Abstract
Purpose: To investigate the feasibility and validity of real-time guidance using a fusion imaging system that combines ultrasonography (US) and computerised tomography (CT) information in the targeting and subsequent microwave ablation of a liver target inconspicuous on US.
Materials and methods: The study was an experimental ex vivo study in calf livers with internal targets, simulating a focal liver lesion, focused on the accuracy of real-time US using a multimodality fusion imaging system in combination with 15 gauge (G) microwave antennae. US image and pre-procedural CT image were fused by the external markers registration procedure. Microwave antennae were inserted into the liver to ablate the target assisted by the CT-US fusion imaging system. Finally, a post-procedural CT with needles in situ and multiplanar reconstructions were performed to compare with pre-procedural CT information in order to calculate the accuracy of positioning (distance between the needle tip and the target).
Results: Eight insertions were planned and eight ablations were performed in four calf livers. The calf livers were undertaken successfully on external markers registration procedure. The mean registration error in the four livers was 2.1 ± 0.1 mm, 2.8 ± 0.1 mm, 3.4 ± 0.1 mm and 2.3 ± 0.1 mm, respectively. The accuracy of the matched US-CT images was very satisfactory in the fact that it was found there was a mean discrepancy of 1.63 ± 1.06 mm.
Conclusion: Real-time registration and fusion of pre-procedural CT volume images with intraprocedural US is feasible and accurate for microwave (MW) ablation in experimental setting. Further studies are warranted to validate the system under clinical conditions.
Introduction
Thermal ablation therapy, such as radio frequency (RF) ablation or MW ablation, is a widely used method for solid tumours, especially for liver tumours, which has been a curative method for small liver cancer Citation[1]. MW ablation is a promising minimally invasive treatment method for liver tumours, which has been performed by using US, CT or magnetic resonance imaging (MRI) guidance Citation[2–4]. US is real time and allows the body to be scanned from different positions and angles, so compared with CT or MRI guidance, MW ablation under the guidance of US is more convenient. However, there are occasions when liver lesions are invisible to US imaging, but are only optimally visualised on contrast-enhanced CT and magnetic resonance imaging, due to the texture or the location of the lesion Citation[5–7]. In this situation it is difficult or cumbersome to target the lesion, also due to the short duration of the different vascular phases and the lack of real-time imaging guidance with CT and MR machines Citation[8].
Imaging-based navigation is a new technology that allows the fusion of real-time US and preoperative CT or MRI data. This multimodality matching, which has been used in nuclear medicine, radiotherapy, and neurosurgery Citation[9–11], can provide more useful integration of information, such as intuitive 3D information. With the development of magnetic navigation systems, it has been possible to apply this technology to interventional abdominal therapy Citation[12], Citation[13]. The real-time Virtual Navigator (VN) system (Esaote, Genoa, Italy) can display the synchronised pictures of both real-time US and CT or MRI in the same section of the body, simultaneously.
In this study we investigate the feasibility and validity of real-time guidance using a fusion imaging system that combines US and CT information in the targeting and subsequent MW ablation of a liver target inconspicuous on US, based on previous published experiences with RF ablations Citation[14].
Materials and methods
Study design
The study was an experimental ex vivo study in calf livers with internal targets, simulating a focal liver lesion, focused on the accuracy of real-time US using a commercially available multimodality fusion imaging system (MyLab90 System equipped with Virtual Navigator, Esaote) in combination with 15 gauge (G) microwave antennae. First, US image and CT image were fused by the external markers registration procedure. Second, microwave antennae were inserted into the liver to ablate the simulated focal liver lesions assisted by the CT-US fusion imaging system. Finally, a post-procedural CT with needles in situ and multiplanar reconstructions was performed to calculate accuracy of positioning, (distance between the needle tip and the target). Also necrosis areas predicted have been compared with the measured ablated areas.
Target
The targets, used to simulate a focal lesion, were 1.5-mm stainless steel pellets inconspicuous on conventional US but with high attenuation on CT. Inserted markers are not clearly visible in the ultrasound image since they are easily confused with the air present inside the ex-vivo veal liver vessels. In order to avoid the disruption of the superior liver surface that may also affect US scanning quality, targets have been implanted into the liver parenchyma from the lateral liver side, using a 14G trocar stylet with percutaneous microwave coagulation therapy (PMCT)-guided needle (15 cm) for osteo-medullary biopsy (PMCT NSP, Hakko Shoji, Tokyo, Japan). In the first calf liver two pellets have been positioned into different parts, one in the second liver, two in the third liver and three in the fourth liver.
