Abstract
Purpose: Enediynes are potent inducers of DNA damage, but their clinical usefulness has been limited. Here we report the thermal enhancement of cytotoxicity of two novel metalloenediyne compounds at concentrations that are either not or minimally cytotoxic at 37°C, and present evidence regarding possible mechanisms for enhanced cytotoxicity.
Materials and methods: HeLa cells were exposed to (Z)-N,N′-bis[1-pyridyl-2-yl-meth-(E)-ylidene]octa-4-ene-2,6-diyne-1,8-diamine (PyED) (which becomes metallated in culture medium) or ((Z)-N,N′-bis[quinolin-2-yl-meth-(E)-ylidene]octa-4-ene-2,6-diyne-1,8-diamine)zinc(II) chloride (QuinED · ZnCl2) at 37°C or 42.5°C for 1 h, and clonogenic survival was compared after treatment at each temperature. Analyses of cell cycle progression and mode of death were performed after treatments.
Results: Treatment with PyED or QuinED · ZnCl2 resulted in a significant decrease in cell survival when cells were treated with drug at 42.5°C compared to 37°C. Enhanced cytotoxicity was attributed to increased apoptosis. However, perturbation of the cell cycle may also play a role. Cells which were only heated or exposed to PyED at 37°C experienced significant G2/M blocks that were eliminated when PyED and heat were administered simultaneously, suggesting that combined treatments override cell cycle arrests normally observed with each agent individually. Conversely, cells heated during treatment with QuinED · ZnCl2 displayed an increased G2/M arrest compared to treatment at 37°C.
Conclusions: With improvements in site-specific heat delivery to tumours, systemic administration of non-toxic metalloenediynes coupled with localised hyperthermia may improve selective enediyne activation/targeting. Therefore PyED and QuinED · ZnCl2, which show significantly enhanced cytotoxicity at elevated temperatures, may represent viable candidates for thermochemotherapy.
Introduction
Enediynes are a group of potent antibiotic DNA binding agents that position themselves within the minor groove; upon activation to a 1,4-dehydrobenzene diradical intermediate, these compounds abstract hydrogen atoms from the opposing deoxyribose chains, causing double strand breaks (DSBs) and cell death Citation[1–3]. Although enediynes have been evaluated for their potential as chemotherapeutic agents in clinical trials worldwide, their utility in the oncology clinic has been limited Citation[4]. Perhaps the greatest challenges centre on their ease of thermodynamic activation (often <37°C), the unspecific nature of their potent diradical intermediates, non-selective activation, lack of resistance to biological radical quenchers, and difficulty of synthesis Citation[5]. Factors that influence enediyne reactivity include ‘critical distance’ (the distance between the two carbon alkyne termini), the difference in strain energy between the unactivated and transition state, concentration of the trapping agents, and which functional groups are present in addition to the enediyne core Citation[6].
Many investigators have attempted to overcome these challenges, with special attention devoted toward controlling Bergman cycloaromatisation (leading to diradical intermediate formation), chemical- and/or photo-sensitive triggering mechanisms Citation[7], or selective recognition and targeted delivery strategies Citation[8], Citation[9]. Modulation of cycloaromatisation has been achieved in many instances through complexation with transition metals, to create metallated enediynes, in order to control critical distance and thus, activation Citation[10]. Activation strategies have largely been centred on UV photo-excitation, but poor tissue transparency to UV light limits the in vivo practicality of this approach Citation[5].
Since enediyne activation is very sensitive to heat, hyperthermia treatment may provide a practical solution to selective drug activation in vivo. Improvements in site-specific heat delivery to a tumour lend credence to the notion that relatively non-toxic drug doses, administered systemically, can be selectively activated at tumour sites with localised heat treatment. Moreover, it is well established that hyperthermia sensitises cells to ionising radiation Citation[11], Citation[12]. Much of this enhanced toxicity is attributed to inhibition of repair of DSBs induced by ionising radiation Citation[12–17]. Under the right conditions, hyperthermia may facilitate site-specific drug activation, and since many enediynes may act as radiomimetics by inducing DSBs Citation[18], Citation[19], hyperthermia may inhibit repair of drug-induced DSBs, resulting in enhanced cell killing.
