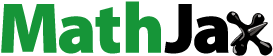
Abstract
Aim
In this study, the effects of cigarette smoking on maximal aerobic capacity, anaerobic capacity, and heart rate variability among female university students were investigated.
Materials and methods
Twelve smokers and 21 nonsmokers participated in this study. All participants performed an intermittent sprint test (IST) and a 20 m shuttle run test to measure their anaerobic capacity and maximal aerobic capacity. The IST was comprised of 6 × 10-second sprints with a 60-second active recovery between each sprint. Heart rate variability was recorded while the participants were in a supine position 20 minutes before and 30 minutes after the IST.
Results
The total work, peak power, and heart rate of the smokers and nonsmokers did not differ significantly. However, the smokers’ average power declined significantly during sprints 4 to 6 (smokers versus nonsmokers, respectively: 95% confidence interval =6.2–7.2 joule/kg versus 6.8–7.6 joule/kg; P<0.05), and their fatigue index increased (smokers versus nonsmokers, respectively: 35.8% ± 2.3% versus 24.5% ± 1.76%; P<0.05) during the IST. The maximal oxygen uptake of nonsmokers was significantly higher than that of the smokers (P<0.05). The standard deviation of the normal to normal intervals and the root mean square successive difference did not differ significantly between nonsmokers and smokers. However, the nonsmokers exhibited a significantly higher normalized high frequency (HF), and significantly lower normalized low frequency (LF), LF/HF ratio, and natural logarithm of the LF/HF when compared with those of the smokers (P<0.05).
Conclusion
Smoking may increase female smokers’ exercise fatigue and decrease their average performance during an IST, while reducing their maximal aerobic capacity. Furthermore, smoking reduces parasympathetic nerve activity and activates sympathetic cardiac control.
Introduction
Cigarette smoking has long been recognized as a critical topic in public health, and it has been increasing in many developing countries around the world. With social developments and greater rationalization of tobacco market transactions worldwide, the population of female smokers has increased.Citation1,Citation2 Additionally, students with peer pressure-driven smoking habits have attracted substantial attention.Citation3 Previous studies have verified the detrimental effects that smoking exerts on the human body, including the development of coronary artery disease, peripheral vascular disease, myocardial infarction, stroke, and sudden death, as well as exposure to numerous other complex risk factors.Citation4,Citation5 Tobacco contains over 44 harmful chemical compounds, such as nicotine, tar, and carbon monoxide. Nicotine stimulates the sympathetic nervous system, which can lead to increased levels of catecholamine, thereby increasing a person’s heart rate and stroke volume.Citation6 The tar produced by the burning of tobacco can increase pulmonary airway resistance or reduce the contact surface area between oxygen and pulmonary capillaries, thereby decreasing the capacity of the arteries to transport oxygenated blood during exercise.Citation7 Carbon monoxide is the byproduct of incomplete carbon combustion, the excessive inhalation of which increases carbon monoxide concentrations in the airways and the blood. Carbon monoxide’s hemoglobin-binding affinity is over 200 times that of oxygen. Increased levels of carbon monoxide in the bloodstream can limit the amount of oxygen transported in muscular capillaries, which adversely affects skeletal muscle performance.Citation8
To understand the link between smoking and cardiovascular disease, previous studies have employed noninvasive methods to investigate heart rate variability (HRV).Citation9–Citation17 HRV refers to the continuous effects on each heartbeat driven by the sympathetic nervous system and parasympathetic nervous system of the autonomic nervous system, and can be employed for the noninvasive observation of any sympathetic nervous system and parasympathetic nervous system changes.Citation18,Citation19 Currently, HRV is recognized as an effective and noninvasive tool for evaluating autonomic nervous system regulations of the heart, as they can be measured using time-domain analysis and frequency-domain analysis. These two methods can quantify the R–R interval between two adjacent normal heartbeats on an electrocardiogram. According to research conducted by the Task Force of the European Society of Cardiology and the North American Society of Pacing and Electrophysiology,Citation18 in a time-domain analysis, higher values of the standard deviation of the normal to normal intervals (SDNN), root mean square successive difference (RMSSD), and percentage of adjacent normal R–R intervals >50 ms (pNN50) indicate stronger parasympathetic dominance. Among the frequency-domain measurement indicators, a high frequency (HF) denotes parasympathetic activity, and a low frequency (LF) is related to the activity of both the sympathetic nervous system and the parasympathetic nervous system.
Studies have shown that smoking increases the risk of sudden death in women by up to fivefold; the influence that smoking exerts on sympathetic activity is believed to be among the main causes of this increase.Citation20 Smokers and people exposed to secondhand smoke may experience reduced HRV, which indicates an increased risk of heart damage,Citation16 and may be accompanied by increased blood pressure.Citation15 Certain studies have reported that a smoking group in a resting state exhibited significantly lower SDNN, RMSSD, and HF values, and a significantly higher mean heart rate and LF/HF ratio, compared with a control group.Citation10,Citation21 These results clearly show that smoking impairs the sympathovagal balance, weakens the regulatory capabilities of the cardiac vagus nerve, and increases sympathetic nervous system stimulation. Hering et alCitation22 indicated that female smokers had greater smoking-related increases in systolic blood pressure variability and greater decreases in R–R variability compared with male smokers. These findings may suggest that female smokers are vulnerable to acute cardiovascular events. Additionally, cardiac function changes quickly during the immediate recovery after exercise. An increase in parasympathetic activity and a decrease in sympathetic activity are known to occur after exercise cessation,Citation23 and the increased intensity of endurance exercise results in the slower recovery of HF and lower levels of HF in healthy women.Citation24,Citation25 However, few studies have investigated the association between female smokers, HRV, and physiologic parameters during the early recovery phase following anaerobic and intermittent sprint exercises; therefore, the dynamics of HRV during the immediate recovery after anaerobic, intermittent sprint exercise remain unclear.
