Abstract
Purpose: To investigate the potential of multi-slice computed tomography (MSCT) as a tool for non-invasive temperature measurement.
Materials and methods: Samples of water, 0.9% saline, sunflower oil and dilutions of (1:32, 1:64, 1:128) contrast agent (Iopromid 370, BayerSchering Pharma, Berlin) were heated in a plexiglass phantom. In a first set-up, samples of 0.9% saline solution were scanned at defined temperatures (25°–75°C; 5°C intervals) using a clinical CT scanner. Scan parameters (tube current–time product, tube voltage, collimation, slice thickness) were systematically varied. In a second set-up samples of the different fluids (water, sunflower oil, contrast agent dilutions) were scanned using the following scan protocol: 250 mAs, 140 kV, 1.2 mm collimation, 9.6 mm slice thickness. CT numbers were measured in reconstructed axial images at the different temperatures. A regression analysis was performed to investigate the relationship between temperature and CT number.
Results: Standard deviation of measured CT numbers decreased with increasing tube current–time product, increasing tube voltage, thicker collimation and higher slice thickness. Regression analysis showed an inverse relationship between temperature and CT number for all fluids with regression coefficients of −0.471 (0.9% saline), 0.447 (water), −0.679 (sunflower oil), −0.420 (contrast agent 1:32), −0.414 (contrast agent 1:64) and −0.441 (contrast agent 1:128), respectively.
Conclusion: Multi-slice computed tomography can depict thermal density expansion of different fluids. Based on these results the implementation of a temperature discrimination of several degrees C at a high spatial resolution is achievable.
Introduction
In recent years image-guided thermal ablation techniques such as radiofrequency (RF) ablation (RFA) Citation[1], laser-induced thermotherapy (LITT) Citation[2] and microwave ablation (MWA) Citation[3], Citation[4] have been developed for the minimally invasive therapy of different malignancies. Especially for the treatment of primary and secondary malignant liver tumours, RF ablation found its way into clinical routine during the last decade. Although surgical resection remains the gold standard in patients suffering from metastatic liver disease or hepatocellular carcinoma (HCC), image-guided hyperthermal ablation techniques are the therapy of choice in patients who are not suited for curative surgical resection due to severe comorbidities or who refuse surgery. In contrast to hyperthermia, which is used in combination with radiation or chemotherapy, target temperature in hyperthermal ablation techniques is between 60° and 100°C. At 60°C a nearly instantaneous protein denaturation is achieved while temperatures above 100°C result in boiling of interstitial fluid followed by desiccation leading to a decreased thermal and electrical conductivity Citation[5]. In order to maintain the desired temperature within the target volume temperature measurements are helpful. Therefore, some RF systems feature integrated temperature sensors allowing temperature measurements at dedicated positions during the RF ablation procedure. Nevertheless, the measurement of local temperature at certain points does not necessarily reflect the heat distribution within the target volume that should be ablated. Magnetic resonance imaging (MRI) has been shown to be a potential tool for non-invasive depiction of local temperature distribution using the temperature-dependent phase shift, for example Seror et al. Citation[6]. However, different technical problems may limit the use of this technique in clinical routine. More than three decades ago the temperature dependence of computed tomography (CT) numbers was investigated. A temperature-dependent shift of CT numbers was found for different materials but due to technical capabilities of the CT scanners used, this application was not further developed.
Therefore, the aim of our study was to investigate the potential of multi-slice CT as a tool for non-invasive temperature measurement for monitoring of RF ablation in vitro and to identify an optimal scan protocol for non-invasive CT temperature measurement.
Materials and method
First, temperature-dependent changes of CT numbers were investigated for 0.9% NaCl solution employing a systematic variation of scan parameters in order to analyse the influence of tube voltage, tube current–time product, collimation and slice thickness on the standard deviation of CT numbers. Second, the optimal scan protocol regarding the afore mentioned scan parameters, which provided the lowest mean standard deviation of CT numbers, was used to analyse temperature-dependent change of the CT numbers in samples of water and sunflower oil. Furthermore, three different dilutions (1:32, 1:64, 1:128) of iodine-containing contrast agents (iopromide 370) were also investigated.
