against NBPP, the ICER fell below the predefined ‘good value for money’ threshold of €50,000 per QALY gained. A threshold analysis found that a minimum of vaccine effectiveness of 11.6% against NBPP, which is much lower than the assumption in the base-case analysis (39.0%), would be sufficient to achieve cost–effectiveness at a threshold of €50,000 per QALY gained.
When assuming no change in the age-specific incidence of IPD, the ICER was estimated to be 33.9% lower than in the base-case (€11,282 per QALY gained). As no change in epidemiology would correspond to a higher incidence rate in the unvaccinated population, more IPD cases could be prevented. When assuming no change in the number of IPD cases associated with PCV13 not PCV7 serotypes, the ICER decreased to €14,621 per QALY gained (85.7% of the base-case ICER).
report the results of the PSA. Each data point represents the incremental costs and effects obtained from each model simulation following the draw of model parameters from a predefined distribution reflecting the associated uncertainty. The variation of cost–effectiveness of PPV23 was limited. When compared with no vaccination, PPV23 was cost effective in 86.7% of the cases when the willing-to-pay was set at €30,000 per QALY gained, while the rate increased to 98.1% when the willing-to-pay was at €50,000 per QALY gained, both from a TPP perspective.
Discussion
Following a hypothetical cohort of adults eligible for pneumococcal vaccination, PPV23 was estimated to reduce the incidence of IPD by 13.9% and the incidence of NBPP by 6.7% under a lifetime horizon . These relatively low changes may be explained by two main factors: low annual vaccination coverage rates and assumptions about the mean duration of protection conferred by the vaccine combined with a very low revaccination rate.
From the TPP perspective, it was estimated that 32.6% of the cost of vaccination including administration would be offset. From a societal perspective, cost offsets were relatively smaller (27.9%), as a result of a higher copayment on the vaccine (€8.2; as incremental costs) than for managing pneumococcal diseases (€7 per disease episode; as cost offset associated with reduced number of pneumococcal disease cases due to vaccination).
Despite epidemiological changes, PPV23 vaccination was still found to be cost-effective with an ICER well below €50,000 per QALY gained. Sensitivity analyses showed that the results were robust to the different model parameters. The ICER exceeded €50,000 only when the vaccine effectiveness against NBPP was assumed to be lower than 11.6%, which is much lower than the effectiveness reported in observational studies (39.0–63.0% Citation[63,Citation77,78]) and assumed in the base-case analysis (39.0%). This is due to the fact that although IPD is much more severe than NBPP, the absolute number of cases of noninvasive diseases, including NBPP, is much higher.
Strengths of the study
There are several strengths of the model. First, the model takes into account the changing epidemiology of IPD associated with the introduction of routine PCV vaccination of children. This approach better reflects the future environment in which the health technology will be used and assessed. A range of sensitivity analyses also allowed a better understanding of the impact of uncertainty around these assumptions.
Second, the model is based on local burden of disease and epidemiological data. The vaccine uptake of PCV7/13 in children and PPV23 was based on published sales data observed in Germany Citation[11]. Furthermore, the results were compared with the real epidemiology of IPD reported by the German National Centre for Streptococci Citation[11,25].
Limitations
This study has several limitations. Although the study used the German incidence and serotype coverage of IPD for the period before universal PCV vaccination in children, the epidemiological trend was based on data from the USA, another industrialized country. The overall validity of the approach has been assessed by comparing model predictions to observed data, but exact trends differ . Sensitivity analyses were conducted to assess the impact of assumptions about epidemiological trends on the ICER.
The model was not designed to capture the herd protection induced by PPV23 vaccination (i.e., indirect protection of unvaccinated individuals), which consists of future reduction of pneumococcal diseases in both the vaccinated and unvaccinated. The reason for doing so was to avoid incorporating more uncertainty stemming from assumptions on the transmission of the disease, which are not well established.
