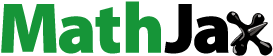
Abstract
Background
The purpose of this study was to investigate the repeatability of retinal oximetry using slit-lamp adapted ultrahigh-resolution optical coherence tomography (SL-UHR-OCT).
Methods
SL-UHR-OCT was developed and fringe patterns were obtained for a major retinal artery and a major retinal vein. A-scans at the central wavelengths of 805 nm and 855 nm were analyzed for calculating optical density ratios (ODRs), from which the percentage oxygen saturation was calculated. Measurements were made on two occasions for each person. Repeatability and coefficients of repeatability were calculated.
Results
The mean ODRs of the artery were 0.79 ± 0.86 and 0.88 ± 0.97 in sessions 1 and 2, respectively. The mean ODRs of the vein were −0.08 ± 0.69 and 0.14 ± 0.77 between the two sessions, and were significantly lower than that of the artery (P < 0.05). The coefficients of repeatability were 1.44 and 1.81 for the artery and vein, respectively. The mean oxygen saturation of the major retinal artery was 94% ± 45% and 98% ± 51% in sessions 1 and 2, respectively, and the mean oxygen saturation of the major retinal vein was 48% ± 36% and 60% ± 40% between sessions.
Conclusion
Optical coherence tomographic oximetry for evaluating retinal oxygen saturation was subject to variation, although the averaged measurements in repeated sessions were matched. Further work on reducing variation will be needed.
Introduction
Retinal oxygen saturation is a measure of the metabolic state of the retina. Many ocular diseases, including diabetic retinopathy,Citation1–Citation8 glaucoma,Citation9–Citation11 and retinal vein occlusion,Citation12,Citation13 result in altered retinal blood flow and oxygen saturation. A reliable method of measuring retinal oxygen saturation would be beneficial for studying, monitoring, and managing these disorders. Retinal oximetry devices based on spectral image analysis through a fundus camera system have been developed for imaging retinal oxygen saturation. However, commercially available systems have not been used widely in clinics, possibly due to their high cost, lack of US Food and Drug Administration clearance, and insufficient clinical studies to justify their routine use in clinics.Citation14–Citation17 Optical coherence tomography (OCT) is an advanced imaging modality in ophthalmology and provides high-resolution imaging of retinal structures. Kagemann et alCitation18 have demonstrated that spectral data in images captured by ultrahigh-resolution optical coherence tomography (UHR-OCT) provide useful information for retinal oximetry. They detected a statistically significant difference in oxygen saturation between the major retinal artery and the major retinal vein. The measurement of oxygen saturation was based on the principle of differences in the absorption spectra for oxyhemoglobin and deoxyhemoglobin.Citation18,Citation19 Their experiments demonstrated that spectral data in UHR-OCT might greatly extend the usefulness of OCT, and therefore, UHR-OCT-based retinal oximetry may be an alternative to fundus camera-based retinal oximetry. The advantage of UHR-OCT retinal oximetry is the simultaneous imaging of the retinal structure and measurement of retinal oxygen saturation. This extension of the capabilities of OCT has the potential to improve further both ophthalmic research and patient care. Before extensive trials of OCT oximetry can be undertaken, it is necessary to determine how repeatable the measurements are. There is no information available on repeatability using OCT oximetry. We have adapted slit-lamp ultrahigh-resolution optical coherence tomography (SL-UHR-OCT) for facilitating retinal imaging.Citation20 With the development of a spectral analysis algorithm for retinal oximetry, the goal of the present study was to assess the feasibility and repeatability of OCT-based retinal oximetry in healthy subjects.
Materials and methods
OCT retinal oximetry was performed using our newly developed SL-UHR-OCT, which was connected to an advanced optical delivery system for retinal imaging, as detailed elsewhere.Citation20 Briefly, the system has approximately 3 μm depth resolution and a computer-controlled fixation target. A light source (T840, Superlum Diodes Ltd, Moscow, Russia) with a central wavelength of 840 nm and a bandwidth of 100 nm was used. Variability of the OCT light power output depends on the light source. The variability was stable and fixed over time. A spectrometer with a charge-coupled device camera collected reflected fringes. In the sample arm, an X-Y galvanometer scanner was mounted on a standard slit-lamp (Reichart, XCEL 250, Depew, NY). The power of the incident light was within the safety standards set by the American National Standards Institute (ANSI Z136.1-2000). To scan the retina, an ocular lens (60D, Volk Optical, Mentor, OH) was placed in front of the eye. The scan speed of the system was set to 24,000 A-scans per second, and the duration of data acquisition for a single dataset was 2.7 seconds. No compensation of eye movement was used. The field of the scan was set to a width of 6 mm, which corresponded to a retinal field of approximately 15–20 degrees. Raster scans were performed to obtain optic disk-centered image frames encompassing a 6 × 6 mm slice of retinal tissue (). Each frame consisted of 512 axial scans with 1365 points/A-scan, taking a total of 128 frames. OCT fundus images (en face view) were reconstructed from the acquired three-dimensional dataset, and the retinal veins and arteries were identified.
