Abstract
Purpose: To identify specific Pluronic triblock copolymer structural properties which are critical to its function as a sensitiser in hyperthermia treatment of experimental colorectal adenocarcinoma.
Materials and methods: DHD/K12/TRb rat colorectal adenocarcinoma cells were exposed to Pluronics, a family of triblock copolymers with the general structure EOx-POy-EOx (EO: ethylene oxide, and PO: propylene oxide), at a range of molecular weights (Mw) and EO:PO:EO sub-unit lengths and then submitted to sublethal heat (43°C) treatment. Outcomes indicating Pluronic performance as a thermal sensitiser were correlated with its structural properties; lead candidates were determined accordingly. Finally, one of the lead candidates, Pluronic L61, a 2000 Da copolymer, was used to assess sensitising functionality in vivo in a subcutaneous rat model of colorectal carcinoma.
Results: Pluronics with 1100 ≤ Mw ≤ 3200 Da and hydrophilic lipophilic balance (HLB) between 1–8 demonstrated the highest thermosensitising ability. Pluronics L31, L61, L62, L10 and L64 were found to be among the most effective copolymers for hyperthermia sensitisation under tested conditions. Most encouraging, L61 in synergy with hyperthermia significantly reduced tumour growth progression in vivo compared to tumours treated with hyperthermia alone.
Conclusions: Pluronic copolymer structure properties including, Mw, HLB and PO length are essential to its hyperthermia sensitising function.
Introduction
Pluronics (also known by the generic name poloxamer), a family of triblock copolymers of hydrophilic ethylene oxide (EO) and hydrophobic propylene oxide (PO) arranged in the general structure EOx-POy-EOx (where x and y indicates the number of subunits), have been an integral part of many recent therapeutic formulations for treatment of cancer. Among these applications, Husseini et al. used Pluronic 105 micelles as anticancer drug carriers and applied ultrasound to trigger the release of the drug from these micelles [Citation1–3]. Katz et al. used Pluronic F68 to suspend 1-Docosanol for treating various types of viruses Citation[4]. In addition, Pluronics such as L61, L81 and F127 have been used as particle size modulators and stabilising agents Citation[5], Citation[6]. Furthermore, Pluronic P85 and P105 have been used as sensitising agents for doxorubicin (Dox) for treating multidrug resistant (MDR) cancer Citation[7] and others [Citation8–16]. It has been shown that certain Pluronics selectively sensitise MDR cancer cells to chemotherapy through mechanisms of inhibiting Pgp efflux, changing membrane fluidity, depleting intracellular ATP and inhibiting mitochondrion respiration Citation[7], Citation[10], Citation[13]. While a chemosensitiser is a compound that inhibits one or more functions of cells making them more susceptible to chemotherapy, a thermosensitiser is any agent that can facilitate hyperthermia-induced cell death. An ideal thermosensitiser should exhibit a synergistic effect, showing little inherent activity and increasing the effectiveness of hyperthermic stress in the affected cell.
Prior work in our group has explored the use of Pluronic as a thermosensitiser with the eventual goal of enhancing hyperthermia induced by radiofrequency (RF) ablation of solid tumours Citation[17]. RF ablation is a viable alternative to surgical resection for patients who either have a tumour mass that is surgically inaccessible or cannot tolerate surgery due to confounding morbidities Citation[18]. To apply this technique, the physician inserts a needle electrode percutaneously into the tumour using computed tomography, ultrasound or magnetic resonance imaging guidance. The electrode tip passes high-frequency current upon completing the circuit through a grounding pad to the surrounding tissue. The electric current leads to ionic agitation in the tissue and consequently frictional heat which, in the end, induces cell destruction and coagulation necrosis Citation[19]. Despite integration of RF ablation into routine clinical practice, tumour recurrence at the tumour–normal tissue boundary remains a problem and continues to be a common cause of treatment failure Citation[20]. Recent reports on hepatocellular carcinoma showed that up to 12% of patients had local tumour recurrence after RF ablation. While factors leading to tumour recurrence are multifaceted, one of the primary reasons for tumour