Human metabolism consists of three major types of energy-supplying pathways: an immediate contribution from the breakdown of phosphatecreatine (PCr) and adenosine triphosphate (ATP) stores; a short-term source from the anaerobic breakdown of glycogen and glucose; and a long-lasting supply from aerobic mechanisms. The anaerobic energy contribution consists of PCr and ATP stores, and the processes of the Embden–Meyerhof pathway. During intense anaerobic activity, a steady state of exercise cannot be attained in the same manner as with aerobic activity. Intense and intermittent exercises with shorter recovery are equal to anaerobic exercises, such as basketball, football, hockey, and cycling, or any alternative exercises that require intermittent sprinting capabilities. The average heart rate for these types of intermittent exercises is approximately 70%–90% of the maximum heart rate, and oxygen consumption is approximately 60%–75% of the maximal oxygen uptake (VO2max).Citation26 Most of the literature regarding smoking and exercise emphasize the relationship between smoking and aerobic capacity and maximal oxygen uptake,Citation5 but few studies have explored the effects of smoking on anaerobic capacity and HRV during the early recovery stage in female smokers. Although studies have shown that smoking is not conducive to aerobic capacity or VO2max in male participants,Citation5,Citation27 whether this is also true for aerobic capacity in women requires further investigation and more evidence. The decrease in the VO2max may be attributed to a substance in cigarettes that increases carbon monoxide saturation of the blood, thereby reducing its oxygen-carrying capacity.Citation27 By examining the level of oxygen partial pressure, other studies have shown that substances in cigarettes gradually increase airway resistance and reduce oxygen diffusion between alveoli and the lungs, subsequently increasing carbon monoxide concentrations in the blood, and reducing the levels of myoglobin-mediated oxygen delivery to the mitochondria.Citation5 Additionally, exercises that emphasize specific repeated sprint at maximum speed and rapid recovery capabilities, particularly intermittent sprint exercises, use substantial amounts of ATP and PCr as a source of energy, which affects the blood lactate concentrations and ultimately limits the anaerobic activity.Citation26 A previous study also showed that smoking increases the production of blood lactate during endurance exercises, clearly demonstrating that smoking can affect the metabolism of carbohydrates and influence the level of blood lactate.Citation28 However, the effects of smoking on physiological responses (for example, blood lactate concentrations and heart rate) during anaerobic exercise are unclear and require further examination.
In summary, smoking can increase carbon monoxide concentrations in the airways, lungs, and blood, increase the risk of sudden death in women, interfere with autonomic nervous system activity, reduce HRV in the resting state, and affect aerobic capacity in men. Moreover, increased intensity in endurance exercises may decrease HRV in nonsmokers. However, a study exploring the influence that smoking exerts on anaerobic exercise performance, physiological response, and HRV changes prior to and after anaerobic exercise has yet to be conducted. Smoking may increase sympathetic dominance at lower levels of submaximal work, and it may, theoretically, decrease the recovery efficiency of HRV after high-intensity exercise. The lack of studies examining this issue may be due to potential methodological problems. Furthermore, the maximal aerobic performance in young female smokers has not been investigated. Thus, the objectives of this study are: 1) to investigate the physiological parameters and HRV in a resting state and in early recovery after an anaerobic exercise test; and 2) to conduct a maximal oxygen uptake test of young female smokers and nonsmokers.
Materials and methods
Participants
Medical histories were obtained through direct interviews, and the exclusion criteria included a history of cardiovascular disease; heart-rate-altering medications; pulmonary or respiratory disorders, including asthma; orthopedic injury; and a history of chronic diseases (for example, thyroiditis, diabetes, and nephropathy), or other metabolic diseases known to affect outcome measures. After a thorough explanation of the study protocol and after showing the study equipment to participants, written informed consent was obtained. This study involved 33 volunteers, and they completed this experiment. Twelve participants were heavy habitual cigarette smokers; 21 nonsmoking volunteers of similar ages served as controls (). The smokers had smoked continuously for 2 or more years (for an average of 46.8 ± 20.0 months; >12 cigarettes per day; 0.6–1.0 mg of nicotine; and 8–12 mg of tar), and the nonsmokers were female university students who had never smoked. All participants were instructed to avoid caffeine and alcohol for 48 hours before and during the experiment. The participants who were smokers were prohibited from smoking on the day of the experiment to avoid the possibility of a rapid change in HRV caused by smoking.Citation16,Citation29 In addition, all participants were instructed to avoid intensive exercise 48 hours before the experiment; however, they were allowed to continue their regular physical activities and diet. This study was approved by the Institutional Review Board of our university.
Table 1 Physiological characteristics of the participants
Experiment design
All participants were required to complete a practice test and a formal test. The practice test was identical to the formal test because the purpose was to enable the participants to familiarize themselves with the testing methods and the study process to prevent a learning effect. The practice test involved the participants completing 6 × 10-second bouts of high-intensity, intermittent sprinting on a Cyclus II electric bicycle ergometer (h/p/cosmos® sports & medical gmbh, Nussdorf-Traunstein, Germany) and a 20-minute shuttle run test after a week-long rest period. One week after the completion of the practice test, the participants were contacted in random order to repeat the process for the formal test. A diagram of the high-intensity intermittent sprinting test (IST) is shown in .
Figure 1 Schematic of the 6 × 10-second intermittent sprint test.


