Abstract
Magnetic nanoparticles are increasingly used for clinical applications such as drug delivery, magnetic resonance imaging and magnetic fluid hyperthermia. A novel method of interstitial heating of tumours following direct injection of magnetic nanoparticles has been evaluated in humans in recent clinical trials. In prostate cancer this approach has been investigated in two separate phase I studies, employing magnetic nanoparticle thermotherapy alone and in combination with permanent seed brachytherapy. The feasibility and good tolerability was shown in both trials, using the first prototype of an alternating magnetic field applicator. As with any other heating technique, this novel approach requires specific tools for planning, quality control and thermal monitoring, based on appropriate imaging and modelling techniques. In these first clinical trials a newly developed method for planning and non-invasive calculations of the 3-dimensional temperature distribution based on computed tomography was validated.
Limiting factors of the new approach at present are patient discomfort at high magnetic field strengths and irregular intratumoural heat distribution. Until these limitations are overcome and thermoablation can safely be applied as a monotherapy, this treatment modality is being evaluated in combination with irradiation in patients with localised prostate cancer.
Introduction
Hyperthermia is recognised as an alternative treatment that can be delivered alone or as an adjunct to radiation and/or chemotherapy to treat cancer Citation[1]. An increase of temperature above 40°C either enhances the effects of radiation and chemotherapy or leads to direct cell killing (thermoablation) as a function of time and temperature Citation[2]. Thermal therapies have been evaluated extensively for both hyperplastic and malignant conditions of the prostate during the last few decades Citation[3]. Nanotechnology is a rapidly evolving field, which has had a tremendous impact on medicine in general and on the diagnosis and treatment of cancer in particular Citation[4]. Hyperthermia is one of several promising biomedical applications of magnetic nanoparticles for the treatment of cancer Citation[5]. As opposed to thermoablation monotherapy, strategies aimed at combining hyperthermia with radiation to exploit the known therapeutic synergism of these two modalities to treat prostate cancer have long been pursued Citation[6–13]. By enhancing the biological dose of radiation with hyperthermia, lower radiation doses may yield an equal efficacy while decreasing radiation-induced toxicity. A biological rationale for thermoradiotherapy of prostate cancer has been demonstrated in vitro and in vivo Citation[14]. However, clinical hyperthermia of prostate cancer is complex due to the surrounding tissues and the high perfusion of this organ. Effective temperatures achieved in the whole prostate with radiofrequency or ultrasound techniques were often limited by electric field elevation at electrical boundaries. These phenomena have limited achievable temperatures and thermal homogeneity in the prostate with conventional heating techniques Citation[6–13]. As a result, the search for new hyperthermia applicator techniques offering better heating characteristics is ongoing Citation[3] and thermoradiotherapy of prostate cancer is still not routinely employed.
Hyperthermia with small magnetites was pioneered by Gilchrist in 1957 and further developed by Gordon and others 20 years later Citation[15]. Widespread clinical use of this technique was hindered by technical limitations such as uncertainties regarding intratumoural distribution of particles and heat. Besides, no documentation of the effect of heat on tumour growth was provided in these early studies. Magnetic nanoparticle thermotherapy is a novel minimally invasive approach developed for interstitial thermal therapy Citation[16]. In this technique a dispersion of biocompatible iron-oxide nanoparticles (magnetic fluid) is injected directly into superficial or deep-seated tumours and consecutively heated in an alternating magnetic field. This technology is the first heating technique using magnetic nanoparticles to have entered clinical trials. In this review, the current results of magnetic nanoparticle thermotherapy in prostate cancer are described and future perspectives are outlined.
Magnetic nanoparticles for thermal therapy: Rationale and preclinical data
Materials used for magnetically mediated hyperthermia include seeds (rods of several mm size), multidomain particles (1–300 µm) and nanoparticles (1–100 nm), the latter including subdomain particles (below 20 nm). Heating of a medium depends on the specific absorption rate (SAR) of the implant in an alternating magnetic field. Thermal energy is released to the surrounding medium as a result of physical processes that differ according to the size of the magnetic material used and the strength of the applied magnetic field Citation[16]. Whereas larger implants like seeds generate heat by resistance to circumferential eddy currents induced on the surface of the seeds by an alternating magnetic field, multidomain particles typically heat by hysteresis loss effects. By contrast, nanoparticle and in particular subdomain particle suspensions generate heat mainly by Brownian and Néel relaxation processes Citation[16].
