Abstract
Background
Fungal infections are becoming more prevalent and threatening because of the continuous emergence of azole-resistant fungal infections. The present study was aimed to assess the activity of free Methylglyoxal (MG) or MG-conjugated chitosan nanoparticles (MGCN) against fluconazole-resistant Candida albicans.
Materials and Methods
A novel formulation of MGCN was prepared and characterized to determine their size, shape and polydispersity index. Moreover, the efficacy of fluconazole or MG or MGCN was determined against intracellular C. albicans in macrophages and the systematic candidiasis in a murine model. The safety of MG or MGCN was tested in mice by analyzing the levels of hepatic and renal toxicity parameters.
Results
Candida albicans did not respond to fluconazole, even at the highest dose of 20 mg/kg, whereas MG and MGCN effectively eliminated C. albicans from the macrophages and infected mice. Mice in the group treated with MGCN at a dose of 10 mg/kg exhibited a 90% survival rate and showed the lowest fungal load in the kidney, whereas the mice treated with free MG at the same dose exhibited 50% survival rate. Moreover, the administration of MG or MGCN did not induce any liver and kidney toxicity in the treated mice.
Conclusion
The findings of the present work suggest that MGCN may be proved a promising therapeutic formulation to treat azole-resistant C. albicans infections.
Introduction
Candida albicans is a normal commensal, but it can act as a pathogen in immunocompromised persons, ie patients receiving chemotherapy for organ transplantation and cancer treatment, or persons on prolonged antibiotic or steroid therapy.Citation1–Citation3 Candida albicans causes infections that range from the mucosal to the systemic candidiasis in immunocompromised subjects. Azoles (fluconazole, itraconazole) are commonly used antibiotics to treat fungal infections in the human population, but their widespread use has resulted in the emergence of azole-resistant fungal pathogens.Citation4–Citation7 Amphotericin B, a polyene antibiotic, is a gold standard and the most effective antifungal drug used in the treatment of deep-seated fungal infections, but the acute hepatic and renal toxicity limits its use in human beings.Citation8,Citation9
The status of the immune system plays a critical part in the outcome of active C. albicans infection. Since neutrophils and macrophages are the front line cells that start an onslaught against C. albicans, mice deficient in leukocytes and macrophages showed severe susceptibility to C. albicans.Citation10,Citation12 Moreover, human immunodeficiency virus (HIV)-infected individuals showed greater susceptibility to orogastric candidiasis owing to the impairment of macrophages.Citation13 The CD4+ T helper cells and CD8+ cytotoxic T cells play an important role in mounting anti-fungal immunity to candidiasis.Citation14
Methylglyoxal (MG) is a reactive dicarbonyl compound that is produced as a natural by-product of glucose and fatty acid metabolic pathways. It has been shown to kill the cancerous cells through the inhibition of glycolysis and mitochondrial respiration.Citation15 MG, an active component in Manuka honey, has shown efficacy against the biofilm formation in Pseudomonas aeruginosa and Staphylococcus aureus.Citation16 MG has been shown to act as an antibiotic adjuvant and increases the activity of linezolid against Staphylococcus aureus.Citation17 A combination of honey and phage has been shown to possess a synergistic effect against the biofilm formation by Escherichia coli.Citation18 Antibacterial activity of MG is suggested to be mediated through the structural changes of bacterial fimbriae and flagella. Furthermore, MG has demonstrated antimalarial activities as well.Citation19
Chitosan is a natural and biodegradable polysaccharide with a broad-spectrum antimicrobial activity that is correlated to its deacetylation as a higher degree of deacetylation increases the antimicrobial activity of chitosan.Citation20–Citation22 Besides, the antimicrobial activity of chitosan is also pH-dependent. It shows increased activity at lower pH because of its cationic nature.Citation21 Cationic chitosan binds to the negatively charged cell membrane that causes an interference in the energy metabolism of pathogens.Citation23 Chitosan exerts its activity against Candida albicans through the inhibition of SAGA complex and was supported by the fact that chitosan inhibited ADA2 and ada2–regulated cell wall-related genes. Moreover, it reduced the levels of chitin and β-glucan that alters the structure of cell wall and plasma membrane via the inhibition of SAGA complex component expression.Citation24
In recent years, various drug delivery systems including liposomes and nanoparticles (NPs) have been developed as the carriers of therapeutic agents. We have earlier shown that the encapsulation of antifungal drugs in liposomes resulted in enhanced activity against C. albicans, Aspergillus fumigatus and Cryptococcus neoformans.Citation25–Citation27 In the present study, we determined the activity of MG or MGCN against C. albicans both in vitro or in vivo studies as well. The results of the present study demonstrated that MGCN, a formulation of methylglyoxal-conjugated chitosan nanoparticles, effectively eliminated C. albicans infection from a murine model.
Materials and Methods
Materials
Methylglyoxal and fluconazole were procured from Santa Cruz Biotechnology (Dallas, TX, USA). RPMI, glutaraldehyde and low-molecular-weight chitosan were purchased from Sigma-Aldrich (St. Louis, MO, USA). ALT, AST, BUN and creatinine kits were purchased from the Quimica Clinica Aplicada (Amposta, Tarragona, Spain). Sodium sulfate, sodium metabisulfite, Tween 80, percholic acid and 1,2-diaminobenzene (DAB) were purchased from Sigma-Aldrich (St. Louis, MO, USA).
Methods
Preparation of MG Chitosan Nanoparticles (MGCN)
A formulation of MGCN was prepared by dissolving 200 mg of chitosan in 1% glacial acetic acid aqueous solution for overnight on a magnetic stirrer as described earlier.Citation23 To this solution, 1 mL of MG 40% aqueous solution was added in a drop-wise manner to chitosan solution, followed by the addition of 1 mL of Tween-80. Subsequently, 500 µL of 20% sodium sulfate solution, 50 µL of 50% glutaraldehyde solution and 1 mL of 10% sodium metabisulfite were added to the above solution. After overnight incubation, the final reaction mixture was concentrated by Amicon filters. All components used in the preparation of nanoparticles were nonpyrogenic. Sham nanoparticles were prepared using the same procedure without MG and sodium sulfate.