recurrence following ablation is insufficient heat delivery to the tumour either due to large tumour size, particularly when tumour sizes are greater than 3 cm in diameter Citation[21], Citation[22], irregular shape or the presence of heat-sinking blood vessels (flowing blood carries heat energy away from tumour tissue, preventing local temperature elevation) at the periphery of the tumour. Multiple strategies have been explored to reduce the post-RF ablation tumour recurrence rate. These include instrumentation modifications to increase volume of ablation Citation[23], Citation[24], physiological manipulations to occlude arteries and prevent heat sink, and pharmacological modifications typically serving as adjuvant chemotherapy to increase cell death Citation[25]. Our unique strategy uses Pluronic as a thermosensitiser which on its own has little effect but when coupled with sublethal temperatures as low as 43°C acts in synergy with the hyperthermia to lead to improved cell death. Our results have previously shown that both Pluronic P85 and L61 are effective in sensitising DHD/K12/TRb rat colorectal cancer cells to low grade (43°C) hyperthermia in vitro Citation[17], Citation[26]. Furthermore, P85 was also effective for enhancing RF ablation treatment of tumours formed by these cells in vivo Citation[17]. It has previously been reported that the chemosensitising effects of Pluronics are dependent on the hydrophilic lipophilic balance (HLB) (Equation 1) and the number of polypropylene oxide (PO) units, with HLB <20 and intermediate (between 30–60) PO units being the most effective in inhibiting Pgp efflux Citation[11].where Mhydrophilic and Mhydrophobic are the molecular masses of the hydrophilic and hydrophobic portions of the molecule. The goal of the current study was to determine how Pluronic structure affects its ability to sensitise cancer cells to hyperthermia Citation[27].
Materials and methods
Materials
Pluronic P85 (EO26-PO40-EO26) and L61 (EO2-PO30-EO2) were generously donated by BASF Corporation (Florham Park, NJ). All other Pluronic copolymers were purchased from Sigma Aldrich (Milwaukee, WI). summarises the properties of all Pluronic polymers used in these studies. Trypsin-EDTA, Dulbecco's phosphate buffered saline (DPBS, without calcium or magnesium), RPMI medium 1640 (with L-glutamine and with phenol red), and penicillin/streptomycin were purchased from Gibco (Grand Island, NY). Fetal bovine serum (FBS) was purchased from Hyclone (Logan, UT). DispoDialyzer system (Spectra/Por®) and sterile 0.22 µm syringe driven filter units (Millex™-GP) and opaque-walled 96-well cell culture plates were purchased from Fisher Scientific (Pittsburgh, PA). The Caspase-Glo® 3/7 assays were purchased from Promega (Madison, WI).
Table I. Pluronic information.
Colorectal carcinoma model
The DHD/K12/TRb, rat colorectal adenocarcinoma was used in these studies Citation[28]. This metastatic cell line originated from a 1, 2-dimethylhydrazine-induced colon adenocarcinoma in BDIX rats. This tumour model possesses well defined properties essential for study of metastasis of colon carcinomas, such as a reproducible pattern of in vivo behaviour and the ability to be propagated both in vitro and in vivo. The cells were maintained in complete RPMI medium, containing 10% FBS and 1% (v/v) penicillin/streptomycin, in a 37°C humidified incubator with 5% CO2. For tumour propagation, all cells were from the second passage.
Cell treatments
Cells (105/mL) were seeded in 96- or 24-well plates overnight to allow adhesion. Prior to treatment, cell medium was removed and treatment solutions of Pluronics dissolved in RPMI were added to the corresponding wells (50 and 300 µL in 96- and 24-well plates, respectively). Cells were exposed to the treatment solutions for 0–20 min at 37°C and those in the hyperthermia group were further incubated at 43°C in a circulating water bath for 20 min. This temperature was selected to mimic the minimum sublethal hyperthermia received by tumour tissue at the periphery of the ablated volume. After treatment, the test medium was removed; cells were washed twice and replenished with 100 µL of completed RPMI. Plates were returned to the incubator. WST-1 and caspase assays (described below) were carried out at 2 and 24 h after treatment. At each of the assay times, additional plates of cells were trypsinised and viable cells counted with a haemocytometer.