The physical potential of magnetic nanoparticles for heating purposes and their superiority over larger materials was first demonstrated by Jordan et al. Citation[17]. The excellent power absorption capabilities of magnetic fluids in a magnetic field can be attributed to the large number and surface of heating elements. A further characteristic of magnetic nanoparticles is intracellular hyperthermia. Due to their aminosilane-type coating, the nanoparticles (NanoTherm AS1, MagForce Nanotechnologies, Berlin, Germany) are taken up intracellularly by differential endocytosis Citation[18], Citation[19]. Moreover, selective uptake into prostate cancer cells has been shown in vitro Citation[20]. Animal studies on mouse mammary carcinoma, rat glioblastoma and prostate cancer have demonstrated the feasibility and efficacy of this heating method, as well as a very low clearance rate of the nanoparticles from tumours, allowing for serial heat treatments following a single magnetic fluid injection Citation[21–23]. In a prostate tumour model, thermoablative temperatures of up to 70°C were achieved Citation[24]. The feasibility and efficacy of combined thermal therapy using magnetic nanoparticles and irradiation has also been shown in a rat model of prostate cancer Citation[25].
Clinical studies on prostate cancer
Magnetic nanoparticle thermotherapy as a monotherapy
A prospective phase I study investigated the feasibility of thermotherapy using magnetic nanoparticles in patients with locally recurrent prostate cancer Citation[26], Citation[27]. An important prerequisite for treatment planning and quality control in thermal therapy is adequate imaging after instillation of the magnetic fluid. Magnetic resonance imaging (MRI) cannot be used because of susceptibility artefacts, i.e. signal loss in the regions containing iron oxide nanoparticles. Using computed tomography (CT), deposits of these particles appeared at a mean density of 450 HU and could be visualised in prostate tissue with a sensitivity of around 90% () Citation[26], Citation[27]. Feasibility was defined as the ability to heat the prostate, to achieve sufficient interstitial deposition of nanoparticles in the prostate for six sequential treatments and to evaluate a CT-based approach for quality control and non-invasive calculations of the 3-dimensional temperature distribution. Other endpoints of this study were toxicity and quality of life (QoL), evaluated using the European Organization for Research and Treatment of Cancer (EORTC) QLQ-C30 and QLQ-PR25 questionnaires. Oncological outcome was assessed by serum prostate-specific antigen (PSA)-measurements and prostate biopsies.
Figure 1. Example of an unenhanced computed tomography scan obtained one year after a single injection of magnetic fluid into the prostate, followed by six thermotherapy treatments. Hyperdense nanoparticle deposits in the prostate are still visible.
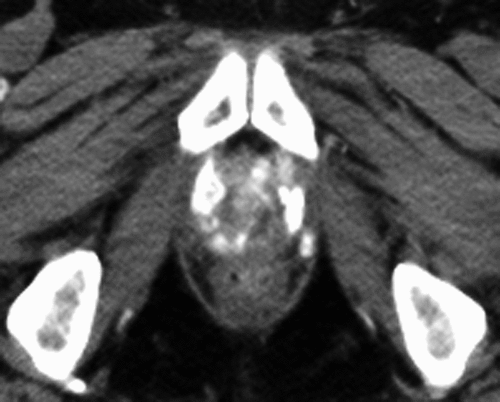
Ten patients with biopsy-proven locally recurrent prostate cancer were enrolled. Three patients had a local recurrence following prostatectomy and irradiation, the other patients had radio-recurrent disease, which was androgen-independent in two cases and hormone-refractory in one case. The nanoparticle dispersion was injected transperineally into the prostates under general anaesthesia and TRUS/fluoroscopy guidance Citation[26]. Invasive thermometry of the prostate was performed during the first and last session, while intraluminal thermometry in the urethra and in the rectum was carried out during each treatment. Temperature distribution in the target region was calculated from the iron mass (derived from nanoparticle volume and density in CT), the magnetic field strength during treatments and the known SAR of the magnetic fluid used (assuming a constant perfusion) by means of the bio-heat transfer equation. These calculations were correlated with direct