Abstract
Engineered nanomaterials (ENMs) are a diverse group of materials finding increasing use in manufacturing, computing, food, pharmaceuticals, and biomedicine due to their very small size and exceptional properties. Health and safety concerns for ENMs have forced regulatory agencies to consider preventive measures and regulations for workers’ health and safety protection. Respiratory system toxicity from inhalable ENMs is the most important concern to health specialists. In this review, we focus on similarities and differences between conventional microparticles (diameters in mm and μm), which have been previously studied, and nanoparticles (sizes between 1 and 100 nm) in terms of size, composition, and mechanisms of action in biological systems. In past decades, respirable particulate matter (PM), asbestos fibers, crystalline silicate, and various amorphous dusts have been studied, and epidemiological evidence has shown how dangerous they are to human health, especially from exposure in working environments. Scientific evidence has shown that there is a close connection between respirable PM and pulmonary oxidative stress through the generation of reactive oxygen species (ROS) and reactive nitrogen species (RNS). There is a close connection between oxidative stress in the cell and the elicitation of an inflammatory response via pro-inflammatory gene transcription. Inflammatory processes increase the risk for lung cancer. Studies in vitro and in vivo in the last decade have shown that engineered nanoparticles (ENPs) at various doses can cause ROS generation, oxidative stress, and pro-inflammatory gene expression in the cell. It is assumed that ENPs have the potential to cause acute respiratory diseases and probably lung cancer in humans. The situation regarding chronic exposure at low doses is more complicated. The long-term accumulation of ENPs in the respiratory system cannot be excluded. However, at present, exposure data for the general public regarding ENPs are not available.
Introduction
In the beginning of the 21st century, engineered nanomaterials (ENMs) and their applications in a great range of biomedical, pharmaceutical, and consumer products caused great excitement among the scientific and engineering communities. Total worldwide sales revenues for nanotechnology were US$11.6 billion in 2009, and are expected to increase to more than US$26 billion (11% increase) in 2015.Citation1 The workforce in nanotechnology products is expected to reach 6 million by 2020, and annual investment in global nanotechnology research and development (R&D) will increase substantially from US$17.8 billion (2010).Citation2
Much has been written in the scientific literature about ENMs and the possible dangers to human health and the environment.Citation3,Citation4 ENMs are a diverse group of materials finding increasing use in manufacturing, energy, motorcars, textiles, computing, food, pharmaceuticals, and biomedicinal products due to their very small size and exceptional engineered properties. The percentage distribution of ENM applications in 2007 was as follows: chemicals 53%; semiconductors 34%; electronics 7%; aerospace and defence 3%; pharmaceuticals and healthcare 2%; and automotive 1%.Citation5–Citation8
From the beginning, the applications of nanotechnology products raised concerns among toxicologists and occupational health and safety (H&S) specialists over risks to human health and the environment. Adverse health effects from ENMs are expected to be observed in workers who are exposed during the manufacturing process, preparation, and formulation of ENMs in various stages of product formulation. Consumers are the second line of concern regarding ENM exposure during their use, especially with inhalation or skin penetration. Another group of ENM users are patients receiving nano-biomedical applications and delivered pharmaceuticals with the help of nanoparticle technologies. Exposure may therefore occur in a variety of occupational settings where ENMs are used, handled, or processed and consequently become airborne and can be inhaled, or come into contact with the skin; for example, in contexts from healthcare or laboratory work, to maintenance or construction work.Citation9–Citation12
The most important route of exposure to various particles (environmental or occupational) remains inhalation and subsequent risks to the respiratory system because of their minute size and penetration into the lung’s alveoli. Coarse particles range in size between 10,000 nm and 2,500 nm (1 nm =10−9 m); fine particles between 2,500 nm and 100 nm; and ultrafine particles (UFPs) between 100 nm and 1 nm.
Studies in recent decades have indicated that fine particles of toxic and carcinogenic chemicals with high persistency can influence toxicological mechanisms. These particles have the potential to generate reactive oxygen species (ROS), oxidative stress, inflammation, and carcinogenic mechanisms in the respiratory system.Citation13–Citation16 Additionally, tobacco smoke and environmental airborne respirable particulate matter (PM) can act synergistically to increase inflammatory reactions and risk for lung carcinogenesis.Citation17,Citation18
H&S concerns for ENMs have forced regulatory agencies to consider preventive measures and regulations. The European Union (EU) legislation on worker protection applies to nanomaterials, although it does not refer explicitly to them. Of particular relevance are the Framework Directive 1989/391/EEC (H&S of workers at work), the Chemical Agent Directive 1998/24/EC (H&S of workers from the risks related to chemical agents at work), and the Carcinogen and Mutagen Directive 2004/37/EC (protection of workers from the risks related to exposure to carcinogens or mutagens). ENMs are also covered by the legislation on chemicals (EC 1907/2006, Registration, Evaluation, Authorisation and Restriction of Chemicals; REACH), and the CLP Regulation (Classification, Labelling and Packaging, EC No 1272/2008) that ensures that the hazards presented by chemicals are clearly communicated to workers and consumers in the EU.
The Environmental Protection Agency (EPA) and other regulatory H&S bodies in the USA are using a comprehensive environmental assessment approach to identify and prioritize research to support future assessments and risk management decisions for ENMs. The EPA is using scientific methods to research what ENMs are, and how they act, travel, and change over time. The EPA’s Chemical Safety and Pollution Prevention Office and other regulatory bodies in the last decade have made chemical and regulatory decisions to better protect human health and the environment from ENMs.Citation19
The US Food and Drug Administration (FDA) regulates a wide range of products (foods, cosmetics, drugs, devices, veterinary products) that may utilize nanotechnology or contain nanomaterials. In April 2012, the US FDA issued two product-specific draft guidance documents to address the use of nanotechnology by the food and cosmetics industries as part of the US FDA’s ongoing implementation of recommendations from the US FDA’s 2007 Nanotechnology Task Force Report.Citation20
Similar H&S laws and regulations have been initiated in other developed industrialized countries. The Organization for Economic Co-operation and Development (OECD) has introduced a database, Research into the Safety of Manufactured Nanomaterials, which helps to identify research gaps and assist researchers in future collaborative efforts. The database also assists the projects of the OECD’s Working Party on Manufactured Nanomaterials (WPMN) as a resource of research information.Citation21,Citation22
H&S regulations mean that employers are required to assess and manage the risks of ENMs at work. If the use and generation of ENMs cannot be eliminated or substituted with less hazardous materials and processes, worker exposure must be minimized through prevention measures, technical control measures at source, organizational safety measures, and, as a last resort, use of personal protection equipment.
