Abstract
The aim of this review is to make researchers aware of the benefits of an efficient quality control system for prediction of a developed vaccine’s efficacy. Two major goals should be addressed when inactivating a virus for vaccine purposes: first, the infectious virus should be inactivated completely in order to be safe, and second, the viral epitopes important for the induction of protective immunity should be conserved after inactivation in order to have an antigen of high quality. Therefore, some problems associated with the virus inactivation process, such as virus aggregate formation, protein crosslinking, protein denaturation and degradation should be addressed before testing an inactivated vaccine in vivo.
Reaction with (A) DNA or RNA (adenine) and, in a similar fashion, (B) amino acids (e.g., lysine) of proteins with a primary amine group: monohydroxy methylation and methylene bridge formation.
A: Adenine; Lys: Lysine.
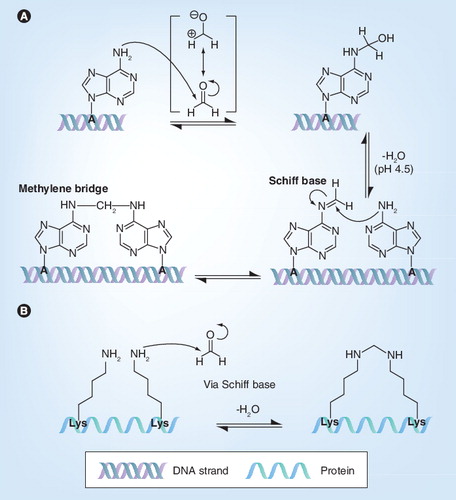
Lys: Lysine.
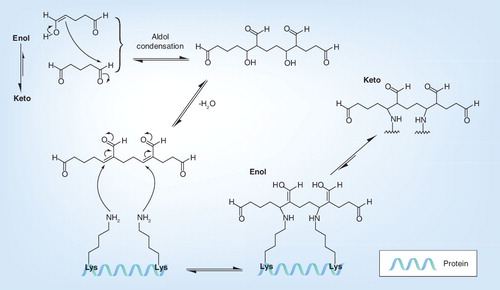
Classically, two main types of vaccines are used to protect man and animals clinically and viro-logically on challenge: modified live virus (attenuated virus) vaccines and killed virus (KV; inactivated virus) vaccines. KV vaccines are often preferred because of safety reasons, but some problems may be encountered using them. First, virus inactivation may turn out to be incomplete with outbreaks after vaccination as a consequence Citation[1–3]. Second, the viral-neutralizing epitopes may be destroyed during inactivation, resulting in a poor neutralizing antibody response and a poor protection on challenge Citation[4–6]. Therefore, efficient quality control of the inactivated antigen is necessary to evaluate virus inactivation and the effect of the inactivation procedure on neutralizing epitopes.
In the following paragraphs, different and most commonly used inactivation procedures for viruses will be discussed and their usefulness for the development of a KV vaccine will be evaluated. An overview of the discussed inactivation methods and the relevant chemical conditions involved is given in .
Cross-linkers
Formaldehyde
KV vaccine development based on inactivation with formaldehyde has been investigated for many viruses. A few of them are discussed below and presented in . Some formaldehyde-inactivated vaccines work well and protect against disease on challenge. This accounts for inactivated Ross River virus (RRV) Citation[7], West Nile virus Citation[8], dengue-2 virus Citation[9], equine herpes virus type 1 Citation[10], papilloma virus Citation[11], Hantaan virus Citation[12], influenza virus Citation[13], hepatitis A virus Citation[14], tick-borne encephalitis virus Citation[15,16] and Japanese encephalitis virus Citation[17]. However, with some vaccines inactivated in this way, problems have been encountered. In order to gain insight into the reasons for failure, a more detailed understanding of the chemistry is needed.
Formaldehyde , also known as formalin when diluted in water, has an electron-deficient central carbon atom and is therefore electrophilic, as illustrated through one of the resonance forms. As a consequence, a nucleophile, such as a nonprotonated amino group, can attack the central carbonyl carbon. This chemical reaction is called a nucleophilic addition.
Formaldehyde has an effect on both genome and proteins. First of all, it monohydroxymethylates adenine Citation[18]: the nonprotonated exocyclic amine on adenine (N6) acts as a nucleophile and reacts with the carbocation of formaldehyde Citation[19]. Because of this nucleophilic addition, adenine is monohydroxymethylated, which blocks genome reading Citation[20]. This monohydroxymethylation of adenine , also known as alkylation, can occur on either DNA or RNA Citation[21]. Monohydroxymethyladenine (NH–CH2OH) is stable for days at room temperature and is more likely to exist than the Schiff base (N=CH2) Citation[22]. However, if adenine and formaldehyde are stored for days at room temperature and at a pH of 4.5 then methylene bis-adenine can be formed Citation[19].
Formaldehyde can also react with nonprotonated amino groups of the N-terminal amino acid residue and the amino acids featuring N-containing functionality in their side chain, such as lysine, arginine, glutamine, tryptophan and histidine, or a sulfhydryl group in their side chain, such as cysteine. More specifically, as exemplary illustrated for the lysine side chain , a nonprotonated amine acts as a nucleophile and reacts with the carbocation of formaldehyde. As a result, the amino group is monohydroxymethylated, as described above for adenine. The loss of a water molecule from the unstable hemiaminal results in Schiff base formation Citation[23], also called an imine intermediate. The resulting Schiff base or imine intermediate can crosslink with arginine and tyrosine and to a lesser extent with glutamine, asparagine, trypto-phan and histidine residues by a nucleophilic addition reaction Citation[23]. In this way, inter- and intra-molecular methylene bridges can be formed Citation[24]. Because of these bridges, proteins become inter- and intra-molecularly crosslinked.
In addition, these reactions can also cause crosslinking between genome and proteins through combining the above described functionalities Citation[25,26], which prevents the transcriptional machinery from reaching the genome Citation[27].
One of the problems observed with formaldehyde is that some vaccines based on formaldehyde inactivation can contain incompletely inactivated virus particles, which can cause outbreaks of virus infections on vaccination Citation[28]. This is reported for several viruses such as foot-and-mouth disease virus (FMDV) Citation[1] and Venezuelan equine encephalitis virus (VEEV) Citation[28]. Molecular analysis proved clearly that outbreaks of FMDV in western Europe in the 1980s and VEEV in central America in 1970s are a consequence of incompletely inactivated virus vaccines Citation[28]. This can be due to crosslinking of nucleocapsid proteins with RNA. As a consequence, RNA cannot be degraded, and some virus particles escape from inactivation with formaldehyde Citation[29]. It is also possible that the safety testing was not sufficient and that a higher concentration of formaldehyde is necessary to inactivate these viruses properly. Darnell et al. examined formaldehyde inactivation of the severe acute respiratory syndrome coronavirus (SARS-CoV) Citation[30]. They showed that formaldehyde can inactivate the virus in a temperature-dependent manner. The virus could not be inactivated at a low temperature of 4°C even after 3 days. However, when using a higher temperature of 25 or 37°C, formaldehyde could inactivate most of the virus after 1 day Citation[30]. Although not investigated in this particular study, in addition to temperature, the pH during inactivation can play a major role, as is further illustrated in this review (see ‘pH’ section). When SARS-CoV is treated with 0.05% formaldehyde for 48 h, it could be inactivated properly Citation[31]. Vaccines based on formaldehyde-inactivated SARS-CoV are shown to induce virus-specific and virus-neutralizing antibodies Citation[32,33]; however, some virus still remained infectious on day 3 of inactivation Citation[30].