Characterization of MGCN
Determination of Size and Polydispersity Index
Size of MGCN was measured by Malvern Nano zeta sizer (Malvern instruments, Southborough, Massachusetts), using dynamic light scattering technique.
Analysis of Functional Groups by Fourier Transforms Infrared Spectroscopy
FTIR was performed to analyze and compare the changes due to the conjugation of polymer with drug in the formation of nanoparticles. FTIR spectra of MGCN were recorded on the FIR spectrophotometer using a sensitive pyroelectric detector. For this purpose, the lyophilized pressed pellets were prepared by grinding the pellets with KBr in a mortar in a 1: 100 ratio.Citation28
Determination of Diameter and Shape
The size and shape of MGCN were determined by the Transmission electron microscopy (TEM). Samples were prepared by drop-casting samples on a carbon-coated copper grid. This grid was placed on a drop of staining agent for more than 5 s followed by drying. The images were recorded on the high-resolution TEM operating on 200 kV.Citation28
Surface Morphology
This was done with the Scanning electron microscopy (SEM). A small drop of the sample was placed on a clean coverslip and dried over for a day. The dried sample was coated with a gold sputter coater.Citation28
Determination of the Quantity of Methylglyoxal Associated with Nanoparticles
The quantity of MG associated with nanoparticles was calculated by plotting the standard curve and then determining the amount of MG in an unknown sample using the standard plot.Citation29,Citation30 Since MG does not have any aromatic ring for absorbance, we can use substrate for MG and subsequently plot standard curve for their product. About 200 µL of nanoparticle formulation was taken and treated with 0.5 M perchloric acid to release the entrapped MG. It was then treated with 1.8 mM 1,2-diaminobenzene (DAB) to form 2-methylquinoxaline, the spectra of which were recorded using a UV-Vis spectrophotometer between 200 and 400 nm after the addition of DAB. The maximum absorbance was recorded at 336 nm. The unknown concentration was calculated from the standard curve.
Test Strain
An isolate of C. albicans (KFH 121) was acquired from the Department of Microbiology, King Fahad Hospital, Buraydah, Saudi Arabia and was maintained on Sabouraud Dextrose Agar plates. The identification of C. albicans strain was confirmed in chromogenic media and by germ tube induction test in serum.
Determination of the Activity of MG and Fluconazole Against C. albicans
The antifungal activity of MG was determined by the agar well-diffusion method as described earlier.Citation31 Sabouraud dextrose agar (SDA) plates were prepared and spread with C. albicans. The wells (8 mm in diameter) were made and a 50 µL volume containing 12.5, 25 and 50 µg of MG was loaded in each well and incubated at 37ºC for 48 h. The anti-candida activity of MG was assessed by measuring the growth inhibition zone. The well containing equal volumes of saline was considered as a negative control.
Minimum inhibitory concentration (MIC) of MG and fluconazole was determined by the broth microdilution antifungal susceptibility method according to the guidelines of Clinical and Laboratory Standards Institute (CLSI), Wayne (NCLLS).Citation32 The final concentration of fluconazole and MG was taken in the range of 0.125 to 128 µg/mL. Antifungal susceptibility testing was done in 96-well round-bottom microtitration plates. The inoculum of C. albicans was prepared in 5% dextrose and was diluted in RPMI buffered with MOPS (pH 7.0) to give a final concentration of 5 × 103 cells per mL. The corning 96-well cell culture plates (Sigma-Aldrich, St. Louis, USA) were incubated at 37°C for 48 h. The optical density was measured at 530 nm (BIO-TEK EL310 Microplate Auto-Reader, Biotek instruments). The MIC was the lowest drug concentration that caused 99% growth inhibition as compared to growth control without drug.
Effect of Free MG or MGCN on Hyphae Formation in C. albicans
Candida albicans were incubated with human serum for 8 h at 37°C in the presence or absence of 1 and 2 µg/mL of fluconazole or MG or MGCN as described earlier.Citation33 After 8 h, a very small aliquot of cell suspension was spread on a slide to visualize the formation of hyphae under a microscope with ×100 magnification.
Efficacy of MGCN Against Intracellular Replication of C. albicans
J774 macrophages (ATCC® TIB-67™) were plated in triplicates in 24-well Costar plates (Sigma-Aldrich, St. Louis, USA) with 1 × 106 cells/well in DMEM (Sigma-Aldrich, St. Louis, USA) supplemented with 10% fetal bovine serum for 24 h. Macrophages were infected with C. albicans at the multiplicity of infection of 2 (MOI=2). After 6 h of infection, cells were washed with 0.1 M Phosphate-buffered saline (pH 7.4) to remove unphagocytosed C. albicans. Candida albicans infected macrophages were treated at the doses of 10 and 20 µM of fluconazole or free MG or MGCN. After 48 h, macrophages were treated with 1% Triton X-100 (Sigma-Aldrich, St. Louis, USA) in order to release phagocytized yeasts. Various dilutions of lysate were prepared in PBS and 100 µL of each aliquot was platted on SDA. CFUs were calculated by multiplying the numbers of colony-forming units (CFUs) by the dilution factor as described earlier.Citation34
Mice
Female BALB/C mice of 12 weeks were obtained from the animal house facility of the college of Applied Medical Sciences, Qassim University, Buraydah, Saudi Arabia. All experiments in the mice were performed after approval from the animal ethics committee of the College of Applied Medical Sciences, Qassim University, Buraydah, Saudi Arabia. An anesthesia was given by injecting a combination of ketamine and xylazine mixture (90 mg/kg + 10 mg/kg) through the intraperitoneal route as described earlier.Citation35
Infection Model
Each mouse was infected by injecting 7 × 105 CFUs of C. albicans through the lateral tail vein as described earlier.Citation36
Fluconazole Therapy of C. albicans Infected Mice
Treatment was started by injecting various doses of fluconazole on days 1, 3 and 5 post 24 h of C. albicans infection. Mice were divided into the following groups and each group contained 10 mice:
(1) Normal saline
(2) Fluconazole −5 mg/kg
(3) Fluconazole −10 mg/kg
(4) Fluconazole −20 mg/kg
Determination of Antifungal Activity of MG or MGCN
MG or MGCN at the doses of 1, 5 and 10 mg/kg were tested to assess the efficacy against C. albicans. A 40% aqueous stock solution of MG was diluted with sterile normal saline and administered in C. albicans infected mice through an intraperitoneal route. Mice were divided into eight groups:
(1) Normal saline
(2) Sham chitosan nanoparticles (SCN)
(3) MG −1 mg/kg
(4) MGCN-1 mg/kg
(5) MG −5 mg/kg
(6) MGCN-5 mg/kg
(7) MG −10 mg/kg
(8) MGCN-10 mg/kg.