Similarities of conventional coarse, microparticles, and fine particles with engineered nanoparticles (ENPs)
In the present paper, we use the terms “coarse”, “microparticles”, and “fine particles” for a range of respirable PM and mineral fibers that have been the subject of research efforts in the working environment in recent decades; whereas the size of superfine particles from combustion sources is very close to that of nanoparticles.
For comparison reasons, it is important to address similarities and differences between coarse particles, microparticles, and fine and superfine particles versus nanoparticles in terms of size, composition, and mechanisms of action in biological systems. Respirable PM, mineral fibers (eg, asbestos), crystalline silicate, amorphous dusts, and aerosols occur naturally, but most of the dangerous inhalable PM is anthropogenic in origin. PM from combustion is a complex, heterogeneous mixture that encompasses many different chemical components and physical characteristics, many of which have been cited as potential contributors to toxicity. Airborne combustion particles from fossil fuels are considered to be the main atmospheric particulate pollutants that can lodge in the lungs and cause oxidative stress, initiate inflammatory responses, and promote carcinogenic mechanisms to lung tissues.Citation23,Citation24
Over the last few decades, many studies have been carried out with various dusts, inorganic and organic particle mineral fibers, cement dust, crystalline silica, and fly ash in the size range of 10–100 μm: inhalable suspended particles with diameters of 10 μm (PM10; 1 μm=10–6 m), or smaller and airborne fine particles (dynamic diameter of 2.5 μm; PM2.5). UFPs have a diameter less than 100 nm, and automobile exhaust soot is in the range of 0.01–1 μm (PM0.1). UFPs are deposited in the lungs, where they have the ability to penetrate tissue and undergo interstitialization, or to be absorbed directly into the bloodstream. Size and surface properties are very important. Exposure to UFPs, even if components are not very toxic, may cause oxidative stress and inflammatory damage and could induce lung diseases.Citation25–Citation27
Coarse particles and microparticles have, for decades, been the subject of numerous toxicological studies, and their relationship to respiratory adverse health effects has been observed in various occupational environments. Also, airborne PM (PM10, PM2.5, and PM0.1) in urban areas has been connected to respiratory morbidity and premature mortality by numerous epidemiologic studies.Citation28–Citation30
ENMs constitute an emerging man-made pollution source that is, so far, literally buried beneath much larger natural sources and ultrafine (with nanosize particles) pollution from automobile exhaust soot in urban areas. Irrespective of size, some particles, such as metal dusts, welding fumes, and quartz dust, are inherently toxic, while others have a much lower toxicity, but can cause inflammatory toxic effects in the lungs. This is especially true for biodurable particles without known specific toxicity, including mineral dusts, carbon black, coal-mine dust, titanium dioxide (TiO2), and others as listed in .
Table 1 Particles with proven lung carcinogenic effects in animals and/or humans (most of the classifications are also classified by the International Agency for Research on Cancer, IARC)
Scientific studies have identified a variety of mechanisms that play key roles in particle-induced chronic and acute respiratory diseases. In high concentrations and with prolonged exposure in occupational environments, PM can advance lung carcinogenesis. The most important mechanisms are 1) generation and oxidative action by ROS and reactive nitrogen species (RNS), causing extensive lipid peroxidation (cellular membranes), enzyme, and DNA damage; 2) oxidative stress; 3) ROS-stimulated signaling moieties involved in many carcinogenic pathways (induction of cell and tissue proliferation, angiogenesis, etc); and 4) inflammation-driven processes with high significance for lung mutagenesis and carcinogenesis.Citation33–Citation35
In vitro observations demonstrate inflammation-independent particle effects related to the physicochemical properties of particles (eg, surface activity, polycyclic aromatic hydrocarbons [PAHs], nitro-PAHs, metals with redox potential, etc); however, the outcomes of in vitro and in vivo studies should be interpreted with caution for risk-assessment purposes, as they are mainly the result of high-dose exposures for long periods of time that are unlikely to be relevant for the human situation, ie, exposure in urban environments.Citation36
Additionally, many epidemiologic studies have provided experimental support for associations between various microscale PM exposure and adverse pulmonary effects in occupational environments. Upon deposition, PMs can trigger ROS/RNS production, inflammatory processes that potential lead to airway obstruction, compromised gas exchange, and the exacerbation of pre-existing conditions. Occupational and urban exposure to PM is associated with lung pathology, including chronic obstructive pulmonary disease (COPD), bronchitis, asthma, and lung cancer.Citation37–Citation39
Differences between ENPs and inhalable mineral fibers, coarse, micro-size, and fine particles
Experimental results with ENPs indicate that various physicochemical characteristics and factors cause nanoparticles to behave significantly differently than conventional mineral fibers, and coarse or micro-size particles (see ).Citation40,Citation41
Table 2 Similarities and differences of coarse particles, microparticles, fine particles, and ultrafine and ENPs
Shape and surface
In ENPs, the arrangement of molecules on the surface causes smooth property scaling due to the fraction of atoms at the surface. In bulk materials larger than 1 μm, the percentage of atoms at the surface is insignificant in relation to the number of atoms in the bulk mass of the material. The unusual properties of ENPs are therefore largely due to the large surface area and high particle numbers per unit mass, which greatly influence their cellular uptake. Probing these various interfaces allows the development of predictive relationships between structure and activity that are determined by nanomaterial properties such as size, shape, surface chemistry, roughness, and surface coatings. All these physicochemical aspects have proved very important with regards to the safe use of nanomaterials.Citation42,Citation43
Examining the influence of shape and size of nanoparticles on cell interactions has proved crucial in toxicity. Another very important factor is that, even though nanoparticles display a certain size after synthesis, during in vitro and in vivo studies they might aggregate into vastly different shapes and sizes.Citation44 Nanoparticle behavior, ie, dispersion or aggregation, plays an important role in toxicity (including photo-oxidation products). Carbon nanotubes (CNTs) apparently have greater pulmonary toxicity (inflammation, granuloma) in mice than does fine-scale carbon graphite, and their metal content may affect toxicity.Citation45
