Abstract
Aim:
A limited number of studies have attempted to quantify the advantages of using in-house 3D printed models. We designed a pilot study to assess the value of in-house production of patient-specific 3D-printed models in pre-surgical planning.
Methods:
A 12-question survey was designed and sent to surgeons to assess any purported benefits of 3D-printed anatomic models. In-house 3D model printing was completed on a Stratasys J750 Polyjet printer. Each 3D printed model was delivered to the supervising surgeon days before the procedure for final validation. The 3D models were subsequently utilized in the operating room.
Results:
A total of 50 surveys were completed for a response rate of 100%. Approximately 94% of the participants strongly agreed that the 3D-printed model was a valuable clinical tool for pre-surgical planning. The majority of the respondents (78%) reported improved surgical efficiency.
Conclusion:
3D models is a valuable tool for surgical planning.
Three-dimensional (3D) modeling and printing has revolutionized the use of medical imaging in clinical settings. Physicians have traditionally relied on magnetic resonance imaging (MRI) and computed tomography (CT) scans for a more comprehensive understanding of underlying pathophysiology. In the past two decades, additive manufacturing technology has enabled the transformation of digital CT and MRI data into 3D anatomical models that are built up layer by layer [Citation1]. This method relies on the use of computer-aided-design and manufacturing (CAD/CAM) for the rapid prototyping of patient-specific 3D models. The delivery of a patient-specific 3D model generally entails three steps: image acquisition, image processing, and finally 3D printing in an in-house or third-party lab. Image acquisition is initiated by obtaining high quality imaging from either CT, MRI, or 3D surface-imaging modalities [Citation1,Citation2]. Acquired data is then stored in a standardized Digital Imaging and Communications in Medicine (DICOM) format, and subsequently converted to Standard Triangulation Language (STL) files [Citation1,Citation2]. The STL files are then processed using software that enables image segmentation and creation of a 3D mesh model, that eventually becomes the blueprint for the rapid prototyping of a 3D model. As 3D printing protocols have expanded from industry to clinical settings, hospitals have begun to invest in high-definition 3D printers to facilitate quality in-house production of 3D printed models.
3D printed models have been recognized as a beneficial clinical tool for planning and execution of surgical procedures [Citation2]. The use of anatomic models provides a more comprehensive approach to presurgical planning, as the models allow for tactile feedback and visualization of complex anatomy – serving as a useful intraoperative reference [Citation1–5]. The ability to print out a high-resolution 3D representation of an injury, for example, allows surgeons to conduct detailed pre-operative planning and preparation that might be more efficient and accurate than with 2D imaging alone. These patient-specific 3D prototypes can also be used in the operating room to improve the accuracy of implant placement. Previous studies have also shown that 3D models can be used as educational tools to facilitate a better understanding of spatial anatomic relationships and unique variations in anatomy by medical students, surgeons, and radiologists [Citation3–5].
The use of 3D printed models has become increasingly popular in surgical planning for cardiothoracic surgery, neurosurgery, orthopedic, craniomaxillofacial, and reconstructive surgery [Citation6–11]. Some 3D printed models are currently printed in-house, rather than procured from third party labs, which dramatically decreases the cost relative to 3D printing commercial entities. Since the workflow of creating in-house 3D models requires sufficient technical expertise in the use of CAD, most in-house labs have developed a protocol that relies on extensive communication and collaboration between surgeons and biomedical engineers, who are tasked with the technical fabrication of 3D printed models [Citation11]. While engineers focus on the initial image acquisition and processing steps, the supervising surgeons can confirm the accuracy and clinical utility of anatomic designs during the image segmentation steps and immediately after the 3D model has been printed [Citation11]. This process encourages an efficient workflow for subsequent refinements and adjustments to the final product, drawing from the technical and medical expertise of both parties. This in-house 3D printing workflow results in certain benefits such as decreased cost, increased speed of delivery, and improved communication between the surgeon and 3D printing lab [Citation12,Citation13].
While the benefits of using 3D models in surgical planning have been well documented, a limited number of studies have attempted to quantify the benefits of using in-house 3D printed models. We designed a pilot study to assess the value of hospital-based production of patient-specific 3D printed models in presurgical planning. In this preliminary report, we evaluated the benefits of in-house 3D printed models by surveying surgeons about their experience at a single institution. We hypothesize that the 3D models improve pre-operative surgical preparedness and have a beneficial impact on the clinical decisions that govern the management of complex reconstructive cases.