temperature mappings in defined measurement points to estimate the three-dimensional intraprostatic temperature distribution in each patient Citation[27]. Six thermotherapy sessions were delivered at weekly intervals using the first alternating magnetic field applicator for humans (NanoActivator F100, MagForce Nanotechnologies AG, Berlin; ), operating at a frequency of 100 kHz and a variable field strength of 2.5-15 kA/m. Treatment planning and the injection procedure were found to be feasible in all patients. However, the optimal (pre-calculated) distribution of magnetic nanoparticles in the target region could not be achieved due to mechanical resistance of pre-irradiated prostate tissue to the injection of the magnetic fluid. Median amount of magnetic fluid applied was 11.4 mL (4–14 mL). Maximum temperatures of up to 55°C were achieved in the prostates. Median temperatures in 20, 50 and 90% of the prostates (T20, T50 and T90) under constant magnetic field strengths between 4–5 kA/m, which were tolerated throughout the treatment time by all patients, were 41.1° (40.0°–47.4°), 40.8° (39.5°–45.4°) and 40.1°C (38.8°–43.4°), respectively. Median urethral and rectal temperatures were 40.5°C (38.4°–43.6°) and 39.8°C (38.2°–43.4°). The median thermal dose derived from the fit of invasive and non-invasive measurements was 7.8 (3.5–136.4) cumulative equivalent minutes at 43°C in 90% of the prostates (CEM 43°C T90; mean: 20.9 min). Deviations between invasive measurements and calculated temperature distributions were found near to the floor of the bladder, in the urethra (calculations higher than direct measurements) and at the perineum (calculations lower than direct measurements). In the prostate, a satisfactory agreement between measurements and calculations was found Citation[27].
Figure 2. Alternating magnetic field applicator for humans (NanoActivator F100, MagForce Nanotechnologies AG, Berlin) operating at a frequency of 100 kHz and a variable field strength of 0–15 kA/m). A fibre-optic thermometry unit is part of the applicator.
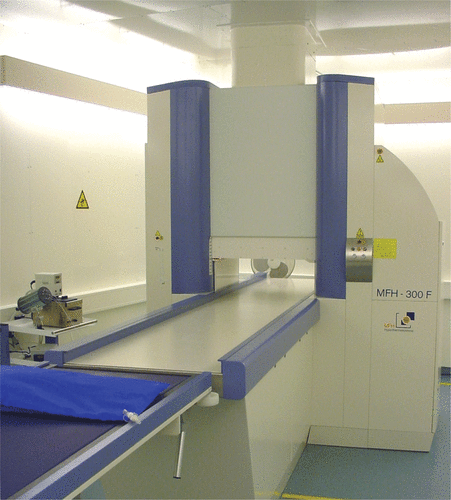
In the CT images the nanoparticle deposits in the prostates were still clearly visible one year after thermotherapy Citation[28]. Particles could also be detected histologically in these patients (). However, repeated heating was not attempted.
Figure 3. Histological image obtained by prostate biopsy one year after direct magnetic fluid injection into the prostate and thermal treatment. Iron-oxide nanoparticles are still present in the prostate tissue (haematoxylin-eosin staining, ×200).
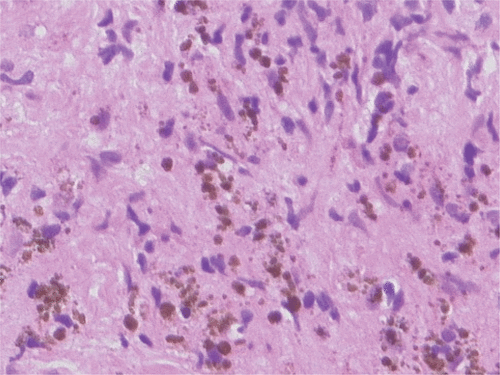
Morbidity, quality of life and outcome of this phase I study was published in a separate report Citation[28]. No systemic toxicity was observed. Temporary bladder drainage due to acute urinary retention occurred in four patients with previous history of urethral stricture/impaired urinary flow rate following radiation therapy. Grade 3 urinary toxicity was noted in two patients, observed only following magnetic nanoparticle injection and subsequent first thermal treatment. Dysuria grade 2 was present in two and grade 1 in three patients. Alternating magnetic field strengths of 4–5 kA/m were tolerated throughout treatment by all patients. Higher magnetic field strengths caused discomfort in the groin or perineal region. In some patients, temperature maxima of up to 44°C were observed at the skin level, typically in folds of the scrotal and anal region, but could be managed by cooling and ventilation. While the intensity of pain in both of these anatomical regions correlated with increasing magnetic field strength during thermal therapy, there was no direct correlation of these side effects with achieved temperatures in the prostates. Late treatment-related morbidity was not observed in this study at a median follow-up of 17.5 months (3–24) in the original publication Citation[28].