In the present study we examined the effects of hyperthermia on the cytotoxicity of two novel metalloenediynes, PyED (which becomes metallated when diluted into cell culture medium) and QuinED · ZnCl2 on HeLa CCL-2 cells, and conducted initial experiments to determine the potential mechanisms or pathways by which hyperthermia enhances metalloenediyne cytotoxicity. These compounds were designed to have a unique bimodal activation profile where the organic framework is thermally stable over 24 h, but can be activated instantaneously in the presence of specific divalent metal ions at slightly elevated temperatures Citation[10]. The selective activation conveys in these drugs the potential for less systemic toxicity and, when coupled with a specific physical delivery mechanism, it is envisioned that these drugs could be administered at doses far lower than previously tested agents from the enediyne family, which could further decrease overall systemic toxicity.
Materials and methods
Synthesis of (Z)-N,N′-bis[quinolin-2-yl-meth-(E)-ylidene]octa-4-ene-2,6-diyne-1,8-diamine (QuinED)
Solutions of 1,8-diaminooct-4-ene-2,6-diyne (33.1 mg, 0.2 mmol) and 2-quinolinecarboxaldehyde (81.2 mg, 0.5 mmol) in dichloromethane were stirred over Na2SO4 at room temperature. After 4 h, charcoal (1 g) was added and the reaction stirred for an additional 30 min. The reaction mixture was filtered and solvent removed in vacuo to yield the product as a deep yellow oil. Yield: 89.6%; 1H NMR (300 MHz, 298 K, CDCl3): 8.99 (s, 2H), 8.11 (m, 6H), 7.80 (d, J = 8.1 Hz, 2H), 7.71 (t, J = 7.5 Hz, 2H), 7.55 (t, J = 7.5 Hz, 2H), 6.01 (s, 2H, CH), 5.01 (s, 4H, NCH2); 13C NMR (300 MHz, 298 K, CDCl3): 163.57 (CH), 154.78 (Cquat), 147.94 (Cquat), 136.57 (CH), 129.87 (CH), 129.87 (CH), 128.86 (Cquat), 127.78 (CH), 127.56 (CH), 119.79 (CH), 118.61 (CH), 91.96 (Cquat), 86.03 (Cquat), 48.16 (NCH2); MS (MALDI) m/z: 413 (M+ + H).
Synthesis of ((Z)-N,N ′-bis[quinolin-2-yl-meth-(E)-ylidene]octa-4-ene-2,6-diyne-1,8-diamine)zinc(II) chloride (QuinED · ZnCl2)
The Zn(II) complex (QuinED · ZnCl2) was prepared in situ by incubation of QuinED with ZnCl2 in MeOH at −78°C for 1 h followed by removal of the solvent under vacuum to yield the product as a red-brown solid.
Synthesis of (Z)-N,N ′-bis[1-pyridyl-2-yl-meth-(E)-ylidene]octa-4-ene-2,6-diyne-1,8-diamine (PyED)
This compound was prepared as originally described by Rawat and Zaleski Citation[20].
Structures
Structures for each compound are shown in . After purification, the compounds were stored at −20°C. It should be noted that PyED readily forms a Mg complex upon reacting with MgCl2 in equal molar ratios; thus, the presence of Mg2+ in the cell culture medium (see below) used for dilutions may promote formation of metalloenediyne constructs in situ. Formation of metallic complexes with other metal ions (e.g. trace metals such as Zn) introduced by supplementation of the culture medium with calf serum is also possible (unpublished data).
Cell culture and drug treatments
Human HeLa CCL-2 cells were cultured in McCoy's 5A modified medium with L-glutamine (Mediatech, Manassas, VA) supplemented with 10% iron-supplemented bovine calf serum (Hyclone, Logan, UT). Three days prior to experiments, 2 × 105 cells were plated into 25 cm3 (T-25) tissue culture flasks containing 5 mL medium and grown to ∼90% confluence (∼1 × 106 asynchronous cells). Enediyne compounds were dissolved in DMSO to make stock concentrations on the day of each experiment. Cell growth medium containing DMSO only or final concentrations of each compound was prepared immediately before treatment of the cells.