WST-1 assay
Dehydrogenase activity was measured with the WST-1(WST: water soluble tetrazolium, or 4-[3-(4-iodophenyl)-2-(4-nitrophenyl)-2H-5-tetrazolio]-1,3-benzene disulphonate) assay. This assay works based on the principle that mitochondrial succinate dehydrogenase, which becomes inactive in non-viable cells, catalyses the conversion of a soluble form of tetrazolium, a salt, to a coloured dye formazan changing absorbance. Theoretically, the quantified dehydrogenase activity is directly proportional to the number of live cells. The WST-1 assay was performed 24 h after treatment following manufacturer directions. Briefly, supernatant was aspirated while reagents were diluted with completed RPMI medium. 100 µL of the diluted reagents was added to each well, plates were kept for 1 h at room temperature, and the optical density was determined at λ = 450 nm using a microplate reader (ELx808, Bio-Tek, Winooski, VT).
Caspase Glo® 3/7 assay
Apoptosis was determined with the Promega Caspase Glo® 3/7 assay. This assay works on the principle that caspase 3 or 7, which is activated in cells undergoing apoptosis, cleaves the added luminogenic substrate releasing aminoluciferin. This luciferin molecule subsequently reacts with luciferase resulting in the production of light. Briefly, the assay reagent was prepared, and the cells in 96-well plates were removed from the incubator. Once the reagent and the plates were equilibrated to room temperature, 100 µL of the reagent was added to each of the wells after thorough mixing by vortex. Subsequently, the contents in each well were gently mixed with a plate shaker at 300 rpm for 30 s. The plate was kept at room temperature, and the luminescence was detected 1 h later with a plate reader (TECAN, Durham, NC).
Structure–function correlation
For identifying the relationship between Pluronic structure and its thermosensitising function, one concentration (2.5 mg/mL) and exposure time (20 min) were selected to simplify the experimental design. Thirteen Pluronics, L31, L35, L44, L61, L62, L81, L64, L10, L92, L121, P85, P123 and F87 were tested. Based on these data, the enzyme activities of cells treated with the copolymers were plotted against their molecular weight (Mw).
In vivo tumour treatment efficacy studies with Pluronic L61
To demonstrate the utility of the thermosensitising strategy in vivo, L61 was selected from our in vitro results as one of the most promising candidates. To test its efficacy in enhancing hyperthermia tumours were treated with systemic L61 in combination with RF ablation. All procedures involving animals were carried out under a protocol approved by the Institutional Animal Care and Use Committee (IACUC) at Case Western Reserve University. Adult male BDIX rats, bred in-house (originally obtained from Charles River Laboratories), between 5–8 weeks old received one subcutaneous tumour inoculation on each of the upper hind legs. On the day of tumour inoculation, cells were harvested by trypsin-EDTA, washed in RPMI, centrifuged at 800 rpm for 5 min and resuspended at a final concentration of 2 × 106 cells/mL. Cells were then loaded into individual 1-mL syringes equipped with 27-gauge needles, and a separate syringe was used for each injection site. For tumour inoculation, the rats were anaesthetised with 1% isoflurane with an O2 flow rate of 1 L/min (EZ150 Isoflurane Vaporizer, EZ Anesthesia™). Cell suspension (50 mL) was injected subcutaneously at each site.
At an initial tumour volume of 2502 ± 302 mm3, rats were randomly divided into two groups. One group received 0.1 mg/kg of L61 in saline intravenously followed by RF ablation 4 h later. This pretreatment time of Pluronic L61 was based on results of our pilot study and published Pluronic in vivo half-life Citation[29]. The other group of rats received intravenous saline followed with RF ablation. The RF ablation protocol was as follows: ablation probe: 17-gauge,1-cm active single tip Radionics RF probe; temperature: 90°C; ablation duration: 3 min. Rat weight and tumour diameters (measured with calipers) were recorded weekly. The tumour volumes were estimated by:where at is the longest tumour diameter and bt is the perpendicular diameter Citation[30].
Statistical analysis
Two-tailed unpaired Student's t-test with unequal variances were used for in vitro analysis. The P values were determined with Microsoft Excel. Statistical significance was defined when P ≤ 0.05. Unless otherwise noted, all data are presented as mean ± standard deviation (SD).
For in vivo data, the mean tumour volume changes were compared with a paired Student's t-test. In addition, Kaplan-Meier curves were used to estimate the proportion of tumours that had not achieved a 100% reduction from baseline volume at weeks 1 to 4. Because tumours from the same animal cannot be assumed to be independent, a Cox proportional hazards regression model which takes the clustering into account was used to compare whether significant difference occurs between the curves [Citation31–33]. This analysis was also repeated using a threshold of 50% reduction from the baseline.