In vitro studies were performed using a cylindrical plexiglass phantom with a diameter of 200 mm that was scanned in a clinical dual source CT scanner (Somatom Definition, Siemens, Forchheim, Germany). The temperature of the fluid samples was controlled by a temperature-stabilised heating bath which circulated the fluid within the phantom. The phantom was placed on the CT table with the phantom being positioned in the centre of the CT scanner gantry (). An optical temperature probe (SFF-2m, Luxtron Corporation, Santa Clara, CA) was connected to a thermometer and placed in the phantom to continuously measure the exact temperature during the CT scans.
Figure 1. Experimental set-up featuring fluid-filled phantom with heating bath (white arrow) and thermometer with optical temperature probe (black arrow).
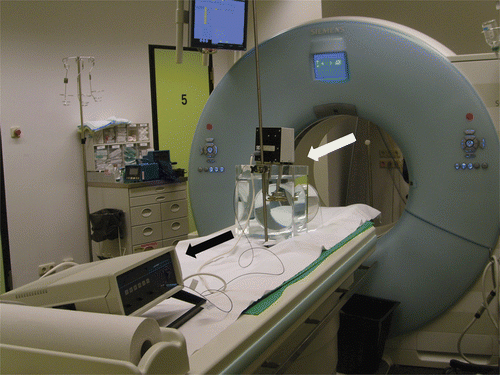
Scan protocol
As recommended by the manufacturer, we performed the so-called check-up procedure prior to our measurements. The CT scanner automatically then performs several calibration steps to ensure preciseness of measured CT numbers. This includes an air calibration process employing different predefined voltage and tube current-time product settings. This method sets the CT number of air to be −1000 HU. The temperature of the fluid samples within the phantom was systematically increased in 5°C steps from 25°–75°C. shows an overview about the used scan protocols. In the first set of experiments tube voltage was kept constant at 120 kV, whereas the tube current–time product was systematically varied between 20 and 320 mAs in 20 mAs intervals. A single full tube rotation (rotation time: 1 s) without table feed was obtained using a collimation of either 12 × 0.6 mm or 24 × 1.2 mm, which resulted in a scan range of 7.2 mm (12 × 0.6 mm) and 28.8 mm (24 × 1.2 mm), respectively, at the isocentre. Using a full scan, reconstruction slices with 2.4, 4.8, 9.6 mm thickness were reconstructed. For the second set of experiments tube current–time product was set 250 mAs. Tube voltage was varied between 80 and 140 kV and again, collimation was set 12 × 0.6 mm and 24 × 1.2 mm, respectively. All Image data was reconstructed applying a field-of-view of 300 × 300 mm and 512 × 512 reconstruction matrix.
Table I. Scan parameters used for the two sets of experiments.
Image analysis
After raw data acquisition axial images were reconstructed using a soft tissue reconstruction kernel (Siemens B 40f). Slice thickness was 2.4 mm for 12 × 0.6 mm collimation and 2.4, 4.8 and 9.6 mm for 24 × 1.2 mm collimation, respectively. The area averaged CT numbers was measured using a 10 mm2 region of interest that was placed centrally within the cross-section of the cylindrical phantom part. Standard deviation was automatically calculated by the software. At every temperature step 10 measurements were performed resulting in 110 measurements per scan protocol.
Statistical analysis
A multi-factorial analysis of variance (ANOVA) was performed to compare the mean standard deviations of the measured CT numbers for the different scan protocols which were performed using 0.9% NaCl solution samples. P values <0.05 were considered to be significant. In case of significance, post-hoc Tukey tests were carried out to investigate which settings differed. For all fluids a regression analysis was performed to analyse the relationship between temperature and measured area averaged CT number. All analysis was done using SAS Software 9.1.3 (SAS Institute, Cary, NC).
Results
The systematic variation of tube current–time product showed a decrease of the standard deviation of the area-averaged CT numbers for 0.9% saline samples scanned at 120 kV as shown in . Regarding tube current-time product the ANOVA followed by post-hoc Tukey tests showed that all pair-wise comparisons were significant except 220 versus 240, 240 versus 250, 240 versus 260, 250 versus 260, 250 versus 280, 260 versus 280, 280 versus 300 and 300 versus 320. Furthermore, standard deviation decreased with increasing tube voltage (). A thicker collimation and reconstruction of thicker slices resulted in a decrease of the standard deviation of the measured CT numbers as well ( and ). For tube voltage, collimation and slice thickness it was found that all pair-wise comparisons showed significant differences (p < 0.0001). The lowest mean standard deviation for 0.9% saline samples was found using the following imaging parameters: 250 mAs, 140 kV, 1.2 mm collimation, 9.6 mm slice thickness. The volume CT dose index (CTDIvol) of this scan protocol was 25.05 mGy and the dosis length product (DLP) was 72.14 mGy*cm.