Clonogenic survival experiments
HeLa cells were plated into T-25 flasks ∼72 h prior to exposure to enediyne compounds or vehicle (1.5% DMSO, final concentration in medium) for 1 h at either 37°C or 42.5°C. For cells receiving heat treatments, flasks were immersed in a water bath (42.5° ± 0.1°C) during exposure to the compounds. A control sample with medium containing no DMSO at 37°C was also prepared. After 1 h, medium containing the drug or vehicle was aspirated off the cells and the monolayer was washed with 5 mL medium. The cells were trypsinised and resuspended in medium at 37°C. Cells were counted with a Z2 particle count and size analyser (Beckman Coulter, Brea, CA) and re-plated into new flasks (in triplicate) containing 5 mL medium. Flasks were maintained for 10–14 days in an incubator at 37°C and 5% CO2 until visible colonies had formed. Colonies were fixed with 1:3 mixture of glacial acetic acid:methanol, rinsed with water, and stained with crystal violet. Colonies of 50 cells or more were counted. Surviving fractions were normalised to plating efficiencies obtained from vehicle controls at either temperature. Standard error of the mean (SEM) for each treatment was calculated from multiple experiments. Treatment of cells with 1.5% DMSO for 1 h at 37°C was non-toxic; there was no difference in surviving fraction between cells exposed to 1.5% DMSO and no DMSO at 37°C for 1 h. The surviving fraction of cells exposed to 1.5% DMSO for 1 h at 42.5°C was 0.768 (SEM ± 0.033) when normalised to cells exposed to 1.5% DMSO at 37°C for 1 h.
Apoptosis detection assays
Cells were plated as described above for survival experiments, and exposed to medium containing vehicle (1.5% DMSO) or various enediyne concentrations for 1 h at either 37°C or 42.5°C. After 1 h, the medium containing the drug or vehicle was removed, and cells were washed with 5 mL fresh medium. Cell samples were prepared at various times after treatment. Cells were dislodged from the flasks with trypsin and re-suspended in medium at 37°C. An Annexin V-EGFP apoptosis detection kit (BioVision, Mountain View, CA) was used to prepare 5 × 105 cells per sample for flow cytometric analysis. Cell suspensions were centrifuged and medium was aspirated. The pellet was re-suspended in 500 µL 1X binding buffer as per manufacturer's instructions. Five µL of Annexin V-EGFP and 5 µL propidium iodide (50 µg/mL) was added to the suspension. The samples were incubated in the dark for 5 min at room temperature and stored on ice for up to 1 h prior to flow cytometric analysis. Samples were analysed using a FACScan flow cytometer (Becton-Dickinson, Franklin Lakes, NJ), and the data analysed using CellQuest Pro software (Becton-Dickinson). Bivariate plots were generated and identical quadrants were set for samples for each treatment. Apoptotic fractions were plotted as a function of time after treatment, and SEMs for each time point were calculated from multiple experiments.
Cell cycle analysis
Cells were plated as described above and treated with enediyne compounds or 1.5% DMSO for 1 h by incubating cells at 37°C or 42.5°C. At various times after the treatment, cells were trypsinised, a single cell suspension was obtained, and 106 cells were centrifuged and washed in 2 mL PBS at 4°C. The supernatant was decanted, and the pellet was re-suspended in 2 mL PBS. Five mL of 100% ethanol at −20°C was added drop-wise to the cell suspension while gently vortexing. Samples were kept at 4°C for up to 14 days prior to flow cytometric analysis. On the day of the analysis, samples were centrifuged and washed in 1 mL PBS at 4°C. The pellet was resuspended in 1 mL propidium iodide staining buffer (1 mg/mL sodium citrate, 3 µL/mL triton-× 100, 50 µg/mL propidium iodide, 100 µg/mL DNase-free RNase, and deionised water) for 15 min at room temperature. The samples were centrifuged and re-suspended in 1 mL PBS and analysed using a FACScan flow cytometer and ModFit LT software (Verity Software House, Topsham, ME).
Results
Enhancement of enediyne cytotoxicity by hyperthermia
Since enediyne activation is dependent upon temperature, we sought to determine whether the cytotoxicity of QuinED · ZnCl2 and PyED, would be enhanced when administered to cells during hyperthermia treatment. Treatment with 10 µM PyED for 1 h at 37°C was relatively non-toxic, and the 37°C survival curve exhibited an initial shoulder region up to about 20 µM (). The slope of the survival curve then steepened, but eventually plateaued. The survival curve after treatment of cells with QuinED · ZnCl2 for 1 h at 37°C also possessed a broad shoulder. Treatment with QuinED · ZnCl2 was relatively non-toxic up to a concentration of ∼100 µM (); at higher concentrations, cell survival then approximated an exponential function of dose.
Figure 2. Potentiation of enediyne toxicity by hyperthermia. HeLa CCL-2 cells were treated with various concentrations of either PyED or QuinED · ZnCl2 for 1 h at 37°C or 42.5°C. Surviving fraction was normalised to vehicle only (1.5% DMSO) for each respective temperature. Error bars represent SEM (n = 2 for drug curves and n = 4 for DMSO only curves). (A) PyED; (B) QuinED · ZnCl2.