Changes in QoL were evaluated during and after magnetic nanoparticle thermotherapy in comparison with the baseline status. There was no significant deterioration of physical functioning, global health status and treatment-related symptoms during the study Citation[28]. A significant deterioration of social functioning (p < 0.0001), role functioning (p = 0.0008), fatigue (p = 0.0154), pain (p = 0.0081), financial difficulties (p = 0.0022), urinary symptoms (p = 0.0445) and sexual functioning (p = 0.0096) were observed. Of these, only social functioning remained impaired three months after thermal therapy. Two patients complained worsening of erectile dysfunction following thermotherapy and received treatment with phosphodiesterase-5-inhibitors.
A decrease of serum PSA was observed in eight patients at the end of treatment (>70% in one patient, 40% in another patient and ≤25% in the remaining patients). Mean duration of PSA-control was 5 months (3–8). All patients have ultimately progressed during follow-up with local progression in seven and distant disease in three patients.
Magnetic nanoparticle thermotherapy combined with permanent brachytherapy
In a separate phase I trial, the feasibility of combined interstitial thermoradiotherapy was evaluated Citation[29]. Eight patients with locally recurrent prostate cancer after definitive radiotherapy received magnetic nanoparticle thermotherapy combined with low dose rate (LDR) brachytherapy (prescription dose 90–100 Gy) as a salvage approach. Transperineal injection of nanoparticle suspension was carried out in the same setting as permanent implantation of 125-iodine seeds under general anaesthesia, guided by TRUS and fluoroscopy.
The procedures of seed implantation and magnetic fluid injection were successful in all cases. Median amount of magnetic fluid applied in this study was 8.5 ml (6.0–12.5 mL). The median T90 achieved was 39.9°C (38.8°–42.3°). The measured and calculated maximum intraprostatic temperatures were 42.4° (40.6°–45.5°) and 41.5°C (40.9°–45.4°), respectively, showing a good correlation between intraluminal urethral temperature measurements and non-invasive calculations. The median CEM 43°C T90 was 5.8 min (1–90) in this series of patients Citation[29].
Only local toxicity was observed. In two patients grade 1–2 perineal pain persisted for several months, most likely related to the permanent seeds rather than to the magnetic fluid. Another patient underwent two transurethral resections in a different institution six and nine months after seeds implantation due to bladder outlet obstruction. In this patient, a rectal fistula to the urinary tract occurred one year after combined permanent seed implantation and thermotherapy and required a temporary colostomy.
Discussion and future perspectives
Clinical hyperthermia of prostate cancer remains a challenging problem. The prostate is shielded anteriorly by fat and bone and surrounded posteriorly and cranially by hollow organs containing air and fluid. The different conductivities of these tissues to radiofrequency or ultrasound waves applied for heating purposes may cause undesired reflexion, scattering or absorption of thermal energy outside the target area in critical regions, while the high intra- and periprostatic perfusion may act as a heat sink. All these phenomena lead to limited achievable temperatures in the prostate using conventional heating techniques Citation[6–13].