Table II. Least square means of the standard deviation of area averaged CT numbers depending on tube current time product at 120 kV.
Table III. Least square means of the standard deviation of area averaged CT numbers depending on tube voltage at 250 mAs.
Table IV. Least square means of the standard deviation of area averaged CT numbers depending on collimation and reconstructed slice thickness.
Table V. Results of the regression analysis (temperature versus CT number) for the different fluids.
The regression analysis showed negative regression coefficients for all tested fluids. The regression coefficients for all water-based fluids appeared to be within a small range (−0.414, −0.471) whereas sunflower oil showed a remarkably low regression coefficient (−0.679), indicating a stronger influence of temperature on the CT number in this setting ( and ; ).
Discussion
The temperature-dependent change of CT numbers in water and biological tissue samples is known since the late 1970s Citation[7]. Initially this observation was discussed in the context of absolute CT numbers for the differentiation of materials and CT-scanner calibration. A few years later first studies on the applicability of non-invasive thermometry using clinical CT scanners during hyperthermia were published Citation[8]. In an in vitro study Fallone et al. described an observable CT number thermal shift in water and muscle tissue samples. Furthermore, they concluded that due to a coefficient of thermal density expansion of water near human body temperature of −3.6*10−4/°C, a temperature increase of 1°C would be reflected by an increase of 0.4 HU in a water-containing subject. In our study we found a regression coefficient for thermal dependent change of CT number of water of 0.447 HU/°C. However, the authors stated that a temperature discrimination of a fraction of °C at a spatial resolution of the order of 10 mm would be achievable. But for the application of non-invasive thermometry during hyperthermal ablation therapies such as LITT or RF ablation as was suggested in the literature Citation[9], a spatial resolution of <2 mm would be desirable. Considering the sub-millimetre spatial resolution of modern clinical CT scanners a temperature discrimination of 3–5°C could be realised.
Due to the significant inter- and intrascanner variability of CT number, an individual calibration process for non-invasive thermometry using CT during hyperthermal ablation therapies is mandatory Citation[10]. In this context the use of a RF probe featuring an integrated thermocouple or the placement of a separate optical thermocouple parallel to the RF probe may be helpful. The temperature values directly measured by the temperature probe during the heating process, while RF energy is applied, could be used to analyse the individual temperature-dependent changes of CT numbers in the vicinity of the temperature probe. This could be extrapolated to the whole target volume that should be ablated although differences in CT number shift between tumour and healthy tissue at the margin of the target area would be disregarded.
It can be assumed that artefacts occurring during local ablation can hinder a reliable temperature monitoring based on CT numbers. In context of RF ablation, the metal and beam hardening artefacts caused by the RF probe may especially represent a potentially severe problem, though the temperature in the immediate vicinity of the RF probe, where artefacts are expected to be severe, is not as relevant as the temperature at the border area of the target volume. Software algorithms which are used to minimise metal artefacts may help to overcome this problem, although these algorithms use interpolation techniques which might influence the absolute measured CT number Citation[11]. Furthermore, there still remains a loss of detail around the metal–tissue interface Citation[12]. A second reason for artefacts during hyperthermal ablation procedures is tissue boiling and vaporisation, but usually this occurs in the direct vicinity of the RF probe as well as where the highest local temperatures are achieved.
Besides ultrasound Citation[13], Citation[14] and microwave imaging Citation[15], MR-based thermometry has been used for non-invasive temperature measurement during hyperthermal ablation procedures. There are several temperature-sensitive MR parameters providing a base for MR thermometry such as the proton resonance frequency, the diffusion coefficient, T1 and T2 relaxation times, magnetisation transfer, the proton density, and temperature-sensitive contrast agents Citation[16], Citation[17]. Temperature monitoring during LITT especially can be performed using MR imaging because there is no interference between the laser equipment used and the MR scanner. In contrast, during RF ablations or hyperthermia, MR systems are susceptible to interferences from the RF system used. Technical solutions of this problem can be provided by implementation of appropriate filtering Citation[18], Citation[19] or interleaving of RF ablation cycles and MR data acquisition Citation[20]. Furthermore MR-guided radiofrequency ablation requires the use of special MR-compatible RF probes. In this context, non-invasive thermometry using CT would be beneficial due to the lack of interference between RF probe and system and the CT scanner and the possibility to use standard RF equipment. However, MR thermometry provides a technique for non-invasive temperature monitoring without the need of radiation exposure, whereas CT-based temperature measurement would require repeated CT examinations using high dose protocols.