Upon arrival at the laboratory at the appointed time, the participants were shown into a quiet room and instructed to lie on a bed in a supine position to rest for 20 minutes. As the participants rested, an examiner recorded their resting HRV using a Polar heart rate monitor (Polar RS800 CX™; Polar Electro Inc, Kempele, Finland) and a heart rate transmitter attached to the participants’ chest. The participants’ blood pressure (HEM 7111; Omron Healthcare Co, Ltd, Kyoto, Japan) and oxygen saturation levels (Pulse Oximeter [SpO2] SB-100 probe; Rossmax International Ltd, Taipei, Taiwan) were then measured. Approximately 5 μ L of blood was then sampled from the participants’ earlobes and analyzed using a blood lactate analyzer (Lactate Pro™; KDK Corporation, Tokyo, Japan) to measure the resting blood lactate level. After these steps were completed, the participants were instructed to perform a standardized warm-up consisting of general aerobic and dynamic activities. The aerobic activity consisted of riding a bicycle ergometer for 5 minutes, with the loading set to 50 watts, while performing two bouts of 2–4 seconds of intermittent sprinting within the final minute, followed by a series of static stretching activities. The static stretching protocol consisted of six static stretches, each targeting the prime movers of the lower extremities, including hamstrings, quadriceps, gastrocnemius, soleus, hip flexors, and adductors. Each stretch was 30 seconds long with a 5-second rest between stretches. After the participants finished the warm up, they were instructed to sit on the bicycle ergometer to allow the examiner to adjust the seat height and pedal position. After the pedal straps were securely fastened, the participants were instructed to accelerate to their maximal pedaling rate during the IST, which consisted of six 10-second cycling bouts using a formula for the loading of:
Each 10-second bout was followed by 60 seconds of active rest for 60–70 rpm against a loading of 50 watts. In the final 5 seconds of the 60-second rest period, the participants were reminded to prepare to sprint, and each was given the same encouragement and feedback by the examiner. The sprint testing data that were analyzed included the peak power, average power, and the fatigue index.Citation30–Citation32 These three parameters can be employed to understand anaerobic exercise performance and capability. A Polar heart rate monitor (Polar Electro Inc) was used to track the participants’ heart rate, and they were asked to evaluate their self-perceived efforts and experiences using the Borg Rating of Perceived Exertion scale (6–20). The participants’ oxygen saturation levels and blood pressure (postexercise) were measured after they had completed the six bouts of sprints. Subsequently, the participants rested for 5 minutes, and 5 μL of earlobe blood was sampled to analyze the blood lactate level. Finally, the participants were instructed to rest in a supine position in a quiet room for 30 minutes, while their HRV and recovery blood pressure were monitored. The data collected from the tests were calculated using the ergometer’s built-in program and were outputted as a Microsoft Excel file (Microsoft Corporation, Redmond, WA, USA). Total work, peak power, average power, and fatigue index variables were then obtained through computer calculations, based on the process used in Glaister et al.Citation30
One week after performing the IST, the participants completed the 20-minute shuttle run test. The participants were instructed to run increasingly faster until voluntary withdrawal based on exhaustion and an inability to maintain the instructed speed, at which point the test was concluded. The speed of the shuttle run test was controlled using an audio tape recording. The starting speed for the first level was 8 km/hour, with the total speed increased by 0.5 km/hour in each successive level. During the test, a specific sound on the audio tape recording was used to signal the beginning of the next level. The results of this test can be employed to determine the participants’ maximum aerobic capacity by inputting the total number of laps run and their speed into an equation; the resulting value corresponds to the participants’ VO2max ().Citation33,Citation34
Heart rate variability
After attaching the Polar heart rate monitor (Polar Electro Inc) to the participants’ wrist and ensuring that the chest heart rate transmitter was securely connected and matched to the radial arterial pulse, the participants’ HRV was measured. Before the participants performed the IST, they were instructed to lie in a supine position for 20 minutes in a quiet room while their heart rate was measured. After the sprinting test, the participants were, once again, instructed to rest in a supine position for 30 minutes while their HRV was monitored.
After data collection, the nonlinear heart rate signals were automatically corrected using the Polar Precision Performance SW 3.0 software package (Polar Electro Inc). This software was used to correct the ectopic beats and missed beats, and altered data was then converted into the American Standard Code for Information Interchange format. The number of ectopic beats was used to identify the R–R interval within 15% for the power spectral analysis.Citation35–Citation37 In this study, two participants’ HRV data required modification by 8.2% and 12.9%; other participants did not require this correction. Kubios HRV analysis software 2.0 (Biosignal Analysis and Medical Imaging Group, Department of Applied Physics, University of Eastern Finland, Kuopio, Finland) was employed to analyze the HRV data from 10 minutes before exercise (resting value) and 5–10 minutes, 10–20 minutes, and 20–30 minutes postexercise. HRV analysis of the test results can be divided into two categories: time-domain indicators and frequency-domain indicators. The parameters of the time-domain indicators include R–R intervals, SDNN, RMSSD, and pNN50; frequency-domain analysis was conducted using the fast Fourier transform with the Kubios HRV analysis software. Two components are measured in the HRV power spectrum analysis – namely, LF (power in the 0.04–0.15 Hz frequency band) and HF (power in the 0.15–0.4 Hz frequency band), which is considered to be an expression of peripheral vasomotor regulation.Citation18,Citation38 Given that the raw values of HF and LF did not conform to normal distribution, a natural logarithmic transformation was applied (lnLF, lnHF, and lnLF/lnHF). LF and HF were further normalized to better quantify autonomic balance:Citation39,Citation40
Data processing and analysis methods
In this study, the Statistical Package for the Social Sciences version 17.0 for Windows (IBM Corporation, Armonk, NY, USA) was used for data processing and analysis, with the level of significance set as α=0.05 and the effect size denoted as η2 (eta squared). Data collected in this study are presented as the mean values ± standard deviations. A two-way mixed analysis of variance (time × group) was performed to assess the changes in the dependent variables (for example, peak power, average power, total work, fatigue index, rating of perceived exertion, heart rate, and HRV). An independent samples t-test was conducted to assess the VO2max of both groups during the 20-minute shuttle run test. If the results exhibited an interaction, the simple main effect was calculated and analyzed using a Bonferroni post hoc comparison test. Mauchly’s sphericity test was also employed to test the sphericity of this study. Additionally, Levene’s test was used to calculate each variable for homogeneity outcomes and 95% confidence intervals (95% CI) were used to demonstrate statistical precision.