In the studies reported in this review, magnetic nanoparticle thermotherapy was demonstrated to be feasible and hyperthermic to thermoablative temperatures could be achieved in the prostates at relatively low magnetic field strengths of 4–5 kA/m Citation[26–29]. Higher field strengths caused local discomfort, originating from two distinct phenomena independent from the presence of nanoparticles, namely boundary effects between tissues of different dielectric constants and conductivity, which can occur between bone surfaces and soft tissues, and increases of current density at skin level, particularly in folds, which may lead to relative hot spots. These effects were only observed during treatment of pelvic tumours, where the cross-section of the body is relatively high. In fact, magnetic field strengths tolerated during magnetic nanoparticle thermotherapy of brain tumours were much higher, e.g. 10–14 kA/m Citation[30]. Since field strengths of up to 15 kA/m can be applied with the applicator used in these studies and given the quadratic increase of SAR with increasing magnetic field strength, significantly higher temperatures could be achieved with this technique. Also, by increasing homogeneity of intraprostatic nanoparticle distribution, lower temperature gradients and higher minimal temperatures in the target region would be attainable Citation[29].
Regarding oncological outcome, PSA declines following thermotherapy were observed in both studies, which suggest potential efficacy of this treatment. However, responses in the monotherapy trial were of limited extent and duration. Due to the heterogeneous patient cohort including patients with multiple pre-treatments and mostly unfavourable prognostic features, the results of this phase I study do not allow a meaningful interpretation of oncological efficacy.
The results of these studies as well as animal experiments performed previously suggest that the technique may in principle be suitable for thermal ablation as a monotherapy or hyperthermia in combination with radiotherapy of prostate cancer. However, since at present average temperatures achieved in the prostates with this technique are hyperthermic, the combination with irradiation is currently being further evaluated. From a radiobiological point of view, simultaneous rather than sequential application of heat and irradiation is considered ideal for thermoradiotherapy Citation[31]. In particular, the combination of mild hyperthermia with LDR irradiation has been proposed for some time as a promising concept, but to our knowledge never realised clinically Citation[32]. In this regard, a phase II study in previously untreated patients with clinically localised prostate cancer and intermediate risk criteria (PSA 10–20ng/mL or Gleason sum 7) is ongoing at the Charité Clinic Berlin. In this trial patients receive LDR brachytherapy (125-iodine seeds, prescription dose 145 Gy) combined with magnetic nanoparticle thermotherapy. Endpoints are biochemical control (PSA), toxicity and QoL. First results from this study can be expected in 5 years.
Conclusions
Interstitial heating using magnetic nanoparticles is feasible in patients with prostate cancer. Treatment-related toxicity can be considered moderate and QoL is only temporarily impaired. Limiting factors of this technique at present are discomfort at higher magnetic field strengths and irregular intratumoural heat distribution. In contrast, deposition of nanoparticles in the prostates is highly durable.
At present there is no technique available either for direct real-time visual control of the magnetic fluid injection, or for reliable imaging of cancer within the prostate. Thus, selective ablation of cancer cells while sparing healthy tissue is not yet possible in this often multifocal and heterogeneous cancer, but may become an interesting issue in the future with improved diagnostic imaging techniques. Until then, improvement of the thermal efficacy of this approach can be achieved by a more homogeneous heat distribution, refinement of the application techniques and by using higher concentrations of the applied magnetic fluid.
Acknowledgements
The corresponding author wishes to thank Prof. S.A. Loening, renowned urologist and pioneer of ferromagnetic seed hyperthermia of prostate cancer, for his inspiration and support that was instrumental in the preclinical and clinical evaluation of magnetic nanoparticle hyperthermia for prostate cancer.
Declaration of interest: Andreas Jordan is a manager and Burghard Thiesen is an employee at MagForce Nanotechnologies AG, Berlin, Germany. The other authors declare that they do not have any affiliations that would lead to conflict of interest. The authors alone are responsible for the content and writing of the paper.