Solubility
Toxic effects of ENPs may be attributed to two different actions: chemical toxicity based on the chemical composition (eg, release of toxic ions), and particle surface catalyzed reactions that generate damaging ROS. Differentiating between these two types of cytotoxicity is not straightforward, especially when particles are partially dissolved during culture treatment. In order to test this proposal, seven different industrially relevant oxide nanoparticles were tested with two well established cell lines (one human, one rodent). The results confirmed that solubility greatly affected the cell culture response to the nanoparticles. Therefore, it appears reasonable to group the different nanoparticles into slightly soluble and insoluble nanomaterials.Citation46
Another physical phenomenon that drew the attention of scientists researching ENPs was dissolution (the dynamic process by which a particle goes into the solution phase to form a homogeneous mixture). Translocation and disposition have been shown to play a key role in the fate and biological effects of inhaled particles and fibers. Concepts that have been applied in the micron size range can be usefully applied to the nanoscale range. Solute concentration, surface area, surface morphology, surface energy, dissolution layer properties, adsorbing species, and aggregation are relevant parameters in considering dissolution at the nanoscale. Dissolution is a critical step for some ENPs in determining biological fate in the environment and within the human body.Citation47,Citation48
Optical properties, photoactivity, redox potential
ENPs often possess unexpected optical characteristics as they are small enough to confine their electrons and produce quantum effects. Changes in optical properties at the nanoscale level are the result of electrons not being as free to move as they are in bulk materials. The very large surface-to-volume (S/V) ratio has a major influence on their optical and surface properties. As a result, semiconductor nanomaterials have attracted significant attention in research and applications in energy conversion, photonics, and biomedicine. ENPs have played a critical role in many important chemical reactions as reactants or photocatalysts and their reactivities are often altered or enhanced due to size-dependent changes in their redox potentials and high density of active surface states. Although the potential for the generation of ROS by ENPs is useful in various applications (degradation of pollutants), it can be dangerous in the respiratory system or to aquatic organisms (from nanomaterial waste in the aquatic environment).Citation49,Citation50
A typical example is TiO2 nanoparticles, which are highly photoactive; their photocatalyst functions drive much of the application demand for TiO2. TiO2 has been used in various ENMs, especially in antibacterial coatings and wastewater disinfection. However, at the same time, TiO2 has the potential for cytotoxicity. In vivo tests of TiO2 toxicity with aquatic organisms have typically shown low toxicity, and results across studies have been variable. Overall, oxidative stress in seawater contaminated by TiO2 can decrease the resiliency of marine ecosystems. Phototoxicity must be considered when evaluating the environmental impacts of various ENMs.Citation51
Quantum dot (QD) effects
ENPs show discontinuous behavior due to quantum confinement effects in materials with delocalized electrons. QD factors affect the chemical reactivity of ENMs, as well as their mechanical, optical, electric, and magnetic properties (superparamagnetism). These properties make ENMs superior to various applications (site-specific medical imaging, drug delivery, etc) but also increase the cytotoxicity of ENMs. QDs are an additional characteristic affecting a rational risk assessment of ENMs. There are mechanisms by which QDs can damage cells, including oxidative stress elicited by ROS.Citation52
Suspensions
Suspensions of ENPs are possible, since the interaction of the particle surface with the solvent is strong enough to overcome density differences, which otherwise usually results in a material either sinking or floating in a liquid. Suspensions of ENPs in aquatic environments are potentially dangerous to aquatic organisms. Experiments regarding the potential ecotoxicity of nanosized TiO2, silicon dioxide (SiO2), and zinc oxide (ZnO) in water suspensions were conducted in test organisms. These photosensitive nanomaterials were observed to be harmful.Citation53 Similarly, the potential for ecotoxicity from various metal oxide nanoparticle suspensions released to aquatic environments were tested with zebrafish 96 hour embryo–larval bioassay. Results demonstrated that ENPs of ZnO were very toxic to zebrafish embryos and larvae.Citation54
Chemical composition
ENMs are considered chemically uniform by one or more chemical pure constituents. Coarse minerals are salts with impurities, microparticles can have two or more components, combustion products are highly toxic pollutants with mixed compositions of oxidative, toxic, and carcinogenic substances (heavy metals, PAHs, dioxins, etc). Nanoparticles can be composed of a single pure constituent material or be a composite of two to three chemical materials.
Toxicological results indicate that most ENPs have produced an array of different toxic effects in many different types of in vivo and in vitro studies. The types of effects that ENPs have produced are those on the pulmonary, cardiac, reproductive, renal, and cutaneous systems, as well as on various cell lines. After inhalation exposure, significant accumulations of ENPs have been found in the lungs, brain, liver, spleen, and bones of test species. Toxicity of ENPs has been linked to their surface properties. Soluble ENPs are rendered toxic because of their constituents; however, the situation is entirely different for insoluble ENPs. Stable metal oxides do not show any toxicity, whereas metallic ENPs that have redox potential may be cytotoxic and genotoxic.Citation55
To illustrate size differences between a conventional size strand of hair, microparticles, and nanoparticles, presents one carbon microparticle with a diameter of 60 μm, which has a mass of 0.3 μg and a surface area of 0.01 mm2. Nanoparticles with a diameter of 600 nm can have 1 million particles, whereas nanoparticles with a diameter of 60 nm have a surface area of 11.3 mm2 and consist of 1 billion nanoparticles (). The S/V ratio (or mass) for a particle with a diameter of 60 nm is 1,000 times larger than a particle with a diameter of 60 μm. As a result, chemical reactivity is substantially enhanced. The atoms situated at the surface have fewer neighbors than bulk atoms, resulting in lower binding energy per atom. Reduced binding energy per atom can cause a decrease in melting point.Citation42
Figure 1 Comparative sizes for (from left to right) human hair (60 μm diameter), spherical microparticle of the same diameter, 1 million particles 600 nm diameter, and 1 billion nanoparticles of 60 nm diameter.
The article/figure is published under Creative Commons License 2.0 CC-BY.38.