Results
Physiological characteristics of the participants
shows the demographic characteristics of the participants in the smoking group (n=12) and the nonsmoking group (n=21). The results of cardiopulmonary endurance tests showed that the VO2max of the nonsmoking group was significantly higher than that of the smoking group (31.6 ± 4.7 mL/minute/kg versus 26.5 ± 3.4 mL/minute/kg; t=−3.2; P<0.01; power =1.0).
Testing performance and perceived fatigue
The participants’ performance regarding the IST was denoted by total work (), peak power (), average power (), and the fatigue index (). The results showed a significant difference in the interaction of average power (F=6.0; P<0.01; η2=0.16; power =0.94), but the interaction of total work (F=7.0; P>0.05; η2=0.19; power =0.96) and peak power (F=4.3; P>0.05; η2=0.12; power =0.8) did not exhibit significant differences. The post hoc comparison shows that the average power of the smoking group for bouts 4–6 was significantly lower than that of the nonsmoking group. Nevertheless, both groups exhibited a decline in average power as they progressed through the bouts. The main effect for the total work (F=0.03; P>0.05; η2=0.0; power =0.05) and peak power (F=0.05; P>0.05; η2=0.0; power =0.06) showed that the between-group factors did not exhibit significant differences, unlike the time factor. The results of the fatigue index showed no interaction between the two factors (F=0.7; P>0.05; η2=0.02; power =0.28), but the group (F=14.8; P<0.01; η2=0.32; power =0.96) and time (F=27.9; P<0.01; η2=0.84; power =1.0) factors both exhibited a significant main effect. The results show that the fatigue index of the smoking group (95% CI =31.0–40.5) was significantly higher than that of the nonsmoking group (95% CI =20.9–28.2; P<0.01). Additionally, the results of the rating of perceived exertion scale show no interaction between smoking and time (F=0.9; P>0.05; η2=0.03; power =0.21) (). The between-group main effect also showed no significant difference (F=0.4; P>0.05; η2=0.00; power =0.06); only the time factor exhibited a significant difference (F=58.4; P<0.01; η2=0.92; power =1.0). A post hoc comparison of the time factor indicated that for both groups, the rating of perceived exertion increased as the participants progressed through the IST (smoking group 95% CI =13.7–15.8 versus the nonsmoking group 95% CI =13.8–15.4).
Figure 2 The participants’ anaerobic performance and fatigue index during the 6 × 10-second IST.
Abbreviation: IST, an intermittent sprint test.

Physiological responses
The dependent variables of physiological responses included systolic blood pressure and diastolic blood pressure, heart rate during exercise, and changes in blood lactate and oxygen concentrations at various time points during the sprinting test. The results showed no interaction effects for the systolic blood pressure (F=2.23; P=0.11; η2=0.07; power =0.44) and diastolic blood pressure (F=1.50; P=0.23; η2=0.05; power =0.31). The group factor also showed no significant difference in systolic blood pressure and diastolic blood pressure (P>0.05; η2=0.05–0.07; power =0.25–0.32), but the time factor exhibited a significant difference (P<0.01). The results for various times indicated that the systolic and diastolic blood pressure measurements obtained immediately after the IST were significantly higher than those obtained at baseline and during recovery ().
Table 2 Blood pressure at various time points for the participants in the smoking and nonsmoking groups
The heart rate results for each 10-second sprint interval indicated that the group and time factors had no interaction (F=0.85; P>0.05; η2=0.03; power =0.21). The group factor exhibited no main effect (P>0.05); only the time factor showed a main effect (P<0.01). A post hoc comparison of the time factor showed that the participants’ heart rates increased with increasing sprint counts. The average heart rates of the participants in the smoking group when performing bouts 1–6 were as follows: 159 ± 13 beats per minute (bpm), 168 ± 8 bpm, 172 ± 8 bpm, 174 ± 9 bpm, 175 ± 9 bpm, and 177 ± 9 bpm. For the participants in the nonsmoking group, the average heart rates across bouts 1–6 were 158 ± 12 bpm, 166 ± 14 bpm, 172 ± 11 bpm, 174 ± 11 bpm, 176 ± 14 bpm, and 179 ± 10 bpm.
The blood lactate concentrations showed a significant interaction with the time and group factors (F=4.15; P=0.05; η2=0.12; power =0.51). However, after comparing the simple main effect, a post hoc comparison of the group factor showed no significant difference (smoking group versus nonsmoking group at rest =0.95 ± 0.3 mmol/L versus 1.0 ± 0.4 mmol/L; P>0.05; at postexercise =10.4 ± 1.5 versus 9.1 ± 2.2 mmol/L; P>0.05). A post hoc comparison of the time factor showed a significant difference (P<0.01), and the participants’ blood lactate concentrations were higher after exercise than when at rest. Furthermore, the oxygen saturation levels of the participants in the different groups and across various times showed no interaction (F=0.06; P>0.05; η2=0.00; power =0.06); the time and group factors also did not exhibit any significant differences (P>0.05). Thus, the results clearly show that neither smoking nor nonsmoking affects oxygen saturation levels before and after anaerobic exercise.