Materials & methods
Fabrication of 3D model protocol
This study was conducted in accordance with the guidelines set forth by the institutional review board at Rhode Island Hospital. A total of fifty surgical procedures requiring a 3D model from the in-house 3D Printing Lab at Rhode Island Hospital were identified and included in this study. Patient CT or MRI scans were acquired as DICOM files. All patient imaging was screened for optimal slice thickness, with required values below 1 mm. Notably, slice thickness exceeding 2 mm has been shown to lead to poor anatomical accuracy of 3D models, with loss of fine anatomical structures such as blood vessels [Citation14]. Acquired images were imported into 3D Slicer v4.10.1 (Surgical Planning Laboratory; Cambridge, MA) for segmentation and mesh model creation in STL format. In-house 3D model printing was completed on a Stratasys J750 Polyjet printer (Stratasys, Eden Prairie, MN). The Stratasys J750 Polyjet printer can produce models in a combination of 500,000 colors with various textures, densities, and transparencies with microscopic layer resolution down to 14 microns [Citation15]. Depending on the surgical case, the surgeon can communicate the desired level of flexibility, toughness, durability, and translucency of the material to the local 3D printing engineer during the design process. Each 3D printed model was delivered to the supervising surgeon several days before the procedure for final validation of anatomical accuracy during presurgical planning. The 3D models were subsequently utilized in the operating room for intraoperative guidance, allowing visualization of accurately scaled anatomical structures and active tactile feedback. demonstrates examples of 3D printing models used in this study. Our 3D models were printed from patient with different facial fractures, hand injuries and spinal disorders and their ages ranged from 2 to 80 years.
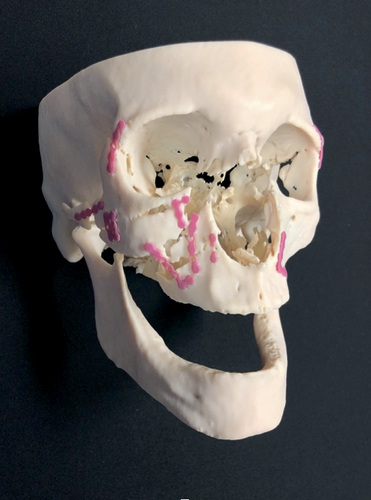
Survey
A cross-sectional, 12 question survey was designed to assess for any purported benefits of 3D printed anatomic models. This survey was given to participating physicians whenever a model was ordered, to evaluate their specific experiences with each case. Each survey was linked to an individual anatomic model. One question asked respondents to identify their surgical specialty, six queries requested respondents to score statements regarding the 3D printed model on a 5-point Likert scale, and five questions prompted a Yes or No response with additional open-ended feedback. Survey data was collected on standardized forms, downloaded into a data management program, and evaluated by the lead authors.
Results
A total of 50 surveys were sent out to four different surgical departments that had requested a 3D printed model through the 3D Printing Laboratory (). A total of 50 surveys were completed for a response rate of 100% by surgeons specializing in plastic surgery (56%), neurosurgery (26%), orthopedic surgery (12%) and interventional cardiology (6%). No subject was excluded. All surgeons were board certified by the American boards of Plastic Surgery, Orthopedic Surgery and Neurosurgery and had >10 years of experience. All of the participants agreed (and 94% strongly agreed) that the 3D printed model was a valuable clinical tool for presurgical planning. Most respondents (92%) strongly agreed that the 3D printed model was more comprehensive of patient anatomy than imaging alone. The remaining 8% were divided between “Agree” and “Neutral” (4% each). All of the participants either strongly agreed (91.6%) or moderately agreed (8.3%) that the 3D model was a valuable clinical tool for intraoperative reference. Most (92%) of the surgeons reported benefits in using the 3D model as a valuable communication tool with patients and an educational tool for trainees, residents, and fellows. The majority of the respondents (78%) endorsed improved surgical efficiency, decreasing total estimated operating time by an average of 59 minutes (standard deviation ±31 min). Approximately 46% of respondents reported that the insights gleaned from using the 3D model reduced the risk of post-operative complications. Finally, in 48% of cases, surgeons documented that the model led to changes in surgical planning. One respondent noted that the 3D model decreased the likelihood of post-operative nerve or artery injury in a spine-fusion procedure. Likewise, another surgeon noted that the use of the anatomic print decreased the chance of aberrant screw placement in a thoracic fusion case. In two reported spinal tumor resection cases, respondents noted that the 3D surgical reference allowed for better visualization of the vertebral artery while anticipating “critical structures in relationship to the tumor”. Some respondents also mentioned that the use of 3D printed models led to some “totally unanticipated” changes in surgical planning, including increasing the extent of dissection at the skull base, changing the access approach to the orbit to account for more severe injuries, and avoidance of an unnecessary coronal incision.
