Abstract
This review will focus on gastrointestinal mucosal protection against cytotoxic agents and cellular stress mainly from the viewpoint of expression and function of heat shock proteins, in their role of ‘molecular chaperones’, as internal cytoprotectants. Also, recently identified target molecules of heat shock protein in damaged gastric mucosal cells are introduced. Elucidation of such stress-responses and repairing process of damaged protein by heat shock proteins in the gastrointestinal mucosa may provide a better understanding for the mechanisms of cytoprotection and cellular repair. In addition, these findings in post-genomic level may provide new strategies for the therapy of gastrointestinal disorders.
Introduction
Many recent reports indicate that heat shock proteins (HSPs), also called molecular chaperones, have important functions in response to stress-related events in a variety of organs including digestive organs Citation[1–7]. Since their structures are conserved from prokaryotic cells to eukaryotic cells, it is considered that they have essential functions for survival of cells and developmental process Citation[1]. Some recent reports have proved the cytoprotective functions of HSPs against environmental stresses, and these functions are considered to be important for living cells to obtain tolerance to adapt for environmental changes Citation[2–7].
Our recent studies have focused on the ‘chaperone’ function of HSPs mainly their cytoprotective functions in digestive organs and cells Citation[5–22]. In this series of studies, we have demonstrated that (1) HSPs are induced by several environmental stresses including heat, neuropeptide, neuroamine or drugs also in vivo; (2) induced HSPs are different even by same stress in each organ; (3) in view of cytoprotection, important HSPs are different in each organ. Also, based on our in vivo findings, chaperone-inducing therapy, which enhances cytoprotective ability, might be a candidate for a new therapeutic tool. Therefore, some of our recent studies are designed to develop chaperone-inducing drugs, including the induction mechanism of HSP by the drug, or chaperone-inducing gene therapy (mainly Hsp70) Citation[12], Citation[22].
Further, in order to obtain important information regarding the post-genomic events during acute mucosal cellular damage, we recently have analysed and identified the target molecules of the Hsp70 family in injured gastric mucosa using affinity chromatography. Since HSP has been known to rapidly bind to degenerated proteins to normalise their structure in vitro, identification of HSP-binding proteins might contribute to finding key molecules for the pathogenesis in many other disorders. In this review, some of these new findings are also introduced and discussed.
Regulation mechanism of heat shock proteins mediated by heat shock transcription factor (HSF)1
Mammals have three different HSFs that have been considered functionally distinct. HSF1 is essential for the heat shock response and is also required for developmental processes, whereas HSF-4 and HSF-2 are important for differentiation and development Citation[23]. Specifically, HSF-4 is involved in corticogenesis and spermatogenesis, and HSF-2 is required for maintenance of sensory organs, such as the lens and the olfactory epithelium Citation[24], Citation[25].
Regulation mechanisms of HSPs are summarised in . Environmental stresses induce heat shock transcription factor (HSF) mainly HSF1. Accumulation of aggregated or denatured proteins in cytosol is thought to be a trigger for the induction of HSF1 as the first step of stress response. Under unstressed conditions, HSF1 is inactivated by binding to Hsp90 or Hsp70. Under stressed conditions, where protein degeneration occurs, Hsp90 and Hsp70 dissociate from HSF1 to bind to degenerated or aggregated proteins for assisting their folding. Thus free HSF1 can be activated, and trimer formation of phosphorylated HSF1 allowed this molecule to obtain ability to move into the nucleus and to bind to the promoter region (HSE). Thus HSP genes are activated Citation[26], Citation[27].