Microwave system
The microwave unit (KY-2000, Kangyou Medical, China) consists of three independent MW generators with frequency of 2450 MHz, three flexible coaxial cables and three water-pumping machines, which can drive three 15G and 18 cm long cooled-shaft antennae (1.1 cm antenna tip) simultaneously. The MW unit generators are capable of producing 1–100 W of power. The cooled-shaft antennae are coated with Teflon which is used to prevent adhesion. Inside the antenna shaft, there are dual channels through which distilled water is circulated by a peristaltic pump, continuously cooling the shaft to prevent overheating.
Fusion imaging system
The system consists of an ultrasound real time scanner equipped with an electromagnetic tracking device enabling the image fusion based on the geometry data and the content of the second modality dataset. A tracking device is mounted on the transducer, which provides the position and orientation in space during a standard ultrasound scanning of the patient. The information from the tracking device and the 3D dataset of the second modality (CT) is combined to compute a virtual slice image that is spatially consistent with the displayed US image. Virtual Navigator displays on the monitor both the ultrasound and the second modality image in the same dimension and cut plane. The system specifications are provided in .
Table I. System specifications.
Fusion imaging system set-up
Before the intervention the study has to be structured as follows:
In order to correlate (register) the CT dataset with the US scan, 1.5-mm radio-opaque fiducial markers (X-Spots, Beekley, Bristol, CT) have been placed on the calf livers surfaces (15 markers on liver 1, 8 on liver 2, 9 on liver 3 and 11 on liver 4).
1-mm slice thickness acquisition has been performed (SOMATOM Sensation 16, Siemens, Germany) and the CT DICOM series loaded in MyLab90 Virtual Navigator system.
1.5-mm stainless steel pellets, used to simulate a focal lesion, have been localised and marked as targets on CT DICOM series ().
The positions of all the fiduciary markers in the CT volume () were identified and numbered, verifying the cursor position displayed on the three 2D views (sagittal, coronal, axial).
By using the registration pen (), for the VN external marker registration procedure, the positions of all the fiduciary markers have been correlated in accordance with the sequence number given during the previous step.
At the end of the registration, an error value will be calculated showing:
The quality of the registration in comparison to the other markers for each marker. For each marker an error value is calculated, which shows the quality of the registration (in %) in comparison to the other markers.
The average registration error for global registration (). The software calculates the error based on the RMS (Root Mean Square) value between the acquired points and the points marked on the volume of the second modality (gold standard).
Based on the markers points, the system fits the virtual volume minimising the distance between the identified point in the virtual volume and the acquired actual one. This implies that even if the points are not acquired well (human error), the system is able to identify the best position of the virtual volume in the real space in order to minimise the error. Real-time US of the calf livers was then performed using a 3.5 MHz curvilinear probe, having in a VN environment the ultrasound live image and the image taken from a second modality (CT dataset) displayed side by side. Additionally, the second modality image may be overlapped on the current ultrasound situation.
Targeting and microwave ablation
A sterilisable biopsy kit (ABS 421, Esaote) and sterilisable mounting brackets with disposable needle guide (DBS421/431, Protek Medical, Coralville, IA), with biopsy needle angles of 20° and 30°, have been used to guide the needle insertions. The target, well visible in the CT (and inconspicuous on US) has been ‘targeted’ using the information coming from the CT image and using the virtual needle line to better identify the path. A 15 G MW antenna (Kangyou Corporation, China) was inserted into the liver using a real-time guidance and biopsy kit. The virtual needle was displayed computing its projection on the biopsy line (). The tip of the needle should ideally be placed in the target, but microwaves’ straight antennae cannot be fixed to the parenchyma after initial positioning and were at risk for movement after the placement, especially during the transportation of the liver into the CT scanner. For this reason and for the non-penetrable nature of the pellet, we considered using a precise known distance to the pellet along the planned trajectory Citation[14]. In order to steady the needle as much as possible, avoiding any further movement after MW ablation, the depth and especially the insertion trajectory have been taken into account; preferring as much as possible coronal plane insertions rather than axial and sagittal insertions. After insertion of the antenna, MW ablation was performed with a 2450 MHz MW generator (KY-2000, Kangyou, Nanjing, China) in continuous mode at 50 W for 10 min. Continuous real-time US monitoring was performed during MW ablation.
Figure 2. In two insertions we have done an accuracy evaluation, performing an insertion at high depth, of the Virtual Navigator estimation (distance between needle tip and lesion).