Figure 1 OCT images obtained with SL-UHR-OCT. An OCT fundus image that was reconstructed from an OCT dataset (A). The identified vein, artery and tissue were shown on the enlarged fundus image (B); ten fringe images at the locations with marked dots were sampled from the artery, vein and tissue to calculate the optical densities. OCT B-scan image (C) across the sampled range was from the scan marked with the yellow line in image A.

Data processing and calculation of oxygen saturation were similar to that reported in the pioneer work by Kagemann et al.Citation18 To obtain the light intensity at an oxygen-sensitive wavelength (855 nm) as well as the isosbestic wavelength (805 nm), the spectrum of the light source in our UHR-OCT was first calibrated to identify the pixel location of each wavelength on the charge-coupled device. A customized code written in Matlab (MathWorks Inc, version 7.3) was developed to recalculate the light intensity of the A-scans from the sampled unprocessed fringe after subtracting the background. Ten fringe patterns were sampled at the side of the optic nerve head from the edge of a major (first degree) artery, adjacent tissue, and the edge of a major vein. A total of 95 pixels within a 10 nm range of each wavelength of interest (805 nm and 855 nm, respectively) of the OCT light spectrum was sampled (). The sampled data were then processed by resampling, dispersion compensation, fast Fourier transform, and magnitude computation to obtain the intensity profile of the locations. The intensity of the entire A-scan was summed as the light intensity of the wavelength of interest. Optical densities and optical density ratios (ODRs) for each of the 10 measurements were calculated and then averaged. The calculation of optical densities (OD) and ODRs for each vessel was as follows:
Figure 2 Spectrum of the light source of the slit-lamp adapted ultrahigh-resolution optical coherence tomography.

In Equationequation 1(1) ,Iλ and Iλ0 are the average pixel values inside and immediately adjacent, respectively, to a blood vessel when illuminated at wavelength λ. In Equationequation 2
(2) , ODsensitive and ODisosbestic are optical densities at oxygen-sensitive and oxygen-insensitive wavelengths. The percent hemoglobin oxygen saturation (SO2) is linearly proportional to the ODRs, and expressed as follows (Equationequation 3
(3) ):
where a and b are constants. In addition, retinal oxygen use (ODR of the artery-vein) was calculated as the difference between the oxygen saturation of the major retinal artery and the major retinal vein outside the optic nerve head. To calibrate and convert ODR to oxygen saturation, we used a method similar to that previously used by Hardarson et al,Citation15 based on the mean of oxygen saturation for arterioles (96%) and venules (54%). The results of all 20 subjects (two measurements from one eye of each subject) were used. Solving Equationequation 3(3) gave the results of a and b, which were used to convert ODR to oxygen saturation.
This study was approved by the institutional review board of the University of Miami and was conducted in accordance with the tenets of the Declaration of Helsinki and the privacy and security regulations of The Health Insurance Portability and Accountability Act of 1996. Informed consent was obtained from each of the study participants. Twenty normal subjects (15 women and five men, mean age 37.7 ± 9.4 years) were recruited for participation, including 10 Asian subjects, eight Caucasian subjects, and two African-American subjects. Inclusion criteria included best-corrected visual acuity of 20/20 or better, no history of ocular or systematic disease, no history of ocular surgery or laser, and normal appearance of the macula and optic disc. All subjects underwent visual acuity testing, complete slit-lamp biomicroscopic examination, and ophthalmoscopic examination. One randomly selected eye from each subject was scanned twice, with an interval of several minutes between the measurements. During image acquisition, the subjects were asked to place their chin on the chin rest of the slit-lamp and to look at the fixation target. The room lighting was dimmed, and the subject’s pupils were not dilated. OCT images were acquired and analyzed to obtain the results. To standardize the image acquisition procedure, image acquisition was conducted for all the subjects and data analysis was performed by the first author. If apparent artifacts occurred due to eye movement, the imaging procedure was repeated. During imaging alignment, the subject was asked to blink normally then hold the eye open during acquisition.