Results
Enzyme activity after Pluronic exposure
Pluronic polymers L31, L61, L62, L64 and L10 in synergy with low-grade hyperthermia were able to reduce cellular enzyme activity to 25.8 ± 8.5%.0 ± 1.8%, 0, 0.2 ± 0.2% and 0.6 ± 0.3% of the untreated control, respectively. In contrast, cells treated with Pluronic alone showed a modest change to 93.1 ± 21.1%, 66.9 ± 21.9%, 72.8 ± 19.5%, 89.5 ± 29.3% and 87.2 ± 30.2% of the untreated control. Pluronics L81, L92 and L121 appeared to be toxic to cells at the studied dose; administered alone they reduced enzyme activity to 10.8 ± 9.9, 6.5 ± 3.1 and 32.1 ± 7.5% of the control, respectively ().
Figure 1. Pluronic thermosensitising effects on DHD/K12/TRb cells in vitro after 20 min of Pluronic and hyperthermia (at 43°C) co-exposure. Pluronic concentrations are 2.5 mg/mL. Data presented as Mean ± SD (standard deviation, n = 3). *Significant difference compared to Pluronic only; †significant difference compared to untreated control (P < 0.05).
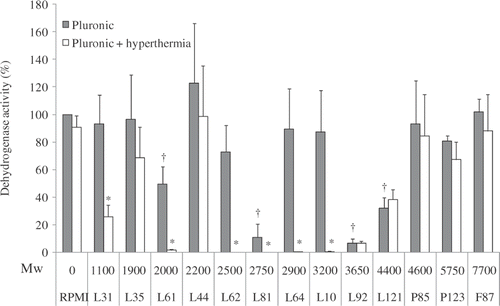
The investigated Pluronics were divided into two groups based on their HLB. The hydrophobic polymers were defined as those with HLB between 1 and 7, and the hydrophilic polymers were defined as those with HLB above 7. Then, the cellular enzyme activities acquired with WST-1 assay from these two groups of polymers were plotted against Pluronic structural properties including NPO/NEO ratio (NPO: number of propylene oxide repeating units; NEO: number of ethylene oxide repeating units), Mw, HLB and NPO. For the hydrophilic polymers, the data did not exhibit any trends (). Similarly, when cells were treated with hydrophobic Pluronics alone, no specific trends were detectable from these data. In contrast, cellular enzyme activity of cells in the hydrophobic polymer + hyperthermia treated group followed a second order polynomial function when plotted against their molecular weight () with R2 = 0.98. However, no detectable trends were observed when the enzyme activity was plotted against NPO/NEO, HLB or NPO. Further studies are necessary to explain these observations. In summary, Pluronics with 1100 ≤ Mw ≤ 3200 Da and hydrophilic lipophilic balance (HLB) between 1 to 8 were the most effective. Out of these, two Pluronics (L81, L92) lead to a drastic reduction of enzyme activity but were inherently toxic, and only one (L64) was above an HLB of 4 in this Mw range. Based on this data, we concluded that hydrophobic copolymers L31, L61, L62 and hydrophilic copolymers L64 and L10 are the most effective thermosensitisers for DHD/K12/TRb cells under the test conditions.
Cell apoptosis in response to Pluronic L61
Pluronic L61 was selected among the most effective polymers for further testing due to the availability of its pharmacokinetic information and its active role (in the formulation SP1049C) in a currently ongoing Phase III clinical trial for treating late stage upper gastrointestinal cancer Citation[13], Citation[34]. Apoptosis was determined with a caspase 3/7 assay. According to time-dependent characterisation, caspase activity was detectable 2 h after treatment. The caspase activity was normalised by the number of viable cells per well (). Results indicated that at 2 h, no significant difference in caspase activity was detected between L61 (0.03 ± 0.0) treated and the untreated control (0.05 ± 0.01) cells (P = 0.1), while L61 in synergy with hyperthermia (0.1 ± 0.0) led to an insignificant increase of caspase activity compared to L61 treated cells (P = 0.07) or cells treated with hyperthermia only (0.05 ± 0.0, P = 0.016). Likewise, 24 h after treatment, L61 in synergy with hyperthermia caused drastic increase in caspase activity (0.4 ± 0.1; P = 0.005) compared to cells exposed to hyperthermia only (0.1 ± 0.0). These data suggest that L61 in synergy with low-grade hyperthermia can lead to continuous apoptosis of the cells while neither treatment alone caused lethal harm to the cells.