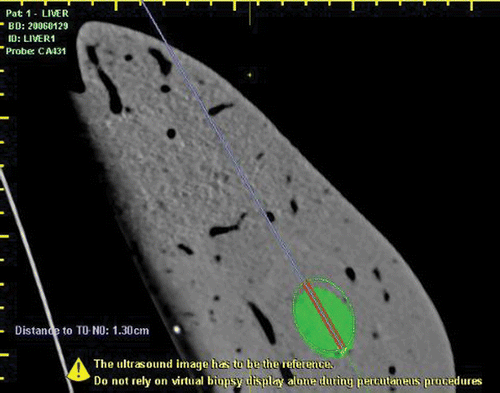
The target is heat resistant and therefore remained intact, allowing visualisation on a repeat CT post procedure. Eight insertions were planned and executed, and eight ablations were performed in total in four calf livers. In four insertions we have done an accuracy evaluation, performing an insertion at high depth (between 120 and 171 mm) in consideration of the Virtual Navigator estimation (distance between the needle tip and the lesion). In the other four insertions the tip of the needle has been placed as close as possible to the lesion, using graphical matching of the navigator (without numerical information). Also, in these four cases the Virtual Navigator distance estimation was recorded in order to compare precise distances post-ablation. After ablation, a second CT scan with needles in situ was performed in order to assess the accuracy of needle positioning. Unenhanced MDCT of the liver was performed with a 1.2-mm collimation and a reconstruction interval of 1 mm, covering the entire liver. The distances between the tip of the MW antenna and the target were calculated on multiplanar reconstructed CT images. The liver was then cut along the antenna axis and the ablation zone was measured.
Data analysis
Average registration errors due to matching misalignment expressed in cm by the navigation system were converted into mm. Results were expressed as mean ± SD for continuous data.
Target accuracy, intended as distance between needle tip and target, was initially registered in cm using Virtual Navigator distance predictions. Distance annotations have been taken during the probe removal from the biopsy attachment (microwave burning already started).
The comparison accuracy was performed measuring the distance in cm on second CT images with needles in situ.
The discrepancies between real time fusion imaging and post-procedural CT were calculated in mm.
Necrosis area parameters () have been measured after each treatment in cm and average values have been calculated. Results were expressed as mean ± SD for continuous data.
Results
An external markers registration procedure was successfully undertaken on the calf livers. The mean registration error in the four livers was 2.1 ± 0.1 mm (15 markers used), 2.8 ± 0.1 mm (8 markers used), 3.4 ± 0.1 mm (9 markers used) and 2.3 ± 0.1 mm (11 markers used), respectively. The accuracy of the matched US-CT images, as subjectively assessed by the operator, was considered satisfactory in all cases.
Each pellet (target) was used for US-CT-guided targeting insertions, and thanks to VN prediction estimation and fusion imaging graphical matching, the data have been registered (). The needle-target distances on post-procedural CT with needles in situ have been measured (), which was performed in order to verify the VN estimation accuracy. The accuracy of the matched US-CT images was very satisfactory in that it was found there were discrepancies of 1 mm in lesion A (needle depth 15 cm), 0 mm in lesion B (needle depth 10.5 cm), 1 mm lesion C (needle depth 17.1 cm), 1 mm in lesion D (needle depth 15 cm), 3 mm in lesion E (needle depth 13 cm), 2 mm in lesion F (needle depth 12 cm), 3 mm in lesion G (needle depth 12 cm) and 2 mm in lesion H (needle depth 8.2 cm). () The mean discrepancy was 1.75 ± 0.96 mm in four insertions performed at high depth considering the VN estimation, and the mean discrepancy was 1.5 ± 1.29 mm in the other four insertions in which the tip of the antenna has been placed as close as possible to the lesion. There was no significant difference in discrepancy between the two ways of insertion (p = 0.766). The mean discrepancy of all eight insertions was 1.63 ± 1.06 mm.
Figure 4. The needle-target distance on post-procedural CT with needles in situ was measured in order to assess the accuracy of needle positioning.
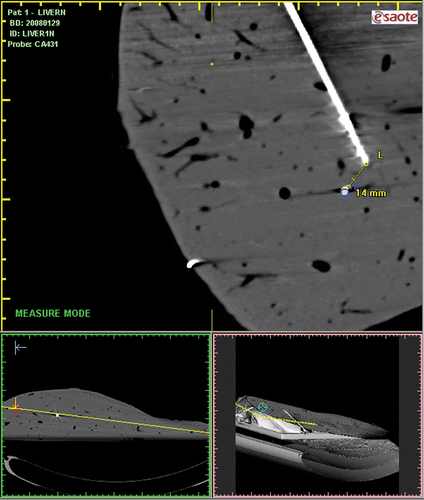
Table II The distance between target and antenna tip(T-T) in navigation and in second CT imaging.