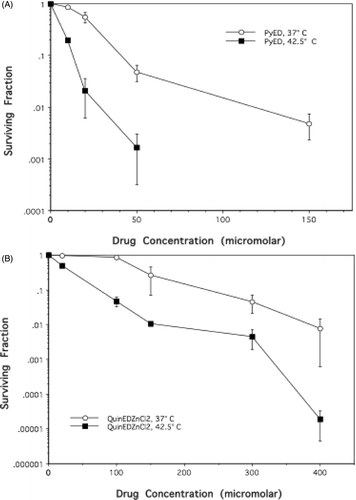
The cytotoxicity of both enediyne derivatives was potentiated when cells were heated at 42.5°C during the 1 h drug treatment ( and ). Thermal enhancement of cell killing was observed at all concentrations tested for each compound, as hyperthermia eliminated the shoulder of the survival curve that was observed when both drugs were administered at 37°C. Cell survival after treatment with PyED at 42.5°C was an exponential function of drug dose. Cytotoxicity was increased by at least an order of magnitude when cells were treated with 20–150 µM PyED () or 100–400 µM QuinED · ZnCl2 during heat treatment, compared to treatment at 37°C.
Heating during enediyne treatment increases the apoptotic fraction
While treatment of cells with 100 µM QuinED · ZnCl2 and 20 µM PyED at 37°C resulted in significantly different surviving fractions (0.835 ± 0.07 and 0.499 ± 0.014, respectively), these concentrations were selected for subsequent studies because cell survival after exposure to 42.5°C heating for 1 h in the presence of the drugs was similar (surviving fractions were 0.04 and 0.021, respectively). Thus, any differences in mode of death or cell cycle progression could not simply be attributed to large differences in cell survival between the two populations.
Light microscopic examination suggested that most cells that had been heated during drug treatment underwent apoptotic death when observed at various times after treatment (not shown). Flow cytometric analysis of annexin V and propidium iodide staining was used to confirm that apoptosis was the primary mode of death for cells exposed to enediynes at 37°C and 42.5°C, and determine apoptotic fraction. In the annexin V/PI staining assay, apoptotic cells are positive for annexin V but negative for PI staining, while necrotic cells or late apoptotic cells stain positive for both annexin and PI. In the experiments described below, cells incubated with DMSO only (vehicle only) served as untreated controls.
Cells were treated with either 20 µM PyED or 100 µM QuinED · ZnCl2 diluted in DMSO in McCoy's 5 A medium for 1 h at 37°C or 42.5°C. At various times after drug treatment, cells were analysed for relative changes in Annexin V and PI fluorescence using flow cytometry. shows bivariate plots (Annexin V-EGFP fluorescence versus PI fluorescence) for cells incubated for 24 h at 37°C after treatment of cells with PyED or QuinED · ZnCl2 at 37°C or 42.5°C, which coincided with the time at which the maximum apoptotic fraction was observed. Cell populations treated with DMSO only at 37°C showed minimal apoptosis (6.4%) (). Hyperthermia treatment alone induced only a minimal increase in apoptosis at 24 h after the heat treatment, compared to unheated control cells (∼7% greater than untreated cells). Approximately 35% of cells were apoptotic when measured 24 h after treatment with PyED at 37°C. However, ∼59% of cells treated with PyED at 42.5°C were apoptotic 24 h after treatment (, 3B). Additionally, we found that ∼36% of cells were apoptotic when measured 24 h after treatment with QuinED · ZnCl2 at 37°C, and ∼61% of cells treated with QuinED · ZnCl2 at 42.5°C were apoptotic 24 h after treatment (, B).
Figure 3. Alterations in the mode of death after treatment with PyED or QuinED · ZnCl2 for 1 h at 37°C or 42.5°C. Apoptosis was measured using the Annexin V/PI assay as described in the Materials and methods section. (A) Bivariate plots of PI (y-axis) versus Annexin V-EFGP (x-axis) fluorescence. HeLa CCL-2 cells were incubated with DMSO or drugs for 1 h at 37°C or 42.5°C for 1 h and fixed 24 h after incubation. Percentage of cells in lower right quadrant was taken as the apoptotic fraction. (B) Apoptotic fractions obtained from bivariate plots were plotted as a function of time for each enediyne treatment. Y-error bars represent SEM (n = 2 for drug curves and n = 4 for DMSO only curves).