Third, the effectiveness of PPV23 in the analysis was based on a meta-analysis of clinical trials in all individuals Citation[29]. Some evidence suggests that the effectiveness might be lower in the elderly or immunocompetent people Citation[4]. Therefore, the analysis might have biased down the ICER of PPV23 when compared with no vaccination.
Finally, it has been identified that NBPP is an important driver of cost–effectiveness and additional data are required to obtain a more reliable estimate of the incidence of NBPP.
Comparison with previously published cost–effectiveness analyses
In 2009, Ogilvie et al. conducted a systematic literature review to document the cost–effectiveness analysis of PPV23 vaccination Citation[39]. Five studies Citation[34–36,41,79] were identified reporting costs per QALY gained when comparing PPV23 vaccination with no vaccination in elderly adults (50 or 65 above). In total, 18 of the 19 collected ICERs were below the threshold of US$50,000 per QALY gained (ICERs were expressed in 2007 USD) and the median ICER was about US$25,000 per QALY gained. The only study that included an analysis in the German context Citation[35] reported an ICER of approximately US$26,000. This study focused on individuals aged 65 years old or older and only IPD was accounted for.
When converted to US dollars, the base-case ICER in the authors’ study ranges from approximately US$15,000 to US$20,000 depending on the perspective and the scenario, which is in line with the literature. Further comparison is not possible because of different model structures and parameters.
Expert commentary & five-year view
The current study confirms the cost–effectiveness of PPV23 vaccination in an environment where the epidemiology of pneumococcal disease is changing. However, our estimate relies on trends observed in the USA and the applicability of these data to the German settings will need to be confirmed. This would require surveillance studies to explore the impact of universal PCV vaccination in children on adults.
Furthermore, with the recent extension of indication of PCV13 to adults aged 50 years and older to prevent IPD in Europe Citation[107], the comparative cost–effectiveness between PPV23 and PCV13 should be assessed using a similar approach. It is crucial to understand the extrinsic value of both vaccines given the changing epidemiology, before an informed decision can be made.
More broadly speaking, the epidemiological changes of IPD are not directly associated with the studied health technology, but arose as externalities (i.e., benefits to unvaccinated adults) of an earlier public health decision (i.e., universal vaccination of children). Future cost–effectiveness analyses should incorporate externalities whenever they are identifiable, together with associated uncertainties, to better reflect the expected benefit of a health technology in any given changing environment.
Conclusion
The recent introduction of universal PCV vaccination in children has led to a change in the epidemiology of IPD in adults. The incidence of IPD associated with PCV serotypes is expected to decrease and that associated with other serotypes is expected to increase in adults. Despite a reduction in the incidence of IPD, vaccinating the elderly and at-risk adults with PPV23 in Germany against IPD and NBPP remains a cost-effective strategy because of its broad serotype coverage.
Table 1. Clinical parameters.
Table 2. Vaccine effectiveness.
Table 3. Costs and resource use.
Table 4. Health-related quality of life.
Table 5. Results: number of disease cases.
Table 6. Results: costs from third-party payer perspective (in €1000).
Table 7. Results: cost from societal perspective (in €1000).
Table 8. Results: quality-adjusted life years (in 1000).
Table 9. Results: incremental cost–effectiveness ratio.
Key issues
• Infection with Streptococcus pneumoniae may lead to invasive pneumococcal disease (IPD) or noninvasive forms of pneumococcal diseases. In Germany, IPD alone accounts for approximately 1200 deaths each year.
• The introduction of universal pneumococcal conjugate vaccine (PCV) vaccination in children has led to a change in the epidemiology of IPD in vaccinated children, as well as in unvaccinated adults. It has been observed that in the USA, the incidence of IPD associated with PCV serotypes decreased and the incidence of IPD not associated with PCV serotypes increased in adults, with increasing cumulative PCV coverage in children.
• Even with the epidemiological changes, vaccinating the elderly and at-risk adults with 23-valent pneumococcal polysaccharide vaccine (PPV23) was still found to be cost effective in Germany, when compared with no vaccination, because of its broad serotype coverage.