The data are presented as the mean ± standard deviation. Measurements were compared using the two-tailed paired-samples t-test. P < 0.05 was considered to be statistically significant. The statistical analysis was performed using Statistical Package for the Social Sciences software version 16 (SPSS Inc, Chicago, IL). The methods outlined by Bland and AltmanCitation21 were used to assess the agreement between measurements taken during the two sessions. Coefficients of repeatability of the ODRs were calculated. The coefficient of repeatability is a measure of the 95% limit of agreement, as proposed originally by Bland and Altman,Citation21 and adopted by the International Organization for Standardization; it is calculated as the mean ± 1.96 × the standard deviation of the difference between repeated measurements obtained from the same subject by the same observer.
Results
The retinal image-based datasets of 20 eyes from 20 healthy subjects were successfully acquired using OCT oximetry, and en face views of the scan area were reconstructed (). The mean ODRs of the major retinal artery were 0.79 ± 0.86 and 0.88 ± 0.97 in sessions 1 and 2, respectively, and the mean ODRs of the major retinal vein were −0.08 ± 0.69 and 0.14 ± 0.77 in sessions 1 and 2, respectively (). Mean ODR values for artery-vein were 0.87 ± 1.06 and 0.74 ± 1.14 in sessions 1 and 2, respectively. There were significant differences in the ODRs of the major retinal artery and the major retinal vein in both sessions (P = 0.002, session 1; P = 0.009 session 2). There were no significant differences in the ODRs for the major retinal artery, major retinal vein, and oxygen use (P = 0.581, P = 0.302, and P = 0.621, respectively) between the two sessions. The coefficient of repeatability was 1.44 for the retinal artery and 1.81 for the retinal vein. Intraclass correlation coefficients were calculated to measure the agreement of each measurement. The 95% limit of agreement and intraclass correlation coefficients are shown in .
Table 1 Retinal oxygen saturation measured with ultrahigh-resolution optical coherence tomography (n = 20)
Calibration from 20 eyes for OCT oximetry gave a = 52.3 and b = 52.1, and the conversion equation for oxygen saturation (SO2) is
Mean oxygen saturation of the major retinal artery was 94% ± 45% and 98% ± 51% in sessions 1 and 2, respectively, and the mean ODR of the major retinal vein was 48% ± 36% and 60% ± 40% in sessions 1 and 2, respectively (). There were no significant differences between oxygen saturation in the major retinal artery, the major retinal vein, and oxygen use (P > 0.05) between the two sessions. Bland-Altman plots of difference against mean oxygen saturation of the artery, vein, and artery-vein measurements from the two sessions are shown in .
Figure 3 Bland-Altman plot of the difference between two measurements. The plots of mean artery SO2 (A), vein SO2 (B), and artery-vein SO2 (C) against differences in optical density ratio obtained during two sessions of SO2 measurements in 20 healthy subjects.
Abbreviations: SO2, oxygen saturation; SD, standard deviation.

Discussion
The retina is one of the most active metabolic tissues in the body, and adequate oxygen circulation is necessary for maintaining retinal health. Numerous attempts have been made to develop methods for measuring oxygen availability in the retinal tissue and vasculature. Direct measurements of oxygen pressure in the retina outside the retinal vessels or close to the vessels have been used to determine whether a supply deficit exists.Citation22–Citation24 Some studies have measured oxygen pressure using an oxygen-sensitive electrode.Citation22–Citation25 However, these methods are invasive, so are only used in animal experimentsCitation22,Citation23 or during intraocular surgery in human studies.Citation24 Another way to measure the retinal oxygen supply is based on differences in the absorption spectra of oxygenated and deoxygenated hemoglobin. Several human retinal oximetry devices based on spectral imaging of the fundus with two wavelengths or with multiple wavelengths have been developed.Citation2,Citation14,Citation15,Citation17,Citation26,Citation27 Currently, two systems to measure retinal oxygen saturation are commercially available, ie, a retinal oximeter (Oxymap, ehf, Reykjavik, Iceland)Citation6,Citation12–Citation15,Citation28,Citation29 and a retinal vessel analyzer (DVA, Imedos Systems UG Jena, Germany).Citation30,Citation31 Although oximetry devices are increasingly being used for research, none of these systems are widely available for routine clinical use, possibly due to high costs,Citation14,Citation15,Citation17 lack of clearance by the Food and Drug Administration, and lack of sufficient clinical studies to justify their routine use in clinics.