The optimum scan protocol used in the present study resulted in a CTDIvol of 25.05 mGy covering 28.8 mm in z-direction. Using CT-based thermometry for monitoring of RF ablations it would probably be necessary to perform a baseline CT examination before the energy application is started, followed by repeated CT scans to monitor the temperature-dependent changes of CT numbers. Depending on the RF probe used and intended ablation volume control, CT scans would be performed with a time interval of several minutes. The image data obtained immediately before the end of the energy application is of special interest because it reflects maximum heat distribution. On the basis of this temperature information the extent of the coagulation area can be assumed, because from in vivo measurements it is known that in liver parenchyma temperatures between 59.8°C and 68.4°C are found at the ablation margin Citation[21]. In our experience a typical hepatic RF ablation procedure of a single lesion (30 mm diameter) may take up to 60 min which means that about 6 CT scans for non-invasive temperature measurements would be performed. For the treatment of a single hepatic lesion with a diameter of 30 mm the generation of a coagulation volume with a diameter of approximately 50 mm is necessary due to the ablation of a 10-mm safety margin. Assuming this dimension of the target volume the volume coverage of the optimum scan protocol (28.8 mm) needs to be extended equally. This will result in doubling of the total applied radiation dose. In the assumed case (single lesion, 50-mm coagulation diameter, 10 CT scans) the additional radiation exposure associated with CT-based temperature monitoring would be approximately 1252.5 mGy*cm (DLP). Considering a skin erythema threshold dose of 200 cGy the additionally applied radiation dose should not result in any skin damage. Further studies are needed in order to exactly measure the applied radiation dose at different parts of the body and to assess potential side effects due to the additional radiation exposure of healthy organs which are adjacent to the RF ablation volume. On the other hand the mostly palliative character of hepatic RF ablation in oncology patients with limited life expectancy needs to be considered. Nevertheless, technical improvements regarding reconstruction algorithms and image data processing may help to reduce the applied radiation dose in the future.
Regarding potential artefacts caused by interference between the operating RF ablation system and the CT scanner, Brennan et al. reported severe artefacts during hepatic RF ablation Citation[22]. They speculated that an electromagnetic cross-talk occurred between CT scanner and RF system during RF ablation. However, due to the fact that there have been no further publications dealing with this problem, it seems to be a unique event.
The observation of the low regression coefficient found for sunflower oil indicating a strong influence of temperature on the CT number may be a hint that temperature monitoring using CT works better in fatty tissues. It needs to be further evaluated whether a transarterial embolisation using oily substances such as Lipiodol before the hyperthermal ablation procedure may be beneficial for the use of CT-based temperature monitoring.
Some limitations of the present study need to be discussed. First, results of an in vitro study cannot be directly transferred into the in vivo situation. The achievable temperature discrimination at a high spatial resolution especially needs to be evaluated in an in vivo model, though the presented data shows an inverse relationship between temperature and CT number for different fluids, which means that temperature monitoring using CT is basically feasible. In contrast to homogeneous fluids, biological tissues such as liver parenchyma have a complex composition with different compartments containing mainly intra- and intercellular fluid but also larger molecules such as protein or DNA. Therefore, changes of CT numbers during hyperthermal ablation will not only be due to temperature-dependent changes in density but also a result of desiccation and protein coagulation. For this reason it may be helpful to maintain a high fluid content during the ablation procedure for example by injection of saline solution using perfused RF probes.
In conclusion the presented data shows an almost linear relationship between CT number and temperature of the different fluid samples providing the base for non-invasive thermometry using a clinical CT scanner. In order to investigate the applicability of non-invasive thermometry using thermal dependent shift of CT numbers, further ex vivo and especially in vivo studies are needed.
Declaration of interest: The authors report no conflicts of interest. The authors alone are responsible for the content and writing of the paper.