Heart rate variability
The average heart rates of both groups when at rest, 5–10 minutes postexercise, 10–20 minutes postexercise, and 20–30 minutes postexercise showed no significant interaction (F=1.58; P>0.05; η2=0.05; power =0.30). The main effect of the group factor also showed no signifi-cant difference (F=1.41; P>0.05; η2=0.04; power =0.21), although the main effect of the time factor did (F=73.92; P<0.01; η2=0.88; power =1.0). A post hoc comparison of the time factor indicated that the participants’ average resting heart rates were substantially lower than their heart rates at 5–10 minutes, 10–20 minutes, and 20–30 minutes postexercise. Furthermore, the participants’ average heart rates at 5–10 minutes and 10–20 minutes postexercise were higher than their average heart rate at 20–30 minutes postexercise. The average heart rates at various time intervals for the participants in both the smoking and nonsmoking groups were as follows: resting (72 ± 12 bpm versus 71 ± 7 bpm); 5–10 minutes postexercise (104 ± 21 bpm versus 96 ± 8 bpm); 10–20 minutes postexercise (94 ± 9 bpm versus 92 ± 7 bpm); and 20–30 minutes postexercise (90 ± 10 versus 86 ± 7 bpm).
The time-domain results shown in indicate that the R–R interval (F=0.98; η2=0.03; power =0.18), SDNN (F=0.30; η2=0.01; power =0.09), RMSSD (F=0.03; η2=0.00; power =0.05), and pNN50 (F=1.02; η2=0.03; power =0.17) of both groups at different times exhibited no significant interaction (P>0.05). The group factor of the time-domain variables showed no significant main effect (P>0.05), but the time factor showed a significant difference (P<0.01). shows the following frequency-domain results: lnLF: F=0.31, η2=0.01, power =0.11; lnHF: F=0.99, η2=0.03, power =0.22; lnLF/lnHF: F=0.74, η2=0.02, power =0.17; LF/ HF: F=0.94, η2=0.03, power =0.21; LFnu: F=0.56, η2=0.02, power =0.14; and HFnu: F=0.56, η2=0.02, power =0.14. These dependent variables show no significant differences in terms of an interaction (P>0.05). The results showed that the main effects of each frequency-domain indicator on the time factor differed significantly (P<0.01). However, regarding the main effect on the group factor, only LFnu, HFnu, lnLF/ lnHF, and LF/HF exhibited a significant difference (P<0.05), whereas lnLF and lnHF demonstrated no significant main effect (P>0.05).
Figure 4 Time-domain analysis of heart rate variability at rest and after the 6 × 10-second intermittent sprint test for the participants in the smoking and nonsmoking groups.
Abbreviations: Pre-Ex, preexercise; Post-Ex, postexercise; SDNN, standard deviation of the normal to normal interval; RMSDD, root mean square successive difference; pNN50, percentage of adjacent normal R–R intervals >50 ms.

Figure 5 Frequency-domain analysis of heart rate variability at rest and after the 6 × 10-second intermittent sprint test for the participants in the smoking and nonsmoking groups.
Abbreviations: lnLF, natural logarithm of low frequency; Pre-Ex, preexercise; Post-Ex, postexercise; lnHF, natural logarithm of high frequency; lnLF/lnHF, natural logarithm of low/high frequency; LF/HF, low frequency/high frequency ratio; LFnu, normalized LF; HFnu, normalized HF.

Discussion
Testing performance and physiological responses
The values of the total work, peak power, rating of perceived exertion, heart rate during exercise, blood pressure, blood lactate concentrations, and oxygen saturation levels collected during the anaerobic high-intensity IST all showed no significant difference between the two groups. Nevertheless, the smoking group exhibited a significantly lower average power and a higher fatigue index when exercising, compared with the nonsmoking group. The VO2max of the participants in the smoking group during aerobic exercise was also lower than that of the nonsmoking group.
According to the results of the cardiopulmonary endurance tests, the participants’ VO2max represents their maximum cardiopulmonary capacity when performing full-body active exercise. The VO2max of the nonsmoking women exceeded that of the smokers by 16%, indicating that smoking does affect women’s VO2max. This finding agrees with that reported by Klausen et al.Citation27 Other research has also highlighted that smoking activates Na+–K+–ATPase enzymes in the heart, which reduces the vascular contraction force.Citation41 This subsequently affects the blood oxygen carrying capacity, increases carboxyhemoglobin concentrations in the blood, and reduces the rate of oxygen dissolution.Citation42 In addition, this can potentially limit the oxygen-carrying capacity of myoglobin during maximum exercise,Citation43 leading to a reduced VO2max.Citation5 If a smoker’s VO2max is higher than the average level, the effects that smoking exerts on the human body may be reduced.Citation27 Additionally, female smokers can improve cardiopulmonary fitness if they abstain from smoking for a certain period of time and engage in regular physical activity.Citation44
The results of the physiological responses during exercise for both the smoking and nonsmoking groups showed no significant difference. This implies that for female university students, whether or not they smoke does not affect their total work or peak power output during high-intensity intermittent sprints, nor does it affect their heart rate during the IST or their rating of perceived exertion. However, the average power of the nonsmoking participants during bouts 4–6 was significantly higher than that of the smoking participants. Thus, although smoking does not affect sprint performance during the initial stages of exercise, it does reduce the amount of muscular strength available during the later stages of exercise. Investigating the effects that smoking exerts on muscle strength, Morse et alCitation45 observed no difference in the maximal voluntary isometric knee extension torque of the quadriceps between smokers and nonsmokers. However, as the tests were repeated, the smokers’ fatigue index increased by 17%, showing that smokers were significantly less capable of coping with fatigue, compared with nonsmokers. These results support this study’s findings of a higher fatigue index for smokers when performing high-intensity intermittent sprints, compared with nonsmoking participants. A possible cause of this phenomenon may be related to carbon monoxide reducing the ability of skeletal muscles to resist fatigue.Citation45,Citation46 This study used the same relative units as evaluation criteria for peak power and average power; that is, the power output of each participant’s pedaling was divided by their weight and converted into identical units for comparison. That both groups exhibited a similar anaerobic exercise performance in peak power suggests that smoking does not significantly affect initial high-intensity intermittent sprint performance. Nevertheless, the substantial increase in the fatigue index of the smoking group when performing the IST shows that smoking does not benefit the participants’ ability to endure fatigue in the later phase during the intermittent sprint test that are a part of anaerobic exercise. The fatigue index was calculated using maximum and minimum power and can be used to represent the decline in the maximum power output of skeletal muscles during high-intensity intermittent sprinting. Thus, another cause of the higher fatigue index among participants in the smoking group may be the limitations of their cardiopulmonary function, because aerobic energy metabolism plays a critical role in supplying energy during intermittent sprinting exercises.Citation32 Because smokers typically have poor cardiopulmonary fitness, their body may compensate by limiting the amount of energy supplied by the aerobic system for exercise. Another possible explanation is that smoking limits oxygen delivery via the capillaries in muscles, reducing the ability of skeletal muscles to endure fatigue.Citation8