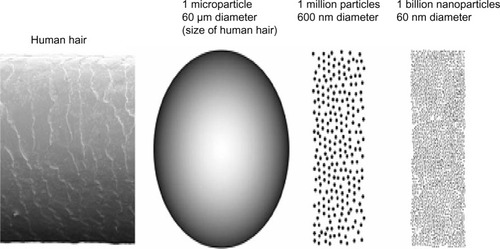
Potential respiratory toxicity and carcinogenesis of inhaled ENPs
Based on past toxicological studies of the relationship between inhalation of PM (so-called conventional particles, coarse, fine, and superfine) and respiratory diseases, scientists directed their attention to similar effects relating to ENPs. The potential of ENPs inhaled under various exposure conditions to cause oxidative stress in the respiratory system and, subsequently, inflammation and initiation of lung carcinogenesis has been under investigation in the last decade.Citation56–Citation58
Experimental data have shown limited evidence that ENPs cause acute respiratory diseases and lung cancer in humans. Until now, manufactured microparticles and UFPs (nanosize), such as TiO2, carbon black, crystalline silica, aluminum dust, etc, have been produced for many decades, but epidemiologic evidence of an increased cancer risk from exposure to these particles in humans is very limited.Citation59,Citation60 However, in the case of long-term exposure to asbestos fibers, especially blue asbestos (crocidolite) in occupational environments, there is positive evidence of increased risk for lung carcinogenesis, multiplying substantially in combination with tobacco smoking because of synergistic effects.Citation61–Citation63
Nanosized durable fibrous materials such as CNTs have raised safety concerns similar to those raised about asbestos fibers. However, the mechanism by which particulates with ultrafine structure cause inflammation and ultimately cancer (eg, malignant mesothelioma and lung cancer) is largely unknown. This is partially because the particulates are not uniform and they vary in a plethora of factors (length, diameter, surface area, density, shape, contaminant metals, and crystallinity). These factors play an important role in particulate toxicity both in vitro and in vivo. Thus, the elicited biological responses from fibrous nanomaterials are very complicated.Citation64
Toxicologists in the last decade have investigated many mechanistic pathways to test the potential for ENPs to initiate inflammation and subsequently to cause cancer, based on data from toxicology of conventional particles and on data accumulated so far. The evidence thus far does suggest that harmful nanoparticles have, in general, the same types of effects at the cellular level as coarse and bulkier particles. Scientists have to make the distinction that ENPs are heterogeneous, like larger particles, in the type of damage they can cause to the respiratory system. Results showed that ENPs have the ability to increase the formation of ROS/RNS and other oxidants, to cause oxidative stress, inflammation and initiation (and promotion) of carcinogenic mechanisms or/and genotoxicity.Citation65–Citation67
Are ENPs capable of initiating biological mechanisms of carcinogenesis?
Currently, there is little evidence as to the carcinogenic effects of ENPs in humans. In addition, there are few recent in vivo studies addressing a cancer endpoint for ENPs. We can examine the stages of mechanisms leading to initiation of carcinogenic processes.
Generation of ROS/RNS by ENPs in the biological cell environment
The first indication of biological damaging effects is the ability of the nanoparticle to generate ROS or RNS in the vicinity of or inside the cell. This is normally the response of airway epithelium to particles entering the lungs through inhalation. The most important oxidative ROS and RNS are the hydroxyl radical (HO•), the superoxide anion (O2•−), alkoxyl radical (RO•), hydrogen peroxide (H2O2), and the peroxynitrite O=ONO−. They can cause oxidative damage to lipid membranes, protein–enzymes, and cellular or mitochondrial DNA (cDNA, mtDNA).Citation68,Citation69
Past studies show that respiratory exposure to conventional PM is thought to impact on genotoxicity as well as on cell proliferation via the PM’s ability to generate ROS and RNS. Although evidence suggests that ROS/RNS mediate particle-induced genotoxicity and mutagenesis, there is a long process leading to neoplastic changes.Citation70 Experiments showed that ROS could arise indirectly due to the effects of internalized particles on the mitochondrial respiration system or in depletion of antioxidant species within the cell. Studies with nanoparticles (nano/ultrafine, cerium oxide, and hematite nanoparticles) showed that they have the potential to damage lung cells, leading to lung cancer, as well as to cause lipid peroxidation, depletion of glutathione levels after 72 hours’ exposure to MRC-5 lung fibroblast cells.Citation70–Citation72
Biokinetic mechanisms of ENPs in the body
Understanding the biokinetics of ENPs in biological systems can provide important information on internal doses to secondary organs and is essential to designing and interpreting in vitro toxicity studies. Unlike free or loosely bound molecules, the transport, accumulation, transformation, and clearance of nanomaterials in the human body is intimately associated with physical form as well as chemical composition.Citation73–Citation76
Inhalation studies using iridium nanoparticles (15 and 80 nm) have demonstrated the translocation of inhaled particles to extrapulmonary organs.Citation77 Of particular concern from experimental studies has been the nature of interactions between nanoparticles and the central nervous system.Citation78 Experiments showed that inhaled nanoparticles can translocate to the central nervous system via olfactory neurons following nasal deposition and induce significant inflammation-related effects. This transport route is unique to nanometer-scale particles and raises the possibility of previously unidentified organ-specific doses and responses.Citation79,Citation80
Mechanisms of oxidative stress by ENPs at the cellular level
The generation of ROS/RNS at the cellular level by ENPs subsequently causes oxidative damage to important biomolecules and depletion of antioxidant enzymes and small molecular-weight antioxidants (eg, ascorbic acid, vitamin E). The extent to which ROS accumulate is determined by the antioxidative system, which enables organisms to maintain proteins and other cellular components in an active state for metabolism. Redox homeostasis is governed by the presence of large pools of these antioxidants that buffer reductants and oxidants. Redox signal transduction is another universal feature of aerobic life honed through evolution to balance information from metabolism and the environment. Oxidative stress in aerobic life is the result of an imbalance between the production of ROS and a biological system’s ability to detoxify the reactive intermediates or to repair the resulting damage.Citation81–Citation83
The scientific literature already contains experimental data showing that ENPs can generate ROS, which overwhelm antioxidant defenses in the cellular environment and, as a consequence, develop oxidative stress. This is considered a valid mechanism for toxicity studies of ENPs.Citation84–Citation86
It has been shown experimentally that water-soluble fullerenes, TiO2 nanoparticles, CNTs, and many other ENPs induce the production of ROS, lipid peroxidation, and oxidative stress, as well as generation of proinflammatory response in tissue cultures and in animal studies. Inevitably, respiratory oxidative stress is very prominent in inhalation studies of ENPs. The relative importance of oxidative stress lies in the ability to mediate to a number of active processes in the cells, such as apoptosis, DNA adduct formation and pro-inflammatory gene expression. Nanosized particles have high efficiency for deposition in the upper and lower regions of the respiratory tract and can be retained in the lungs for a long period of time. Potentially, ENPs can induce oxidative stress and cause greater inflammatory effects than their fine-sized equivalents.Citation87–Citation90
Lung inflammation as a result of oxidative stress by ENPs
Scientific evidence has shown that there is a close connection between oxidative stress in the cell and the elicitation of an inflammatory response via pro-inflammatory gene transcription. Many studies have reported pro-inflammatory effects of ENPs at various doses on pro-inflammatory gene expression in the cell. The redox-responsive nuclear factor (NF)-κB and activator protein (AP)-1 transcription factors have also been reported to be activated in mononuclear phagocytes (MNP)-exposed cells.Citation91–Citation93 In addition, numerous studies report the induction of inflammation itself in the lungs following deposition of ENPs by intratracheal installation or inhalation exposures.Citation94
Are ENPs potential carcinogenic or/and genotoxic agents?