• When assessing the cost–effectiveness of PPV23, it is crucial to take into account the changing epidemiology so that its extrinsic value can be estimated with greater accuracy. With the extension of the 13-valent PCV indication against IPD to elderly adults in Europe, its cost–effectiveness against PPV23 should be examined in a similar approach that captures the epidemiological change before an informed decision can be made.
Financial & competing interests disclosure
This study was conducted by Amaris and funded by Sanofi Pasteur MSD. Y Jiang and A Gauthier are employees of Amaris. L Annemans received a consultancy fee from Sanofi Pasteur MSD for his role as an advisor in the design of the model. M van der Linden has received research grants from GlaxoSmithKline, Pfizer and Sanofi Pasteur MSD, is a member of advisory boards for GlaxoSmithKline, has received speakers’ honoraria from GlaxoSmithKline, Pfizer and Sanofi Pasteur MSD. L Nicolas-Spony and X Bresse are employees of Sanofi Pasteur MSD. The authors have no other relevant affiliations or financial involvement with any organization or entity with a financial interest in or financial conflict with the subject matter or materials discussed in the manuscript apart from those disclosed.
No writing assistance was utilized in the production of this manuscript.
References
- Lynch JP 3rd, Zhanel GG. Streptococcus pneumoniae: epidemiology, risk factors, and strategies for prevention. Semin. Respir. Crit. Care Med. 30(2), 189–209 (2009).
- Prato R, Tafuri S, Fortunato F, Martinelli D. Why it is still important that countries know the burden of pneumococcal disease. Hum. Vaccin. 6(11), 918–921 (2010).
- Fedson DS. Pneumococcal vaccination for older adults: the first 20 years. Drugs Aging 15(Suppl. 1), 21–30 (1999).
- 23-valent pneumococcal polysaccharide vaccine. WHO position paper. Wkly Epidemiol. Rec. 83(42), 373–384 (2008).
- Cartwright K. Pneumococcal disease in western Europe: burden of disease, antibiotic resistance and management. Eur. J. Pediatr. 161(4), 188–195 (2002).
- Hülsse C, Littmann M, Fiedler K, Kaltofen U, Hundt C. Epidemiologic and serologic studies of pneumococcal infections with reference to the new STIKO recommendations. Gesundheitswesen 61(8-9), 393–397 (1999).
- Pneumococcal conjugate vaccine for childhood immunization – WHO position paper. Wkly Epidemiol. Rec. 82(12), 93–104 (2007).
- Licensure of a 13-valent pneumococcal conjugate vaccine (PCV13) and recommendations for use among children – Advisory Committee on Immunization Practices (ACIP), 2010. MMWR Morb. Mortal. Wkly Rep. 59(9), 258–261 (2010).
- Nuorti JP, Whitney CG; Centers for Disease Control and Prevention (CDC). Prevention of pneumococcal disease among infants and children - use of 13-valent pneumococcal conjugate vaccine and 23-valent pneumococcal polysaccharide vaccine - recommendations of the Advisory Committee on Immunization Practices (ACIP). MMWR Recomm. Rep. 59(RR-11), 1–18 (2010).
- Mitteilung der Ständigen Impfkommission am Robert Koch-Institut: Neuerungen in den aktuellen Empfehlungen der Ständigen Impfkommission (STIKO) am RKI vom Juli 2006. Epid. Bull. 32, 271–276 (2006).
- Sanofi Pasteur MSD data on file (2011).
- Scott DA, Komjathy SF, Hu BT et al. Phase 1 trial of a 13-valent pneumococcal conjugate vaccine in healthy adults. Vaccine 25(33), 6164–6166 (2007).
- Baxendale HE, Keating SM, Johnson M, Southern J, Miller E, Goldblatt D. The early kinetics of circulating pneumococcal-specific memory B cells following pneumococcal conjugate and plain polysaccharide vaccines in the elderly. Vaccine 28(30), 4763–4770 (2010).