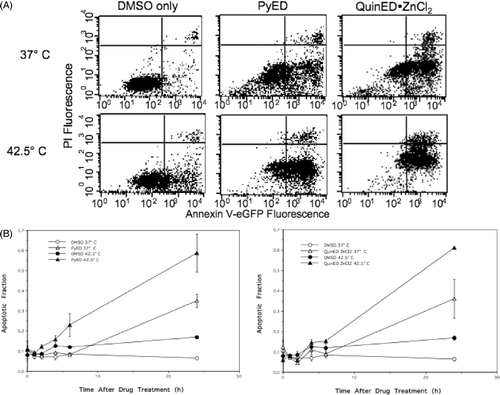
Perturbation of cell cycle progression after combined treatment
An analysis of cell cycle progression revealed that cells heated at 42.5°C for 1 h (no drug, DMSO only) experienced a large G2/M block 18 h post-treatment, with recovery of cell cycle progression by 24 h ( and ). Cells exposed to 20 µM PyED at 37°C for 1 h experienced a similar G2/M block at 18 h, and recovery by 24 h. However, cells exposed to 20 µM PyED during heating at 42.5°C for 1 h only experienced only a slight G2/M block at 18 h post-treatment ( and ). Interestingly, the opposite effect was observed in cells exposed to 100 µM QuinED · ZnCl2; a large G2/M block was observed 18 h after cells were heated in the presence of the ligand for 1 h at 42.5°C, and recovery did not occur until 36 h after treatment, whereas no G2/M block was observed in the cells treated at 37°C for 1 h and then incubated at 37°C for 18 h post-treatment. Increases in the percentage of cells observed in the G2/M phases represented true cell cycle blocks rather than a loss of cells from other phases of the cell cycle through apoptosis and/or necrosis. This conclusion is based on the observation that the total number of drug and heat-exposed cells at time points at which cell cycle blocks were noted were not significantly different from the number of heated or unheated control cells that were or were not exposed to QuinED · ZnCl2. Also, G1 populations increased from 18 to 24 h in heat and drug-treated cell populations in which there was an observed G2/M block at 18 h, indicating that as cells were exiting G2/M at 18 h post-treatment, many, if not all, of the blocked cells were re-entering G1 phase.
Figure 4. Perturbation of the cell cycle in cells treated with enediynes and hyperthermia. Shown are representative DNA distributions accumulated for HeLa CCL-2 cells incubated with DMSO only or either PyED or QuinED · ZnCl2 for 1 h at 37°C or 42.5°C and fixed 16 h after incubation prior to staining with propidium iodide and analysis by flow cytometry.
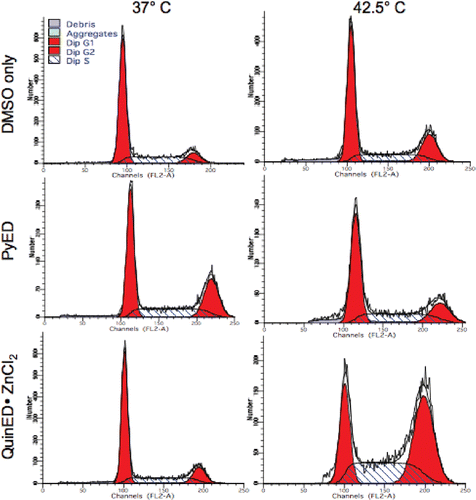
Figure 5. Alterations in cell cycle progression of cells treated with enediynes and hyperthermia. The fraction of cells in each phase of the cell cycle was plotted as a function of time after drug (PyED or QuinED · ZnCl2) and heat treatments. Y-error bars represent SEM (n = 2 for drug curves and n = 4 for DMSO only curves).
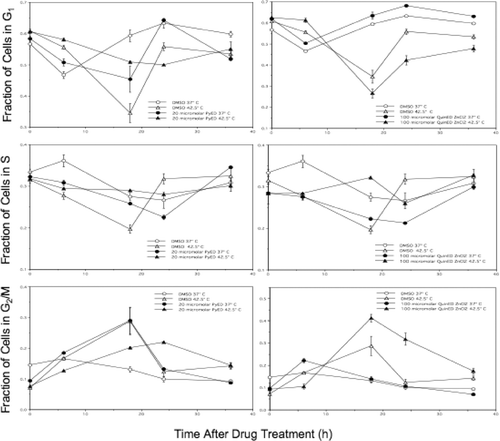
Discussion
The potential for using heat to enhance the effects of chemotherapeutic agents has not been fully realised, perhaps because the agents are often cytotoxic when administered systemically at normal body temperature Citation[21]. A drug that could be administered systemically with little or no toxicity to normal tissue, but which could be activated or have the cytotoxic effects amplified in a targeted manner, within a defined tissue volume (e.g. a tumour) would be clinically significant. Hyperthermia, administered locally to the targeted tumour volume (via microwaves, for example), could therefore serve to target drug action by increasing tumour cell killing without affecting systemic toxicity, increasing the therapeutic gain via a localised activation of compounds.