Some KV vaccines based on formaldehyde inactivation do not induce a virus-neutralizing antibody response and do not protect on challenge, for example respiratory syncytial virus (RSV) Citation[34], inactivated HIV Citation[5], SIV Citation[35] and VEEV vaccines Citation[6]. A poor immune response on vaccination can even lead to atypical or enhanced disease after infection of vaccinated recipients. This is most likely due to the poor induction of neutralizing antibody responses together with the formation of immune complexes between virus and antibodies, and further facilitated uptake and infection of these complexes by monocytic cells, termed antibody-dependent enhanced infection Citation[36]. This is observed with inactivated measles Citation[37] and RSV Citation[34,38] vaccines.
Formaldehyde can destroy the viral structure, which can result in a poor immune response. When poliovirus (PV) is treated with formaldehyde, the extent of the modification of the antigenic sites is dependent on the strain used and correlates with the immune response that can be induced Citation[39]. Treatment of porcine reproductive and respiratory syndrome virus (PRRSV) with formaldehyde inactivates the virus but does not preserve the viral entry-associated domains. These domains may be important for the induction of protective immunity, as neutralizing antibodies are directed to the viral epitopes that are involved in the attachment to the PRRSV receptors and internalization into the host cell Citation[40]. Since crosslinking of viral entry-associated domains might interfere with the subsequent presentation of viral-neutralizing epitopes to cells of the adaptive immune system, this procedure is not useful for inactivated PRRSV vaccine development Citation[41]. Modification of the virion structure on inactivation with formaldehyde is also reported for FMDV Citation[3], HIV Citation[42] and canine parvovirus Citation[43].
In conclusion, although formaldehyde has been shown to correctly inactivate some viruses, given the problems discussed above, care must be taken regarding the exact protocol used. In part, some failures could be due to an insufficient inactivation protocol. Problems, such as incomplete virus inactivation, that lead to outbreaks have to be kept in mind when using this inactivation method for KV vaccine development. Formaldehyde can be used for KV development for some viruses, but several KV vaccines based on formaldehyde inactivation do not protect the host on challenge with virulent virus. This could be due to RNA–protein crosslinking Citation[26,27] or modification of viral proteins by formaldehyde, as reported for PV, FMDV and HIV Citation[3,39,42].
Glutaraldehyde
Examples of virus inactivation with glutaraldehyde are summarized in . Although there are few examples, complete virus inactivation with glutaraldehyde seems to be less problematic than with formaldehyde.
Glutaraldehyde is a saturated, five-carbon dialdehyde. The carbonyl carbons are electrophilic and therefore a nucleophile, such as a nonprotonated amino group, might attack these carbons in a nucleophilic addition reaction, just like formaldehyde.
Genomic DNA or RNA is the target of glutaraldehyde. Although the exact mechanism of action has not clearly been described, it is likely that glutaraldehyde acts in the same way as formaldehyde, since similar chemical groups are present on formaldehyde and glutaraldehyde Citation[44]. The exocyclic amino group on adenine (N6) is the reactive group, which attacks glutaraldehyde Citation[45]. It is certain that glutaraldehyde can have an effect on the genome, since RNA and protein synthesis can be blocked by this aldehyde Citation[46].
Glutaraldehyde can have an effect on proteins as well, because nonprotonated amines of amino acids, such as lysine, can be cross-linked with each other Citation[47]. However, the reaction mechanism of glutaraldehyde with proteins is different from that of formaldehyde . First, glutaraldehyde forms unsaturated aldehydes by aldol condensation and elimination of water Citation[48]. Then, several amino acids can be linked to glutaraldehyde via a Michael-type addition, the nonprotonated amines acting as nucleophiles and reacting with the double bond of the glutaraldehyde oligomer Citation[49]. In this way, the amino acids are coupled by formation of a bridge Citation[50]. Unlike the reaction with formaldehyde, intermediate Schiff bases or imines are not formed Citation[44,50], but both pathways result in covalently linked amino acid side chains.
In addition, the reactions described above can also cause crosslinking of the genome and proteins Citation[25], which blocks genome reading Citation[27].
To investigate virus inactivation with glutaraldehyde, SARS-CoV was incubated with glutaraldehyde at two different dilutions and at different temperatures. The glutaraldehyde exhibited a temperature dependence in its ability to inactivate SARS-CoV. The virus could not be inactivated by glutaraldehyde at 4°C even after 3 days. However, when a higher temperature of 25 or 37°C was used, the virus could be inactivated in 2 and 1 days, respectively Citation[30].
As glutaraldehyde is a bi-functional or, after aldol condensation, multifunctional crosslinking agent, virus damage might be more extensive. Therefore, the induction of a protective immune response can be more of a problem with glutaraldehyde-inactivated virus vaccines. Duck HBV (DHBV) and bovine RSV (BRSV) are viruses for which the use of glutaraldehyde has been tested for the production of a KV vaccine Citation[4,51]. Glutaraldehyde-inactivated DHBV and BRSV developed virus-specific antibodies, but ducks vaccinated with glutaraldehyde-inactivated DHBV and lambs vaccinated with glutaraldehyde-inactivated BRSV were not fully protected against viremia following challenge. This indicates that there was no induction of protective immunity in these animals Citation[4,51].
The destruction of the viral structure by glutaraldehyde may be a problem. Similar to formaldehyde, glutaraldehyde could inactivate PRRSV but did not preserve the viral entry-associated domains and is therefore not useful for inactivated PRRSV vaccine development Citation[41]. Glutaraldehyde was also shown to change the conformational structure of infectious bussal disease virus Citation[52].
In conclusion, glutaraldehyde can inactivate viruses properly, but there is not always protection against infection on challenge for the KV vaccines discussed here. This could be due to destruction of viral proteins by glutaraldehyde.
Aldrithiol or 2,2´-dithiodipyridine
Examples of virus inactivation with aldrithiol, dithiodipyridine (AT-2), are summarized in . This procedure for virus inactivation for KV vaccines has been investigated for HIV Citation[42], PRRSV Citation[41] and RSV Citation[53].
AT-2 oxidizes S-H groups, which results in formation of S-S bridges that crosslink proteins . As the aromatic thiolate is the better leaving group, the reaction equilibrium is shifted toward the aliphatic disulfides. The internal viral proteins are subjected to the intracellular (reducing) environment, and as a consequence the cysteine residues are present in thiol form (S-H). By contrast, the surface proteins of viruses are subjected to the extracellular (oxidizing) environment, and as a consequence, the cysteines are present as disulfides (S-S). Treatment with AT-2 results in covalent modification and functional inactivation of S-H-containing internal viral proteins, such as the nucleocapsid protein, which is required for HIV infectivity, whereas the envelope glycoproteins with disulfide bonded cysteines remain unaffected Citation[54].
HIV preparations inactivated with AT-2 did not retain detectable infectivity, but showed maintenance of conformational and functional integrity. This suggests that such virions may be useful as vaccine antigens Citation[42]. An RSV vaccine based on AT-2 inactivation was found to be effective in the protection of cotton rats against virus replication in the lungs. This suggests that compounds that inactivate retroviruses by targeting the zinc finger motif in their nucleocapsid proteins are also effective against RSV Citation[53].
However, AT-2 cannot inactivate all viruses. PRRSV is not sensitive for AT-2 and could not be inactivated by it Citation[41]. For PRRSV, disulfide brigdes between nucleocapsid proteins are important for virus infection, but as stated above, these disulfide bridges remain unaffected by AT-2 Citation[55]. It seems there are no free thiol groups in PRRSV that are important for infection. Probably, only viruses where free thiol groups are important for virus infection can be inactivated by AT-2.
There are also some vaccination studies where no full protection after vaccination can be assured. A vaccination study with AT-2 inactivated SIV showed that an immune response could be induced, and there was virological protection on challenge with homologous, but not heterologous, virus Citation[56]. Another study could not detect an immune response before challenge. There was an antibody response on challenge, but vaccination only partly protected against viremia and infection on challenge Citation[57].