- Ridda I, Macintyre CR, Lindley R et al. Immunological responses to pneumococcal vaccine in frail older people. Vaccine 27(10), 1628–1636 (2009).
- Miernyk KM, Butler JC, Bulkow LR et al. Immunogenicity and reactogenicity of pneumococcal polysaccharide and conjugate vaccines in Alaska native adults 55–70 years of age. Clin. Infect. Dis. 49(2), 241–248 (2009).
- Baxendale HE, Johnson M, Keating SM et al. Circulating pneumococcal specific plasma and memory B cells in the elderly two years after pneumococcal conjugate versus polysaccharide vaccination. Vaccine 28(42), 6915–6922 (2010).
- Scott D, Ruckle J, Dar M, Baker S, Kondoh H, Lockhart S. Phase 1 trial of 13-valent pneumococcal conjugate vaccine in Japanese adults. Pediatr. Int. 50(3), 295–299 (2008).
- Dransfield MT, Nahm MH, Han MK et al. COPD Clinical Research Network. Superior immune response to protein-conjugate versus free pneumococcal polysaccharide vaccine in chronic obstructive pulmonary disease. Am. J. Respir. Crit. Care Med. 180(6), 499–505 (2009).
- Goldblatt D, Southern J, Andrews N et al. The immunogenicity of 7-valent pneumococcal conjugate vaccine versus 23-valent polysaccharide vaccine in adults aged 50-80 years. Clin. Infect. Dis. 49(9), 1318–1325 (2009).
- de Roux A, Schmöle-Thoma B, Schmöele-Thoma B et al. Comparison of pneumococcal conjugate polysaccharide and free polysaccharide vaccines in elderly adults: conjugate vaccine elicits improved antibacterial immune responses and immunological memory. Clin. Infect. Dis. 46(7), 1015–1023 (2008).
- Musher DM, Rueda AM, Nahm MH, Graviss EA, Rodriguez-Barradas MC. Initial and subsequent response to pneumococcal polysaccharide and protein-conjugate vaccines administered sequentially to adults who have recovered from pneumococcal pneumonia. J. Infect. Dis. 198(7), 1019–1027 (2008).
- Fedson DS, Nicolas-Spony L, Klemets P et al. Pneumococcal polysaccharide vaccination for adults: new perspectives for Europe. Expert Rev. Vaccines 10(8), 1143–1167 (2011).
- Varon E. Epidemiology of acute bacterial meningitis in adult patients in France. Med. Mal. Infect. 39(7-8), 432–444 (2009).
- Trotter CL, Waight P, Andrews NJ et al. Epidemiology of invasive pneumococcal disease in the pre-conjugate vaccine era: England and Wales, 1996–2006. J. Infect. 60(3), 200–208 (2010).
- Imöhl M, Reinert RR, van der Linden M. Temporal variations among invasive pneumococcal disease serotypes in children and adults in Germany (1992–2008). Int. J. Microbiol. 2010, 874189 (2010).
- van der Linden M, Imohl M. R2762 Serotype distribution among bacteraemic pneumococcal pneumonia in adults in Germany. Clin. Microbiol. Infect. 17(S4), S832 (2011).
- Bekanntmachungen des Bundesgesundheitsamtes. Bundesgesundhbl. 25(5), 170–171 (1982).
- Impfempfehlungen der Ständigen Impfkommission (STIKO) am Robert Koch-Institut/Stand: März 1998. Epid. Bull. 15, 101–114 (1998).
- Moberley SA, Holden J, Tatham DP, Andrews RM. Vaccines for preventing pneumococcal infection in adults. Cochrane Database Syst. Rev. 1, CD000422 (2008).
- Rodriguez-Barradas MC, Goulet J, Brown S et al. Impact of pneumococcal vaccination on the incidence of pneumonia by HIV infection status among patients enrolled in the Veterans Aging Cohort 5-Site Study. Clin. Infect. Dis. 46(7), 1093–1100 (2008).