References
- Wust P, Hildebrandt B, Sreenivasa G, Rau B, Gellermann J, Riess H, Felix R, Schlag PM. Hyperthermia in combined treatment of cancer. Lancet Oncol 2002; 3: 487–497
- Hildebrandt B, Wust P, Ahlers O, Dieing A, Sreenivasa G, Kerner T, Felix R, Riess H. The cellular and molecular basis of hyperthermia. Crit Rev Oncol Hematol 2002; 43: 33–56
- Stauffer PR. Evolving technology for thermal therapy of cancer. Int J Hyperthermia 2005; 21: 731–744
- Hartman KB, Wilson LJ, Rosenblum MG. Detecting and treating cancer with nanotechnology. Mol Diagn Ther 2008; 12: 1–14
- Pankhurst QA, Thanh NKT, Jones SK, Dobson J. Progress in applications of magnetic nanoparticles in biomedicine. J Phys D: Appl Phys 2009; 42: 224001
- Van Vulpen M, De Leeuw AA, Raaymakers BW, Van Moorselaar RJ, Hofman P, Lagendijk JJ, Battermann JJ. Radiotherapy and hyperthermia in the treatment of patients with locally advanced prostate cancer: Preliminary results. BJU Int 2004; 93: 36–41
- Anscher MS, Samulski TV, Dodge R, Prosnitz LR, Dewhirst MW. Combined external beam irradiation and external regional hyperthermia for locally advanced adenocarcinoma of the prostate. Int J Radiat Oncol Biol Phys 1997; 37: 1059–1065
- Hurwitz MD, Kaplan ID, Hansen JL, Prokopios-Davos S, Topulos GP, Wishnow K, Manola J, Bornstein BA, Hynynen K. Hyperthermia combined with radiation in treatment of locally advanced prostate cancer is associated with a favourable toxicity profile. Int J Hyperthermia 2005; 21: 649–656
- Algan O, Fosmire H, Hynynen K, Dalkin B, Cui H, Drach G, Stea B, Cassady JR. External beam radiotherapy and hyperthermia in the treatment of patients with locally advanced prostate carcinoma. Cancer 2000; 89: 399–403
- Kalapurakal JA, Pierce M, Chen A, Sathiaseelan V. Efficacy of irradiation and external hyperthermia in locally advanced, hormone-refractory or radiation recurrent prostate cancer: A preliminary report. Int J Radiat Oncol Biol Phys 2003; 57: 654–664
- Tilly W, Gellermann J, Graf R, Hildebrandt B, Weissbach L, Budach V, Felix R, Wust P. Regional hyperthermia in conjunction with definitive radiotherapy against recurrent or locally advanced prostate cancer T3 pN0 M0. Strahlenther Onkol 2005; 181: 35–41
- Deger S, Bohmer D, Turk I, Roigas J, Budach V, Loening SA. Interstitial hyperthermia using self regulating thermoseeds combined with conformal radiotherapy. Eur Urol 2002; 42: 147–153
- Maluta S, Dallòglio S, Romano M, Marciai N, Pioli F, Giri MG, Benecchi PL, Comunale L, Porcaro AB. Conformal radiotherapy plus local hyperthermia in patients affected by locally advanced high risk prostate cancer: Preliminary results of a prospective phase II study. Int J Hyperthermia 2007; 23: 451–456
- Ryu S, Brown SL, Khil MS, Kim SH, Kim JH. Preferential radiosensitization of human prostatic carcinoma cells by mild hyperthermia. Int J Radiat Oncol Biol Phys 1996; 34: 133–138
- Jordan A, Maier-Hauff K, Wust P, Johannsen M. Nanoparticles for thermotherapy. Nanomaterials for cancer therapy, CSSR Kumar. Wiley-VCH, Weinheim 2006; 242–258
- Jordan A, Scholz R, Wust P, Fähling H, Wust P. Magnetic fluid hyperthermia (MFH): Cancer treatment with AC magnetic field induced excitation of biocompatible superparamagnetic nanoparticles. J Magn Magn Mater 1999; 201: 413–419
- Jordan A, Wust P, Fähling H, John W, Hinz A, Felix R. Inductive heating of ferrimagnetic particles and magnetic fluids: physical evaluation of their potential for hyperthermia. Int J Hyperthermia 1993; 9: 51–68