In conclusion, AT-2 seems to inactivate HIV and SIV properly with preservation of the virion structure, but vaccination studies cannot prove that AT-2 inactivated virus can protect the host on challenge. AT-2 was not effective with other viruses.
Alkylating agents
β-propiolactone
β-propiolactone (BPL) is widely used to inactivate viruses for vaccine purposes , mainly acting as an alkylating agent on guanine of viral DNA or RNA Citation[58,59]. As illustrated in , the nucleophilic guanine of nucleic acids reacts with the electrophilic BPL through a nucleophilic substitution mechanism causing the ring opening of the strained BPL and the N-alkylation of guanine. As BPL is much more toxic as a carcinogen Citation[60] and displays the same mode of action as binary ethylene imine (BEI) discussed in the section ‘Aminoethyl ethylene imine’ the interested reader is referred to the more specialized literature. Interestingly, the mechanistic details and the chemical modifications of BPL treatment have recently been elucidated through systematic studies on nucleo-base, nucleoside and peptide model systems, revising the above G-alkylation consensus pathway and providing a more nuanced view on the chemistry involved Citation[59]. In fact, acylation and cross-linking reactions also occur, to a significant extent, with proteins (cysteine, methionine and histidine as most reactive residues) being modified more extensively than originally agreed from earlier literature, which can have serious implications for vaccine production. Indeed, modifications on amino acids by BPL treatment can, for example, lead to the disability of influenza viruses to enter cells by virus–membrane fusion and modification of hemagglutinin Citation[61,62].
BPL-inactivated virus vaccines against yellow fever Citation[63], SARS-CoV Citation[64], influenza Citation[65] and rabies Citation[66] were able to induce the production of neutralizing antibodies. However, BPL-inactivated influenza showed reduced hemagglutinin and neuraminidase activity Citation[62]. Vaccines based on BPL-inactivated virus against SARS-CoV Citation[64], hematopoietic necrosis virus (HNV) Citation[67] and influenza Citation[65], were able to protect on challenge.
In conclusion, BPL seems to inactivate viruses properly and is able to induce a protective immune response. This can be expected, as BPL can alkylate the viral genome. However, protein modification should be kept in mind when using BPL.
Aminoethyl ethylene imine
Virus inactivation by ethylene imines was first introduced over 30 years ago Citation[68,69]. Originally, two chemically similar compounds, ethylene imine (aziridine) and acetylethylene imine, were proposed for use in the development of KV vaccines Citation[70,71]. However, the selectivity of aziridine toward nucleic acids is not very high. To improve this selectivity, a product with more proto-nizable amino groups was necessary, and aminoethyl ethylene imine, also called BEI, which has two protonizable amino groups while aziridine only has one, was selected.
If a low concentration of BEI is used, the virus capsid is not alkylated, but BEI passes through the capsid and alkylates the genome Citation[72]. N7-guanine of the genome acts as a nucleophile and reacts with the electrophile BEI. The nucleophilic substitution reaction performed by N7-guanine causes an opening of the BEI ring and guanine becomes alkylated Citation[73]. In addition, adenine is alkylated via a ring-opening reaction with BEI Citation[72]. This ring-opening reaction in RNA nucleosides is approximately two- to three-times faster than that in DNA nucleosides Citation[74]. So far, there are no reports on any interaction of BEI with proteins, at least if low concentrations are used, which suggests that the neutralizing viral epitopes are preserved after treatment of a virus with BEI.
BEI is used for the inactivation of many viruses, such as FMDV Citation[70], RRV Citation[75], sheep pox Citation[76], HIV Citation[77], Nipah virus Citation[78], HNV Citation[67] and PRRSV Citation[79].
As mentioned above, the preservation of the viral structure should not be a problem if a low concentration of BEI is used. This has also been shown for PRRSV, where BEI could inactivate PRRSV with the preservation of the viral entry-associated domains Citation[41]. Furthermore, the inactivation of FMDV with BEI did not affect the antigenity of FMDV Citation[70].
Most vaccines based on BEI-inactivated virus are able to induce a protective immune response. Mice vaccinated with a purified BEI-inactivated RRV vaccine were protected against challenge with live virus. The vaccine induced significant levels of neutralizing antibody in all strains of mice tested Citation[75]. A local Egyptian sheep pox virus strain inactivated with BEI could induce virus-specific antibodies starting from 1-week post-vaccination until 4-weeks post-challenge. Vaccinated lambs were protected on challenge up to 6-months post-vaccination Citation[76]. Race et al. have shown that the experimental BEI-inactivated HIV vaccine induces virus-neutralizing antibodies against both the homologous vaccine strain and a heterologous virus strain Citation[77]. Also, virus-specific antibodies could be induced against BEI-inactivated Nipah virus; however, these did not neutralizing the virus in vitro Citation[78]. After vaccination of pigs with BEI-inactivated PRRSV, they induced a virus-specific and virus-neutralize antibody response, which was able to reduce viremia after challenge Citation[79]. However, rainbow trout vaccinated with BEI-inactivated HNV and challenged with live HNV showed no statistically significant differences between the cumulative percentage mortality in the group of fish immunized with the BEI vaccines and the mortality of fish in the mock-vaccinated control groups when tested 28 or 56 days after vaccination Citation[67].
In conclusion, BEI seems to inactivate viruses properly and most KV vaccines based on BEI inactivation protect the host after challenge with virulent virus, which is expected since BEI does not interfere with the virus structure if a low concentration is used. Only one KV vaccine did not protect the host on challenge, but the possible reason for this remains to be elucidated.
Denaturing procedures
pH
Increasing or decreasing the pH has an effect on proteins by protein denaturation. This means that the proteins adopt a different 3D structure. A low (acidic) or a high (alkaline) pH can inactivate viruses via denaturation of the secondary structures of proteins, thereby altering the conformation of viral proteins that are involved in attachment to and replication in a host cell. The conformation of spike proteins of coronaviruses for instance changes at pH 8 and, as a result, entry of the virus into the host cell is initiated, but a lower or higher pH is associated with a loss of reactivity Citation[80].
The pH also has an effect on the genomic level . The close proximity of the hydroxyl group of RNA to the phosphorous center of each internucleotide linkage facilitates transesterification under strongly acidic or strongly basic conditions Citation[81]. Base-catalyzed reactions proceed via a nucleophilic addition mechanism where the oxygen attacks the adjacent phosphorus center, with a breakage of the phosphodiester bond as a consequence .
To date, there are no reports about the use of extreme pH conditions for the inactivation of viruses for KV vaccines. However, inactivation kinetics were performed for SARS-CoV Citation[30]. The infectivity of SARS-CoV is sensitive to pH extremes, as moderate pH variations from 5 to 9 could not inactivate the virus, whereas extremely alkaline (pH 12 and 14) or acidic (pH 1 and 3) conditions could inactivate the virus .
Preserving the viral structure is a problem if viruses are inactivated under extreme pH conditions. PRRSV inactivation by changing the pH can inactivate the virus, but does not preserve the viral entry-associated domains on PRRSV . This suggests that, at least in the case of PRRSV, inactivation by changing the pH is not suitable to generate a proper KV vaccine Citation[41].
In conclusion, extreme pH can inactivate viruses, but the effect of a KV vaccine based on this inactivation method in terms of protection against challenge is unknown. It is possible that the outcome of a vaccine based on pH inactivation will give a poor immune response, as the virus structure can be destroyed.