- Musher DM, Manof SB, Liss C et al. Safety and antibody response, including antibody persistence for 5 years, after primary vaccination or revaccination with pneumococcal polysaccharide vaccine in middle-aged and older adults. J. Infect. Dis. 201(4), 516–524 (2010).
- Hammitt LL, Bulkow LR, Singleton RJ et al. Repeat revaccination with 23-valent pneumococcal polysaccharide vaccine among adults aged 55–74 years living in Alaska: no evidence of hyporesponsiveness. Vaccine 29(12), 2287–2295 (2011).
- Butler JC, Breiman RF, Campbell JF, Lipman HB, Broome CV, Facklam RR. Pneumococcal polysaccharide vaccine efficacy. An evaluation of current recommendations. JAMA 270(15), 1826–1831 (1993).
- Smith KJ, Zimmerman RK, Lin CJ et al. Alternative strategies for adult pneumococcal polysaccharide vaccination: a cost–effectiveness analysis. Vaccine 26(11), 1420–1431 (2008).
- Evers SM, Ament AJ, Colombo GL et al. Cost–effectiveness of pneumococcal vaccination for prevention of invasive pneumococcal disease in the elderly: an update for 10 Western European countries. Eur. J. Clin. Microbiol. Infect. Dis. 26(8), 531–540 (2007).
- Ament A, Baltussen R, Duru G et al. Cost–effectiveness of pneumococcal vaccination of older people: a study in 5 western European countries. Clin. Infect. Dis. 31(2), 444–450 (2000).
- Smith KJ, Lee BY, Nowalk MP, Raymund M, Zimmerman RK. Cost–effectiveness of dual influenza and pneumococcal vaccination in 50-year-olds. Vaccine 28(48), 7620–7625 (2010).
- Kawakami K, Ohkusa Y, Kuroki R et al. Effectiveness of pneumococcal polysaccharide vaccine against pneumonia and cost analysis for the elderly who receive seasonal influenza vaccine in Japan. Vaccine 28(43), 7063–7069 (2010).
- Ogilvie I, Khoury AE, Cui Y, Dasbach E, Grabenstein JD, Goetghebeur M. Cost–effectiveness of pneumococcal polysaccharide vaccination in adults: a systematic review of conclusions and assumptions. Vaccine 27(36), 4891–4904 (2009).
- Akin L, Kaya M, Altinel S, Durand L. Cost of pneumococcal infections and cost–effectiveness analysis of pneumococcal vaccination at risk adults and elderly in Turkey. Hum. Vaccin. 7(4), 441–450 (2011).
- Sisk JE, Whang W, Butler JC, Sneller VP, Whitney CG. Cost–effectiveness of vaccination against invasive pneumococcal disease among people 50 through 64 years of age: role of comorbid conditions and race. Ann. Intern. Med. 138(12), 960–968 (2003).
- Ament A, Fedson DS, Christie P. Pneumococcal vaccination and pneumonia: even a low level of clinical effectiveness is highly cost-effective. Clin. Infect. Dis. 33(12), 2078–2079 (2001).
- European Centre for Disease Prevention and Control. Annual Epidemiological Report on Communicable Diseases in Europe 2010. ECDC, Stockholm, Sweden 2010.
- Pilishvili T, Lexau C, Farley MM et al. Active Bacterial Core Surveillance/Emerging Infections Program Network. Sustained reductions in invasive pneumococcal disease in the era of conjugate vaccine. J. Infect. Dis. 201(1), 32–41 (2010).
- Ardanuy C, Domenech A, Rolo D et al. Molecular characterization of macrolide- and multidrug-resistant Streptococcus pyogenes isolated from adult patients in Barcelona, Spain (1993-2008). J. Antimicrob. Chemother. 65(4), 634–643 (2010).
- Miller E, Andrews NJ, Waight PA, Slack MP, George RC. Herd immunity and serotype replacement 4 years after seven-valent pneumococcal conjugate vaccination in England and Wales: an observational cohort study. Lancet Infect. Dis. 11(10), 760–768 (2011).