The toxicity of enediynes, which is largely attributed to their ease of thermodynamic activation, has greatly impeded the clinical use of this class of chemotherapeutic agents Citation[5]. Metallation of enediyne compounds appears to be one mechanism by which activation may be controlled Citation[10].
We have shown that 42.5°C hyperthermia sensitises HeLa cells to two different enediyne derivatives, QuinED · ZnCl2 and PyED; sensitisation was observed at concentrations which are non-toxic or only slightly cytotoxic at 37°C ( and ). Enhancement of QuinED · ZnCl2 cytotoxicity by hyperthermia was also observed in two other human tumour cell lines (human U-1 melanoma, PaCa-2 pancreatic); the extent of the enhanced effect was similar to HeLa cells when tested at concentrations up to 150 µM (one experiment, unpublished). Enediynes known to induce DNA damage via diradical intermediates have been shown to induce programmed cell death Citation[22]. The increase in cell death we observed when cells are heated during drug treatment appears to be due, at least in part, to an increase in apoptosis ( and ).
It is unclear exactly how heat sensitises the cells to metalloenediynes, and whether the mechanism of sensitisation is similar for both compounds. The increased toxicity could be due to an increase in plasma membrane permeability when administered during the heat treatment. It is also attractive to speculate that the differences in drug-induced cytotoxicity at the two temperatures may be due to differences in the rate of cyclisation, since an increase in Bergman cycloaromatisation could lead to increased DNA damage. Hyperthermia inhibits DNA repair, and heat-radiosensitisation is believed to be mediated by inhibition of repair of radiation-induced DSBs Citation[12–17]. Enediynes appear to induce DSBs like ionising radiation, and heating likely inhibits efficient repair of DSBs in drug-treated cells. However, it is worth noting that a preferential increase in DSBs in replicating DNA (compared with bulk DNA) was observed following either hyperthermia treatment alone or when heat was administered prior to irradiation; Wong et al. Citation[17] proposed that this preferential increase in DSBs in replicating DNA when heat was combined with radiation may explain at least in part, the high sensitivity of S-phase cells to heat-radiosensitisation. Since enediynes are potent inducers of DSBs Citation[2–4], the enhancement of cytotoxicity of cells exposed to the compounds during heating may be due in part to selective sensitisation of S-phase cells to the drug, mediated by an increase in drug-induced DSBs or an interaction between drug- and heat-induced DSBs in or near replicating regions.
Mammalian cells treated with clastogenic agents generally experience perturbations in cell cycle progression. Lidamycin (LDM), a radiomimetic enediyne that primarily induces DSBs rather than single strand breaks in tumour cells Citation[18], induces a G2/M arrest that has been correlated with the formation of chromosomal aberrations; many of the cells progress into G1 despite retaining chromosomal damage Citation[23]. The LDM-induced G2/M blockage may result from increases in phosphorylation levels of Chk1, Chk2, Cdc25C, and Cdc2 as well as Cdc2 and cyclin B1 expression Citation[24], Citation[25] or activated p38 MAPK Citation[25–28]. Thus, while some enediynes due not perturb cell cycle progression or alter the mode of death, several of them do elicit a G2/M arrest as well as increase apoptosis Citation[29], Citation[30].
Our finding that treatment with one enediyne (PyED after 37°C treatment) induces a G2/M arrest, but the other (QuinED · ZnCl2) does not, is not without precedent. However, our observation that heating during administration of PyED or QuinED · ZnCl2 may decrease or increase, respectively, the ability of the enediyne to induce G2/M arrest in HeLa cells is novel ( and ), and may shed light on the mechanisms by which enediynes induce cell killing, as well as how cytotoxicity might be modulated. Heating during PyED treatment resulted in a reduced delay in the G2/M arrest, potentially allowing cells with damaged DNA to divide before repair has occurred. Conversely, heating during treatment with QuinED · ZnCl2 (though equitoxic but with a 5-fold higher molar concentration of enediyne) resulted in a greater G2/M block, which presumably provided more time for repair of DNA damage induced by the compound.