Temperature
By heating or pasteurizing viruses, they can become inactivated by denaturation of the secondary and/or tertiary structures of the viral proteins. It is possible that the conformation of the viral proteins involved in attachment to and replication in a host cell is changed in this process Citation[82,83]. Thermal inactivation of viruses by RNA degradation via breakage of the phosphodiester bond has also been described Citation[84]. Virus inactivation at ‘low’ temperatures (below 41°C) is considered to be caused by degradation of the nucleic acid, whereas virus inactivation at ‘high’ temperature is related to protein denaturation Citation[84–86].
Heat inactivation has been studied as a method to develop a KV vaccine for SARS-CoV Citation[30], HIV Citation[87] and HNV Citation[67]. To test the ability of heat to inactivate the SARS-CoV, Darnell et al. incubated the virus at three temperatures (56, 65 and 75°C) for increasing periods of time Citation[30]. They found that at 56 and 65°C most of the virus was inactivated. However, some virus remained infectious at a level close to the detection limit for the assay, suggesting that some virus particles were stable at 56 and 65°C. One possible explanation for this result may be the presence of aggregates that slowly dissociate. Although the virus was incompletely inactivated at 56 and 65°C, even at 60 min of incubation, it was completely inactivated at 75°C after 45 min of incubation Citation[30]. Taken together, these results suggest that viral inactivation by pasteurization might be very effective if the treatment is long enough.
For the preservation of the viral structure, a high temperature is suggested to have an effect on the viral proteins, whereas an inactivation temperature below 41°C is suggested to have no effect on protein level Citation[84–86]. In vitro infection assays and western blot analysis showed that in the case of PRRSV the entry-associated domains were indeed preserved after treatment at 37°C and that the virus did not replicate anymore Citation[41]. However, heat-inactivated influenza virus (>55°C) lost hemagglutinin and neuraminidase activity Citation[62].
When it comes to induction of neutralizing antibodies and protection after vaccination, the outcome is vaccine dependent. When BALB/c mice and rhesus macaques (Macaca mulatta) were vaccinated with HIV samples inactivated at 62°C, virus-specific antibodies were observed after the first vaccination. In addition, the sera contained antibodies capable of neutralizing the vaccine strain Citation[87]. In another study, HNV inactivated at 50°C did not protect fish against challenge. The percentage mortality of fish in the vaccinated groups was not statistically different compared with the mortality of fish in either the mock-vaccinated or unhandled control groups Citation[67].
In conclusion, it has been shown that temperature can inactivate viruses, but some heat-resistant particles can remain in the inactivated samples. Some heat-inactivated vaccines could induce a neutralizing antibody response, whereas others could not prevent disease. This is probably due to protein modification, because a high inactivation temperature was used. The inactivation temperature should be below 41°C if protein modifications are not desirable.
Irradiation
Ultraviolet light
Based on wavelength, Ultraviolet (UV) light is subdivided into three classifications: UVA (320–400 nm), UVB (280–320 nm) and UVC (200–280 nm) Citation[30]. UVC is absorbed by RNA and DNA bases and can lead to formation of dimers between two adjacent pyrimidines (uracil and thymine) . UVB can also induce formation of pyrimidine dimers, but is 20- to 100-fold less efficiently than UVC Citation[88]. UVA is weakly absorbed by RNA and DNA and forms pyrimidine dimers much less efficiently than UVB and UVC Citation[88].
Thus, in general, UV causes formation of pyrimidine dimers between two adjacent or opposing pyrimidines Citation[89,90]. The two pyrimidines react through a pericyclic 2π–2π cycloaddition reaction resulting in the formation of a cyclobutane ring . The dimers cause a certain strain in the sugar backbone of the genome, which possibly leads to breaks in the genome. In addition, the uracil dimers formed by UV irradiation inactivate the RNA molecule as a transcription template Citation[91].
This procedure for virus inactivation has been tested to develop a KV vaccine against viruses such as rabbit hemorrhagic disease virus (RHDV) Citation[92], murine leukemia virus strain Cas-Br-M Citation[93] and PRRSV Citation[79] and are summarized in .
Some vaccines based on UV-inactivated viruses are able to induce an immune response. UV-inactivated murine leukemia virus strain Cas-Br-M (UV-Cas), induced a strong, Cas-specific cytotoxic T-lymphocyte response in newborn NFS/N mice. Vaccination with UV-Cas protected mice against disease and reduced virus replication Citation[93]. In addition, vaccination of pigs with UV-inactivated PRRSV induced virus-specific and virus-neutralizing antibody responses, which were able to reduce viremia after challenge Citation[79].
However, there are also UV-inactivated vaccines that do not give protection against virus infection. Rabbits that were vaccinated with UV-inactivated RHDV and challenged with virulent virus, all died within 82 h after challenge. No antibodies were detected at the time of death. These findings show that vaccination with UV-inactivated RHDV does not protect rabbits against challenge with virulent virus in this study Citation[92].
In terms of the preservation of the viral structure after UV inactivation, caution is necessary. UV could inactivate PRRSV with preservation of the viral entry-associated domains Citation[41], but this is not the case for all viruses. The infectivity of mengovirus is lost very rapidly on exposure to UV irradiation, probably due to dimer formation in the viral RNA Citation[90]. Besides this rapid effect on RNA and DNA, UV irradiation also causes structural modifications of the capsid proteins, resulting in the formation of large and small photoproducts, which is a much slower process Citation[90]. The formation of large photoproduct proteins has also been reported for UV-irradiated reovirus Citation[94].
In conclusion, it has been shown that UV irradiation inactivates viruses properly. If the virus structure is preserved after inactivation, the KV vaccine based on this UV-inactivated virus can protect the host on challenge. Some KV vaccines based on UV inactivation, however, do not protect the host after challenge with virulent virus. This might be due to the formation of photoproducts that interfere with the viral proteins.
Gamma irradiation
There are two mechanisms by which gamma irradiation can inactivate biological material. The first mechanism is a direct result of a photon depositing energy into the target. The transfer of this energy results in the dislocation of electrons and breakage of covalent bonds. The second pathway involves indirect damage via free radicals formed after breakage of covalent bonds. Viruses appear to be inactivated primarily by direct damage, via disruption of the genome Citation[95].
This inactivation procedure has been investigated for FMDV, Rauscher leukemia virus, HSV Citation[96] and Lassa virus Citation[97]. Inactivation kinetics showed that Rauscher leukemia virus and HSV were inactivated at a dose of 25 kGy. FMDV was inactivated with a dose of 40 kGy, and this was used successfully as antigen in the preparation of a vaccine against FMDV. The vaccine effectively protected the inoculated cattle against the disease Citation[96]. In another study, rhesus monkeys were vaccinated with gamma irradiation-inactivated Lassa virus. The vaccinated animals had antibodies against the three major viral proteins. However, after challenge all the monkeys showed viremia and died. The inactivated Lassa virus vaccine based on gamma irradiation could not protect the animals after challenge Citation[97].
Precaution is necessary when it comes to the preservation of the viral structure on inactivation by gamma irradiation. Gamma irradiation could inactivate PRRSV with the preservation of the viral entry-associated domains Citation[41], but for other viruses the formation of free radicals should be investigated, as they might destroy the viral proteins Citation[95].
In conclusion, gamma irradiation seems to inactivate viruses properly. Some KV vaccines based on gamma irradiation inactivation protect the host against disease after challenge with virulent virus, whereas others cannot prevent viremia and disease. This can be due to the formation of free radicals, which destroy viral proteins.
Expert commentary
Some issues that are important for virus inactivation for vaccine purposes have been defined. A crucial step in the development of a KV vaccine is a balanced inactivation of the virus. All virus particles need to be completely inactivated, but the inactivation method should only have a minor effect on the viral antigenic properties, as the immune system of the host has to recognize the neutralizing epitopes to produce neutralizing antibodies against the antigen. As the antibody response is mostly investigated in vaccination studies, this review focused on the induction of antibodies on vaccination. However, the effect on cell-mediated immune response is also of crucial importance and should be investigated in more depth when evaluating candidate vaccines. The virus inactivation as well as the virus structure preservation can be a problem that should be kept in mind when developing a KV vaccine.