Evaluation of the Severity of C. albicans Infection
The efficacy of fluconazole or MG or MGCN against the systemic infection of C. albicans was evaluated by the survival rate and fungal burden in the kidney tissues.Citation36 Mice in various groups were observed for 40 days post C. albicans infection. In order to determine the severity of C. albicans infection, three mice from each group were sacrificed on day 4 postinfection and the kidney was taken out and homogenized. The tissue homogenate was cultured on SDA plates to determine the colony-forming units.
Evaluation of Toxicity of Free MG or MGCN in Mice
MG or MGCN was injected in mice at the doses of 5, 10 and 20 mg/kg for 5 days. On day 6, the blood was taken from three mice of each group to estimate the liver and kidney toxicity parameters, including Aspartate transaminase (AST), alanine transaminase (ALT), blood urea nitrogen (BUN) and creatinine as described earlier.Citation37
Statistical Analyses
Survival data are shown by the Kaplan–Meier curve and analyzed by the Log-rank Chi-square test. The fungal burden data were analyzed by one-way ANOVA followed by a Bonferroni post-test using GraphPad Prism software, version 6.0 (La Jolla, CA, USA).
Results
Physical Characterization of Nanoparticles
Size, Polydispersity Index and Analysis of the Functional Group
The diameter of MGCN was measured by the dynamic light scattering (DLS) technique. This technique not only reveals the diameter, but also polydispersity index (PDI) of nanoparticles. It indicates the homogeneity of particles with the value between 0 and 1. Lower values indicate high homogeneity or more uniformity. The stability of the system is related to its PDI value, not by the zeta potential. The particles with higher PDI value have lower stability, whereas those with lower PDI value has high stability. The size of nanoparticles was in the range of 50–100 nm and PDI values were 0.2 to 0.4 as measured by DLS ().
Figure 1 Physical characterization of chitosan nanoparticles. Zeta potential of (A) Sham chitosan nanoparticles and (B) Methylglyoxal-conjugated chitosan nanoparticles (MGCN). Fourier Transform Infrared Spectra of (C) Sham chitosan nanoparticles and (D) Arrow indicates the formation of imine bond in MGCN (Nano-MG).
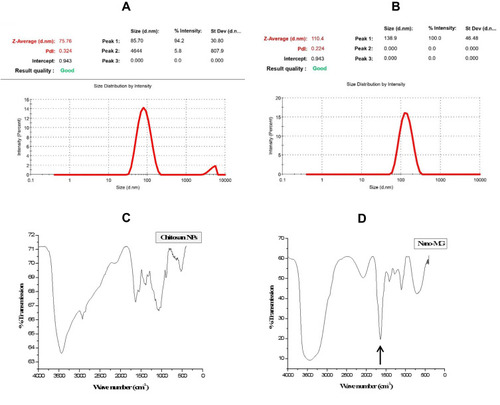
Fourier Transform Infrared Spectroscopy (FTIR) is performed to examine the formation and breaking of the functional groups. Chitosan has a free primary NH2 group present on its ring, whereas MG is a carbonyl compound. When chitosan interacts with MG, the formation of Schiff base takes place between primary -NH2 group of chitosan and ˃C=O group of MG. This Schiff`s base is an imine compound containing carbon nitrogen double bond. An imine bond is formed in the formation of MGCN, which gives FTIR peak in their characteristic C=N stretching range 1690–1640 cm−1 as indicated by an arrow ().
Morphological Characterization of Nanoparticles by TEM and SEM
The transmission electron microscopy (TEM) confirmed the size distribution of MGCN as obtained by DLS. Although the size of MGCN was in a range of 50–100 nm, but the average size was approximately 70 nm (). TEM also confirmed the typical spherical shape of nanoparticles. The size distribution of MGCN through SEM reveals that the surface is smooth with an increase in size due to agglomeration and gold coating ().
The Amount of MG Associated with Chitosan Nanoparticles
Chitosan associated MG (µM) = 2417.22 ± 61.3426 per mL of nanoparticle formulation. This is 2417.22 µM MG or 2.4 mM of MG in 1 mL.
Molarity = Weight/Molecular weight
Weight =Molarity × Molecular mass
=2.4 mM × 72 = 172 µg of MG per 1 mL
So, the final concentration was 172 µg of MG per 1 mL of formulation.
C. albicans Showed in vitro Susceptibility to Methylglyoxal
The agar well diffusion and the dilution methods were used to determine the susceptibility of C. albicans to MG. MG demonstrated remarkable activity against C. albicans by the agar well diffusion and dilution methods. A negative control well loaded with vehicle did not show any growth inhibition (), whereas MG at the concentrations of 12.5, 25, 50 µg/mL exhibited the inhibition zones of 19, 30 and 35 mm, respectively ().
Figure 3 Anti-Candida activity of MG by agar well diffusion method. (A) Vehicle control, (B) 12.5 µg, (C) 25 µg and (D) 50 µg of MG.