- Changing epidemiology of pneumococcal serotypes after introduction of conjugate vaccine: July 2010 report. Wkly Epidemiol. Rec. 85(43), 434–436 (2010).
- Hanage WP, Finkelstein JA, Huang SS et al. Evidence that pneumococcal serotype replacement in Massachusetts following conjugate vaccination is now complete. Epidemics 2(2), 80–84 (2010).
- Rose M, Zielen S. Impact of infant immunization programs with pneumococcal conjugate vaccine in Europe. Expert Rev. Vaccines 8(10), 1351–1364 (2009).
- Hanage WP. Serotype-specific problems associated with pneumococcal conjugate vaccination. Future Microbiol. 3(1), 23–30 (2008).
- Reinert RR, Haupts S, van der Linden M et al. Invasive pneumococcal disease in adults in North-Rhine Westphalia, Germany, 2001–2003. Clin. Microbiol. Infect. 11(12), 985–991 (2005).
- Dowell SF, Whitney CG, Wright C, Rose CE Jr, Schuchat A. Seasonal patterns of invasive pneumococcal disease. Emerging Infect. Dis. 9(5), 573–579 (2003).
- Weinstein MC, O’Brien B, Hornberger J et al. ISPOR Task Force on Good Research Practices–Modeling Studies. Principles of good practice for decision analytic modeling in health-care evaluation: report of the ISPOR Task Force on Good Research Practices–Modeling Studies. Value Health 6(1), 9–17 (2003).
- Kyaw MH, Rose CE Jr, Fry AM et al. Active Bacterial Core Surveillance Program of the Emerging Infections Program Network. The influence of chronic illnesses on the incidence of invasive pneumococcal disease in adults. J. Infect. Dis. 192(3), 377–386 (2005).
- Ryan J, Zoellner Y, Gradl B, Palache B, Medema J. Establishing the health and economic impact of influenza vaccination within the European Union 25 countries. Vaccine 24(47-48), 6812–6822 (2006).
- Jansen AG, Rodenburg GD, de Greeff SC et al. Invasive pneumococcal disease in the Netherlands: Syndromes, outcome and potential vaccine benefits. Vaccine 27(17), 2394–2401 (2009).
- Harboe ZB, Thomsen RW, Riis A et al. Pneumococcal serotypes and mortality following invasive pneumococcal disease: a population-based cohort study. PLoS Med. 6(5), e1000081 (2009).
- Ewig S, Birkner N, Strauss R et al. New perspectives on community-acquired pneumonia in 388 406 patients. Results from a nationwide mandatory performance measurement programme in healthcare quality. Thorax 64(12), 1062–1069 (2009).
- Welte T, Köhnlein T. Global and local epidemiology of community-acquired pneumonia: the experience of the CAPNETZ Network. Semin. Respir. Crit. Care Med. 30(2), 127–135 (2009).
- Edmond K, Clark A, Korczak VS, Sanderson C, Griffiths UK, Rudan I. Global and regional risk of disabling sequelae from bacterial meningitis: a systematic review and meta-analysis. Lancet Infect. Dis. 10(5), 317–328 (2010).
- Jit M. The risk of sequelae due to pneumococcal meningitis in high-income countries: a systematic review and meta-analysis. J. Infect. 61(2), 114–124 (2010).
- des Portes V. Long-term follow-up of bacterial meningitis - sequels in children and adults: incidence, type, and assessment issues. Med. Mal. Infect. 39(7-8), 572–580 (2009).
- Vila-Córcoles A, Ochoa-Gondar O, Hospital I et al. EVAN Study Group. Protective effects of the 23-valent pneumococcal polysaccharide vaccine in the elderly population: the EVAN-65 study. Clin. Infect. Dis. 43(7), 860–868 (2006).
- Middleton DB, Lin CJ, Smith KJ et al. Economic evaluation of standing order programs for pneumococcal vaccination of hospitalized elderly patients. Infect. Control Hosp. Epidemiol. 29(5), 385–394 (2008).