While the prospect of using hyperthermia as an adjuvant and sensitiser to enediyne chemotherapy has not been explored, there is evidence which suggests that hyperthermia treatment may represent a plausible method to modify enediyne reactivity Citation[31], leading to enhanced clinical relevance. Esperamicin A1 is an enediyne whose activity is very sensitive to thiol reduction Citation[32]. However, activation may occur in the absence of thiols upon heating at 50°C; this results in a pattern of DNA cleavage that is different from that observed upon activation of esperamicin A1 with dithiothreitol at lower temperatures Citation[33]. Thus, direct rearrangement of the enediyne core by thermal vibration or activation of the aglycon moiety with some other non-sulphide group may facilitate unique, heat-induced DNA cleavage patterns Citation[33].
The data presented in this study were accumulated from experiments in which only a single cell line was tested using a single treatment regimen. Further experiments performed with other normal and tumour cell lines and in vivo tumour models, utilising additional clinically relevant drug and hyperthermia dosing regimens (e.g. lower drug concentrations administered for longer durations at lower hyperthermic temperatures), will be required to verify the observed effects and guide any future assessment of the potential clinical utility of combining metalloenediynes with hyperthermia. Since heat can be delivered in a specific and localised manner to a patient's tumour, minimally toxic drug concentrations of metalloenediynes that can be given systemically followed by area-specific heat treatments that sensitise tumour cells to drug delivery may provide a practical solution to many of the complications that prevent enediynes from entering the clinical setting. While the clinical usefulness of metalloenediynes, alone or combined with hyperthermia, can only be optimised if the mechanisms of action of these compounds are better understood, our studies with both PyED and QuinED · ZnCl2 enediynes suggest another therapeutic route to harness the tumouricidal potential of these chemotherapeutic agents while controlling many of the challenges that these agents normally present during treatment.
Acknowledgements
We wish to acknowledge support from the IU Simon Cancer Center Flow Cytometry Facility, at which the flow cytometric analyses were performed. We also wish to thank Joy Garrett and Mary Wampler for technical assistance.
Declaration of interest: This work was supported by NIH grant CA108582 (J.R.D.) and the gracious support of the Walther Cancer Foundation (J.M.Z.).
References
- Bergman RG. Reactive 1,4-dehydroaromatics. Acc Chem Research 1973; 6: 25–31
- Lockhart TP, Bergman RG. Evidence for reactive spine state of 1,4-Dehidrobenzenes. J Am Chem Soc 1981; 103: 4091–4096
- Lockhart TP, Comita PB, Bergman RG. Kinetic evidence for the formation of discrete 1,4-dehydrobenzene intermediates. Trapping by inter- and intramolecular hydrogen atom transfer and observation of high-temperature CIDNP. J Am Chem Soc 1981; 103: 4082–4090
- Gredičak M, Jerić I. Enediyne compounds – New promises in anticancer therapy. Acta Pharm 2007; 57: 133–150
- Bhattacharyya S, Zaleski JM. Metalloendiynes: Advances in the design of thermally and photochemically activated diradical formation for biomedical applications. Curr Topics Med Chem 2004; 4: 1637–1654
- Grissom JW, Gunawardena GU, Klinberg D, Huang G. The chemistry of enediynes, enyne, allens, and related compounds. Tetrahedron 1996; 52: 6453–6518
- Wenk HH, Winkler M, Sander W. One century of aryne chemistry. Angew Chem Int Ed 2003; 42: 502–528
- Jones GB, Wright JM, Hynd G, Wyatt JK, Yancisin M, Brown MA. Protein-degrading enediynes: Library-screening of Bergman cycloaromatization products. Org Lett 2000; 2: 1863–1866
- Purohit A, Wyatt J, Hynd G, Wright J, El-Shafey A, Swamy N, Ray R, Jones GB. Chemical synthesis of hormone receptor probes: High affinity photoactivated enediyne-estrogens. Tetrahedron Lett 2001; 42: 8579–8582
- Rawat DS, Zaleski JM. Geometric and electronic control of thermal Bergman cyclization. Synlett 2004; 3: 393–421