Questions with open-ended feedback revealed that many respondents felt that the use of 3D printed models reduced the likelihood of post-operative complications due to improved visualization of critical anatomy that had not been appreciated with 2D imaging alone.
Discussion
Despite recent advancements in high resolution imaging using CT and MRI data, the interpretation of complex pathophysiology from existing standard of care diagnostic technology remains challenging for even the most experienced surgeons. As 3D printing has become more accessible and affordable, this tool has increased in frequency as a surgical visualization and planning tool among surgical specialties. These developments underscore the importance of assessing the value of in-house 3D printed models during operative procedures. In the New England Journal of Medicine, Porter defined value as health outcomes achieved per dollar spent, emphasizing that achieving high value of care for patients is should be the goal of healthcare delivery [Citation16–18].
Our survey results suggest that there are clear benefits to introducing a hospital-based 3D printing laboratory for surgical subspecialty teams. All participants agreed (94% strongly) that the 3D printed model was a valuable clinical tool for presurgical planning. 92% strongly agreed that the 3D printed model was more comprehensive of patient anatomy than imaging alone. Moreover, all participants either strongly agreed (91.6%) or moderately agreed (8.3%) that the 3D model was a valuable clinical tool for intraoperative reference. Perhaps most importantly, in almost half of the cases (48%), surgeons documented that the model led to changes in surgical planning with examples being decreased risks of nerve or artery injury, more accurate screw placements, or simply just a better visual appreciation pre-operatively (see result section for specific comments). Additionally, the 3D models were also frequently used during presurgical planning discussions with members of the surgical team, including residents and medical students. Our trainees reported enjoying the tactile benefits of handling the 3D printed models during presurgical planning discussion, allowing for a deeper understanding of planned procedures while facilitating a teaching environment that is conducive to an open discussion of anatomical challenges of each specific case. While we had a 100% response rate there is a potential for response bias, as surgeons who found the 3D models particularly beneficial might be more inclined to respond, potentially skewing the results somewhat.
In this study, in-house printed 3D models provided a relatively easy, physical, portable, 3D representation of patient-specific anatomy that optimizes orientation to pathologic structures intraoperatively and provides numerous benefits [Citation3–5]. They allow the surgeon to view critical structures prior to entering the operating room and increase familiarity with complex anatomic relationships, while guiding key surgical steps intraoperatively [Citation6–11]. As represented in , the 3D printed models allow for the visibility of the anatomy surrounding a tumor, fracture, congenital defect, or other pathologies in multiple dimensions. This tool may also contribute additional information beyond what is immediately ascertained from CT or MRI data while providing invaluable tactile feedback. All of these elements may guide operative decision-making in real time [Citation3,Citation4]. With this foundation, 3D printing models arguably provide improved efficiency, safety, and accuracy for surgical outcomes. Since value is defined as outcomes relative to costs [Citation16,Citation19–21], 3D printing models could theoretically increase value of healthcare delivery through its impact on both the efficiency and outcomes of surgical care.
In-house 3D printing offers substantial benefits to traditional commercial models. These commercial products typically take roughly a week to deliver, with a cost of roughly $3000 [Citation22]. Conversely, in-house production is markedly more advantageous as models can be produced over several hours, while the costs are much lower – in the realm of hundreds of dollars [Citation23]. Thus, 3D printing within the hospital setting can be faster, cheaper, with more surgeon control of the product while at the same time potentially providing a model of equal or greater quality [Citation23].
The potential for shorter operative times and improved outcomes may further add to the benefits of an in-house 3D printing lab. Our study shows that surgeons utilizing anatomic models on average saved a total of 59 minutes in the operating room. Although precise cost analysis for OR time is challenging to estimate, previous studies have demonstrated a range of $26–80/min, depending on the hospital setting [Citation17–19]. Given this data, the cost savings using 3D printing model in our institution would range from $1534 to $4720 per procedure.