- Shapiro ED, Berg AT, Austrian R et al. The protective efficacy of polyvalent pneumococcal polysaccharide vaccine. N. Engl. J. Med. 325(21), 1453–1460 (1991).
- Mitteilung der Ständigen Impfkommission am Robert Koch-Institut. Empfehlungen der Ständigen Impfkommission (STIKO) am Robert Koch-Institut/Stand: Juli 2011. Epid. Bull. 30, 276–294 (2011).
- Singleton RJ, Hennessy TW, Bulkow LR et al. Invasive pneumococcal disease caused by nonvaccine serotypes among Alaska native children with high levels of 7-valent pneumococcal conjugate vaccine coverage. JAMA 297(16), 1784–1792 (2007).
- Manoff SB, Liss C, Caulfield MJ et al. Revaccination with a 23-valent pneumococcal polysaccharide vaccine induces elevated and persistent functional antibody responses in adults aged 65 > or = years. J. Infect. Dis. 201(4), 525–533 (2010).
- Claes C, Reinert RR, von der Schulenburg JM. Cost effectiveness analysis of heptavalent pneumococcal conjugate vaccine in Germany considering herd immunity effects. Eur. J. Health Econ. 10(1), 25–38 (2009).
- Schulze-Gattermann H, Illg A, Schoenermark M, Lenarz T, Lesinski-Schiedat A. Cost–benefit analysis of pediatric cochlear implantation: German experience. Otol. Neurotol. 23(5), 674–681 (2002).
- Rozenbaum MH, Hak E, van der Werf TS, Postma MJ. Results of a cohort model analysis of the cost–effectiveness of routine immunization with 13-valent pneumococcal conjugate vaccine of those aged > or =65 years in the Netherlands. Clin. Ther. 32(8), 1517–1532 (2010).
- De Wals P, Petit G, Erickson LJ et al. Benefits and costs of immunization of children with pneumococcal conjugate vaccine in Canada. Vaccine 21(25-26), 3757–3764 (2003).
- Scherbaum WA, Goodall G, Erny-Albrecht KM, Massi-Benedetti M, Erdmann E, Valentine WJ. Cost–effectiveness of pioglitazone in type 2 diabetes patients with a history of macrovascular disease: a German perspective. Cost Eff. Resour. Alloc. 7, 9 (2009).
- Neilson AR, Sieper J, Deeg M. Cost–effectiveness of etanercept in patients with severe ankylosing spondylitis in Germany. Rheumatology (Oxford) 49(11), 2122–2134 (2010).
- Aballéa S, Chancellor J, Martin M et al. The cost–effectiveness of influenza vaccination for people aged 50 to 64 years: an international model. Value Health 10(2), 98–116 (2007).
- Briggs A, Claxton K, Sculpher M. Decision modelling for health economic evaluation. Oxford University Press, Oxford, UK (2006).
- Vila-Corcoles A, Salsench E, Rodriguez-Blanco T et al. Clinical effectiveness of 23-valent pneumococcal polysaccharide vaccine against pneumonia in middle-aged and older adults: a matched case–control study. Vaccine 27(10), 1504–1510 (2009).
- Maruyama T, Taguchi O, Niederman MS et al. Efficacy of 23-valent pneumococcal vaccine in preventing pneumonia and improving survival in nursing home residents: double blind, randomised and placebo controlled trial. BMJ 340, c1004 (2010).
- Sisk JE, Moskowitz AJ, Whang W et al. Cost–effectiveness of vaccination against pneumococcal bacteremia among elderly people. JAMA 278(16), 1333–1339 (1997).