Figure 1. (A) Regulation mechanism of HSP. Environmental stresses (stressor) induce heat shock transcription factor (HSF), mainly HSF1. Under unstressed conditions, HSF1 binds to Hsp90 or Hsp70, and HSF1 is inactivated. Under stressed condition, Hsp90 or Hsp70 dissociates from HSF1 to bind to degenerated proteins for assistance of their folding. Free HSF1 can be activated followed by trimerisation and phosphorylation, and obtains ability to move into the nucleus to bind to HSE. (B) Hsp70 induction by drug (GGA). The heat shock transcription factor HSF1 is suppressed by binding of constitutively expressed Hsp70 (HSC70) through its C-domain in the cytosol under normal conditions. GGA binds to the C-domain of the Hsp70, resulting in dissociation of the Hsp70 from HSF1. Then HSF1 is activated and the trimers can be formed. The activated HSF1 moves into the nucleus from the cytosol and HSF1 binds to HSE.
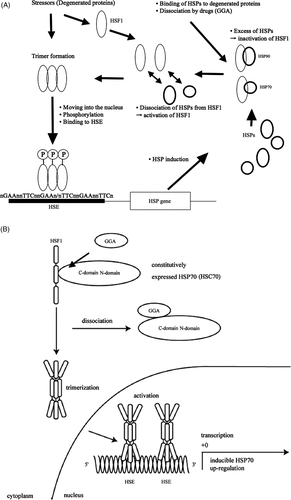
On the other hand, we recently reported the alternative induction mechanism of Hsp70 by geranylgeranylacetone (GGA) that is an anti-ulcer drug introduced as a non-toxic inducer of molecular chaperone (Hsp70) in rat gastric mucosa Citation[28]. GGA is an acyclic isoprenoid compounds with a retinoid skeleton that induces Hsp70 synthesis in various tissues including the gastric mucosa, intestine, liver, myocardium, retina, and central nervous system Citation[29–33]. The induction mechanism is as follows. When concentration of the GGA increases in the cell, GGA preferentially binds to the C-terminal of Hsp70 which is binding to HSF1. After dissociation of Hsp70, free HSF1 is phosphorylated, activated, and acquired the ability to bind to HSE in the promoter region of the heat inducible Hsp70 (known as Hsp72) gene, and finally resulted in the induction of Hsp70 in the cells () Citation[28].
These findings should be also important for the development of ‘chaperone-inducing therapy’ that can be applied for cardiovascular, brain and digestive diseases in the clinical field.
Protection of gastrointestinal mucosa by HSP induction
It is thought that the folding of aggregated (denatured) proteins and many newly synthesised polypeptides in the cell are assisted by a class of proteins (HSPs, molecular chaperones) which function mainly in preventing off-pathway folding reactions that lead to aggregation. HSPs are known to normalise their structure and maintain intracellular environment Citation[1]. These chaperone functions are considered to increase protective ability of the cells against cytotoxic agents and stress-related events. Many reports have suggested cytoprotective ability of HSPs in the gastrointestinal mucosa. As the mechanism for HSP induction in the gastrointestinal mucosa, it has been reported that hyperthermia, mild irritation (gastric acid, zinc) and secondary-induced ischaemic conditions (water-immersion stress, serotonin, thyrotropin-releasing hormone) could be important factors Citation[5–20]. Although some precise mechanisms are still uncertain, especially in vivo, some possible mechanisms are summarised based on the mode of action of stressors in .
Table I. Possible mechanism for HSP induction in vivo-mode of action of stressors.
Oesophagus ()
Little has been known about the expression and function of HSPs in the oesophageal mucosa under stress conditions. The limited data indicate that high levels of Hsp27 are found in the human oesophagus, while its expression is markedly decreased in individuals with Barrett's oesophagus and adenocarcinoma Citation[34]. In acid-induced oesophagitis in opossum, it has been reported that Hsp90 and Hsp60 expressions increase, while Hsp70 expression is decreased Citation[35]. In contrast, we have reported recently that expression of Hsp70 is significantly increased by hyperthermia (42.5°C for 20 min) in rat oesophageal mucosa. In addition, we have demonstrated that reflux oesophagitis is dramatically prevented when Hsp70 was pre-induced by hyperthermia Citation[8]. Furthermore, activation of TNF-α and IL-1β in the oesophageal mucosa is also suppressed in the hyperthermia-treated group. These results suggested that hyperthermia protects the oesophageal mucosa by inducing Hsp70 and suppressing proinflammatory cytokine activation in the reflux oesophagitis model. Although the underlying mechanisms are still unclear in this model, suppression of NF-κB by Hsp70 induction might be a part of the mechanism as reported in the colonic mucosa Citation[9]. Reflux oesophagitis is an increasing disorder that could develop into Barrett's oesophageal mucosa followed by adenocarcinoma. Therefore, to understand these defensive mechanisms of oesophageal mucosa is clinically important.