Based on one cost analysis study, the average amount spent for each in-house 3D printed model was between $119 and $320 [Citation20]. This paper further noted that an in-house 3D printing lab came with an additional fixed cost of $150,000/year ($120,000 for salaries; $20,000 for a segmentation software license; and $10,000 of facilities operational costs, printer maintenance, and unexpected purchases) [Citation20]. Nevertheless, based on the individual hospital’s needs, these costs could be adjusted and lowered. Yet, even if we account for a fixed overall annual cost for operating a 3D printing lab to be $150,000/year, an institution will reach their break-even point after 32 to 98 cases ($150,000/$1534 vs $150,000/$4720), depending on their OR time cost.
Collaborative efforts between the 3D printing laboratory team and the participating surgical subspecialties can enable streamlined production of patient-specific 3D models. An in-house 3D printing service improves accessibility and utilization of additively manufactured models in a clinical setting, resulting in more accurate and personalized care in presurgical planning [Citation21]. Future studies should further focus on the value and benefits of using 3D printed models during presurgical planning and consultations with patients and their families. While 3D virtual reconstructions are relatively common and appreciated during counseling sessions with patients, the ability to present a patient-specific 3D model allows for a more personalized approach. 3D models can be a powerful communication tool that allows the surgeon to set realistic expectations regarding the procedure, explain the steps of the surgery, while also highlighting any possible complications. Future research should focus on developing supplementary quantitative analyses in evaluating the link between the practical outcomes and correlating these benefits to financial aspects of in-house 3D printing.
Conclusion
In this preliminary report, we conclude that nearly all participants found an in-house 3D printed model as a valuable clinical tool for presurgical planning, provided a more comprehensive understanding of patient anatomy than imaging alone, and served as a useful intraoperative reference that in almost half of the cases led to changes in surgical planning. The in-house 3D printed models reduced OR time, improved communication with patients, and increased overall efficiency. Moreover, it also served as a valuable teaching aid for residents and medical students.
Three-dimensional (3D) modeling and printing has revolutionized the use of medical imaging in clinical settings. In the past two decades, additive manufacturing technology has enabled the transformation of digital CT and MRI data into 3D anatomical models that are built up layer by layer.
As 3D printing protocols have expanded from industry to clinical settings, hospitals have begun to invest in high-definition 3D printers to facilitate quality in-house production of 3D printed models.
3D printed models have been recognized as a beneficial clinical tool for planning and execution of surgical procedures. The use of anatomic models provides a more comprehensive approach to presurgical planning, as the models allow for tactile feedback and visualization of complex anatomy – serving as a useful intraoperative reference.
In-house 3D printing capability can not only improve costs but also help with efficacy and speed. Our study showed that there are clear benefits to introducing a hospital-based 3D printing laboratory for surgical subspecialty teams. All participants agreed (94% strongly) that the 3D printed model was a valuable clinical tool for presurgical planning. 92% strongly agreed that the 3D printed model was more comprehensive of patient anatomy than imaging alone. Moreover, all participants either strongly agreed (91.6%) or moderately agreed (8.3%) that the 3D model was a valuable clinical tool for intraoperative reference. Perhaps most importantly, in almost half of the cases (48%), surgeons documented that the model led to changes in surgical planning with examples being decreased risks of nerve or artery injury, more accurate screw placements, or simply just a better visual appreciation preoperatively.
Moreover, our trainees reported enjoying the tactile benefits of handling the 3D printed models during presurgical planning discussion, allowing for a deeper understanding of planned procedures while facilitating a teaching environment that is conducive to an open discussion of anatomical challenges of each specific case.
Financial disclosure
The authors have no financial involvement with any organization or entity with a financial interest in or financial conflict with the subject matter or materials discussed in the manuscript. This includes employment, consultancies, honoraria, stock ownership or options, expert testimony, grants or patents received or pending, or royalties.
Competing interests disclosure
The authors have no competing interests or relevant affiliations with any organization or entity with the subject matter or materials discussed in the manuscript. This includes employment, consultancies, stock ownership or options and expert testimony.
Writing disclosure
No writing assistance was utilized in the production of this manuscript.
References
- Shilo D, Emodi O, Blanc O, Noy D, Rachmiel A. Printing the future-updates in 3D printing for surgical applications. Rambam. Maimonides Med. J. 9(3), e0020 (2018).
- Pugliese L, Marconi S, Negrello E et al. The clinical use of 3D printing in surgery. Updates Surg. 70(3), 381–388 (2018).