OCT is used widely in both clinical and research settings.Citation20,Citation32,Citation33 High-resolution images of both the anterior and posterior structures of the eye can be obtained.Citation34,Citation35 New capabilities of functional imaging using OCT have recently been developed.Citation17,Citation32,Citation36 For example, Kagemann et alCitation18 explored the possibility of retinal oximetry using spectral analysis from UHR-OCT retinal images, and Enfield et alCitation36 explored in vivo imaging of the microcirculation of the skin. In the present study, we tested the repeatability of measuring retinal oxygen saturation in healthy subjects with our newly developed SL-UHR-OCT. The approach was based on the work of Kagemann et al.Citation18
In the present study, the light source for SL-UHR-OCT had a 100 nm bandwidth with a central wavelength of 840 nm, which included both an oxygen-sensitive wavelength (855 nm) and an isosbestic wavelength (805 nm). Our work was based on the assumption that there is a distinct difference in the spectrum of arteries and of that in veins, that is due to the fact that arteries and veins have different proportions of oxyhemoglobin and deoxyhemoglobin. As expected, we found a significantly higher oxygen saturation in the artery than in the vein in the healthy group.
Information about the measurement repeatability of a developing method like OCT retinal oximetry is important for calculating appropriate study sample sizes in future studies.Citation37 In the present study, there were obvious variations in artery ODR, vein ODR, and artery-vein ODR. The coefficients of repeatability were 1.44, 1.81, and 2.21 for arterial, venous, and artery-vein ODR values, respectively. However, it should be mentioned that there were no significant differences between the mean ODRs measured in the two sessions. Similar results have been reported by Kagemann et al.Citation18 This phenomenon may indicate a relatively higher variability of this technique, which results in a need for a large sample size for detecting significant changes in oxygen saturation. For example, up to 40 subjects would be needed to detect an increase of 23% in venular oxygen saturation during inhalation of pure oxygen using OCT oximetry,Citation38 according to a software program (Gpower, version 3.0) developed by Erdfelder et al.Citation39 Further improvement will be needed before this method can be used routinely in clinic.Citation38
The standard deviations in this study were larger than those reported in previous studies based on spectral imaging systems for the fundus. Hardarson et alCitation15 reported considerably smaller standard deviations for retinal oxygen saturation in their group as a whole (9% in arteries and 14% in venules) in healthy subjects when they inhaled 21% O2 (ambient air). The standard deviations of five repeated measurements in a group of 10 subjects inhaling room air were 3.7% and 5.3% in the artery and vein measurements, respectively. They also found that data based on wavelengths of 586 nm and 605 nm were more reproducible than data based on 542 nm and 558 nm for artery and vein measurements, respectively. Hammer et alCitation16 used a fundus camera that can record two monochromatic fundus images at 548 nm and 610 nm simultaneously. Arterial and venous oxygen saturations were 98% ± 10.1% and 65% ± 11.7%, respectively. For measurements in the same segments of arteries and veins from five images per subject, standard deviations of 2.52% and 3.25% were found for oxygen saturation in the arteries and veins, respectively. They also found that venous oxygen saturation varied in individuals with different iris colors and that iris color is a good estimate of fundus pigmentation.Citation16
The benefit of the UHR-OCT method for retinal oximetry is that it combines structural and functional imaging, thereby yielding simultaneous information about retinal layer thickness and oximetry. This may be very helpful in studying and correlating retinal lesions with oxygen supply/use. Further developments in three-dimensional mapping of oxygen saturation and microcirculation similar to the work using OCT to image microcirculation in the skin will make the method more useful. The large variability of this method compared with fundus camera-based retinal oximetry may be attributed to the nature of OCT data acquisition. OCT imaging acquires deep structural information in a cross-sectional format. Compared with fundus camera-based retinal oximetry, substantial variations in the OCT method for retinal oximetry were found in the present study and the previous study by Kagemann et al.Citation18 First, a difference in the wavelengths used for spectral analysis may contribute to this large variation. Spectral imaging systems for the fundus are based on visible light over a wavelength range of 540–605 nm, whereas the SL-UHR-OCT used longer wavelengths of light (central wavelength 840 nm). Fundus camera-based oximetry may be affected by deeper layers of the retina, and fundus pigmentation has been found to affect oximetry.Citation14,Citation16 The reason for such a large variation could be due to the ability of OCT light to be more deeply penetrable because it has a longer wavelength. This along with the combination of cross-sectional imaging used in the OCT method can display structures such as the outer retina, outer choroid, and deeper layers hidden underneath retinal blood vessels, and can all be included in the analysis. This inclusion may affect the analysis by inducing more background noise, although there did not appear to be background noise due to shadows cast by the vessels (). Further investigation may be required to address this point by using short wavelengths. Secondly, one of the major differences in normal OCT test-retest results is the change in the location of the beam in the pupil and small eye movements. The OCT scan requires more time (2.7 seconds) than fundus imagery. No compensation for eye movement was used, which may contribute to the large measurement error. Eye movements or blinking may cause uneven illumination during OCT scanning. Third, analysis of OCT light intensity of the vessels was based on an assumption of a linear relationship between OCT fringe recordings and light intensity absorbed at the vessel. This may warrant further investigation. Last, the small size and gender disparity in this study may limit its generalization to a wider population.