In vivo efficacy with copolymer L61
Pluronic L61 was used in conjunction with RF ablation in treatment of colorectal carcinoma in rats. During week 1–2, no significant difference was seen in tumour progression between the RF ablation only group and the combination treatment. However, beginning at week 3 and at the conclusion of the 4-week monitoring period, the relative volume of tumours treated with a combination of L61 pretreatment and RF ablation was significantly reduced (−60.5 ± 55.5%; P = 0.02) compared to those treated with RF ablation alone (51.1 ± 155.6%). More noteworthy, by physical palpation, 6/17 (35%) tumours treated with L61 + RF ablation were undetectable and more than half, 9/17 (53%), of these tumours reduced in volume by more than 80%. In contrast, 3/17 (18%) of the tumours from RF ablation alone treated groups were not detectable by physical palpation and reduced in volume by more than 80% (). Kaplan–Meier curves were used to estimate the proportion of tumours that had not achieved a 100% reduction from baseline volume at weeks 1–4 Citation[33]. Results from the Cox proportional hazards regression model showed that significant difference occurred between the two treatment groups with L61 + RF ablation being more efficacious than RF ablation (P = 0.03, ). This analysis was repeated using a threshold of 50% reduction from baseline, and results did not differ (P = 0.03).
Figure 4. Change in tumour volume relative to size before treatment in response to radiofrequency ablation with or without Pluronic L61. Data presented as Mean ± SD (standard deviation, n = 17). Portions of the error bars were omitted for presentation clarity. *Indicates statistically significant difference (P < 0.05).
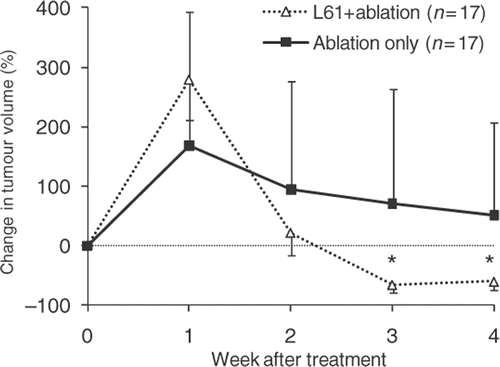
Discussion
The goal of this work was to examine how structural properties of Pluronic influence its performance as a thermosensitising agent in hyperthermia treatment of a model rat colorectal adenocarcinoma cell line. Data from 13 Pluronics were acquired. Our results showed that Pluronic L31, L61, L62, L64 and L10 were the most effective thermosensitisers, while copolymers L81, L92 and L121 showed inherent toxicity to cells. Subsequently, the cellular enzyme activity was correlated to NPO – the number of hydrophobic units and the polymer molecular weight (Mw). It appears that copolymers with lower molecular weight between 1100 to 3200 Da and a HLB <8 are more likely to have effective thermosensitising ability for DHD/K12/TRb cells under the test conditions. Pluronics that were effective thermosensitisers shared one particular structural characteristic − the NPO of these polymers were even multiples of the length of 16 C-C (carbon-carbon) bond length; and it is the typical length of mammalian lipid fatty acid tail of a cell membrane which is commonly 16 or 18 carbon atoms length Citation[35]. This may suggest a geometrical compatibility requirement for the proper interaction between copolymers and the cell membranes. Studies have shown a correlation between cell membrane fluidity change upon hyperthermia treatment, suggesting that the more sensitive a cell type is to hyperthermia, the more fluid the membrane becomes upon hyperthermia treatment. Conversely, a cell type that was more resistant to hyperthermia treatment will show less of a fluidity increase upon hyperthermia treatment Citation[36]. A geometrically compatible copolymer may be able to change the confirmation of cell membrane proteins which has been indicated as a potential pathway for sensitising thermo-tolerant cells Citation[37]. However, more studies are needed in order to draw specific conclusions.