The generation of ROS, oxidative stress, and inflammation by ENPs inevitably raises the question of carcinogenicity and/or genotoxicity. If nanoparticles can directly interact with the cellular DNA and cause mutagenic-type damage (oxidative, strand breaks, lesions, adducts, etc), they can promote mechanisms of carcinogenesis.
Some of the studies of genotoxicity of ENPs were focused on transition metals, which are the largest source of nanomaterials. The nanosize of these metallic particles means they are easily transported into biological systems, thus raising the question of their toxic and carcinogenic effects on the susceptible systems. Scientists suggest that, although advances have been made and insights have been gained on the effect of ENPs with transitional metals (Co, Ni, Cu), there remains much ground to be covered, particularly with respect to our knowledge on their genotoxic and carcinogenic effects.Citation95
A recent review (2011) compared in vitro genotoxicity studies for inorganic nanomaterials with occupational exposure studies of carcinogenicity and epidemiological data. Nearly all types of nanomaterials and control dusts used in the in vitro assays showed genotoxic effects in cell cultures, such as cobalt-chromium (Co-Cr) particles, diesel soot, SiO2 (crystalline and amorphous), TiO2, carbon black; however, this was not consistent in all studies. The overall results of these studies showed that only about 50% were positive.Citation96
Nanoparticles with reactive surfaces may undoubtedly generate inflammation more readily, and inflammation could be sufficiently intense to lead to secondary carcinogenesis via the oxidants that can damage important biomolecules and mitogens produced during inflammation. There is some evidence in vitro that ENPs can penetrate cellular membranes and gain access to the genetic material of the nucleus. Recent studies also suggest some similarities between the pathogenic properties of multi-walled CNTs and those of asbestos fibers. CNTs can cause genetic aberrations by a primary mechanism additional to the inflammation-mediated mechanism.Citation97–Citation99
A review (2009) examined a number of studies investigating the carcinogenic potential of ENP materials, such as TiO2, carbon black, CNT, and fullerenes, to the lung of female rats. CNT-induced mesotheliomas were applied intraperitoneally in rats and mice. Data for fullerenes (C60) were insufficient to evaluate carcinogenic risk. Sub-chronic toxicity data indicated that, in general, nanoparticles form aggregates and agglomerates and cause foreign body reactions at their applied sites with inflammatory cells, including macrophage infiltration.Citation100 These findings are similar to those regarding the biological effects of asbestos, a potent carcinogen, and indicate that careful risk assessment of ENPs is still in the phase of in vivo experimentation. Therefore, it will take a number of years to prove carcinogenic risk to humans and to allow for extensive epidemiological studies.Citation101–Citation103
Metal and metal oxide nanoparticles have a prominent position in the engineered nanoproducts, and there is a wide range of applications for a variety of ENMs. Inevitably, research on their cytotoxicity and carcinogenic potential is considered very important, as is their potential for adverse health effects and safety problems. A recent review (2012) summarized genotoxic effects of metal nanoparticles from recent scientific literature. The studies indicated that some types of metal nanoparticles can cause both DNA strand breaks and chromosomal damages in experimental animals. However, their genotoxic effects depend not only on particle size, surface modification (particle coating), and exposure route, but also on exposure duration. The reviewers noted that, due to considerable inconsistencies in the results and the lack of standardized test methods, a reliable hazard assessment of metal nanoparticles is still limited.Citation104
Metal oxide nanoparticles that are used in various ENMs are also under investigation for potential exposure and hazards. A 2010 review focused on ZnO nanoparticles destined for use in modern sunscreens, and discuss the potential for human exposure and the health hazard at each stage of their manufacture (for exposed workers and consumers who were going to use the sunscreen products). The reviewers examined many research papers but could not reach a firm conclusion as to the hazards; they highlighted a need for further research.Citation105
Gold and silver nanoparticles also have many applications in various ENMs. A 2010 review focused on the safety and evaluation of toxicity associated with human exposure to silver and gold nanoparticles, due to the relative abundance of toxicity data available for these particles. From past studies, it is known that inflammatory, oxidative, genotoxic, and cytotoxic consequences are associated with silver particulate exposure. The primary site of gold and silver particulate accumulation has also been demonstrated to be the liver (in vitro studies). However, in general, there is a lack of in vivo and in vitro toxicity information that allows correlations between the findings to be made. Instead, a focus on the tissue distribution of particles following exposure is evident within the available literature.Citation106
Despite the uncertainties or mixed results of the in vivo studies, the findings to date on the carcinogenic potential of nanomaterials must be taken seriously, and precautionary measures to minimize exposure should go hand in hand with the development of a comprehensive and conclusive toxicological methodology and testing procedure for nanostructured materials that includes all possible exposure routes. Furthermore, criteria for evaluating nano-specific carcinogenic properties should be constantly updated and adapted to the state of knowledge. There is a need here for amendments to be made to EU legislation, as currently various ENMs and certain types of nanoparticles do not represent a separate category of substance in their own right.Citation107
Conclusion
The nanotechnology field is a fast-growing technological field with significant breakthroughs, and several products are commercially available. Studies in vitro and in vivo, and past research on superfine particles in the last decade, have shown that the toxicological data for ENPs, although are very incomplete until now, are adequate to alert scientists as to future health and safety problems for workers and consumers. In particular, inhaled nanoparticles can cause extensive oxidative damage and inflammation of the respiratory system and end up in the bloodstream or the nervous system. ENPs are so tiny that small quantities could have major toxic effects in the respiratory system. Despite the uncertainties, many scientists suggest that the findings to date on the carcinogenic potential of nanomaterials must be taken seriously. H&S authorities must develop comprehensive toxicological methodology and testing procedures for ENPs. Criteria for evaluating the carcinogenic properties of ENPs should constantly be updated and adapted to the state of knowledge. There is a need for amendments to be made to legislation in the EU and in developed countries to cover specific H&S problems of ENPs and the commercial applications of nanoproducts.
Disclosure
The authors report no conflicts of interest in this work.