The results of blood lactate concentrations before and after the IST imply that smoking does not influence the use of energy from matter. High-intensity intermittent sprinting requires an effective ATP system and glycolytic metabolism.Citation26 Although a number of studies support the notion that smoking increases glycolytic metabolism,Citation5 the associated extensive use of carbohydrates as an energy source for muscles increases blood lactate production in muscles.Citation28 Huie et alCitation28 observed that a smoking group had higher blood lactate concentrations compared with a control group; however, these results were obtained from aerobic exercise tests, which differed from the high-intensity IST conducted in this study. We speculate that the blood lactate concentration variations in smokers differ according to the type of exercise they perform. We suggest that smoking did not affect the glycolytic metabolic pathway while participants performed the IST. Whether the effects that smoking exerts on blood lactate concentrations would become more apparent if the number or duration of the sprints were increased requires further investigation. We also observed that systolic and diastolic blood pressure in smokers did not differ from that of nonsmokers. Although numerous factors influence blood pressure, the baseline blood pressure of the female smokers examined in this study was normal. This may have been because of the participants’ young age and relatively short smoking history. The reason that both groups exhibited no differences in blood pressure at the baseline and during the recovery period may be that anaerobic exercise causes equal levels of motor stimulation for smokers and nonsmokers, or it may be due to the fact that the participants’ blood pressure was measured at different time points and in a random order during the testingCitation15 (for example, certain participants may have performed the anaerobic exercise test in the morning and some in the afternoon). Another possible explanation for no differences in blood pressure at the baseline level and during the recovery phase, may be due to variability in the regulation of the central nervous system.Citation47 Since numerous explanations are possible, further control of the factors influencing the experiment is required to determine the potential mechanism behind these effects.
Heart rate variability
The results of this study show that no significant differences in the time-domain indicators of HRV (R–R interval, SDNN, RMSSD, and pNN50) existed between the two groups. However, for the time point of 5–10 minutes postexercise, both groups showed time-domain indicator results that were lower than those for preexercise, 10–20 minutes postexercise, and 20–30 minutes postexercise. For the results of the frequency-domain indicators, no significant difference in the main effect of the lnLF and lnHF was observed between the participant groups. However, the smoking group had a significantly lower HFnu, as well as significantly higher LFnu, LF/HF, and lnLF/lnHF values compared with those of the nonsmoking group. The overall results of the time factor showed that the resting values of lnLF and lnHF were higher than at any time point after exercise. LF/HF, lnLF/lnHF, and LFnu values all significantly increased after exercise, and gradually returned to normal over the course of the rest period. However, HFnu markedly declined in the initial periods after exercise was ceased and gradually increased after extended rest.
Although the time-domain indicator results exhibit a noticeable difference in the HRV of the two groups, the vagus nerve stimulation was substantially higher immediately after exercise when compared with preexercise. The drastic decline in the R–R interval, SDNN, RMSSD, and pNN50 5–10 minutes postexercise in the smokers and nonsmokers may have occurred because the high-intensity of the intermittent anaerobic exercise intervention reduced parasympathetic activity. With extended rest, the parasympathetic nervous system gradually recovers its regulatory capabilities.Citation48 Although the frequency-domain analysis results indicated no significant differences in lnLF between groups, the smoking group exhibited significantly higher LFnu than the nonsmoking group did. Conversely, the nonsmoking group exhibited significantly higher HFnu, indicating that smoking does alter the activity of sympathetic nervous system and parasympathetic nervous system regulation. LF/HF and lnLF/lnHF are quantitative indicators that measure the cardiac sympathetic– parasympathetic balance.Citation19 shows that the LF/ HF of the smoking group exceeds that of the nonsmoking group at 5–10 minutes, 10–20 minutes, and 20–30 minutes postexercise by 41%, 42%, and 58%, respectively. The lnLF/ lnHF of the smoking group for the three time point intervals after the IST also exceeded that of the nonsmoking group by 25%, 21%, and 25%, respectively. These results further confirm that smoking can alter the cardiac sympathetic– parasympathetic balance. Mendonca et alCitation49 reported that the autonomic nervous system functions of a smoker can be influenced in a resting state, and can even become suppressed when the smoker performs exercise at intensities below 30% of the VO2max. This reduces the regulatory ability of the vagus nerve and increases sympathetic dominance. Kaikkonen et alCitation25 observed that HRV declines significantly after a single round of high-intensity exercise, suggesting that exercise intervention can temporarily reduce autonomic nervous system functions. Niewiadomski et alCitation50 investigated the effects of performing two consecutive 30-second sprints on HRV in healthy men. They observed that during the postexercise recovery period, the regulatory functions of the vagus nerve were superior to the sympathetic nervous system activity. This differs substantially from the findings of this study, which indicate that after exercise, the parasympathetic dominance of female smokers is significantly reduced, whereas the sympathetic dominance is enhanced. This highlights that 30 minutes after female smokers perform high-intensity exercise, the parasympathetic nervous system activity remains suppressed, whereas the sympathetic nervous system activity remains stimulated. Smoking may suppress the regulatory drive of the vagus nerve after high-intensity intermittent sprints. Thus, smoking may negatively influence the recovery and regulation of the autonomic nervous system after high-intensity exercise.