First, during the inactivation process one should be aware of possible virus aggregate formation, which can lead to viruses escaping inactivation. A single virus titration does not always detect escaping virus. A quality control system to investigate the safety of an inactivated virus vaccine can be obtained by passaging the treated virus in vitro, which can give more information about the completeness of the virus inactivation. Only if the vaccine passes such a test, can further animal experiments be considered to confirm vaccine safety.
Second, in the development of a KV vaccine, the preservation of the virus structure is very important. Inactivation procedures acting on viral proteins, such as cross-linkers or denaturing procedures Citation[24,39,47,80,82,83], may modify the viral-neutralizing epitopes that are essential for induction of neutralizing antibodies. Thus, it is better to inactivate the virus for KV vaccines with methods that do not affect the viral proteins. Inactivation procedures mainly acting on the genome, such as radiation and alkylating agents Citation[72,90,95], will most likely preserve viral-neutralizing epitopes and should be preferred. Nevertheless, protein degradation after inactivation should always be checked.
Different quality control systems to investigate the antigen quality are available. For influenza, the effect of inactivation is investigated by measuring the hemagglutinating activity before and after inactivation Citation[98]. ELISA may be used for the determination of the attachment of neutralizing antibodies to viral epitopes after inactivation. This is used as quality control of the antigens of HIV Citation[87,99], rabies virus Citation[100,101] and PV vaccines Citation[102]. However, for the previous methods, knowledge of the neutralizing epitopes is necessary. Sometimes, the neutralizing epitopes are not known. As virus-neutralizing antibodies are often directed to viral proteins involved in virus entry Citation[41,103–105], it can be advised to preserve virus entry-associated domains to induce a virus-neutralizing antibody response. In this context, a quality control system for investigating the antigen quality of the inactivated virus can be developed by confirming the preservation of entry-associated domains of a virus Citation[41]. This can be achieved by investigating attachment and internalization of virus into the host cells before and after virus inactivation by immunofluorescence staining and confocal microscopy Citation[41].
In conclusion, many methods have been developed that are potentially suitable to inactivate a certain vaccine virus, but the success of these methods may vary depending on the particular virus and the conditions used (i.e., temperature, pH and concentration). Nonetheless, successful methods to inactivate a virus for vaccine development are best chosen from methods that mainly have an effect on the genome. Since formaldehyde, glutaraldehyde, pH and heat have an influence on protein level, it is possible that the neutralizing epitopes are modified by cross-linking (formaldehyde or glutaraldehyde) or denaturation (pH or temperature). In our opinion, UV irradiation, gamma irradiation, BPL and BEI are the most successful methods to inactivate a virus to create a KV vaccine, as these inactivation procedures mainly target the genome and have no or minor effects on viral proteins. However, it has to be considered that viral proteins may be cross-linked by BPL, or damaged by UV photoproducts and free radicals formed by gamma irradiation. The radiation target theory, however, predicts that the radiation sensitivity of biomolecules depends on their mass Citation[106]. Therefore, viral genomes, with a significantly lower mass, would be significantly more sensitive to damage than proteins.
Five-year view
Until recently, the search for an effective KV vaccine was often a process of trial and error, in which a virus was inactivated by a certain inactivation method and then immediately tested in animals to investigate complete virus inactivation, antibody response on vaccination and protection on challenge. Currently, a series of in vitro tools is available, allowing the creation of a quality control system, in which virus inactivation (safety) and antigen quality (immune response) can be investigated. In this way, vaccine efficacy can be predicted prior to animal experiments. As a consequence, outbreaks due to incomplete virus inactivation and poor immune responses due to viral protein destruction can be avoided as much as possible.
It appears that virus inactivation methods exerting their main effects on the viral genome have most potential in generating an effective immune response and protecting the host against disease. On in vitro testing of several inactivation methods following a quality control system for an inactivated PRRSV vaccine, we have observed that only a few of the available methods allow complete virus inactivation with preservation of the virion structure. Considering the molecular and mechanistic basis of these evaluated methods, it is clear that they all mainly affect the viral genome, leaving the viral proteins unaffected. In our opinion, it is therefore of crucial importance for the induction of a proper immune response and protection against disease, to evolve the development methods for KV vaccines based on viral genome inactivation, which can guarantee an intact virion structure.
Table 1. Overview of inactivation methods for the development of killed virus vaccines.
Table 2. Effect of formaldehyde on different viruses: inactivation, viral protein modification, induction of virus-specific and virus-neutralization antibodies and protection on challenge (reduction of viremia and fewer clinical signs).
Table 3. Effect of glutaraldehyde on different viruses: inactivation, viral protein modification, induction of virus-specific and virus-neutralization antibodies and protection on challenge (reduction of viremia and fewer clinical signs).
Table 4. Effect of 2,2’-dithiodipyridine on different viruses: inactivation, viral protein modification, induction of virus-specific and virus-neutralization antibodies and protection on challenge (reduction of viremia and fewer clinical signs).
Table 5. Effect of β-propiolactone on different viruses: inactivation, viral protein modification, induction of virus-specific and virus-neutralization antibodies and protection on challenge (reduction of viremia and fewer clinical signs).
Table 6. Effect of binary ethylene imine on different viruses: inactivation, viral protein modification, induction of virus-specific and virus-neutralization antibodies and protection on challenge (reduction of viremia and fewer clinical signs).
Table 7. Effect of denaturing agents on different viruses: inactivation, viral protein modification, induction of virus-specific and virus-neutralization antibodies and protection on challenge (reduction of viremia and fewer clinical signs).
Table 8. Effect of irradiation on different viruses: inactivation, viral protein modification, induction of virus-specific and virus-neutralization antibodies and protection on challenge (reduction of viremia and fewer clinical signs).
Key issues
• For safety reasons, killed virus (KV) vaccines are preferred over modified live virus vaccines. However, KV vaccines can suffer from problems related to efficacy.
• A good in vitro quality control system is required for prediction of the effectiveness of a KV vaccine. This quality control system should allow verification of the completeness of virus inactivation and the quality of the resulting antigen.
• In order to create a safe KV vaccine, the virus inactivation method should inactivate the virus mixture completely without risk of resistant virus particles. Due to aggregate formation, incompletely inactivated virus particles can still be present in the vaccine with outbreaks as a consequence.
• For the generation of a KV vaccine able to induce a proper immune response, antigen quality is of high importance. Due to protein crosslinking, protein denaturation and protein degradation, the viral antigen can be completely destroyed, with a poor immune response as a consequence.
• Crosslinkers, such as formaldehyde and glutaraldehyde, or denaturing processes, such as pH and heat, can have an influence on viral proteins and destroy the virion structure. As a consequence, KV vaccines based on these inactivation methods might not induce a proper immune response.
• Next to alkylating agents, such as β-propiolactone and binary ethylene imine, ultraviolet and gamma irradiation mainly influence the viral genome, preserving the virion structure. These methods are thus highly recommended for generation of an efficient and safe KV vaccine.
Financial & competing interests disclosure
I Delrue was funded by the Agricultural Research Program from the Institute for the Promotion of Innovation by Science and Technology in Flanders (IWT-LO 040721). D Verzele and A Madder acknowledge financial support from Ghent University (BOF-01J06111). H Nauwynck acknowledges the EU (Seventh Framework Program; Project No. 245141) for financial support. The authors have no other relevant affiliations or financial involvement with any organization or entity with a financial interest in or financial conflict with the subject matter or materials discussed in the manuscript apart from those disclosed.