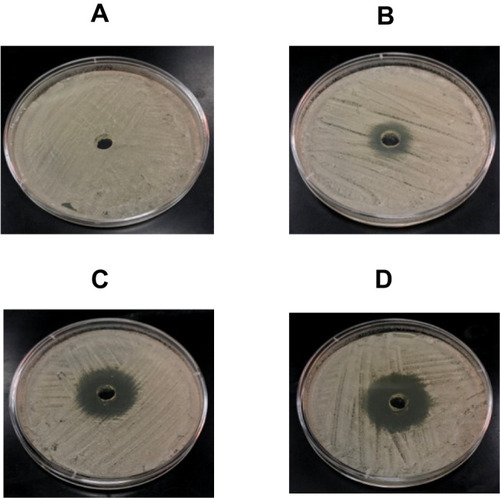
The antifungal activity of fluconazole and MG was also determined by the dilution method as described earlier.Citation31 Candida albicans with MIC value >64 µg/mL of fluconazole has been categorized as a resistant strain according to the CLSI guidelines. The MIC of fluconazole for the present isolate of C. albicans (KFHR 098) was found to be >64 µg/mL, whereas the MIC value of MG was 32 µg/mL.
Methylglyoxal, Not Fluconazole, Inhibits the Hyphae Formation
The effect of lower doses of fluconazole or MG or MGCN was assessed on the transition of C. albicans yeast form into hyphae. C. albicans showed an extensive hyphae formation in fetal bovine serum (FBS) in the control group without any drug (). Fluconazole at the concentrations of 1 and 2 µg/mL did not inhibit the hyphae formation (). Free MG or MGCN considerably inhibited the hyphae formation in C. albicans at the same concentrations ().
MGCN Effectively Inhibits the Intracellular Replication of C. albicans
Candida albicans has the ability to multiply intracellularly as shown by the proliferation of C. albicans in macrophages after 24 h of infection. The numbers of CFUs in C. albicans-infected macrophages were found to be 55,402 ± 5875 in the untreated macrophages (). Treatment with fluconazole at the concentrations of 10 and 20 µM was ineffective to reduce the intracellular multiplication of C. albicans in the macrophages (). The CFUs were found to be 53,344 ± 3061 and 52,075 ± 3699 in C. albicans infected macrophages treated with fluconazole at the doses of 10 and 20 µM, respectively (). Treatment with free MG at the doses of 10 and 20 µM reduced the fungal load to 13,453 ± 2933 and 7281 ± 2785 CFUs in macrophages and was significantly reduced as compared to fungal load in the untreated or fluconazole-treated macrophages (P<0.001). Candida albicans infected macrophages treated with MGCN at the doses of 10 and 20 µM exhibited the lowest CFUs of 5142 ± 1432 and 92 ± 76, which was significantly lowered as compared to CFUs in the untreated or fluconazole-treated macrophages (P<0.001). This shows that chitosan nanoparticles effectively delivered MG to intracellular locations in the macrophages.
Figure 5 Macrophages (1 × 106 cells/well) were infected with C. albicans at multiplicity of infection of 2 (MOI=2) followed by treatment with fluconazole or free MG or MGCN at the concentrations of 10 and 20 µM. After 48 h, the cells were lysed and intracellular yeasts were plated on Saboraud dextrose agar plates to determine the number of CFUs. Data are expressed as mean ± SE. A P value <0.05 was considered to be significant.
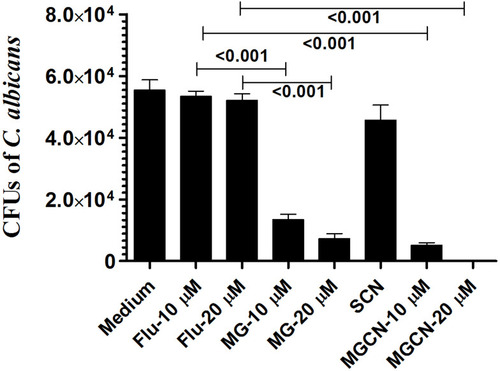
Fluconazole Treatment Was Not Effective Against C. albicans
The antifungal activity of fluconazole at the doses of 5, 10 and 20 mg/kg was assessed against murine candidiasis. The results of the in vivo study correlated well to in vitro findings. Mice did not respond to fluconazole and all infected mice died before day 40 post-infection (). Median survival time of mice in saline-treated group was found to be 4.5 days, whereas the median survival time of mice in the groups treated with fluconazole at the doses of 5, 10 and 20 mg/kg was found to be 5, 8 and 14 days, respectively (). The median survival time of mice in the group treated with fluconazole at a dose of 20 mg/kg was found to be significantly higher as compared to that of mice in saline-treated group (P =0.0019).
Figure 6 Fluconazole did not show antifungal activity against C. albicans. (A) Each mouse was infected with 7 x 105 CFUs of C. albicans. Mice were treated with 5, 10, 20 mg/kg of fluconazole on days 1, 3 and 5 postinfection. Mice were observed for 40 days to monitor their survival. Saline (●), fluconazole (5 mg/kg) (○), fluconazole (10 mg/kg) (♦), fluconazole (20 mg/kg) (♦). (B) On day 4, three mice from each group were sacrificed and their kidney was taken to prepare tissue homogeninate. The kidney tissue homogenate was cultured in order to determine the fungal load. Data are expressed as mean ± SE. A P value <0.05 was considered to be significant.
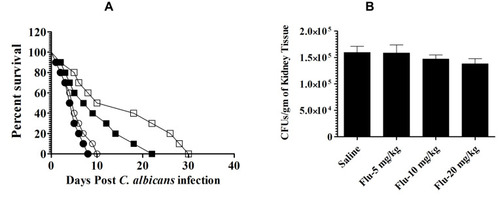
The severity of infection in kidney tissues revealed the highest fungal burden of 159,596 ± 19,869 CFUs per gram of kidney tissue in mice from saline-treated group (). Whereas, the mice in the groups treated with fluconazole at the doses of 5, 10 and 20 mg/kg exhibited the fungal burden of 158,591 ± 26,487, 147,103 ± 18,067 and 138,102 ±16,783 CFUs per gram of kidney, which were found to be insignificant as compared to CFUs in saline-treated mice (P>0.05).