The necrosis areas generated by the Kangyou microwave needle have been measured on the calf liver, in order to verify their accordance with the expected area computed by the navigator system, having an L-diameter (long ellipse diameter) of 3.51 ± 0.19 cm, an S-diameter (short ellipse diameter) of 2.34 ± 0.09 cm and a T-distance (distance from the antenna tip to the anterior margin of the ablation area) 0.54 ± 0.17 cm.
Discussion
MW ablation is one of the effective localised thermal ablation methods for liver tumours. Image guidance is essential in the treatment of liver tumours using percutaneous MW ablation techniques. Apart from careful pre-procedure planning and elaborate post-procedure evaluation, accurate intra-procedure targeting, monitoring and controlling play a critical role in the success of the technique Citation[16]. MW ablation is performed more widely by using US guidance, compared with CT or MRI guidance, due to the character of real-time imaging. However, there are occasions when the liver lesion is inconspicuous on US image but is only optimally visualised on contrast enhanced CT or MRI, due to the texture or the location of the lesion. In this situation the physician conventionally makes use of this information by mentally registering the anatomical information from offline modalities (contrast enhanced CT or MRI) to the modality (US) used for guiding the actual procedure. This process can be inexact and may be prone to human error and inaccuracies.
Therefore it would be ideal if the MW ablation can be performed with real-time US coupled with multiplanar and interactive capabilities (CT or MR images) Citation[16]. Image fusion, the process of aligning, and superimposing images obtained using two different imaging modalities, is a rapidly evolving field of interest, with its own specific operational conditions Citation[16], Citation[17]. In some studies Citation[13], Citation[14] image fusion navigation techniques have been introduced as a promising method in the procedure of RF ablation in liver tumours. To our knowledge, this is the first report concerning MW ablation by using an image fusion system that matches real-time US and CT.
In our feasibility study we demonstrated a high and consistent level of matching accuracy. The calf livers successfully underwent an external markers registration procedure. The mean registration error in the four livers was 2.1 ± 0.1 mm (15 markers used), 2.8 ± 0.1 mm (8 markers used), 3.4 ± 0.1 mm (9 markers used) and 2.3 ± 0.1 mm (11 markers used), respectively. Apart from external markers, registration of CT data to intraprocedural US images using specific anatomic (e.g. portal and hepatic veins) and topographical (xiphoid sternum and umbilicus) landmarks can also be accomplished in real-time during US examination, however, registration using internal markers was not used in our study.
The air-filled vessels in ex vivo livers cause an even worse visualisation of the underlying liver parenchyma. We used a very small target in size (1.5 mm) which was undetectable at US. This ideally represents the situation of a tiny lesion that is visible only at CT. The navigation system therefore represented the only guidance for the procedures. By deciding to insert the needle only once for each targeting/ablation procedure, we reproduced the need for minimal invasiveness. We performed two methods of insertion for the accuracy evaluation in our study. The mean discrepancy was 1.75 ± 0.96 mm in four insertions performed at high depth considering the VN estimation, and the mean discrepancy was 1.5 ± 1.29 mm in four other insertions where the tip of the antenna was placed as close as possible to the lesion. There was no significant difference in discrepancy between the two methods of insertions (p = 0.766). The mean discrepancy of all eight insertions was 1.63 ± 1.06 mm. So the accuracy of the matched US-CT images was very satisfactory in our study.
CT-US fusion imaging system with magnetic navigation has several important features in the application of MW ablation therapy. The first is compatibility. The real-time US imaging modality can be registered with preoperative CT or MRI data, the benefits of which are the simultaneous acquisition of high-resolution image, 3D spatial orientation, and real-time image. The second feature is synchronicity. This guidance technique is based on immediate feedback to highlight small liver lesions that cannot be clearly visualised with US. Therefore we were able to insert the MW antenna into lesions invisible on US using this technique.
The main limitation of the study is that it was conducted in an ideal experimental setting, without respiratory excursion and subject motion in this ex vivo model; however, either or both of these factors will affect the accuracy of registration in clinical application. The markers will cling to the surface of a patient's abdomen in clinical application, and the accurate dimensional position of markers will change following the patient's respiration, so when we complete the registration the CT image is stable while the US image changes following the patient's respiration. Several techniques may be available to decrease the influence of respiratory exertion and subject motion: first, a real-time 3D US image acquisition and display will add more merit to the proposed multi-image modality algorithm; second, a minimal invasive fiduciary, non-invasive miniature position sensor directly on the liver surface, or the internal anatomical landmarks of the liver may be a possible candidate tool for tracking liver movement.