- Jordan A, Wust P, Scholz R, Tesche B, Fähling H, Mitrovics T, Vogl T, Cervos-Navarro J, Felix R. Cellular uptake of magnetic fluid particles and their effects in AC magnetic fields on human adenocarcinoma cells in vitro. Int J Hyperthermia 1996; 12: 705–722
- Jordan A, Scholz R, Wust P, Schirra H, Schiestel T, Schmidt H, Felix R. Differential endocytosis of dextran and silan-coated magnetite nanoparticles and the effect of intracellular hyperthermia on human mammary carcinoma cells in vitro. J Magn Magn Mater 1999; 194: 185–196
- Jordan A, Scholz R, Maier-Hauff K, Johannsen M, Wust P, Nadobny J, Schirra H, Schmidt H, Deger S, Loening SA, et al. Presentation of a new magnetic field therapy system for the treatment of human solid tumors with magnetic fluid hyperthermia. J Magn Magn Mater 2001; 225: 118–126
- Jordan A, Wust P, Scholz R, Mitrovics T, Fähling H, Gellermann J, Vogl T, Cervos-Navarro J, Felix R. Effects of magnetic fluid hyperthermia (MFH) on C3H mammary carcinoma in vivo. Int J Hyperthermia 1997; 13: 587–605
- Jordan A, Scholz R, Maier-Hauff K, Van Landeghem FKH, Waldöfner N, Teichgräber U, Pinkernelle J, Bruhn H, Neumann F, Thiesen B, et al. The effect of thermotherapy using magnetic nanoparticles on rat malignant glioma. J Neurooncol. 2006; 78: 7–14
- Johannsen M, Jordan A, Scholz R, Lein M, Koch M, Deger S, Roigas J, Jung K, Loening SA. Evaluation of magnetic fluid hyperthermia in a standard rat model of prostate cancer. J Endourol 2004; 18: 495–500
- Johannsen M, Thiesen B, Jordan A, Taymoorian K, Gneveckow U, Waldöfner N, Scholz R, Koch M, Lein M, Jung K, et al. Magnetic fluid hyperthermia (MFH) reduces prostate cancer growth in the orthotopic Dunning R3327 rat model. Prostate 2005; 64: 283–292
- Johannsen M, Thiesen B, Gneveckow U, Taymoorian K, Waldöfner N, Scholz R, Deger S, Jung K, Loening SA, Jordan A. Thermotherapy using magnetic nanoparticles combined with external radiation in an orthotopic rat model of prostate cancer. Prostate 2006; 66: 97–104
- Johannsen M, Gneveckow U, Eckelt L, Feussner A, Waldöfner N, Scholz R, Deger S, Wust P, Loening SA, Jordan A. Clinical hyperthermia of prostate cancer using magnetic nanoparticles: Presentation of a new interstitial technique. Int J Hyperthermia 2005; 21: 637–647
- Johannsen M, Gneveckow U, Thiesen B, Taymoorian K, Cho CH, Waldöfner N, Scholz R, Jordan A, Loening SA, Wust P. Thermotherapy of prostate cancer using magnetic nanoparticles: Feasibility, imaging and three-dimensional temperature distribution. Eur Urol 2007; 52: 1653–1661
- Johannsen M, Gneveckow U, Taymoorian K, Thiesen B, Waldöfner N, Scholz R, Jung K, Jordan A, Wust P, Loening SA. Morbidity and quality of life during thermotherapy using magnetic nanoparticles in locally recurrent prostate cancer: Results of a prospective phase I trial. Int J Hyperther 2007; 23: 315–323
- Wust P, Gneveckow U, Johannsen M, Böhmer D, Henkel T, Kahmann F, Sehouli J, Felix R, Ricke J, Jordan A. Magnetic nanoparticles for interstitial thermotherapy – Feasibility, tolerance and achieved temperatures. Int J Hyperthermia 2006; 22: 673–685
- Maier-Hauff K, Rothe R, Scholz R, Gneveckow U, Wust P, Thiesen B, Feussner A, Von Deimling A, Waldöfner N, Felix R, et al. Intracranial thermotherapy using magnetic nanoparticles combined with external beam radiotherapy: Results of a feasibility study on patients with glioblastoma multiforme. J Neurooncol 2007; 81: 53–60
- Kampinga HH, Dikomey E. Hyperthermic radiosensitization: Mode of action and clinical relevance. Int J Radiat Biol 2001; 77: 399–408
- Armour EP, Raaphorst GP. Long duration mild temperature hyperthermia and brachytherapy. Int J Hyperthermia 2004; 20: 175–89