MGCN Eliminates Fluconazole-Resistant Systemic Candidiasis
Free MG or MGCN at the doses of 1, 5 and 10 mg/kg were tested against C. albicans infection in mice. Free MG and MGCN showed antifungal activity against an isolate of C. albicans not responding to fluconazole therapy. Incorporation in chitosan nanoparticles increased the antifungal activity of MG. Candida albicans infected mice treated with MGCN at a dose of 10 mg/kg showed a 90% survival rate, whereas the mice treated with free MG at the same dose showed a 50% survival rate on day 40 (P = 0.0258) (). Mice treated at a dose of 5 mg/kg of MGCN showed a 60% survival rate, whereas the mice treated with free MG at the same dose showed a 20% survival rate (P = 0.0298) (). Treatment with SCN increased the median survival time of C. albicans infected mice to 15 days as compared to 5.5 days in saline-treated mice (P=0.0064).
Figure 7 Treatment with MGCN showed the highest antifungal activity against C. albicans. (A) Mice infected with C. albicans (7 × 105 CFUs/mouse) were treated with 1, 5 and 10 mg/kg of MG or MGCN on days 1, 3 and 5 postinfection. Mice were observed for 40 days to monitor their survival. Saline (○), SCN (●), Free MG-1 mg/kg (∆), MGCN-1 mg/kg (▲), Free MG-5 mg/kg (♦), MGCN-5 mg/kg (♦), Free MG-10 mg/kg (□), MGCN-10 mg/kg (■). Saline vs SCN (P=0.0064), Free MG-1 mg/kg vs MGCN-1 mg/kg (P=0.0284), Free MG-5 mg/kg vs MGCN-5 mg/kg (P=0.0298), Free MG-10 mg/kg vs MGCN-10 mg/kg (P=0.0258). (B) On day 4, three mice from each group were sacrificed and their kidney was taken to make tissue homogenate. The kidney tissue homogenate was cultured to determine the fungal load. A P value <0.05 was considered to be significant. *** (P<0.001).
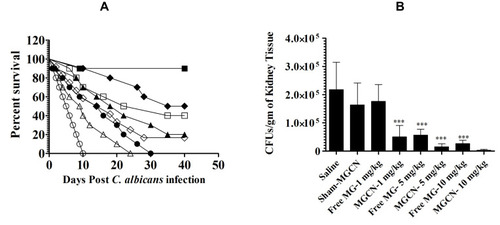
The severity of infection was assessed by measuring the fungal load in the kidney tissues of mice untreated or treated with free MG or MGCN. Mice in the group treated at a dose of 10 mg/kg of MGCN exhibited the greatest reduction to 3175 ± 1281 CFUs as compared to 218,217 ± 38,919 CFUs in the saline-treated group (P<0.001) (). The fungal load of 15,578 ± 4145 CFUs in the kidney tissue of the mice treated at a dose of 5 mg/kg of MGCN was lower to 26,665 ± 4782 CFUs in the mice treated with free MG at a dose of 10 mg/kg ().
Free MG or MGCN Did Not Induce Any Toxicity in Mice
To evaluate whether the administration of MG or MGCN is associated with any toxicity, the hepatic and renal toxicity parameters were analyzed in the treated mice. The administration of MG or MGCN did not induce any hepatic toxicity as shown by the levels of AST and ALT (). Similarly, there was no significant change in the levels of BUN and creatinine in mice treated with MG or MGCN at the doses of 10 and 20 mg/kg (). These results suggest that MG or MGCN is completely safe at the doses used in the present study.
Figure 8 Administration of free MG or MGCN did not induce any toxicity. Mice were injected with MG or MGCN at the doses of 5, 10 and 20 mg/kg for consecutive 5 days. On day 6 postinjection, the blood was taken from three mice of each group to estimate the levels of (A) ALT, (B) AST, (C) BUN and (D) creatinine. Data are expressed as mean ± SE.
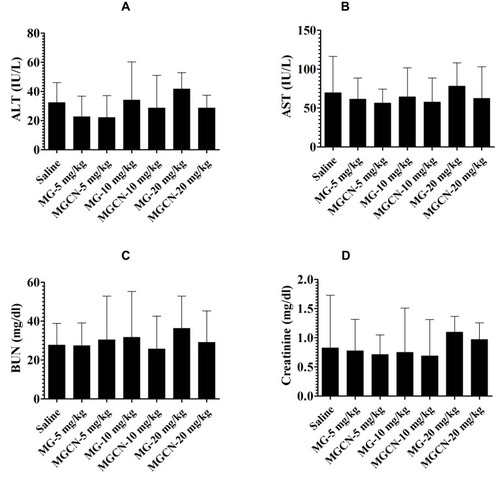
Discussion
Fluconazole is one of the most commonly used antifungal drug to treat fungal infections and its extensive use has resulted in the emergence of drug-resistant fungal infections, including Candida spp.Citation4,Citation5 Multi-drug resistance genes like MDR1, CDR1 or CDR2 or ERG11 are overexpressed in fluconazole-resistant Candida isolates.Citation6,Citation8,Citation38 The findings of this study showed that fluconazole exhibited higher MIC value (>64 mg/L) against the present isolate of C. albicans. To examine whether in vitro findings correlate to in vivo results, the efficacy of fluconazole against C. albicans was assessed in a murine model. The findings of in vivo study were in accordance with in vitro results. Candida albicans infected mice did not respond to fluconazole even at a dose of 20 mg/kg. The survival data were strengthened by the fungal load data in kidney tissues. Candida albicans infected mice treated with fluconazole showed substantially higher fungal load in their kidney.