Interestingly, our results mostly agreed with the four group-grid model developed by Batrakova et al. () indicating that L10, L61, L62 and L64, the effective thermosensitisers in our studies, are the Group II polymers in the 4 group-grid model Citation[11]. This may imply a common pathway between Pluronic copolymer thermo- and chemosensitisation. Based on this 4 group-grid model created by Batrakova et al., Pluronic copolymers L81, L92 and P85 should be effective candidates for thermosensitisation. In our studies, L81 and L92 demonstrated inherent toxicity, while P85 did not show much hyperthermia enhancement. However, our prior work has shown that P85 was effective in sensitising DHD/K12/TRb cells to low-grade hyperthermia at longer exposure times Citation[17], Citation[26]. We speculate that the toxic effects of L81 and L92 are due to relatively high concentration tested in our studies; and the ineffectiveness of P85 in this work is attributable to its low concentration and shorter exposure times compared to the prior data Citation[17]. Further experiments are required to confirm this assumption. Another anomaly between our model and the 4 group-grid model was observed. According to our model, L31, a group IIIa polymer, is an effective thermosensitiser, and L35 and L44 (also group IIIa polymers) are intermediate in effectiveness. However, according to Batrakova et al., all of these polymers have little effect in modulating P-glycoprotein. To fully adapt this model to our results, it may be appropriate to modify the 4 group-grid model by expanding group II polymer population to include the group IIIa polymers.
Figure 6. The four-group Pluronic copolymer grid based on Pluronic HLB and NPO adapted from a previously published model Citation[11]. Here the authors categorised the investigated Pluronic copolymers into four groups according to the HLB and NPO numbers. According to their results, Group II polymers were the most effective in modulating P-glycoprotein, while polymers from Group I and III showed little effect.
![Figure 6. The four-group Pluronic copolymer grid based on Pluronic HLB and NPO adapted from a previously published model Citation[11]. Here the authors categorised the investigated Pluronic copolymers into four groups according to the HLB and NPO numbers. According to their results, Group II polymers were the most effective in modulating P-glycoprotein, while polymers from Group I and III showed little effect.](/cms/asset/f995327b-4ded-4078-86db-d6cdac742351/ihyt_a_599828_f0006_b.gif)
As demonstrated in prior studies, structural properties of Pluronic appear to influence its in vitro and in vivo behaviour Citation[29], Citation[38]. For example Kabanov et al. demonstrated that the tissue distribution of Pluronic is dependent on the length of the hydrophobic block. Specifically, the longer the hydrophobic PO block and the shorter the hydrophilic EO blocks, the higher the tissue distribution coefficient (organ to plasma ratio) and the higher the organ retention. Also in this study, results show that L61 had a higher liver (4.93) distribution coefficient compared to that of P85 which is 3.38 Citation[38]. This information suggests that L61 may be overall more relevant for treating colorectal metastasis to the liver – a common target of RF ablation treatment. Because of this fact, and its readily available pharmacokinetic information and its active role in an ongoing clinical trial for chemosensitisation, L61,which was among the most effective copolymers from our in vitro studies, was selected for testing its efficacy in enhancing RF ablation Citation[13], Citation[34].
Our in vivo data ( and ) show that tumours treated with a combination of L61 pretreatment and RF ablation exhibited a significant reduction in size compared to RF ablation only at 3 and 4 weeks after treatment. Also of note is that, 1 week after treatment, tumour volumes increased due to inflammatory effects. Interestingly, dissimilar to the tumour volumes treated with P85 pretreatment in combination with RF ablation Citation[17], the L61 pretreatment in combination with RF ablation did not reduce the inflammatory response. To discern the cause of this discrepancy, further investigation is needed.
Conclusion
In this work we aimed to determine the ultimate Pluronic copolymer structure for enhancing hyperthermia cancer treatment. Our results indicate that a delicate balance between molecular weight, HLB and number of propylene oxide blocks is critically important to this function. Pluronics with lower molecular weight between 1100 to 3200 Da and a HLB <8 demonstrated the highest thermosensitising ability with Pluronics L31, L61, L62, L10 and L64 among the most effective copolymers for hyperthermia sensitisation under tested conditions. Most encouraging, L61 in synergy with hyperthermia significantly reduced tumour growth progression in vivo compared to tumours treated with hyperthermia alone. These results further imply the positive role Pluronic may play in hyperthermia cancer treatment.
Acknowledgments
The authors thank Mark D. Schluchter for his help with the Kaplan-Meier and Cox regression model analysis. The authors thank Bryan Traughber, Angela Carlson and Sarah Gleeson for their assistance in manuscript preparation.