Table II. Induction and cytoprotective function of HSPs in upper gastrointestinal mucosa.
Stomach
The concept of so-called ‘adaptive cytoprotection’ in the gastric mucosa was first reported by Robert et al. They demonstrated that when rats were treated with a mild irritant such as 10% to 25% ethanol, 0.05–0.35 N HCl, or 2% to 4% NaCl, the gastric mucosa increased its resistance to various necrotising agents such as 100% ethanol, 0.6 N HCl, or 25% NaCl Citation[36]. Prostaglandins (PGs) have been considered to play a main part in this mechanism. On the other hand, the contribution of HSPs to mucosal protection or adaptive cytoprotection has been widely accepted recently. Many reports have suggested that the Hsp70 family especially, which is induced by zinc derivatives Citation[11], Citation[21], GGA Citation[39], hyperthermia Citation[3], low dose-aspirin Citation[20], systemic water immersion stress Citation[16] or thyrotropin-releasing hormone (TRH) Citation[6], have important function in the gastric mucosal protection. Also, gastric mucosal cells overexpressing Hsp70, in which Hsp70 cDNA was transfected, have higher ability in cell restoration in vitro Citation[22]. In contrast, Hsp60 which is induced by administration of serotonin (5-HT) has no cytoprotective function in the gastric mucosa Citation[6]. Therefore, Hsp70-induction therapy including gene transfection therapy can be a candidate for a new therapeutic tool for gastric mucosal injury.
Intestine
We have reported that at the first time, pre-induction of Hsp70 (Hsp72; stress-inducible Hsp70) by systemic hyperthermia protects colonic mucosa from acetic acid-induced mucosal damage in rats Citation[17]. Also, we have reported that Hsp70 induction by intra-rectal administration of zinc derivatives (zinc L-carnosine) enhances protective function of the colonic mucosa through NF-κB inactivation Citation[9]. Some recent reports have supported our studies. Ohkawara et al. have reported that induction of colonic Hsp70 by zinc derivatives or geranylgeranylacetone (GGA) suppressed severity of dextran sulphate sodium (DSS)-induced colitis or 2,4,6-trinitrobenzene sulphonic acid (TNBS)-induced colitis in mice Citation[37]. In the small intestinal mucosa, Sakamoto et al. has reported a possible contribution of Hsp70 to mucosal protection with co-induction of Hsp32 (haeme oxygenase-1) against ischaemia/reperfusion injury in rat small intestine Citation[38].
Hsp60 family (chaperonin homologue) has been reported to be specifically induced by increment of colonic motility or ischaemia induced by thyrotropin-releasing hormone (TRH), or water-immersion stress Citation[15], Citation[18]. In contrast to Hsp70 induction, specific pre-induction of Hsp60 did not protect colonic mucosa from acetic acid-induced mucosal damage Citation[18]. Therefore, in the colonic mucosa, Hsp60 and Hsp70 have different functions in view of chaperone-mediated cytoprotection.
Hsp25 is a member of the small heat-shock protein family that is expressed in nearly all organs under physiological conditions and this protein is also rapidly inducible upon stress activation Citation[38]. Hsp25 has important chaperone properties similar to the GroE/Hsp60 and the Hsp70 family, especially in the kidney and muscle. Overexpression of Hsp25 has been shown to increase survival in response to a wide variety of potentially lethal agents Citation[39]. Chang's group energetically reported the importance of this protein in the colonic epithelial cells. They have reported that specific induction of Hsp25 by short chain fatty acid, interleukin-11 or lipopolysaccharide (LPS) protects colonic mucosa or cultured mucosal epithelial cells from oxidative stress Citation[40–42]. Their series of studies are emphasising that Hsp25 could be a key chaperone in the intestinal epithelial cells as well as Hsp70 family.