Due to limited numbers of available current antifungal drugs, there is a critical need to find new antifungal agents to treat C. albicans infection. MG has been shown to possess the antimicrobial activity against Pseudomonas aeruginosa, Staphylococcus aureus, Enterococcus faecalis and Salmonella typhi.Citation39,Citation40 Moreover, MG and methylglyoxal-bis-guanylhydrazone have shown inhibitory effects against HIV and influenza virus.Citation41,Citation42 None of the earlier studies have shown the effect of MG against fungal pathogens. In the present study, we determined the activity of MG and MGCN against C. albicans infection. Conjugation of MG to chitosan nanoparticles resulted in the increased activity of MG.Citation29,Citation30
Candida albicans shows an ability to grow as unicellular budding yeast cell and filamentous hyphae form. The hyphae form is considered a virulent factor as compared to the yeast form of C. albicans. The immune cells respond differently to the yeast and hyphae forms in relation to the secretion of cytokines. For example, yeast cells of C. albicans induce dendritic cells to secrete IL-12, whereas hyphae form induces the secretion of IL-4.Citation43 Moreover, hyphae did not induce IFN-γ secretion because hyphae show short of recognition by TLR4.Citation44 Thus, the hyphae form of C. albicans has the ability to evade the Th1 immune response. A therapeutic agent that is capable of inhibiting the transition of yeast C. albicans into the hyphae may thwart the immune evasion strategy of C. albicans. The findings of the current study demonstrated that MG or MGCN, not fluconazole, inhibited the transition of C. albicans yeast into hyphae at lower doses. Thus, MG formulations can effectively reduce the virulence of C. albicans. Interestingly, chitosan itself inhibits the growth of important fungal pathogens, including A. fumigatus, C. albicans and C. neoformans.Citation45 Thus, the formulations of chitosan nanoparticles-loaded antifungal drugs may have additional effectiveness against fungal infections. This is also evident from the results that showed a greater survival rate of mice in the group treated with sham chitosan nanoparticles.
Macrophages are an important part of the innate immune response to C. albicans infection.Citation46 Candida albicans adopts various strategies to evade macrophage-mediated onslaught in order to replicate within macrophages.Citation47,Citation48 The conversion of C. albicans from the yeast to hyphae is critical to sabotage the microbicidal action of the phagosome. In addition, C. albicans produces ammonia to alkalinize the acidic environment of phagosomes to facilitate the yeast to hyphae transition.Citation49 Because MG inhibits the transition of the yeast to hyphae, treatment with MG or MGCN can reduce the ability of C. albicans to avoid the onslaught of macrophages. Moreover, MG activates the macrophages to secrete TNF-α and IFN-γ that have a protective role against tumor and infectious diseases.Citation50 The results of the present study demonstrated the macrophage-activating property of MG because MG-treated macrophages exhibited the lowest intracellular fungal burden.
Because of the recent emergence of azole-resistant fungal isolates and toxic manifestations of antifungal drugs, it is important to find new antifungals that show increased efficacy with decreased toxicity. The finding of the present study showed that MGCN has strong antifungal efficacy. Interestingly, C. albicans infected mice treated with sham chitosan nanoparticles significantly increased the survival rate as compared to the saline-treated mice (P=0.0064). MGCN at a dose of 10 mg/kg was highly effective and mice in this group exhibited a 90% survival rate as compared to 50% survival rate of mice treated with free MG at the same dose (P=0.0258). Ironically, the most effective polyene antifungal drugs have shown considerable renal and hepatic toxicity in the treated subjects.Citation9,Citation26 Importantly, administration of MG or MGCN was found to be safe, and treated mice did not reveal any substantial changes in liver inflammation markers such as ALT and AST and renal function parameters such as BUN and creatinine.
In conclusion, MGCN was prepared in this study through the conjugation of the aldehyde group of MG to free amino group of chitosan. Free MG or MGCN showed antifungal activity against an isolate of C. albicans that did not respond to fluconazole therapy. Chitosan also shows an antifungal activity against C. albicans. MGCN demonstrated a synergistic antifungal effect of MG and chitosan against C. albicans both in vitro and in a mouse model. Moreover, a combination therapy of MGCN with antifungal drugs such as fluconazole, itraconazole and amphotericin B may be considered to treat drug-resistant fungal pathogens. The results of the current study revealed that MGCN showed superior anti-Candida activity as compared to free MG at the respective doses. Owing to its ability to inhibit yeast to hyphae transition, intracellular killing, the enhanced in vivo efficacy and reduced toxicity, MGCN may be proved a very potential chemotherapeutic agent to treat C. albicans infection.
Acknowledgment
This work is not funded by any research support agency. We thank the Deanship of Scientific Research, Qassim University for the help and support.
Disclosure
The authors report no conflicts of interest in this work.
References
- Fidel PL Jr. Candida-host interactions in HIV disease: implications for oropharyngeal candidiasis. Adv Dent Res. 2011;23:45–49. doi:10.1177/002203451139928421441480
- Giri S, Kindo AJ. A review of Candida species causing blood stream infections. Indian J Med Microbiol. 2012;30:270–278. doi:10.4103/0255-0857.9948422885191
- Silveria FP, Husain S. Fungal infections in solid organ transplants. Med Mycol. 2007;45:305–320. doi:10.1080/1369378070120037217510855
- Morschhauser J. The genetic basis of fluconazole resistance development in Candida albicans.. Biochem Biophys Acta. 2002;1557:240–248.