References
- Pereira PL. Actual role of radiofrequency ablation of liver metastases. Eur Radiol 2007; 17: 2062–2070
- Vogl TJ, Straub R, Eichler K, Söllner O, Mack MG. Colorectal carcinoma metastases in liver: Laser-induced interstitial thermotherapy–Local tumor control rate and survival data. Radiology 2004; 230: 450–458
- Zhang X, Chen B, Hu S, Wang L, Wang K, Wachtel MS, Frezza EE. Microwave ablation with cooled-tip electrode for liver cancer: An analysis of 160 cases. Hepatogastroenterology 2008; 55: 2184–2187
- Wang Y, Liang P, Yu X, Cheng Z, Yu J, Dong J. Ultrasound-guided percutaneous microwave ablation of adrenal metastasis: Preliminary results. Int J Hyperthermia 2009; 25: 455–461
- Goldberg SN, Dupuy DE. Image-guided radiofrequency tumor ablation: challenges and opportunities–Part I. J Vasc Interv Radiol 2001; 12: 1021–1032
- Seror O, Lepetit-Coiffé M, Le Bail B, de Senneville BD, Trillaud H, Moonen C, Quesson. Real time monitoring of radiofrequency ablation based on MR thermometry and thermal dose in the pig liver in vivo. Eur Radiol 2008; 18: 408–416
- Bydder GM, Kreel L. The temperature dependence of computed tomography attenuation values. J Comput Assist Tomogr 1979; 3: 506–510
- Fallone BG, Moran PR, Podgorsak EB. Noninvasive thermometry with a clinical x-ray scanner. Med Phys 1982; 9: 715–721
- Wood BJ, Locklin JK, Viswanathan A, Kruecker J, Haemmerich D, Cebral J, Sofer A, Cheng R, McCreedy E, Cleary K, et al. Technologies for guidance of radiofrequency ablation in the multi-modality interventional suite of the future. J Vasc Interv Radiol 2007; 18: 9–24
- Birnbaum BA, Hindman N, Lee J, Babb JS. Multi-detector row CT attenuation measurements: Assessment of intra- and interscanner variability with an anthropomorphic body CT phantom. Radiology 2007; 242: 109–119
- Mahnken AH, Raupach R, Wildberger JE, Jung B, Heussen N, Flohr TG, Günther RW, Schaller S. A new algorithm for metal artifact reduction in computed tomography: In vitro and in vivo evaluation after total hip replacement. Invest Radiol 2003; 38: 769–775
- Barrett JF, Keat N. Artifacts in CT: Recognition and avoidance. Radiographics 2004; 24: 1679–1691
- Seip R, Ebbini ES. Noninvasive estimation of tissues temperature response to heating fields using diagnostic ultrasound. IEEE Trans Biomed Eng 1995; 42: 828–839
- Arthur RM, Straube WL, Trobaugh JW, Moros EG. In vivo change in ultrasonic backscattered energy with temperature in motion-compensated images. Int J Hyperthermia 2008; 24: 389–398
- Meaney PM, Zhou T, Fanning MW, Geimer SD, Paulsen KD. Microwave thermal imaging of scanned focused ultrasound heating: Phantom results. Int J Hyperthermia 2008; 24: 523–536
- Rieke V, Butts Pauly, K. MR thermometry. J Magn Reson Imaging 2008; 27: 376–390
- Wyatt C, Soher B, Maccarini P, Charles HC, Stauffer P, Macfall J. Hyperthermia MRI temperature measurement: Evaluation of measurement stabilisation strategies for extremity and breast tumours. Int J Hyperthermia 2009; 25: 422–433
- Vigen KK, Jarrard J, Rieke V, Frisoli J, Daniel BL, Butts Pauly K. In vivo porcine liver radiofrequency ablation with simultaneous MR temperature imaging. J Magn Reson Imaging 2006; 23: 578–584
- Gellermann J, Faehling H, Mielec M, Cho CH, Budach V, Wust P. Image artifacts during MRT hybrid hyperthermia–Causes and elimination. Int J Hyperthermia 2008; 24: 327–335
- Botnar RM, Steiner P, Dubno B, Erhart P, von Schulthess GK, Debatin JF. Temperature quantification using the proton frequency shift technique: In vitro and in vivo validation in an open 0.5 Tesla interventional MR scanner during RF ablation. J Magn Reson Imaging 2001; 13: 437–444
- Mertyna P, Dewhirst MW, Halpern E, Goldberg W, Goldberg SN. Radiofrequency ablation: the effect of distance and baseline temperature on thermal dose required for coagulation. Int J Hyperthermia 2008; 24: 550–559
- Brennan DD, Appelbaum L, Raptopolous V, Kruskal JB, Goldberg SN. CT artifact introduced by radiofrequency ablation. Am J Roentgenol 2006; 186: S284–S846