- Ben-Hur E, Elkind MM, Bronk BV. Thermally enhanced radioresponse of cultured Chinese hamster cells: Inhibition of repair of sublethal damage and enhancement of lethal damage. Radiat Res 1974; 58: 38–51
- Dewey WC, Sapareto SA, Betten DA. Hyperthermic radiosensitization of synchronous Chinese hamster cells: Relationship between lethality and chromosomal aberrations. Radiat Res 1978; 76: 48–59
- Corry PM, Robinson S, Getz S. Hyperthermic effects on DNA repair mechanisms. Radiology 1977; 123: 475–482
- Mills MD, Meyn RE. Effects of hyperthermia on DNA repair mechanisms. Radiat Res 1981; 87: 314–328
- Radford IR. Effects of hyperthermia on the repair of X-ray induced DNA double strand breaks in mouse L cells. Int J Radiat Biol 1983; 5: 551–557
- Dikomey E, Franzke J. Effect of heat on induction and repair of DNA strand breaks in X-irradiated CHO cells. Int J Radiat Biol 1992; 61: 221–233
- Wong RSL, Dynlacht J, Cedervall B, Dewey WC. Analysis by pulsed field gel electrophoresis of DNA double-strand breaks induced by heat and/or X-irradiation in bulk and replicating DNA of CHO cells. Int J Radiat Biol 1995; 68: 141–152
- Xu YJ, Zhen YS, Goldberg IH. C1027 chromophore, a potent new enediyne antitumor antibiotic, induces sequence-specific double-strand DNA cleavage. Biochemistry 1994; 33: 5947–5954
- Povirk LF. DNA damage and mutagenesis by radiomimetic DNA-cleaving agents: Bleomycin, neocarzinostatin and other enediynes. Mutat Res 1996; 355: 71–89
- Rawat DS, Zaleski JM. Mg2+-induced thermal enediyne cyclization at ambient temperature. J Am Chem Soc 2001; 123: 9675–9676
- Hall EJ, Giaccia AJ. Radiobiology for the Radiologist, 6th. Lippincott, Williams and Wilkins, Amsterdam 2006; 166: 546
- Nicolaou KC, Stabila P, Esmaeli-Azad B, Wrasidlo W, Hiatt A. Cell-specific regulation of apoptosis by designed enediynes. Proc Natl Acad Sci USA 1993; 8: 3142–3146
- McHugh MM, Gawron LS, Matsui S, Beerman TA. The antitumor enediyne C-1027 alters cell cycle progression and induces chromosomal aberrations and telomere dysfunction. Cancer Research 2005; 65: 5344–5351
- Abraham RT. Cell cycle check point signaling through the ATM and ATR kinases. Genes Dev 2001; 17: 2177–2196
- Liu X, Bian C, Ren K, Jin H, Li B, Shao R. Lidamycin induces marked G2 cell cycle arrest in human colon carcinoma HT-29 cell through activation of p38 MAPK pathway. Oncol Rep 2007; 17: 597–603
- Bulavin DV, Higashimoto Y, Popoff JJ, Gaarde WA, Basrur V, Potapova O, Appella E, Fornace AJ, Jr. Initiation of a G2/M checkpoint after ultraviolet radiation requires p38 kinase. Nature 2001; 411: 102–107
- Dmitrieva NI, Bulavin DV, Fornace AJ, Jr, Burg MB. Rapid activation of G2/M checkpoint after hypertonic stress in renal inner medullary epithelial (IME) cells is protective and requires p38 kinase. Proc Natl Acad Sci USA 2002; 99: 184–189
- Hsu YL, Kuo PL, Lin LT, Lin CC. Asiatic acid, a triterpene, induces apoptosis and cell cycle arrest through activation of extracellular-signal regulated kinase and p38 mitogen-activated protein kinase in human breast cancer cells. J Pharmacol Exp Ther 2005; 313: 333–344
- Wu ZZ, Chien CM, Yang SH, Lin YH, Hu XW, Lu YJ, Wu MJ, Lin SR. Induction of G2/M phase arrest and apoptosis by a novel enediyne derivative, THDA, in chronic myeloid leukemia (K562) cells. Mol Cell Biochem 2006; 292: 99–105
- Lu YJ, Yang SH, Chien CM, Lin YH, Hu XW, Wu ZZ, Wu MJ, Lin SR. Induction of G2/M phase arrest and apoptosis by a novel enediyne derivative, THDB, in chronic myeloid leukemia (HL-60) cells. Toxicol In Vitro 2007; 1: 90–98
- Nicolaou KC, Ogawa Y, Zuccarello G, Schweiger EJ, Kumazawa T. Cyclic conjugated enediynes related to calicheamicins and esperamicins: Calculations, synthesis, and properties. J Am Chem Soc 1988; 110: 4866–4868
- Long BH, Golik J, Forenza S, Ward B, Rehfuss R, Dabrowiak JC, Catino JJ, Musial ST, Brookshire KW, Doyle TW. Esperamicins, a class of potent antitumor antibiotics: Mechanism of action. Proc Natl Acad Sci USA 1989; 86: 2–6
- Uesawa Y, Sugiura Y. Heat-induced cleavage by esperamicin antitumor antibiotics. Biochemistry 1991; 30: 9242–9246