- Pujol S, Baldwin M, Nassiri J, Kikinis R, Shaffer K. Using 3D modeling techniques to enhance teaching of difficult anatomical concepts. Acad. Radiol. 23(4), 507–516 (2016).
- Chen S, Pan Z, Wu Y et al. The role of three-dimensional printed models of skull in anatomy education: a randomized controlled trail. Sci. Rep. 7(1), 575 (2017).
- Marconi S, Pugliese L, Botti M et al. Value of 3D printing for the comprehension of surgical anatomy. Surg. Endosc. 31(10), 4102–4110 (2017).
- Werz SM, Zeichner SJ, Berg BI, Zeilhofer HF, Thieringer F. 3D printed surgical simulation models as educational tool by maxillofacial surgeons. Eur. J. Dent. Educ. 22(3), e500–e505 (2018).
- Valverde I, Gomez-Ciriza G, Hussain T et al. Three-dimensional printed models for surgical planning of complex congenital heart defects: an international multicenter study. Eur. J. Cardio-Thoracic Surg. 52(6), 1139–1148 (2017).
- Kappanayil M, Koneti N, Kannan R, Kottayil B, Kumar K. Three-dimensional-printed cardiac prototypes aid surgical decision-making and preoperative planning in selected cases of complex congenital heart diseases: early experience and proof of concept in a resource-limited environment. Ann. Pediatr. Cardiol. 10(2), 117 (2017).
- Manganaro MS, Morag Y, Weadock WJ, Yablon CM, Gaetke-Udager K, Stein EB. Creating three-dimensional printed models of acetabular fractures for use as educational tools. Radiographics 37(3), 871–880 (2017).
- Chou PY, Hallac RR, Shih E et al. 3D-printed models of cleft lip and palate for surgical training and patient education. Cleft. Palate-Craniofacial J. 55(3), 323–327 (2018).
- Segaran N, Saini G, Mayer JL et al. Application of 3D printing in preoperative planning. J. Clin. Med. 10(5), 917 (2021).
- Aimar A, Palermo A, Innocenti B. The role of 3D printing in medical applications: a state of the art. J. Healthc. Eng. 2019, 5340616 (2019).
- Diment LE, Thompson MS, Bergmann JHM. Clinical efficacy and effectiveness of 3D printing: a systematic review. BMJ Open 7(12), e016891 (2017).
- Stana J, Grab M, Kargl R, Tsilimparis N. 3D printing in the planning and teaching of endovascular procedures. Radiologie (Heidelb.) 62(Suppl. 1), 28–33 (2022).
- Boyajian MK, Crozier JW, Woo AS. Introduction of medical three-dimensional printing in Rhode Island. RI Med. J. 102(6), 15–18 (2019).
- Porter ME. What is value in health care? N. Engl. J. Med. 363(26), 2477–2481 (2010).
- Childers CP, Maggard-Gibbons M. Understanding costs of care in the operating room. JAMA Surg. 153(4), e176233 (2018).
- Grossi EA, Moore M, Mallow PJ, Rizzo JA. The cost of an operating room minute for heart valve procedures. J. Health Econ. Outcomes Res. 2(2), 170–180 (2015).
- Macario A. What does one minute of operating room time cost? J. Clin. Anesth. 22(4), 233–236 (2010).
- Ballard DH, Mills P, Duszak R Jr, Weisman JA, Rybicki FJ, Woodard PK. Medical 3D printing cost-savings in orthopedic and maxillofacial surgery: cost analysis of operating room time saved with 3D printed anatomic models and surgical guides. Acad. Radiol. 27(8), 1103–1113 (2020).
- Schlegel L, Ho M, Fields JM et al. Standardizing evaluation of patient-specific 3D printed models in surgical planning: development of a cross-disciplinary survey tool for physician and trainee feedback. BMC Med. Educ. 22, 614 (2022).
- Ravi P, Burch MB, Farahani S et al.; University of Cincinnati 3D Printing Clinical Service Participants. Utility and costs during the initial year of 3D printing in an academic hospital. J. Am. Coll. Radiol. 20(2), 193–204 (2023).
- Lor LS, Massary DA, Chung SA, Brown PJ, Runyan CM. Cost analysis for in-house versus industry-printed skull models for acute midfacial fractures. Plast. Reconstr. Surg. Glob Open 8(5), e2831 (2020).