Websites
- Centers for Disease Control and Prevention. Epidemiology and Prevention of Vaccine-Preventable Diseases (The Pink Book). Pneumococcal disease (2011). www.cdc.gov/vaccines/pubs/pinkbook/pneumo.html (Accessed 27 March 2012)
- Reinert RR, Bauer T, Bogner JR et al. Positionspapier. Für höhere Impfraten gegen Pneumokokken-Erkrankungen (2004). Click here for link (Accessed 27 March 2012)
- European Medicines Agency. EPAR Prevenar. Click here for link (Accessed 22 March 2012)
- European Medicines Agency. EPAR Prevenar 13. www.ema.europa.eu/docs/en_GB/document_library/EPAR_-_Summary_for_the_public/human/001104/WC500057248.pdf (Accessed 22 March 2012)
- European Medicines Agency. EPAR Synflorix. www.ema.europa.eu/docs/en_GB/document_library/EPAR_-_Summary_for_the_public/human/000973/WC500054347.pdf (Accessed 22 March 2012)
- European Medicines Agency. Assessment report for Synflorix. www.ema.europa.eu/docs/en_GB/document_library/EPAR_-_Public_assessment_report/human/000973/WC500054349.pdf (Accessed 22 March 2012)
- Pfizer. Gebrauchsinformation Prevenar 13 Injektionssuspension (2011). http://static.pfizer.de/fileadmin/pfizer.de/documents/gi/Prevenar_GI.pdf (Accessed 27 March 2012)
- Centers for Disease Control and Prevention. Active Bacterial Core Surveillance Report: Emerging Infections Program Network, Streptococcus pneumoniae, 2009 (2010). www.cdc.gov/abcs/reports-findings/survreports/spneu09.pdf (Accessed 27 March 2012)
- Institut für Qualität und Wirtschaftlichkeit im Gesundheitswesen (IQWIG). General methods for the assessment of the relation of benefits to costs. www.iqwig.de/download/General_Methods_for_the_Assessment_of_the_Relation_of_Benefits_to_Costs.pdf (Accessed 22 March 2012)
- ACIP Pneumococcal Vaccines Workgroup. Use of pneumococcal polysaccharide vaccine (PPV23) in adults aged >50 years (2008). www.cdc.gov/vaccines/recs/acip/downloads/mtg-slides-jun08/03-1-pneu.pdf (Accessed 22 March 2012)
- Statistisches Bundesamt Deutschland. Bevölkerungsentwicklung in Deutschland bis 2060. Click here for link (Accessed 22 March 2012)
- Eurostat. Harmonized Indices of Consumer Prices (HCIP). http://epp.eurostat.ec.europa.eu/portal/page/portal/hicp/introduction (Accessed 27 March 2012)
- WEBAPO® LAUER-Taxe. Pricing database. www.lauer-taxe-online.de (Accessed 22 March 2012)
- Vereinbarung gemäß § 10 Abs. 9 KHEntgG für den Vereinbarungszeitraum 2010. Click here for link (Accessed 27 March 2012)
- Schreyögg J, Grabka MM. Copayments for ambulatory care in Germany: a natural experiment using a difference-in-difference approach. In: Deutsches Institut für Wirtschaftsforschung Discussion Papers (2008). www.diw.de/documents/publikationen/73/diw_01.c.81490.de/dp777.pdf (Accessed 27 March 2012)
- Kaiser Family Foundation. Cost sharing for health care: France, Germany, and Switzerland (2009). www.kff.org/insurance/upload/7852.pdf (Accessed 27 March 2012)
- Gesundheitsberichterstattung des Bundes. Das Informationssystem der Gesundheitsberichterstattung des Bundes (2011). www.gbe-bund.de/gbe10/pkg_isgbe5.prc_isgbe (Accessed 27 March 2012)
- Statistische Ämter des Bundes und der Länder. Arbeitnehmerentgelt, Bruttolöhne und -gehälter in den Ländern und Ost-West-Großraumregionen Deutschlands. Reihe 1, Band 2 (2010). http://vgrdl.de/Arbeitskreis_VGR/R0B0.asp?R1B2 (Accessed 27 March 2012)
- Organisation for Economic Co-operation and Development. Pensions at a glance 2011: retirement-income systems in OECD and G20 countries (2011). www.oecd.org/els/social/pensions/PAG (Accessed 27 March 2012)