In conclusion, real-time registration and fusion of pre-procedural CT volume images with intraprocedural US is feasible and accurate for MW ablation in an experimental setting. Further studies are warranted to validate the system under clinical conditions.
Declaration of interest: The authors report no conflicts of interest. The authors alone are responsible for the content and writing of the paper.
References
- Bruix J, Hessheimer AJ, Forner A, Boix L, Vilana R, Llovet JM. New aspects of diagnosis and therapy of hepatocellular carcinoma. Oncogene 2006; 25: 3848–3856
- Dong BW, Liang P, Yu XL, Su L, Yu D, Cheng Z, Zhang J. Percutaneous sonographically guided microwave coagulation therapy for hepatocellular carcinoma: Results in 234 patients. Am J Roentgenol 2003; 180: 1547–1555
- Sato M, Watanabe Y, Tokui K, Kawachi K, Sugata S, Ikezoe J. CT-guided treatment of ultrasonically invisible hepatocellular carcinoma. Am J Gastroenterol 2000; 95: 2102–2106
- Kurumi Y, Tani T, Naka S, Shiomi H, Abe H, Endo Y, Morikawa S. MR-guided microwave ablation for malignancies. Int J Clin Oncol 2007; 12: 85–93
- Minami Y, Kudo M, Kawasaki T, Chung H, Ogawa C, Shiozaki H. Treatment of hepatocellular carcinoma with percutaneous radiofrequency ablation: Usefulness of contrast harmonic sonography for lesions poorly defined with B-mode sonography. Am J Roentgenol 2004; 183: 153–156
- Meloni MF, Goldberg SN, Livraghi T, Calliada F, Ricci P, Rossi M, Pallavicini D, Campani R. Hepatocellular carcinoma treated with radiofrequency ablation: Comparison of pulse inversion contrast-enhanced harmonic sonography, contrast-enhanced power Doppler sonography, and helical CT. Am J Roentgenol 2001; 177: 375–380
- Rode A, Bancel B, Douek P, Chevallier M, Vilgrain V, Picaud G, Henry L, Berger F, Bizollon T, Gaudin JL, Ducerf C. Small nodule detection in cirrhotic livers: Evaluation with US, spiral CT, and MRI and correlation with pathologic examination of explanted liver. J Comput Assist Tomogr 2001; 25: 327–336
- Kliewer MA, Sheafor DH, Paulson EK, Helsper RS, Hertzberg BS, Nelson RC. Percutaneous liver biopsy: A cost benefit analysis comparing sonographic and CT guidance. Am J Roentgenol 1999; 173: 1199–1202
- Tsukamoto E, Ochi S. PET/CT today: System and its impact on cancer diagnosis. Ann Nucl Med 2006; 20: 255–267
- Ma CM, Paskalev K. In-room CT techniques for image-guided radiation therapy. Med Dosim 2006; 31: 30–39
- Grunert P, Darabi K, Espinosa J, Filippi R. Computer-aided navigation in neurosurgery. Neurosurg Rev 2003; 26: 73–99
- Hong J, Nakashima H, Konishi K, Ieiri S, Tanoue K, Nakamuta M, Hashizume M. Interventional navigation for abdominal therapy based on simultaneous use of MRI and ultrasound. Med Bio Eng Comput 2006; 44: 1127–1134
- Maeda Takashi, Hong Jaesung, Konishi Kozo, Nakatsuji Takanori, Yasunaga Takefumi, Yamashita Yo-ichi, Taketomi Akinobu, Kotoh Kazuhiro, Enjoji Munechika, Nakashima Hideaki, et al. Tumor ablation therapy of liver cancers with an open magnetic resonance imaging-based navigation system. Surg Endosc 2009; 23: 1048–1053
- Crocetti L, Lencioni R, Debeni S, See TC, Pina CD, Bartolozzi C. Targeting liver lesions for radiofrequency ablation: An experimental feasibility study using a CT–US fusion imaging system. Invest Radiol 2008; 43: 33–39
- Goldberg SN, Grassi CJ, Cardella JF, Charboneau JW, Dodd GD, 3rd, Dupuy DE, Gervais D, Gillams AR, Kane RA, Lee FT Jr, et al. Image-guided tumor ablation: Standardization of terminology and reporting criteria. Radiology 2005; 235: 728–739
- Besl PJ, McKay ND. A method for registration of 3-D shapes. IEEE Trans Pattern Anal Machine Intell 1992; 4: 239–256
- van den Elsen PA, Pol EJD, Viergever MA. Medical image matching a review with classification. IEEE Eng Med Biol 1993; 2: 26–39