- Masia Canuto M, Gutirrez Rodero F. Antifungal drug resistance to azoles and polyenes. Lancet Infect Dis. 2002;2:550–563. doi:10.1016/S1473-3099(02)00371-712206971
- Lee MK, Williams LE, Warnock DW, et al. Drug resistance genes and trailing growth in Candida albicans isolates. J Antimicrob Chemother. 2004;53:217–224. doi:10.1093/jac/dkh04014688046
- Jiang C, Dong D, Yu B, et al. Mechanisms of azole resistance in 52 clinical isolates of Candida tropicalis in China. J Antimicrob Chemother. 2013;68:778–785. doi:10.1093/jac/dks48123221625
- Duppont B. Overview of lipid formulations of amphotericin B. J Antimicrob Chemother. 2002;49(Suppl 1):31–36. doi:10.1093/jac/49.suppl_1.31
- Khan MA, Owais M. Toxicity, stability and pharmacokinetics of amphotericin B in immunomodulator tuftsin-bearing liposomes in a murine model. J Antimicrob Chemother. 2006;58:125–132. doi:10.1093/jac/dkl17716709592
- Gazendam RP, van Hamme JL, Tool AT, et al. Two independent killing mechanisms of Candida albicans by human neutrophils: evidence from innate immunity defects. Blood. 2014;124(4):590–597. doi:10.1182/blood-2014-01-55147324948657
- Khan MA, Khan A, Owais M. Prophylactic use of liposomized tuftsin enhances the susceptibility of Candida albicans to fluconazole in leukopenic mice. FEMS Immunol Med Microbiol. 2006;46(1):63–69. doi:10.1111/j.1574-695X.2005.00014.x16420598
- Qian Q, Jutila MA, Van Rooijen N, Cutler JE. Elimination of mouse splenic macrophages correlates with increased susceptibility to experimental disseminated candidiasis. J Immunol. 1994;152(10):5000–5008.8176217
- Crowe SM, Vardaxis NJ, Kent SJ, et al. HIV infection of monocyte-derived macrophages in vitro reduces phagocytosis of Candida albicans. J Leukocyte Biol. 1994;56:318–327. doi:10.1002/jlb.56.3.3188083603
- de Repentigny L, Lewandowski D, Jolicoeur P. Immunopathogenesis of oropharyngeal candidiasis in human immunodeficiency virus infection. Clin Microbiol Rev. 2004;17(4):729–759. doi:10.1128/CMR.17.4.729-759.200415489345
- Talukdar D, Ray S, Ray M, Das S. A brief critical overview of the biological effects of methylglyoxal and further evaluation of a methylglyoxal-based anticancer formulation in treating cancer patients. Drug Metabol Drug Interact. 2008;23:175–210. doi:10.1515/DMDI.2008.23.1-2.17518533369
- Kilty SJ, Duval M, Chan FT, Ferris W, Slinger R. Methylglyoxal: (active agent of Manuka honey) in vitro activity against bacterial biofilms. Int Forum Allergy Rhinol. 2011;1:348–350. doi:10.1002/alr.2007322287464
- Hayes G, Wright N, Gardner SL, Telzrow CL, Wommack AJ, Vigueira PA. Manuka honey and methylglyoxal increase the sensitivity of Staphylococcus aureus to linezolid. Lett Appl Microbiol. 2018;66(6):491–495. doi:10.1111/lam.1288029575121
- Oliveira A, Ribeiro HG, Silva AC, et al. Synergistic antimicrobial interaction between honey and phage against Escherichia coli biofilms. Front Microbiol. 2017;8:2407. doi:10.3389/fmicb.2017.0240729276503
- Pavlovic-Djuranovic S, Kum JF, Schultz JE, Beitz E. Dihydroxyacetone and methylglyoxal as permeants of the Plasmodium aquaglyceroporin inhibit parasite proliferation. Biochem Biophys Acta. 2006;1758:1012–1017. doi:10.1016/j.bbamem.2005.12.00216427024
- Kong M, Chen XG, Xing K, Park HJ. Antimicrobial properties of chitosan and mode of action: a state of the art review. Int J Food Microbiol. 2010;144(1):51–63. doi:10.1016/j.ijfoodmicro.2010.09.01220951455
- Peña A, Sánchez NS, Calahorra M. Effects of chitosan on Candida albicans: conditions for its antifungal activity. Biomed Res Int. 2013;2013:527549. doi:10.1155/2013/52754923844364
- Hosseinnejad M, Jafari SM. Evaluation of different factors affecting antimicrobial properties of chitosan. Int J Biol Macromol. 2016;85:467–475. doi:10.1016/j.ijbiomac.2016.01.02226780706
- Raafat D, von Bargen K, Haas A, Sahl HG. Insights into the mode of action of chitosan as an antibacterial compound. Appl Environ Microbiol. 2008;74(12):3764–3773. doi:10.1128/AEM.00453-0818456858
- Shih PY, Liao YT, Tseng YK, Deng FS, Lin CH. A potential antifungal effect of chitosan against Candida albicans is mediated via the inhibition of SAGA complex component expression and the subsequent alteration of cell surface integrity. Front Microbiol. 2019;10:602. doi:10.3389/fmicb.2019.0060230972050
- Khan MA, Nasti TH, Saima K, et al. Co-administration of immunomodulator tuftsin and liposomised nystatin can combat less susceptible Candida albicans infection in temporarily neutropenic mice. FEMS Immunol Med Microbiol. 2004;41(3):249–258. doi:10.1016/j.femsim.2004.03.01115196575
- Khan MA, Faisal SM, Mohammad O. Safety, efficacy and pharmacokinetics of tuftsin-loaded nystatin liposomes in murine model. J Drug Target. 2006;14(4):233–241. doi:10.1080/1061186060072038416777682
- Khan MA, Nasti TH, Owais M. Incorporation of amphotericin B in tuftsin-bearing liposomes showed enhanced efficacy against systemic cryptococcosis in leucopenic mice. J Antimicrob Chemother. 2005;56(4):726–731. doi:10.1093/jac/dki30716126780
- Yang W, Fu J, Wang T, He N. Chitosan/sodium tripolyphosphate nanoparticles: preparation, characterization and application as drug carrier. J Biomed Nanotechnol. 2009;5(5):591–595. doi:10.1166/jbn.2009.106720201437
- Pal A, Talukdar D, Roy A, et al. Nanofabrication of methylglyoxal with chitosan biopolymer: a potential tool for enhancement of its anticancer effect. Int J Nanomedicine. 2015;10:3499–3518. doi:10.2147/IJN.S7828425999714
- Chakrabarti A, Talukdar D, Pal A, Ray M. Immunomodulation of macrophages by methylglyoxal conjugated with chitosan nanoparticles against Sarcoma-180 tumor in mice. Cell Immunol. 2014;287(1):27–35. doi:10.1016/j.cellimm.2013.11.00624368179
- Alrumaihi F, Allemailem KS, Almatroudi A, Alsahli MA, Khan A, Khan MA. Tinospora cordifolia aqueous extract alleviates cyclophosphamide-induced immune suppression, toxicity and systemic candidiasis in immunosuppressed mice: in vivo study in comparison to antifungal drug fluconazole. Curr Pharm Biotechnol. 2019;20(12):1055–1063. doi:10.2174/138920101966619072215112631333126
- Clinical and Laboratory Standards Institute. Reference Method for Broth Dilution Antifungal Susceptibility Testing of Yeasts; Approved Standard-Third Edition; CLSI Document M27-A3. Wayne, PA, USA: CLSI, Clinical and Laboratory Standards Institute; 2008a.