The values of HFnu and lnHF at rest substantially exceeded those after exercise, showing that a higher level of vagus nerve regulatory activity occurs before exercise. Previous research has revealed that smoking can reduce RMSSD and SDNN while increasing the LF/HF ratio, clearly demonstrating that smoking has negative effects on the modulation of the autonomic nervous system to control the cardiovascular system’s regulatory activities.Citation11 Studies have also shown that nonsmokers have a higher resting HF than smokers do,Citation10,Citation14 indicating that smoking weakens the regulatory activities of the vagus nerve when in a resting state. Both short- and long-term smokers are at risk of an acute or transient decline in vagus cardiac control;Citation13 thus, smoking could easily affect the regulatory functions of the parasympathetic nervous system. Research has also indicated that the autonomic nervous system of heavy smokers exhibits weakened regulatory capabilities, which may be a key contributing factor to cardiovascular disease.Citation14 The results of this study show that lnLF before exercise was significantly higher than at all time points after exercise, which means that the combined stimulation of the sympathetic and vagus nerves exceeded that after exercise. Furthermore, the results of LF/HF and lnLF/lnHF in this study confirm that exercise can increase and maintain sympathetic nervous system modulation. Other factors that affect sympathetic nervous system stimulation may include the number of years that the participant has smoked,Citation14 the age of the participant,Citation20 and whether or not the participant is in a smoke-filled environment before exercise, because acute exposure to a smoke-filled environment lowers HRV.Citation16 However, this study instructed the participants in the smoking group not to smoke and to avoid secondhand smoke exposure on the day of the test to avoid affecting the results; therefore, the possibility of acute exposure to a smoke-filled environment prior to exercise can be excluded as a contributing factor. Although a previous study showed no significant difference in the breathing rate between smoker and control groups, the breathing rate may change HRV indicators, and this is a potential limitation of this study.Citation49 Overall, cigarette smoking impaired sympathetic–parasympathetic balance at rest and after intermittent sprint exercise in young female students.
Conclusion
This study concludes that smoking is detrimental to cardiopulmonary functions and may reduce the VO2max of women. The IST performance of female smokers begins to noticeably decline in the later stages of anaerobic exercise, and the fatigue-resisting ability of their skeletal muscles is reduced. However, smoking does not affect a person’s physiological responses, such as heart rate during exercise, blood lactate concentrations, and systolic and diastolic blood pressure before and after exercise. Additionally, the sympathetic dominance in female smokers was significantly higher than that of nonsmokers following IST. The enhanced regulatory capabilities of the sympathetic nervous system and substantially weakened capabilities of the parasympathetic nervous system for female smokers may negatively affect the regulatory capabilities of the vagus nerve during the postexercise recovery period. Therefore, this study encourages female smokers to participate in smoking cessation activities. This can improve the regulatory capabilities of their vagus nerve and enhance the regulatory activity of their autonomic nervous system during postexercise recovery.
Acknowledgments
The authors thank all participants for their efforts and help in implementing the study, and thank the research assistants for their collaboration.
This paper was presented at the oral session of the 18th Annual Congress of the European College of Sport Science during June 26–29, 2013 at Barcelona, Spain. The abstract was published in the congress proceedings.
This study was partly funded by the National Sun Yat-sen University, Kaohsiung, Taiwan.
Disclosure
The authors report no conflicts of interest in this work.
References
- PetoRLopezADBorehamJThunMHeathCJrDollRMortality from smoking worldwideBr Med Bull199652112218746293
- EzzatiMLopezADEstimates of global mortality attributable to smoking in 2000Lancet2003362938784785213678970
- AlexanderCPiazzaMMekosDValenteTPeers, schools, and adolescent cigarette smokingJ Adolesc Health2001291223011429302
- JonasMAOatesJAOckeneJKHennekensCHStatement on smoking and cardiovascular disease for health care professionals. American Heart AssociationCirculation1992865166416691423984
- McDonoughPMoffattRJSmoking-induced elevations in blood carboxyhaemoglobin levels. Effect on maximal oxygen uptakeSports Med199927527528310368876
- ColbergSRCasazzaGAHorningMABrooksGAIncreased dependence on blood glucose in smokers during rest and sustained exerciseJ Appl Physiol199476126328175515
- NadelJAComroeJHAcute effects of inhalation of cigarette smoke on airway conductanceJ Appl Physiol19611671371613727341
- KingCEDoddSLCainSMO2 delivery to contracting muscle during hypoxic or CO hypoxiaJ Appl Physiol19876327267323654434
- GrassiGSeravalleGCalhounDAMechanisms responsible for sympathetic activation by cigarette smoking in humansCirculation19949012482538026005
- BarutcuIEsenAMKayaDCigarette smoking and heart rate variability: dynamic influence of parasympathetic and sympathetic maneuversAnn Noninvasive Electrocardiol200510332432916029383
- CagirciGCaySKarakurtOInfluence of heavy cigarette smoking on heart rate variability and heart rate turbulence parametersAnn Noninvasive Electrocardiol200914432733219804508
- Felber DietrichDSchwartzJSchindlerCSAPALDIA-teamEffects of passive smoking on heart rate variability, heart rate and blood pressure: an observational studyInt J Epidemiol200736483484017440032