References
- bcc Research. Nanotechnology [webpage on the Internet]WellesleyBCC Research LLC2013 Available from: http://www.bccresearch.com/market-research/nanotechnology/Accessed October 17, 2013
- RocoMCThe long view of nanotechnology development: the National Nanotechnology Initiative at 10 yearsJ Nanopart Res2011132427445
- DrexlerKEEngines of Creation: The Coming Era of NanotechnologyNew YorkAnchor Books/Doubleday1986
- JohalMSUnderstanding NanomaterialsBoca Raton (FL)CRC Press (Taylor and Francis Group)2011
- OzinGAArsenaultACademartiriLNanochemistry: A Chemical Approach to Nanomaterials 2nd revised edCambridgeRSC Publishing2008
- MulhallDOur Molecular Future: How Nanotechnology, Robotics, Generics, and Artificial Intelligence Will Transform Our WorldAmherst (NY)Prometheus Books2002
- OchsnerAShokuhfarANew Frontiers of Nanoparticles and Nanocomposite Materials: Novel Principles and TechniquesNew YorkSpringer2013
- FosterLENanotechnology: Science, Innovation, and OpportunityNew YorkPrentice Hall2005
- Department of Health and Human Services, Centers for Disease Control and Prevention, National Institute for Occupational Safety and HealthCurrent Intelligence Bulletin 65Occupational Exposure to Carbon Nanotubes and NanofibersWashington, DCNIOSH2013 Available from: http://www.cdc.gov/niosh/docs/2013-145/pdfs/2013-145.pdfAccessed September 30, 2013
- Department of Health and Human Services, Centers for Disease Control and Prevention, National Institute for Occupational Safety and HealthProgress Toward Safe Nanotechnology in the Workplace: A Report from the NIOSH Nanotechnology Research CenterCincinnati (OH)NIOSH2007 Available from: http://www.cdc.gov/niosh/docs/2007-123/pdfs/2007-123.pdfAccessed September 30, 2013
- Kosk-BienkoJEuropean Agency for Safety and Health at WorkWorkplace Exposure to NanoparticlesBilbaoEU-OSHA2009 Available from: https://osha.europa.eu/en/publications/literature_reviews/workplace_exposure_to_nanoparticlesAccessed September 30, 2013
- Del CastilloAMPNanomaterials and Workplace Health and Safety: What Are the Issues for Workers?BrusselsETUI Publications (The European Trade Union Institute)2013
- ValavanidisAFiotakisKVlachogianniTAirborne particulate matter and human health: toxicological assessment and importance of size and composition of particles for oxidative damage and carcinogenic mechanismsJ Environ Sci Health C Environ Carcinog Ecotoxicol Rev200826433936219034792
- MøllerPFolkmannJKForchhammerLAir pollution, oxidative damage to DNA, and carcinogenesisCancer Lett20082661849718367322
- MøllerPJacobsenNRFolkmannJKRole of oxidative damage in toxicity of particulatesFree Radic Res201044114619886744
- LiNXiaTNelAEThe role of oxidative stress in ambient particulate matter-induced lung diseases and its implications in the toxicity of engineered nanoparticlesFree Radic Biol Med2008441689169918313407
- ValavanidisAVlachogianniTFiotakisKTobacco smoke: involvement of reactive oxygen species and stable free radicals in mechanisms of oxidative damage, carcinogenesis and synergistic effects with other respirable particlesInt J Environ Res Public Health20096244546219440393
- ValavanidisAVlachogianniTFiotakisKLoridasSPulmonary oxidative stress, inflammation and cancer: respirable particulate matter, fibrous dusts and ozone as major causes of lung carcinogenesis through reactive oxygen species mechanismsInt J Environ Res Public Health20131093886390723985773
- Nanotechnology and nanomaterials research [webpage on the Internet]United States Environmental Protection Agency (EPA)2013 Available from: http://www.epa.gov/nanoscience/Accessed July 31, 2013
- US Food and Drug AdministrationFDA issues two draft guidances related to nanotechnology applications in cosmetics and food substances [webpage on the Internet] Available from: http://www.fda.gov/ScienceResearch/SpecialTopics/Nanotechnology/ucm301093.htmAccessed October 17, 2013
- OECD Database on Research into the Safety of Manufactured Nano-materials [database on the Internet]ParisOrganisation for Economic Co-operation and Development (OECD)2013 Available from: http://www.oecd.org/science/nanosafety/ (Users can access the database through an internet interface at: http://webnet.oecd.org/NanoMaterials)Accessed August 31, 2013
- Organisation for Economic Co-operation and Development (OECD)Current Developments in Delegations on the Safety of Manufactured Nanomaterials – Tour de TableSeries on the Safety of Manufactured Nanomaterials. No. 37ParisOECD Publications2132013 Available from: http://search.oecd.org/officialdocuments/displaydocumentpdf/?cote=env/jm/mono(2013)2&doclanguage=enAccessed October 17, 2013
- FahmyBDingLYouDLomnickiSDellingerBCormierSAIn vitro and in vivo assessment of pulmonary risk associated with exposure to combustion generated fine particlesEnviron Toxicol Pharmacol201029217318220369027
- KellyFJFussellJCSize, source and chemical composition as determinants of toxicity attributable to ambient particulate matterAtmos Environ201260504526
- AustAECookPMDodsonRFMorphological and chemical mechanisms of elongated mineral particle toxicitiesJ Toxicol Environ Health B Crit Rev2011141–4407521534085
- TerzanoCDi StefanoFContiVGrazianiEPetroianniAAir pollution ultrafine particles: toxicity beyond the lungEur Rev Med Pharmacol Sci2010141080982121222367
- KreylingWGSemmler-BehnkeMMöllerWUltrafine particle-lung interactions: does size matter?J Aerosol Med2006191748316551218
- HopkePKRossnerAExposure to airborne particulate matter in the ambient, indoor, and occupational environmentsClin Occup Environ Med20065474777117110290
- SametJMDominiciFCurrieroFCCoursacIZegerSLFine particulate air pollution and mortality in 20 US cities, 1987–1994N Engl J Med2000343241742174911114312
- MehtaSShinHBurnettRNorthTCohenAJAmbient particulate air pollution and acute lower respiratory infections: a systematic review and implications for estimating the global burden of diseaseAir Qual Atmos Health201361698323450182
- KnaapenAMBormPJAlbrechtCSchinsRPInhaled particles and lung cancer. Part A: mechanismsInt J Cancer2004109679980915027112
- BormPJSchinsRPAlbrechtCInhaled particles and lung cancer, part B: paradigms and risk assessmentInt J Cancer2004110131415054863