Research aspects in post-genomic events in cellular injury utilising chaperone function
The target molecule, which is degenerated by pathogenic condition, recognised by Hsp70 family proteins has not yet been elucidated in vivo. If the target molecule of HSPs in the injured cells was identified it might provide important information regarding the initial post-genomic event during cellular damage, since HSP has been known to rapidly bind to degenerated protein to normalise the structure in vitro. In particular, in the gastric mucosa, cognate HSP (HSC70) might be the first molecular chaperone that plays an important function since stress-inducible Hsp70 (Hsp72) is not sufficiently induced in the cells at this time point (extremely early stage of mucosal injury). Recently, we analysed the degenerated gastric mucosal proteins, which are specifically recognised by Hsp70 family proteins using HSC70-affinity chromatography, and their amino acid sequences were analysed () Citation[43]. Specific HSC70-binding proteins with molecular weights of 200-kDa and 45-kDa were eluted from the affinity column by ATP when gastric mucosal homogenate of extremely early stage was applied. The amino acid sequencing showed that these binding proteins were cytoskeletal myosin heavy chain (200-kDa) and actin (45-kDa).
Figure 2. Gastric mucosal damage and protection by Hsp70 family. When gastric mucosa is exposed to attacking agents or events, degeneration or denaturation of cellular proteins is started. As an acute stress response, constitutively expressed cognate Hsp70 (HSC70) recognises and binds to denatured proteins to fold or normalise the structure. However, when attacking agents are strong enough to develop further cellular damage, HSP-insufficient stage may occur prior to ulcer formation.
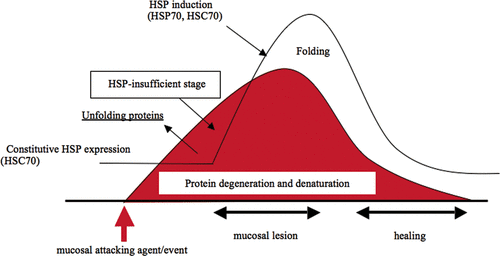
Conclusions
In this paper, induction mechanism and cytoprotective ability of HSPs in the gastrointestinal tract were reviewed. Also, identification of HSP-binding proteins was introduced as a new concept in chaperone research.
We would like to emphasise that understanding the differences in expression and function of HSPs in each organ is important when HSP (chaperone)-inducing therapy would be applied for disease treatment utilising their unique characters as a non-specific internal cytoprotectant. Actually, some diseases are caused by dysfunction or insufficiency of molecular chaperone (chaperone diseases) Citation[44]. Some cause-unknown diseases might be caused by this kind of mechanism. It is also considered that the binding substances, which reduce chaperone function, can be drugs, chemicals, toxins, accumulated denatured proteins or peptides in a category of ‘chaperone diseases’.
Also as shown in this review, identification of HSP-binding proteins would create a new aspect for understanding the unknown mechanisms underlying the diseases. Application of this type of approach to diseases, also including diseases in other organs, may provide new information to elucidate ‘post-genomic’ abnormality or molecular events in the pathogenesis of the diseases and leading to development of new therapeutic strategies.
Acknowledgements
The authors express great thanks to the editors for the opportunity to submit a review article to this journal. Also, the authors thank Professor Hideaki Itoh for scientific collaboration, and members of the GI-group at Juntendo University and Akita University for technical assistance. The authors thank Dr Kumiko Tamaki for proof reading our manuscript.
Declaration of interest: The authors report no conflicts of interest. The authors alone are responsible for the content and writing of the paper.