- Matare T, Nziramasanga P, Gwanzura L, Robertson V. Experimental germ tube induction in candida albicans: an evaluation of the effect of sodium bicarbonate on morphogenesis and comparison with pooled human serum. Biomed Res Int. 2017;2017:1976273. doi:10.1155/2017/197627328656137
- Khan MA, Jabeen R, Nasti TH, Owais M. The enhanced anticryptococcal activity of chloroquine in Phosphatidylserine-containing liposomes in a murine model. J Antimicrob Chemother. 2005;55:223–228. doi:10.1093/jac/dkh52215590713
- Khan MA, Aldebasi YH, Alsuhaibani SA, et al. Therapeutic potential of thymoquinone liposomes against the systemic infection of Candida albicans in diabetic mice. PLoS One. 2018;13(12):e0208951. doi:10.1371/journal.pone.020895130589842
- Khan MA, Aljarbou AN, Khan A, Younus H. Liposomal thymoquinone effectively combats fluconazole-resistant Candida albicans in a murine model. Int J Biol Macromol. 2015;76:203–208. doi:10.1016/j.ijbiomac.2015.02.01525709021
- Laskar AA, Khan MA, Rahmani AH, Fatima S, Younus H. Thymoquinone, an active constituent of Nigella sativa seeds, binds with bilirubin and protects mice from hyperbilirubinemia and cyclophosphamide-induced toxicity. Biochimie. 2016;127:205–213. doi:10.1016/j.biochi.2016.05.02027265787
- Rodrigues CF, Rodrigues ME, Silva S, Henriques M. Candida glabrata biofilms: how far have we come? J Fungi (Basel). 2017;3:1.
- Afzal RK, Khalid F, Hannan A, Ahmed SA. Methylglyoxal: antimicrobial activity against blood culture isolates of Salmonella Typhi and other Gram negative rods. Pak J Med Sci. 2019;35(4):1110–1114.31372152
- Bulman SEL, Tronci G, Goswami P, Carr C, Russell SJ. Antibacterial properties of nonwoven wound dressings coated with manuka honey or methylglyoxal. Materials (Basel). 2017;16:(8)10.
- Charyasriwong S, Haruyama T, Kobayashi N. In vitro evaluation of the antiviral activity of methylglyoxal against influenza B infection. Drug Discov Ther. 2016;10(4):201–210. doi:10.5582/ddt.2016.0104527558282
- Jin X, McGrath MS, Xu H. Inhibition of HIV expression and integration in macrophages by methylglyoxal-bis-guanylhydrazone. J Virol. 2015;89(22):11176–11189. doi:10.1128/JVI.01692-1526223636
- d’Ostiani CF, Del Sero G, Bacci A, et al. Dendritic cells discriminate between yeasts and hyphae of the fungus Candida albicans. Implications for initiation of T helper cell immunity in vitro and in vivo. J Exp Med. 2000;191(10):1661–1674. doi:10.1084/jem.191.10.166110811860
- van der Graaf CA, Netea MG, Verschueren I, van der Meer JW, Kullberg BJ. Differential cytokine production and Toll-like receptor signaling pathways by Candida albicans blastoconidia and hyphae. Infect Immun. 2005;73(11):7458–7464. doi:10.1128/IAI.73.11.7458-7464.200516239547
- Lopez-Moya F, Suarez-Fernandez M, Lopez-Llorca LV. Molecular mechanisms of Chitosan interactions with fungi and plants. Int J Mol Sci. 2019;20(2):E332. doi:10.3390/ijms2002033230650540
- Ashman RB. Protective and pathologic immune responses against Candida albicans infections. Front Biosci. 2008;13:3334–3351. doi:10.2741/292918508436
- Jiménez-López C, Lorenz MC. Fungal immune evasion in a model host-pathogen interaction: candida albicans versus macrophages. PLoS Pathog. 2013;9:e1003741. doi:10.1371/journal.ppat.100374124278014
- Frohner IE, Bourgeois C, Yatsyk K, Majer O, Kuchler K. Candida albicans cell surface superoxide dismutases degrade host-derived reactive oxygen species to escape innate immune surveillance. Mol Microbiol. 2009;71:240–252. doi:10.1111/j.1365-2958.2008.06528.x19019164
- Westman J, Moran G, Mogavero S, Hube B, Grinstein S. Candida albicans hyphal expansion causes phagosomal membrane damage and luminal alkalinization. MBio. 2018;11(5):9.
- Bhattacharyya N, Pal A, Patra S, Haldar AK, Roy S, Ray M. Activation of macrophages and lymphocytes by methylglyoxal against tumor cells in the host. Int Immunopharmacol. 2008;8(11):1503–1512. doi:10.1016/j.intimp.2008.06.00518617020