Our results using this small sample size are generally in agreement with previously reported findings from Kagemann et al.Citation18 However, there are some noteworthy differences. Our arterial ODR appeared to be lower than theirs. This may be due to racial differences between the study participants that predispose them to having different fundus pigmentations.Citation16 Our healthy control group included 10 Asian subjects, eight Caucasian subjects, and two African-American subjects, whereas Kagemann et alCitation18 did not report the races of their subjects. Interestingly, we obtained ODRs with smaller standard deviations than those in the report by Kagemann et al.Citation18
In conclusion, OCT oximetry for evaluating retinal oxygen saturation was subject to variation, although the averaged measurements in repeated sessions were matched. Further investigation on sources causing variations may improve the method and its use in clinic research. Extending the function of OCT to include measuring oxygen saturation may extend the degree to which OCT is used.
Acknowledgments
This study was in part supported by research grants from the National Institutes of Health (P30EY014801, R01EY020607, R01EY020607S) and from Research to Prevent Blindness.
Disclosure
The authors report no conflicts of interest in this work.
References
- TiedemanJSKirkSESrinivasSBeachJMRetinal oxygen consumption during hyperglycemia in patients with diabetes without retinopathyOphthalmology199810531369442776
- SchweitzerDLaschAvan der VorstSChange of retinal oxygen saturation in healthy subjects and in early stages of diabetic retinopathy during breathing of 100% oxygenKlin Monbl Augenheilkd2007224402410 German17516370
- HickamJBSiekerHOFrayserRStudies of retinal circulation and A-V oxygen difference in manTrans Am Clin Climatol Assoc195971344414401681
- HammerMVilserWRiemerTDiabetic patients with retinopathy show increased retinal venous oxygen saturationGraefes Arch Clin Exp Ophthalmol20092471025103019404666
- HolekampNMShuiYBBeebeDLower intraocular oxygen tension in diabetic patients: possible contribution to decreased incidence of nuclear sclerotic cataractAm J Ophthalmol20061411027103216765670
- HardarsonSHStefanssonERetinal oxygen saturation is altered in diabetic retinopathyBr J Ophthalmol20129656056322080478
- LangeCAStavrakasPLuhmannUFIntraocular oxygen distribution in advanced proliferative diabetic retinopathyAm J Ophthalmol201115240641221723532
- ArdenGBSivaprasadSHypoxia and oxidative stress in the causation of diabetic retinopathyCurr Diabetes Rev2011729130421916837
- OlafsdottirOBHardarsonSHGottfredsdottirMSHarrisAStefanssonERetinal oximetry in primary open-angle glaucomaInvest Ophthalmol Vis Sci2011526409641321715353
- TraustasonSHardarsonSHGottfredsdottirMSDorzolamide-timolol combination and retinal vessel oxygen saturation in patients with glaucoma or ocular hypertensionBr J Ophthalmol2009931064106719416932
- HardarsonSHGottfredsdottirMSHalldorssonGHGlaucoma filtration surgery and retinal oxygen saturationInvest Ophthalmol Vis Sci2009505247525019494205
- HardarsonSHStefanssonEOxygen saturation in branch retinal vein occlusionActa Ophthalmol20119046647021518303
- HardarsonSHStefanssonEOxygen saturation in central retinal vein occlusionAm J Ophthalmol201015087187520875633
- BeachJMSchwenzerKJSrinivasSKimDTiedemanJSOximetry of retinal vessels by dual-wavelength imaging: calibration and influence of pigmentationJ Appl Physiol1999867487589931217
- HardarsonSHHarrisAKarlssonRAAutomatic retinal oximetryInvest Ophthalmol Vis Sci2006475011501617065521