- HayanoJYamadaMSakakibaraYShort- and long-term effects of cigarette smoking on heart rate variabilityAm J Cardiol199065184882294686
- LuciniDBertocchiFMallianiAPaganiMA controlled study of the autonomic changes produced by habitual cigarette smoking in healthy subjectsCardiovasc Res19963146336398689656
- MinamiJIshimitsuTMatsuokaHEffects of smoking cessation on blood pressure and heart rate variability in habitual smokersHypertension1999331 Pt 25865909931170
- PopeCAEatoughDJGoldDRAcute exposure to environmental tobacco smoke and heart rate variabilityEnviron Health Perspect2001109771171611485870
- SteinPKRottmanJNKleigerREEffect of 21 mg transdermal nicotine patches and smoking cessation on heart rate variabilityAm J Cardiol19967797017058651120
- Heart rate variability: standards of measurement, physiological interpretation and clinical use. Task Force of the European Society of Cardiology and the North American Society of Pacing and ElectrophysiologyCirculation1996935104310658598068
- KleigerRESteinPKBiggerJTHeart rate variability: measurement and clinical utilityAnn Noninvasive Electrocardiol20051018810115649244
- HeringDSomersVKKaraTSympathetic neural responses to smoking are age dependentJ Hypertens200624469169516531797
- AlyanOKaçmazFOzdemirO[High levels of high-sensitivity C-reactive protein and impaired autonomic activity in smokers.]Turk Kardiyol Dern Ars2008366368375 Turkish19155639
- HeringDSomersVKKaraTHeightened acute circulatory responses to smoking in womenBlood Press200817314114618608198
- PierpontGLStolpmanDRGornickCCHeart rate recovery post-exercise as an index of parasympathetic activityJ Auton Nerv Syst200080316917410785283
- KaikkonenPNummelaARuskoHHeart rate variability dynamics during early recovery after different endurance exercisesEur J Appl Physiol20071021798617899162
- KaikkonenPRuskoHMartinmäkiKPost-exercise heart rate variability of endurance athletes after different high-intensity exercise interventionsScand J Med Sci Sports200818451151918067516
- GlaisterMMultiple sprint work: physiological responses, mechanisms of fatigue and the influence of aerobic fitnessSports Med200535975777716138786
- KlausenKAndersenCNandrupSAcute effects of cigarette smoking and inhalation of carbon monoxide during maximal exerciseEur J Appl Physiol Occup Physiol19835133713796685036
- HuieMJCasazzaGAHorningMABrooksGASmoking increases conversion of lactate to glucose during submaximal exerciseJ Appl Physiol1996805155415598727539
- NarkiewiczKvan de BornePJHausbergMCigarette smoking increases sympathetic outflow in humansCirculation19989865285349714109
- GlaisterMHowatsonGPattisonJRMcInnesGThe reliability and validity of fatigue measures during multiple-sprint work: an issue revisitedJ Strength Cond Res20082251597160118714226
- TrumpMEHeigenhauserGJPutmanCTSprietLLImportance of muscle phosphocreatine during intermittent maximal cyclingJ Appl Physiol1996805157415808727542
- SpencerMBishopDDawsonBGoodmanCPhysiological and metabolic responses of repeated-sprint activities:specific to field-based team sportsSports Med200535121025104416336007
- LégerLALambertJA maximal multistage 20-m shuttle run test to predict VO2maxEur J Appl Physiol Occup Physiol19824911127201922
- LégerLAMercierDGadouryCLambertJThe multistage 20 metre shuttle run test for aerobic fitnessJ Sports Sci198862931013184250
- AubertAERamaekersDBeckersFThe analysis of heart rate variability in unrestrained rats. Validation of methods and resultsComput Methods Programs Biomed199960319721310579513
- AcarBSavelievaIHemingwayHMalikMAutomatic ectopic beat elimination in short-term heart rate variability measurementComput Methods Programs Biomed200063212313110960745
- ChengCFLinHMTsaiHCChuCHLinJC[Effects of acute exposure to moderate altitude on heart rate variability in rowers]Physical Education Journal20053815567 Chinese
- EckbergDLSympathovagal balance: a critical appraisalCirculation1997969322432329386196
- ParekhALeeCMHeart rate variability after isocaloric exercise bouts of different intensitiesMed Sci Sports Exerc200537459960515809558
- PichonAPde BisschopCRoulaudMDenjeanAPapelierYSpectral analysis of heart rate variability during exercise in trained subjectsMed Sci Sports Exerc200436101702170815595290
- MiuraHToyamaKPrattPFGuttermanDDCigarette smoking impairs Na+-K+-ATPase activity in the human coronary microcirculationAm J Physiol Heart Circ Physiol20113001H109H11721076023
- RietbrockNKunkelSWörnerWEyerPOxygen-dissociation kinetics in the blood of smokers and non-smokers: interaction between oxygen and carbon monoxide at the hemoglobin moleculeNaunyn Schmiedebergs Arch Pharmacol199234511231281538790
- HirschGLSueDYWassermanKRobinsonTEHansenJEImmediate effects of cigarette smoking on cardiorespiratory responses to exerciseJ Appl Physiol1985586197519814008417
- AlbrechtAEMarcusBHRobertsMFormanDEParisiAFEffect of smoking cessation on exercise performance in female smokers participating in exercise trainingAm J Cardiol19988289509559794350
- MorseCIWüstRCJonesDAde HaanADegensHMuscle fatigue resistance during stimulated contractions is reduced in young male smokersActa Physiol (Oxf)2007191212312917550408
- MorseCIPritchardLJWüstRCJonesDADegensHCarbon monoxide inhalation reduces skeletal muscle fatigue resistanceActa Physiol (Oxf)2008192339740117970829
- ValentiVEVanderleiLCFerreiraCSidestream cigarette smoke and cardiac autonomic regulationInt Arch Med2013611123497654
- JavorkaMZilaIBalhárekTJavorkaKHeart rate recovery after exercise: relations to heart rate variability and complexityBraz J Med Biol Res2002358991100012185393
- MendoncaGVPereiraFDFernhallBEffects of cigarette smoking on cardiac autonomic function during dynamic exerciseJ Sports Sci201129987988621547834
- NiewiadomskiWGasiorowskaAKraussBMrózACybulskiGSuppression of heart rate variability after supramaximal exertionClin Physiol Funct Imaging200727530931917697028