- YingZKampfrathTThurstonGAmbient particulates alter vascular function through induction of reactive oxygen and nitrogen speciesToxicol Sci20091111808819182107
- FubiniBHubbardAReactive oxygen species (ROS) and reactive nitrogen species (RNS) generation by silica in inflammation and fibrosisFree Radic Biol Med200334121507151612788471
- ChuangHCChengYLLeiYCChangHHChengTJProtective effects of pulmonary epithelial lining fluid on oxidative stress and DNA single-strand breaks caused by ultrafine carbon black, ferrous sulphate and organic extract of diesel exhaust particlesToxicol Appl Pharmacol2013266332933423261976
- Van BerloDHullmannMSchinsRPFToxicology of ambient particulate matterEXS201210116521722945570
- GambleJLung cancer and diesel exhaust: a critical review of the occupational epidemiology literatureCrit Rev Toxicol201040318924420156057
- ChenHGoldbergMSVilleneuvePJA systematic review of the relation between long-term exposure to ambient air pollution and chronic diseasesRev Environ Health200823424329719235364
- GreenFHVallyathanVHahnFFComparative pathology of environmental lung disease: an overviewToxicol Pathol200735113614717325982
- RodunerESize matters: why nanomaterials are differentChem Soc Rev200635758359216791330
- AlbaneseATangPSChanWCThe effect of nanoparticle size, shape, and surface chemistry on biological systemsAnnu Rev Biomed Eng20121411622524388
- BuzeaCPachecoIIRobbieKNanomaterials and nanoparticles: sources and toxicityBiointerphases200724MR17MR7120419892
- NelAEMädlerLVelegolDUnderstanding biophysicochemical interactions at the nano-bio interfaceNature Mater20098754355719525947
- VermaAStellacciFEffect of surface properties on nanoparticle–cell interactionsSmall201061122119844908
- TsujiJSMaynardADHowardPCResearch strategies for safety evaluation of nanomaterials, part IV: risk assessment of nanoparticlesToxicol Sci2006891425016177233
- JiangWKimBYRutkaJTChanWCNanoparticle-mediated cellular response is size-dependentNat Nanotechnol20083314515018654486
- BormPKlaessigFCLandryTDResearch strategies for safety evaluation of nanomaterials, part V: role of dissolution in biological fate and effects of nanoscale particlesToxicol Sci2006901233216396841
- BrunnerTJWickPManserPIn vitro cytotoxicity of oxide nanoparticles: comparison to asbestos, silica, and the effect of particle solubilityEnviron Sci Technol200640144374438116903273
- KarlssonHLCronholmPGustafssonJMöllerLCopper oxide nanoparticles are highly toxic: a comparison between metal oxide nanoparticles and carbon nanotubesChem Res Toxicol20082191726173218710264
- SongWZhangJGuoJZhangJRole of the dissolved zinc ion and reactive oxygen species in cytotoxicity of ZnO nanoparticlesToxicol Lett2010199338939720934491
- MillerRJBennettSKellerAAPeaseSLenihanHSTiO2 nanoparticles are phototoxic to marine phytoplanktonPLoS One201271e3032122276179
- WinnikFMMaysingerDQuantum dot cytotoxicity and ways to reduce itAcc Chem Res201346367268022775328
- AdamsLKLyonDYAlvarezPJComparative eco-toxicity of nanoscale TiO2, SiO2, and ZnO water suspensionsWater Res200640193527353217011015
- ZhuXZhuLDuanZQiRLiRLangYComparative toxicity of several metal oxide nanoparticle aqueous suspensions to Zebrafish (Danio rerio) early developmental stageJ Environ Sci Health A Tox Hazard Subst Environ Engin2008433278284
- KumarVKumariAGuleriaPYadavSKEvaluating the toxicity of selected types of nanochemicalsRev Environ Contam Toxicol20122153912122057930
- DonaldsonKPolandCAInhaled nanoparticles and lung cancer: what we can learn from conventional particle toxicologySwiss Med Wkly2012142w1354722714122
- OberdörsterGOberdörsteEOberdörsterJNanotoxicology: an emerging discipline evolving from studies of ultrafine particlesEnviron Health Perspect2005113782383916002369
- OberdörsterGSafety assessment for nanotechnology and nano-medicine: concepts of nanotoxicologyJ Intern Med201026718910520059646
- DahmannDTaegerDKapplerMAssessment of exposure in epidemiological studies: the example of silica dustJ Expo Sci Environ Epidemiol200818545246118059424
- ValbergPALongCMSaxSNIntegrating studies on carcinogenic risk of carbon black: epidemiology, animal exposures, and mechanism of actionJ Occup Environ Med200648121291130717159645
- BerryGGibbsGWAn overview of the risk of lung cancer in relation to exposure to asbestos and of taconite minersRegul Toxicol Pharmacol200852Suppl 1S218S22217998152
- ValavanidisABalomenouHMacropoulouHZarodimosIA study of the synergistic interaction of asbestos fibers with cigarette tar extracts for the generation of hydroxyl radicals in aqueous buffer solutionFree Radic Biol Med19962068538588728034
- MossmanBTKampDWWeitzmanSAMechanisms of carcinogenesis and clinical features of asbestos-associated cancersCancer Invest19961454664808816862
- NagaiHToyokuniSBiopersistent fiber-induced inflammation and carcinogenesis: lessons learned from asbestos toward safety of fibrous nanomaterialsArch Biochem Biophys201050211720599674
- SeatonATranLAitkenRDonaldsonKNanoparticles, human health hazard and regulationJ R Soc Interface20097Suppl 1S119S12919726441
- AitkenRJBormPDonaldsonNanoparticles – one word: a multiplicity of different hazardsNanotoxicology200934263264
- BalbusJMMaynardADColvinVLMeeting report: hazard assessment for nanoparticles – report from an interdisciplinary workshopEnviron Health Perspect2007115111654165918007999
- MartinLDKrunkoskyTMDyeJAThe role of reactive oxygen and nitrogen species in the response of airway epithelium to particulatesEnviron Health Perspect1997105Suppl 5130113079400742
- FubiniBHubbardAReactive oxygen species (ROS) and reactive nitrogen species (RNS) generation by silica in inflammation and fibrosisFree Radic Biol Med200334121507151612788471
- LiaoCMChioCPChenWYLung cancer risk in relation to traffic-related nano/ultrafine particle-bound PAHs exposure: a preliminary probabilistic assessmentJ Hazard Mater20111901–315015821458918
- CulcasiMBenameurLMercierAEPR spin trapping evaluation of ROS production in human fibroblasts exposed to cerium oxide nanoparticles: evidence for NADPH oxidase and mitochondrial stimulation20121993161176