- HammerMVilserWRiemerTSchweitzerDRetinal vessel oximetry-calibration, compensation for vessel diameter and fundus pigmentation, and reproducibilityJ Biomed Opt20081305401519021395
- IzhakyDNelsonDABurgansky-EliashZGrinvaldAFunctional imaging using the retinal function imager: direct imaging of blood velocity, achieving fluorescein angiography-like images without any contrast agent, qualitative oximetry, and functional metabolic signalsJpn J Ophthalmol20095334535119763751
- KagemannLWollsteinGWojtkowskiMSpectral oximetry assessed with high-speed ultra-high-resolution optical coherence tomographyJ Biomed Opt20071204121217867801
- HarrisADinnRBKagemannLRechtmanEA review of methods for human retinal oximetryOphthalmic Surg Lasers Imaging20033415216412665234
- JiangHAbukhalilFShenMSlit-lamp-adapted ultra-high resolution OCT for imaging the posterior segment of the eyeOphthalmic Surg Lasers Imaging201243768122251848
- BlandJMAltmanDGStatistical methods for assessing agreement between two methods of clinical measurementLancet198613073102868172
- CringleSJYuDYOxygen supply and consumption in the retina: implications for studies of retinopathy of prematurityDoc Ophthalmol20101209910919830466
- YuDYCringleSJOxygen distribution and consumption within the retina in vascularised and avascular retinas and in animal models of retinal diseaseProg Retin Eye Res20012017520811173251
- StefanssonEMachemerRde JuanEJrMcCuenBWPetersonJRetinal oxygenation and laser treatment in patients with diabetic retinopathyAm J Ophthalmol199211336381728144
- LinsenmeierRAPadnick-SilverLMetabolic dependence of photoreceptors on the choroid in the normal and detached retinaInvest Ophthalmol Vis Sci2000413117312310967072
- SchweitzerDThammEHammerMKraftJA new method for the measurement of oxygen saturation at the human ocular fundusInt Ophthalmol20012334735311944861
- HammerMLeistritzSLeistritzLSchweitzerDLight paths in retinal vessel oxymetryIEEE Trans Biomed Eng20014859259811341533
- PalssonOGeirsdottirAHardarsonSHOlafsdottirOBKristjansdottirJVStefanssonERetinal oximetry images must be standardized: a methodological analysisInvest Ophthalmol Vis Sci2012531729173322395877
- HardarsonSHBasitSJonsdottirTEOxygen saturation in human retinal vessels is higher in dark than in lightInvest Ophthalmol Vis Sci2009502308231119117923
- HammerMHellerTJentschSRetinal vessel oxygen saturation under flicker light stimulation in patients with non-proliferative diabetic retinopathyInvest Ophthalmol Vis Sci2012264063406822589440
- HammerMVilserWRiemerTRetinal venous oxygen saturation increases by flicker light stimulationInvest Ophthalmol Vis Sci20115227427720671271
- WojtkowskiMHigh-speed optical coherence tomography: basics and applicationsAppl Opt201049D30D6120517358
- HuangDSwansonEALinCPOptical coherence tomographyScience1991254117811811957169
- JancevskiMFosterCSAnterior segment optical coherence tomographySemin Ophthalmol20102531732321091018
- DrexlerWFujimotoJGState-of-the-art retinal optical coherence tomographyProg Retin Eye Res200827458818036865
- EnfieldJJonathanELeahyMIn vivo imaging of the microcirculation of the volar forearm using correlation mapping optical coherence tomography (cmOCT)Biomed Opt Express201121184119321559130
- VieiraSCorrenteJEStatistical methods for assessing agreement between double readings of clinical measurementsJ Appl Oral Sci20111948849221986654
- DeloriFCNoninvasive technique for oximetry of blood in retinal vesselsAppl Opt1988271113112520531526
- ErdfelderEFaulFBuchnerAGPOWER: A general power analysis programBehav Res Methods199628111