No writing assistance was utilized in the production of this manuscript
References
- King AM, Underwood BO, McCahon D, Newman JW, Brown F. Biochemical identification of viruses causing the 1981 outbreaks of foot and mouth disease in the UK. Nature 293(5832), 479–480 (1981).
- Beck E, Strohmaier K. Subtyping of European foot-and-mouth disease virus strains by nucleotide sequence determination. J. Virol. 61(5), 1621–1629 (1987).
- Patil PK, Suryanarayana V, Bist P, Bayry J, Natarajan C. Integrity of GH-loop of foot-and-mouth disease virus during virus inactivation: detection by epitope specific antibodies. Vaccine 20(7–8), 1163–1168 (2002).
- Cham BE, Vickery K, Tohidi-Esfahani R, Cossart Y. Delipidation of a hepadnavirus: Viral inactivation and vaccine development. J. Virol. Methods 137(1), 160–163 (2006).
- Niedrig M, Gregersen JP, Fultz PN, Bröker M, Mehdi S, Hilfenhaus J. Immune response of chimpanzees after immunization with the inactivated whole immunodeficiency virus (HIV-1), three different adjuvants and challenge. Vaccine 11(1), 67–74 (1993).
- Jahrling PB, Stephenson EH. Protective efficacies of live attenuated and formaldehyde-inactivated Venezuelan equine encephalitis virus vaccines against aerosol challenge in hamsters. J. Clin. Microbiol. 19(3), 429–431 (1984).
- Kistner O, Barrett N, Brühmann A et al. The preclinical testing of a formaldehyde inactivated Ross River virus vaccine designed for use in humans. Vaccine 25(25), 4845–4852 (2007).
- Samina I, Khinich Y, Simanov M, Malkinson M. An inactivated West Nile virus vaccine for domestic geese-efficacy study and a summary of 4 years of field application. Vaccine 23(41), 4955–4958 (2005).
- Putnak R, Barvir DA, Burrous JM et al. Development of a purified, inactivated, dengue-2 virus vaccine prototype in Vero cells: immunogenicity and protection in mice and rhesus monkeys. J. Infect. Dis. 174(6), 1176–1184 (1996).
- Skinner GR, Davies J. Efficacy of an inactivated vaccine for equine herpesvirus type 1 in a novel hamster model. Intervirology 43(1), 27–35 (2000).
- Bell JA, Sundberg JP, Ghim SJ, Newsome J, Jenson AB, Schlegel R. A formalin-inactivated vaccine protects against mucosal papillomavirus infection: a canine model. Pathobiology 62(4), 194–198 (1994).
- Choi Y, Ahn CJ, Seong KM, Jung MY, Ahn BY. Inactivated Hantaan virus vaccine derived from suspension culture of Vero cells. Vaccine 21(17–18), 1867–1873 (2003).
- Bahgat MM, Kutkat MA, Nasraa MH et al. Characterization of an avian influenza virus H5N1 Egyptian isolate. J. Virol. Methods 159(2), 244–250 (2009).
- Furesz J, Scheifele DW, Palkonyay L. Safety and effectiveness of the new inactivated hepatitis A virus vaccine. CMAJ 152(3), 343–348 (1995).
- Goncharova E, Ryzhikov E, Poryvaev V et al. Intranasal immunization with inactivated tick-borne encephalitis virus and the antigenic peptide 89–119 protects mice against intraperitoneal challenge. Int. J. Med. Microbiol. 296(Suppl. 40), 195–201 (2006).
- Heinz F, Holtzmann H, Essl A, Kundt M. [Analysis of the efficiency of tick-borne encephalitis vaccination in the population in the natural foci of Austria]. Vopr. Virusol. 53(2), 19–27 (2008).
- Appaiahgari MB, Vrati S. Immunogenicity and protective efficacy in mice of a formaldehyde-inactivated Indian strain of Japanese encephalitis virus grown in Vero cells. Vaccine 22(27–28), 3669–3675 (2004).
- Alderson T. The mechanism of formaldehyde-induced mutagenesis. The monohydroxymethlyation reaction of formaldehyde with adenylic acid as the necessary and sufficient condition for the mediation of the mutagenic activity of formaldehyde. Mutat. Res. 106, 77–85 (1964).
- Progress in the nucleic acid research and molecular biology. Davidson JN (Ed.). Academic Press, New York, NY (1973).
- Fraenkel-Conrat H. Reaction of nucleic acid with formaldehyde. Biochim. Biophys. Acta 15(2), 307–309 (1954).
- Fraenkel-Conrat H. Chemical modification of viral ribonucleic acid. I. Alkylating agents. Biochim. Biophys. Acta 49, 169–180 (1961).
- Michelson AM, Letters R. Synthesis of nucleotide-aminoacyl anhydrides. Biochim. Biophys. Acta 80, 242–246 (1964).
- Metz B, Kersten GF, Hoogerhout P et al. Identification of formaldehyde-induced modifications in proteins: reactions with model peptides. J. Biol. Chem. 279(8), 6235–6243 (2004).
- Fraenkel-Conrat H, Mecham DK. The reaction of formaldehyde with proteins; demonstration of intermolecular cross-linking by means of osmotic pressure measurements. J. Biol. Chem. 177(1), 477–486 (1949).
- Kuykendall JR, Bogdanffy MS. Efficiency of DNA-histone crosslinking induced by saturated and unsaturated aldehydes in vitro. Mutat. Res. 283(2), 131–136 (1992).
- Ma TH, Harris MM. Review of the genotoxicity of formaldehyde. Mutat. Res. 196(1), 37–59 (1988).
- Permana PA, Snapka RM. Aldehyde-induced protein-DNA crosslinks disrupt specific stages of SV40 DNA replication. Carcinogenesis 15(5), 1031–1036 (1994).
- Brown F. Review of accidents caused by incomplete inactivation of viruses. Dev. Biol. Stand. 81, 103–107 (1993).
- Twomey T, Newman J, Burrage T, Piatti P, Lubroth J, Brown F. Structure and immunogenicity of experimental foot-and-mouth disease and poliomyelitis vaccines. Vaccine 13(16), 1603–1610 (1995).
- Darnell ME, Subbarao K, Feinstone SM, Taylor DR. Inactivation of the coronavirus that induces severe acute respiratory syndrome, SARS-CoV. J. Virol. Methods 121(1), 85–91 (2004).
- Spruth M, Kistner O, Savidis-Dacho H et al. A double-inactivated whole virus candidate SARS coronavirus vaccine stimulates neutralising and protective antibody responses. Vaccine 24(5), 652–661 (2006).
- Qu D, Zheng B, Yao X et al. Intranasal immunization with inactivated SARS-CoV (SARS-associated coronavirus) induced local and serum antibodies in mice. Vaccine 23(7), 924–931 (2005).
- Zhang CH, Guo ZM, Zheng HY et al. Humoral immune responses in rabbits induced by an experimental inactivated severe acute respiratory syndrome coronavirus vaccine prepared from F69 strain. Chin. Med. J. 117(11), 1625–1629 (2004).
- Openshaw PJ, Culley FJ, Olszewska W. Immunopathogenesis of vaccine-enhanced RSV disease. Vaccine 20(Suppl. 1), S27–S31 (2001).
- Ishizaka ST, Israel ZR, Gettie A et al. Induction of mucosal antibody responses by microsphere-encapsulated formalin-inactivated simian immunodeficiency virus in a male urethral challenge model. Vaccine 17(22), 2817–2825 (1999).
- Huisman W, Martina BE, Rimmelzwaan GF, Gruters RA, Osterhaus AD. Vaccine-induced enhancement of viral infections. Vaccine 27(4), 505–512 (2009).