- RaduMMunteanuMCPetracheSDepletion of intracellular glutathione and increased lipid peroxidation mediate cytotoxicity of hematite nanoparticles in MRC-5 cellsActa Biochim Pol201057335536020835408
- MöllerWFeltenKSommererKDeposition, retention, and translocation of ultrafine particles from the central airways and lung peripheryAm J Respir Crit Care Med2008177442643217932382
- CreutzenbergOBiological interactions and toxicity of nanomaterials in the respiratory tract and various approaches of aerosol generation for toxicity testingArch Toxicol20128671117112222418596
- LiuYGaoYZhangLPotential health impact on mice after nasal instillation of nanosized copper particles and their translocation in miceJ Nanosci Nanotechnol20099116335634319908531
- ZhuMTFengWYWangYParticokinetics and extrapulmonary translocation of intratracheally instilled ferric oxide nanoparticles in rats and the potential health risk assessmentToxicol Sci2009107234235119023088
- KreylingWGSemmler-BehnkeMSeitzJSize dependence of the translocation of inhaled iridium and carbon nanoparticle aggregates from the lung of rats to the blood and secondary target organsInhal Toxicol200921Suppl 15560
- YangZLiuZWAllakerRPA review of nanoparticle functionality and toxicity on the central nervous systemJ R Soc Interface20107Suppl 4S411S42220519209
- OberdösterEManufactured nanomaterials (fullerenes, C60) induce oxidative stress in the brain of juvenile largemouth bassEnviron Health Perspect2004112101058106215238277
- ElderAGeleinRSilvaVTranslocation of inhaled ultrafine manganese oxide particles to the central nervous systemEnviron Health Perspect200611481172117816882521
- DaviesKJOxidative stress: the paradox of aerobic lifeBiochem Soc Symp1995611318660387
- JonesDPRedefining oxidative stressAntioxid Redox Signal200689–101865187916987039
- JonesDPRadical-free biology of oxidative stressAm J Physiol Cell Physiol20082954C849C86818684987
- XiaTKovochichMBrantJComparison of the abilities of ambient and manufactured nanoparticles to induce cellular toxicity according to an oxidative stress paradigmNano Lett2006681794180716895376
- von MoosNSlaveykovaVIOxidative stress induced by inorganic nanoparticles in bacteria and aquatic microalgae: state of the art and knowledge gapsNanotoxicology Epub6192013
- KimSRyuDYSilver nanoparticle-induced oxidative stress, genotoxicity and apoptosis in cultured cells and animal tissuesJ Appl Toxicol2013332788922936301
- MocanTClichiciSAgoşton-ColdeaLImplications of oxidative stress mechanisms in toxicity of nanoparticles (review)Acta Physiol Hung201097324725520843763
- LiNXiaTNelAEThe role of oxidative stress in ambient particulate matter-induced lung diseases and its implications in the toxicity of engineered nanoparticlesFree Radic Biol Med20084491689169918313407
- MadlAKPinkertonKEHealth effects of inhaled engineered and incidental nanoparticlesCrit Rev Toxicol200939862965819743943
- DonaldsonKAitkenRTranLCarbon nanotubes: a review of their properties in relation to pulmonary toxicology and workplace safetyToxicol Sci200692152216484287
- ChoWSDuffinRPolandCADifferential pro-inflammatory effects of metal oxide nanoparticles and their soluble ions in vitro and in vivo; zinc and copper nanoparticles, but not their ions, recruit eosinophils to the lungsNanotoxicology201261223521332300
- MrozRMSchinsRPLiHDrostEMMacneeWDonaldsonKNanoparticle carbon black driven DNA damage induces growth arrest and AP-1 and NFkappaB DNA binding in lung epithelial A549 cell lineJ Physiol Pharmacol200758Suppl 5(Pt 2)46147018204159
- LiRNingZMajumdarRUltrafine particles from diesel vehicle emissions at different driving cycles induce differential vascular pro-inflammatory responses: implication of chemical components and NF-kappaB signalingPart Fibre Toxicol20107620307321
- ShvedovaAAKisinEMurrayARInhalation vs aspiration of single-walled carbon nanotubes in C57BL/6 mice: inflammation, fibrosis, oxidative stress, and mutagenesisAm J Physiol Lung Cell Mol Physiol20082954L552L56518658273
- MagayeRZhaoJBowmanLDingMGenotoxicity and carcinogenicity of cobalt-, nickel- and copper-based nanoparticlesExp Ther Med20124455156123170105
- RollerMIn vitro genotoxicity data of nanomaterials compared to carcinogenic potency of inorganic substances after inhalational exposureMutat Res20117273728521458593
- BonnerJCCarbon nanotubes as delivery systems for respiratory disease: do the dangers outweigh the potential benefits?Expert Rev Respir Med20115677978722082164
- StellaGMCarbon nanotubes and pleural damage: perspectives of nanosafety in the light of asbestos experienceBiointerphases201162P1P1721721837
- ShvedovaAAKisinERPorterDMechanisms of pulmonary toxicity and medical applications of carbon nanotubes: two faces of Janus?Pharmacol Ther2009121219220419103221
- TsudaHXuJSakaiYFutakuchiMFukamachiKToxicology of engineered nanomaterials: a review of carcinogenic potentialAsian Pac J Cancer Prev200910697598020192569
- SakamotoYNakaeDFukumoriNInduction of mesothelioma by a single intrascrotal administration of multi-wall carbon nanotube in intact male Fischer 344 ratsJ Toxicol Sci2009341657619182436
- SeraNTokiwaHMiyataNMutagenicity of the fullerene C60-generated singlet oxygen dependent formation of lipid peroxidesCarcinogenesis19961710216321698895484
- TakagiAHiroseANishimuraTInduction of mesothelioma in p53+/− mouse by intraperitoneal application of multi-wall carbon nanotubeJ Toxicol Sci200833110511618303189
- KlienKGodnić-CvarJGenotoxicity of metal nanoparticles: focus on in vivo studiesArh Hig Rada Toksikol201263213314522728795
- OsmondMJMcCallMJZinc oxide nanoparticles in modern sunscreens: an analysis of potential exposure and hazardNanotoxicology201041154120795900
- JohnstonHJHutchisonGChristensenFMPetersSHankinSStoneVA review of the in vivo and in vitro toxicity of silver and gold particulates: particle attributes and biological mechanisms responsible for the observed toxicityCrit Rev Toxicol201040432834620128631
- BeckerHHerzbergFSchulteAKolossa-GehringMThe carcinogenic potential of nanomaterials, their release from products and options for regulating themInt J Hyg Environ Health2011214323123821168363