References
- Itoh H, Tashima Y. The stress (heat shock) proteins. Int J Biochem 1991; 23: 1185–1191
- Yenari M, Liu J, Zheng Z. Antiapoptotic and anti-inflammatory mechanisms of heat-shock protein protection. Ann NY Acad Sci 2005; 1053: 74–83
- Nakamura K, Rokutan K, Narui N, Aoike A, Kawai K. Induction of heat shock proteins and their implication in protection against ethanol-induced damage in cultured guinea pig gastric mucosal cells. Gastroenterology 1991; 101: 161–166
- Hightower LE. Cultured animal cells exposed to amino analogues or puromycin rapidly synthesize several polypeptide. J Cel Physiol 1980; 102: 407–427
- Otaka M, Konishi N, Odashima M, Jin M, Wada I, Matsuhashi T, Horikawa Y, Ohba R, Watanabe S. Is Mongolian gerbil really adequate host animal for study of Helicobacter pylori infection-induced gastritis and cancer?. Biochem Biophys Res Commun 2006; 347: 297–300
- Otaka M, Watanabe S. Expression and function of stress (heat shock) proteins in digestive organs. (Review article) Jpn J Hyperthermic Oncol 2005; 21: 179–190
- Otaka M, Odashima M, Watanabe S. Role of heat shock proteins (molecular chaperones) in intestinal mucosal protection–Mini review. Biochem Biophys Res Commun 2006; 348: 1–5
- Izumi Y, Otaka M, Takahashi T, Takada M, Shimada Y, Asaoka D, Nagahara A, Itoh H, Watanabe S. Specific induction of a 72-kDa heat shock protein protects esophageal mucosa from reflux esophagitis. Life Sci 2009; 84: 17–522
- Odashima M, Otaka M, Jin M, Wada I, Horikawa Y, Matsuhashi T, Ohba R, Hatakeyama N, Oyake J, Watanabe S. Zinc L-carnosine protects colonic mucosal injury through induction of heat shock protein 72 and suppression of NF-kB activation. Life Sci 2006; 79: 2245–2250
- Wada I, Otaka M, Jin M, Odashima M, Komatsu K, Matsuhashi T, Watanabe S. Expression of Hsp72 in the gastric mucosa is regulated by gastric acid in rats–Correlation of Hsp72 expression with mucosal protection. Biochem Biophys Res Commun 2006; 349: 611–618
- Mikami K, Otaka M, Watanabe D, Goto T, Endoh A, Miura K, Ohshima S, Yoneyama K, Sato M, Shibuya T, et al. Zinc L-carnosine protects against mucosal injury in portal hypertensive gastropathy through induction of heat shock protein 72. J Gastroenterol Hepatol 2006; 21: 1669–1674
- Oyake J, Otaka M, Jin M, Odashima M, Komatsu K, Wada I, Horikawa Y, Matsuhashi T, Ohba R, Hatakeyama N, et al. Overexpression of 70-kDa heat shock protein confers protection against monochloramine-induced gastric mucosal cell injury. Life Sci 2006; 79: 300–305
- Otaka M, Itoh H, Kuwabara T, Zeniya A, Fujimori S, Otani S, Tashima Y, Masamune O. Induction of heat shock protein and prevention of caerulein-induced pancreatitis by water-immersion stress in rats. Int J Biochem 1994; 26: 805–811
- Fujimori S, Otaka M, Itoh H, Kuwabara T, Zeniya A, Otani S, Tashima Y, Masamune O. Induction of a 60-kDa heat shock protein in rat gastric mucosa by restraint and water-immersion stress. J Gastroenterol 1994; 29: 544–546
- Kuwabara T, Otaka M, Itoh H, Zeniya A, Fujimori S, Otani S, Tashima Y, Masamune O. Regulation of Hsp60 expression in rat colonic mucosa. J Gastroenterol 1994; 29: 721–726