- Griffin DE, Pan CH, Moss WJ. Measles vaccines. Front. Biosci. 13, 1352–1370 (2008).
- Kalina WV, Woolums AR, Berghaus RD, Gershwin LJ. Formalin-inactivated bovine RSV vaccine enhances a Th2 mediated immune response in infected cattle. Vaccine 22(11–12), 1465–1474 (2004).
- Tano Y, Shimizu H, Martin J, Nishimura Y, Simizu B, Miyamura T. Antigenic characterization of a formalin-inactivated poliovirus vaccine derived from live-attenuated Sabin strains. Vaccine 25(41), 7041–7046 (2007).
- Delputte PL, Meerts P, Costers S, Nauwynck HJ. Effect of virus-specific antibodies on attachment, internalization and infection of porcine reproductive and respiratory syndrome virus in primary macrophages. Vet. Immunol. Immunopathol. 102(3), 179–188 (2004).
- Delrue I, Delputte PL, Nauwynck HJ. Assessing the functionality of viral entry-associated domains of porcine reproductive and respiratory syndrome virus during inactivation procedures, a potential tool to optimize inactivated vaccines. Vet. Res. 40(6), 62 (2009).
- Rossio JL, Esser MT, Suryanarayana K et al. Inactivation of human immunodeficiency virus type 1 infectivity with preservation of conformational and functional integrity of virion surface proteins. J. Virol. 72(10), 7992–8001 (1998).
- Pollock RV, Carmichael LE. Dog response to inactivated canine parvovirus and feline panleukopenia virus vaccines. Cornell Vet. 72(1), 16–35 (1982).
- Embalming Chemistry: Glutaraldehyde Versus Formaldehyde. Bedino JH (Ed.). The Champion Company, Springfield, OH, USA (2003).
- Hemminki K, Suni R. Sites of reaction of glutaraldehyde and acetaldehyde with nucleosides. Arch. Toxicol. 55(3), 186–190 (1984).
- McGucken PV, Woodside W. Studies on the mode of action of glutaraldehyde on Escherichia coli. J. Appl. Bacteriol. 36(3), 419–426 (1973).
- Cheung DT, Nimni ME. Mechanism of crosslinking of proteins by glutaraldehyde II. Reaction with monomeric and polymeric collagen. Connect. Tissue Res. 10(2), 201–216 (1982).
- Richards FM, Knowles JR. Glutaraldehyde as a protein cross-linkage reagent. J. Mol. Biol. 37(1), 231–233 (1968).
- Advances in Protein Chemistry. Anfinsen CB (Ed.). Academic Press, New York, NY, USA (1970).
- Bioconjugate Techiques. Hermanson GT (Ed.). Academic Press, San Diego, CA, USA (2008).
- Keles I, Woldehiwet Z, Murray RD. Vaccination with glutaraldehyde-fixed bovine respiratory syncytial virus (BRSV)-infected cells stimulates a better immune response in lambs than vaccination with heat-inactivated cell-free BRSV. Vaccine 16(11–12), 1172–1178 (1998).
- Cepica A, Beauregard M, Qian B. Fluorescence spectroscopy monitoring of the conformational restraint of formaldehyde- and glutaraldehyde-treated infectious bursal disease virus proteins. Vaccine 16(20), 1957–1961 (1998).
- Boukhvalova MS, Prince GA, Blanco JC. The cotton rat model of respiratory viral infections. Biologicals 37(3), 152–159 (2009).
- Chertova E, Crise BJ, Morcock DR, Bess JW Jr, Henderson LE, Lifson JD. Sites, mechanism of action and lack of reversibility of primate lentivirus inactivation by preferential covalent modification of virion internal proteins. Curr. Mol. Med. 3(3), 265–272 (2003).
- Wootton SK, Yoo D. Homo-oligomerization of the porcine reproductive and respiratory syndrome virus nucleocapsid protein and the role of disulfide linkages. J. Virol. 77(8), 4546–4557 (2003).
- Lifson JD, Rossio JL, Piatak M Jr et al. Evaluation of the safety, immunogenicity, and protective efficacy of whole inactivated simian immunodeficiency virus (SIV) vaccines with conformationally and functionally intact envelope glycoproteins. AIDS Res. Hum. Retroviruses 20(7), 772–787 (2004).
- Vagenas P, Williams VG, Piatak M Jr et al. Tonsillar application of AT-2 SIV affords partial protection against rectal challenge with SIVmac239. J. Acquir. Immune Defic. Syndr. 52(4), 433–442 (2009).
- Perrin P, Morgeaux S. Inactivation of DNA by beta-propiolactone. Biologicals 23(3), 207–211 (1995).
- Uittenbogaard JP, Zomer B, Hoogerhout P, Metz B. Reactions of beta-propiolactone with nucleobase analogues, nucleosides, and peptides: implications for the inactivation of viruses. J. Biol. Chem. 286(42), 36198–36214 (2011).
- Larghi OP, Nebel AE. Rabies virus inactivation by binary ethylenimine: new method for inactivated vaccine production. J. Clin. Microbiol. 11(2), 120–122 (1980).
- Desbat B, Lancelot E, Krell T et al. Effect of the b-propiolactone treatment on the adsorption and fusion of influenza A/Brisbane/59/2007 and A/New Caledonia/20/1999 virus H1N1 on a dimyristoylphosphatidylcholine/ganglioside GM3 mixed phospholipids monolayer at the air-water interface. Langmuir 27(22), 13675–13683 (2011).
- Jonges M, Liu WM, van der Vries E et al. Influenza virus inactivation for studies of antigenicity and phenotypic neuraminidase inhibitor resistance profiling. J. Clin. Microbiol. 48(3), 928–940 (2010).
- Monath TP, Fowler E, Johnson CT et al. An inactivated cell-culture vaccine against yellow fever. N. Engl. J. Med. 364(14), 1326–1333 (2011).
- Roberts A, Lamirande EW, Vogel L et al. Immunogenicity and protective efficacy in mice and hamsters of a β-propiolactone inactivated whole virus SARS-CoV vaccine. Viral Immunol. 23(5), 509–519 (2010).
- Bodewes R, Kreijtz JH, van Amerongen G et al. A single immunization with CoVaccine HT-adjuvanted H5N1 influenza virus vaccine induces protective cellular and humoral immune responses in ferrets. J. Virol. 84(16), 7943–7952 (2010).
- Sabchareon A, Lang J, Attanath P et al. A new Vero cell rabies vaccine: results of a comparative trial with human diploid cell rabies vaccine in children. Clin. Infect. Dis. 29(1), 141–149 (1999).
- Anderson E, Clouthier S, Shewmaker W, Weighall A, LaPatra S. Inactivated infectious haematopoietic necrosis virus (IHNV) vaccines. J. Fish Dis. 31(10), 729–745 (2008).
- Warrington RE, Cunliffe HR, Bachrach HL. Derivatives of aziridine as inactivants for foot-and-mouth disease virus vaccines. Am. J. Vet. Res. 34(8), 1087–1091 (1973).
- Bahnemann HG. Inactivation of viruses in serum with binary ethyleneimine. J. Clin. Microbiol. 3(2), 209–210 (1976).
- Bahnemann HG. Binary ethylenimine as an inactivant for foot-and-mouth disease virus and its application for vaccine production. Arch. Virol. 47(1), 47–56 (1975).
- Bahnemann HG. Inactivation of viral antigens for vaccine preparation with particular reference to the application of binary ethylenimine. Vaccine 8(4), 299–303 (1990).
- Broo K, Wei J, Marshall D et al. Viral capsid mobility: a dynamic conduit for inactivation. Proc. Natl Acad. Sci. USA 98(5), 2274–2277 (2001).
- Gates KS, Nooner T, Dutta S. Biologically relevant chemical reactions of N7-alkylguanine residues in DNA. Chem. Res. Toxicol. 17(7), 839–856 (2004).
- Hendler S, Fürer E, Srinivasan PR. Synthesis and chemical properties of monomers and polymers containing 7-methylguanine and an investigation of their substrate or template properties for bacterial deoxyribonucleic acid or ribonucleic acid polymerases. Biochemistry 9(21), 4141–4153 (1970).
- Aaskov J, Williams L, Yu S. A candidate Ross River virus vaccine: preclinical evaluation. Vaccine 15(12–13), 1396–1404 (1997).
- Awad M, Michael A, Soliman SM, Samir SS, Daoud AM. Trials for preparation of inactivated sheep pox vaccine using binary ethyleneimine. Egypt. J. Immunol. 10(2), 67–72 (2003).
- Race E, Frezza P, Stephens DM et al. An experimental chemically inactivated HIV-1 vaccine induces antibodies that neutralize homologous and heterologous viruses. Vaccine 13(1), 54–60 (1995).
- Berhane Y, Berry JD, Ranadheera C et al. Production and characterization of monoclonal antibodies against binary ethylenimine inactivated Nipah virus. J. Virol. Methods 132(1–2), 59–68 (2006).
- Vanhee M, Delputte PL, Delrue I, Geldhof MF, Nauwynck HJ. Development of an experimental inactivated PRRSV vaccine that induces virus-neutralizing antibodies. Vet. Res. 40(6), 63 (2009).
- Weismiller DG, Sturman LS, Buchmeier MJ, Fleming JO, Holmes KV. Monoclonal antibodies to the peplomer glycoprotein of coronavirus mouse hepatitis virus identify two subunits and detect a conformational change in the subunit released under mild alkaline conditions. J. Virol. 64(6), 3051–3055 (1990).
- Li Y, Breaker RR. Kinetics of RNA degradation by specific base catalysis of transesterification involving the 2´-hydroxyl group. J. Am. Chem. Soc. 121, 5364–5372 (1999).
- Lelie PN, Reesink HW, Lucas CJ. Inactivation of 12 viruses by heating steps applied during manufacture of a hepatitis B vaccine. J. Med. Virol. 23(3), 297–301 (1987).
- Schlegel A, Immelmann A, Kempf C. Virus inactivation of plasma-derived proteins by pasteurization in the presence of guanidine hydrochloride. Transfusion 41(3), 382–389 (2001).
- Fleming P. Thermal inactivation of Semliki Forest virus. J. Gen. Virol. 13(3), 385–391 (1971).
- Dimmock NJ. Differences between the thermal inactivation of picornaviruses at “high” and “low” temperatures. Virology 31(2), 338–353 (1967).
- Laude H. Thermal inactivation studies of a coronavirus, transmissible gastroenteritis virus. J. Gen. Virol. 56(Pt 2), 235–240 (1981).
- Poon B, Safrit JT, McClure H et al. Induction of humoral immune responses following vaccination with envelope-containing, formaldehyde-treated, thermally inactivated human immunodeficiency virus type 1. J. Virol. 79(8), 4927–4935 (2005).
- Perdiz D, Grof P, Mezzina M, Nikaido O, Moustacchi E, Sage E. Distribution and repair of bipyrimidine photoproducts in solar UV-irradiated mammalian cells. Possible role of Dewar photoproducts in solar mutagenesis. J. Biol. Chem. 275(35), 26732–26742 (2000).
- Sinha RP, Häder DP. UV-induced DNA damage and repair: a review. Photochem. Photobiol. Sci. 1(4), 225–236 (2002).
- Miller RL, Plagemann PG. Effect of ultraviolet light on mengovirus: formation of uracil dimers, instability and degradation of capsid, and covalent linkage of protein to viral RNA. J. Virol. 13(3), 729–739 (1974).
- Sauerbier W, Hercules K. Gene and transcription unit mapping by radiation effects. Annu. Rev. Genet. 12, 329–363 (1978).
- Henning J, Meers J, Davies PR. Exposure of rabbits to ultraviolet light-inactivated rabbit haemorrhagic disease virus (RHDV) and subsequent challenge with virulent virus. Epidemiol. Infect. 133(4), 731–735 (2005).
- Sarzotti M, Dean TA, Remington M, Hoffman PM. Ultraviolet-light-inactivated Cas-Br-M murine leukemia virus induces a protective CD8+ cytotoxic T lymphocyte response in newborn mice. AIDS Res. Hum. Retroviruses 10(12), 1695–1702 (1994).
- Subasinghe HA, Loh PC. Reovirus cytotoxicity: some properties of the UV-irradiated reovirus and its capsid proteins. Arch. Gesamte Virusforsch. 39(1), 172–189 (1972).
- Grieb T, Forng RY, Brown R et al. Effective use of gamma irradiation for pathogen inactivation of monoclonal antibody preparations. Biologicals 30(3), 207–216 (2002).
- Smolko EE, Lombardo JH. Virus inactivation studies using ion beams, electron and gamma irradiation. Nuclear Instrum. Methods Physics Res. Sec. B-Beam Interact. Mater. Atoms 236, 249–253 (2005).
- McCormick JB, Mitchell SW, Kiley MP, Ruo S, Fisher-Hoch SP. Inactivated Lassa virus elicits a non protective immune response in rhesus monkeys. J. Med. Virol. 37(1), 1–7 (1992).
- Di Trani L, Cordioli P, Falcone E et al. Standardization of an inactivated H17N1 avian influenza vaccine and efficacy against A/Chicken/Italy/13474/99 high-pathogenicity virus infection. Avian Dis. 47(Suppl. 3), 1042–1046 (2003).
- Grovit-Ferbas K, Hsu JF, Ferbas J, Gudeman V, Chen IS. Enhanced binding of antibodies to neutralization epitopes following thermal and chemical inactivation of human immunodeficiency virus type 1. J. Virol. 74(13), 5802–5809 (2000).
- Rooijakkers EJ, Uittenbogaard JP, Groen J, Osterhaus AD. Rabies vaccine potency control: comparison of ELISA systems for antigenicity testing. J. Virol. Methods 58(1–2), 111–119 (1996).
- Fournier-Caruana J, Poirier B, Haond G et al. Inactivated rabies vaccine control and release: use of an ELISA method. Biologicals 31(1), 9–16 (2003).
- Morgeaux S, Milne C, Daas A. Feasibility study to develop a common in vitro D antigen assay for inactivated poliomyelitis vaccines. Pharmeuropa Bio 2005(1), 19–26 (2005).
- Owsianka AM, Tarr AW, Keck ZY et al. Broadly neutralizing human monoclonal antibodies to the hepatitis C virus E2 glycoprotein. J. Gen. Virol. 89(Pt 3), 653–659 (2008).
- Lee JE, Saphire EO. Neutralizing ebolavirus: structural insights into the envelope glycoprotein and antibodies targeted against it. Curr. Opin. Struct. Biol. 19(4), 408–417 (2009).
- Coughlin MM, Babcook J, Prabhakar BS. Human monoclonal antibodies to SARS-coronavirus inhibit infection by different mechanisms. Virology 394(1), 39–46 (2009).
- Osborne JC Jr, Miller JH, Kempner ES. Molecular mass and volume in radiation target theory. Biophys. J. 78(4), 1698–1702 (2000).
- Feron VJ, Til HP, de Vrijer F, Woutersen RA, Cassee FR, van Bladeren PJ. Aldehydes: occurrence, carcinogenic potential, mechanism of action and risk assessment. Mutat. Res. 259(3–4), 363–385 (1991).