- Zeniya A, Otaka M, Itoh H, Kuwabare T, Fujimori S, Otani S, Tashima Y, Masamune O. Induction and intracellular localization of a 72-kDa heat shock protein in rat gastric mucosa after water-immersion stress. J Gastroenterol 1995; 30: 572–577
- Otani S, Otaka M, Jin M, Okuyama A, Itoh S, Iwabuchi A, Sasahara H, Itoh H, Tashima Y, Masamune O. Effect of pre-induction of heat shock proteins on acetic acid-induced colitis in rats. Dig Dis Sci 1997; 42: 833–846
- Iwabuchi A, Otaka M, Otani S, Jin M, Okuyama A, Itoh S, Sasahara H, Odashima M, Pacheco I, Itoh H, et al. Specific pre-induction of a 60-kDa heat shock protein (chaperonin homolog) by TRH does not protect colonic mucosa against acetic acid-induced lesion in rats. Dig Dis Sci 2000; 45: 1480–1489
- Sasahara H, Otaka M, Itoh S, Iwabuchi A, Odashima M, Wada I, Konishi N, Pacheco I, Tashima Y, Itoh H, et al. Effect of pre-induction of heat shock proteins on acetic acid-induced small intestinal lesion in rats. Dig Dis Sci 1998; 43: 2117–2130
- Jin M, Otaka M, Okuyama A, Itoh S, Otani S, Odashima M, Iwabuchi A, Konishi N, Wada I, Pacheco I, et al. Association of a 72-kDa heat shock protein expression with adaptation to aspirin in rat gastric mucosa. Dig Dis Sci 1999; 44: 1401–1407
- Odashima M, Otaka M, Jin M, Konishi N, Sato T, Kato S, Matsuhashi T, Nakamura C, Watanabe S. Induction of a 72-kDa heat shock protein in cultured rat gastric mucosal cells and rat gastric mucosa by zinc L-carnosine. Dig Dis Sci 2003; 47: 2799–2804
- Otaka M, Matsuhashi T, Itoh H, Odashima M, Jin M, Wada I, Komatsu K, Horikawa Y, Ohba R, Oyake J, et al. Enhancement of cytoprotective ability and restoration in 70-kDa heat shock protein gene-transfected rat gastric mucosal cells. Aliment Pharmacol Ther (Symposium ser) 2006; 2: 272–277
- Åkerfelt M, Trouillet D, Mezger V, Sistonen L. Heat shock factors at a crossroad between stress and development. Ann N Y Acad Sci 2007; 1113: 15–27
- Akerfelt M, Henriksson E, Laiho A, Vihervaara A, Rautoma K, Kotaja N, Sistonen L. Promoter ChIP-chip analysis in mouse testis reveals Y chromosome occupancy by HSF2. Proc Natl Acad Sci USA 2008; 105: 11224–11229
- Fujimoto M, Oshima K, Shinkawa T, Wang BB, Inouye S, Hayashida N, Takii R, Nakai A. Analysis of HSF4 binding regions reveals its necessity for gene regulation during development and heat shock response in mouse lenses. J Biol Chem 2008; 283: 29961–29970
- Zou J, Guo Y, Guettouche T, Smith DF, Voellmy R. Repression of heat shock transcription factor HSF1 activation by Hsp90 (Hsp90 complex) that forms a stress-sensitive complex with HSF1. Cell 1998; 94: 471–480
- Shi Y, Mosser DD, Morimoto RI. Molecular chaperones as HSF1-specific transcriptional repressors. Genes Dev 1998; 12: 654–66
- Otaka M, Yamamoto S, Ogasawara K, Takaoka Y, Noguchi S, Miyazaki T, Nakai A, Odashima M, Matsuhashi T, Watanabe S, et al. The induction mechanism of the molecular chaperone Hsp70 in the gastric mucosa by geranylgeranylacetone (HSP-inducer). Biochem Biophys Res Commun 2006; 353: 399–404
- Hirakawa T, Rokutan K, Nikawa T, Kishi K. Geranylgeranylacetone induces heat shock proteins in cultured guinea pig gastric mucosal cells and rat gastric mucosa. Gastroenterology 1996; 111: 345–357
- Yamagami K, Yamamoto Y, Ishikawa Y, Yonezawa K, Toyokuni S, Yamaoka Y. Effects of geranyl-geranyl-acetone administration before heat shock preconditioning for conferring tolerance against ischemia-reperfusion injury in rat livers. J Lab Clin Med 2000; 135: 465–475
- Ooie T, Takahashi N, Saikawa T, Nawata T, Arikawa M, Yamanaka K, Hara M, Shimada T, Sakata T. Single oral dose of geranylgeranylacetone induces heat-shock protein 72 and renders protection against ischemia/reperfusion injury in rat heart. Circulation 2001; 104: 1837–1843
- Ishii Y, Kwong JM, Caprioli J. Retinal ganglion cell protection with geranylgeranylacetone, a heat shock protein inducer, in a rat glaucoma model. Invest Ophthalmol Vis Sci 2003; 44: 1982–1992
- Fujiki M, Kobayashi H, Abe T, Ishii K. Astroglial activation accompanies heat shock protein upregulation in rat brain following single oral dose of geranylgeranylacetone. Brain Res 2003; 991: 254–257
- Soldes OS, Kuick RD, Thompson IA, Hughes SJ, Orringer MB, Iannettoni MD, Hanash SM, Beer DG. Differential expression of Hsp27 in normal oesophagus, Barrett's metaplasia and oesophageal adenocarcinomas. Br J Cancer 1999; 79: 595–603
- White R, Morris G, Moyes C, Blennerhassett M, Hill C, Pringle G, Paterson W. Analysis of mucosal stress response in acid-induced esophagitis in opossum. Dig Dis Sci 2002; 47: 1873–1885
- Robert A, Nezamis JE, Lancaster C, Davis JP, Field SO, Hanchar AJ. Mild irritants prevent gastric necrosis through ‘adaptive cytoprotection’ mediated by prostaglandins. Am J Physiol 1983; 245: G113–G121
- Ohkawara T, Nishihira J, Takeda H, Katsurada T, Kato K, Yoshiki T, Sugiyama T, Asaka M. Protective effect of geranylgeranylacetone on trinitrobenzene sulfonic acid-induced colitis in mice. Int J Mol Med 2006; 17: 229–234
- Sakamoto N, Kokura S, Okuda T, Hattori T, Katada K, Isozaki Y, Nakabe N, Handa O, Takagi T, Ishikawa T, et al. Heme oxygenase-1 (Hsp32) is involved in the protection of small intestine by whole body mild hyperthermia from ischemia/reperfusion injury in rat. Int J Hyperthermia 2005; 21: 603–614
- Jakob U, Gaestel M, Engel K, Buchner J. Small heat shock proteins are molecular chaperones. J Biol Chem 1993; 68: 1517–1520
- Ropeleski MJ, Tang J, Walsh-Reitz MM, Musch MW, Chang EB. Interleukin-11-induced heat shock protein 25 confers intestinal epithelial-specific cytoprotection from oxidant stress. Gastroenterology 2003; 124: 1358–1368
- Ren H, Musch MW, Kojima K, Boone D, Ma A, Chang EB. Short-chain fatty acids induce intestinal epithelial heat shock protein 25 expression in rats and IEC 18 cells. Gastroenterology 2001; 121: 631–639
- Kojima K, Musch MW, Ropeleski MJ, Boone DL, Ma A, Chang EB. Escherichia coli LPS induces heat shock protein 25 in intestinal epithelial cells through MAP kinase activation. Am J Physiol Gastrointest Liver Physiol 2004; 286: G645–652
- Otaka M, Odashima M, Izumi Y, Nagahara A, Osada T, Sakamoto N, Takada M, Takahashi T, Shimada Y, Tamaki K, et al. Target molecules of molecular chaperon (Hsp70 family) in injured gastric mucosa in vivo. Life Sci 2009; 84: 664–667
- Miyazaki T, Sagawa R, Honma T, Noguchi S, Harada T, Komatsuda A, Ohtani H, Wakui H, Sawada K, Otaka M, et al. 73-kDa molecular chaperone Hsp73 is a direct target of antibiotic gentamicin. J Biol Chem 2004; 279: 17295–17300