Declaration of interest: This study was supported by National Institutes of Health grants (R01CA118399 and R01CA136857 to Agata Exner). The authors alone are responsible for the content and writing of the paper.
References
- Marin A, Sun H, Husseini GA, Pitt WG, Christensen DA, Rapoport NY. Drug delivery in Pluronic micelles: Effect of high-frequency ultrasound on drug release from micelles and intracellular uptake. J Control Release 2002; 84: 39–47
- Husseini GA, Runyan CM, Pitt WG. Investigating the mechanism of acoustically activated uptake of drugs from Pluronic micelles. BMC Cancer 2002; 2(20) doi: 10.1186/1471-2407-2-20
- Husseini GA, Christensen DA, Rapoport NY, Pitt WG. Ultrasonic release of doxorubicin from Pluronic P105 micelles stabilized with an interpenetrating network of N,N-diethylacrylamide. J Control Release 2002; 83: 303–305
- Katz DH, Marcelletti JF, Khalil MH, Pope LE, Katz LR. Antiviral activity of 1-docosanol, an inhibitor of lipid-enveloped viruses including herpes simplex. Proc Natl Acad Sci USA 1991; 88: 10825–10829
- Krupka TM, Solorio L, Wilson RE, Wu H, Azar N, Exner AA. Formulation and characterization of echogenic lipid-Pluronic nanobubbles. Mol Pharm 2010; 7: 49–59
- Lai JI, Shafi KV, Ulman A, Loos K, Lee Y, Vogt T, Lee W, Ong NP. Controlling the size of magnetic nanoparticles using Pluronic block copolymer surfactants. J Phys Chem B 2005; 109: 15–18
- Alakhova DY, Rapoport NY, Batrakova EV, Timoshin AA, Li S, Nicholls D, Alakhov VY, Kabanov AV. Differential metabolic responses to Pluronic in MDR and non-MDR cells: A novel pathway for chemosensitization of drug resistant cancers. J Control Release 2010; 142: 89–100
- Exner AA, Krupka TM, Scherrer K, Teets JM. Enhancement of carboplatin toxicity by Pluronic block copolymers. J Control Release 2005; 106: 188–197
- Batrakova EV, Li S, Alakhov VY, Elmquist WF, Miller DW, Kabanov AV. Sensitization of cells overexpressing multidrug-resistant proteins by Pluronic P85. Pharm Res 2003; 20: 1581–1590
- Kabanov AV, Batrakova EV, Alakhov VY. An essential relationship between ATP depletion and chemosensitizing activity of Pluronic block copolymers. J Control Release 2003; 91: 75–83
- Batrakova EV, Li S, Alakhov VY, Miller DW, Kabanov AV. Optimal structure requirements for Pluronic block copolymers in modifying P-glycoprotein drug efflux transporter activity in bovine brain microvessel endothelial cells. J Pharmacol Exp Ther 2003; 304: 845–854
- Kabanov AV, Batrakova EV, Alakhov VY. Pluronic block copolymers for overcoming drug resistance in cancer. Adv Drug Deliv Rev 2002; 54: 759–779
- Batrakova EV, Li S, Elmquist WF, Miller DW, Alakhov VY, Kabanov AV. Mechanism of sensitization of MDR cancer cells by Pluronic block copolymers: Selective energy depletion. Br J Cancer 2001; 85: 1987–1997
- Miller DW, Batrakova EV, Kabanov AV. Inhibition of multidrug resistance-associated protein (MRP) functional activity with Pluronic block copolymers. Pharm Res 1999; 16: 396–401
- Alakhov V, Moskaleva E, Batrakova EV, Kabanov AV. Hypersensitization of multidrug resistant human ovarian carcinoma cells by Pluronic P85 block copolymer. Bioconjug Chem 1996; 7: 209–216
- Krupka TM, Weinberg BD, Wu H, Ziats NP, Exner AA. Effect of intratumoral injection of carboplatin combined with Pluronic P85 or L61 on experimental colorectal carcinoma in rats. Exp Biol Med (Maywood) 2007; 232: 950–957
- Weinberg BD, Krupka TM, Haaga JR, Exner AA. Combination of sensitizing pretreatment and radiofrequency tumor ablation: Evaluation in rat model. Radiology 2008; 246: 796–803
- Solbiati L, Livraghi T, Goldberg SN, Lerace T, Meloni F, Dellanoce M, Cova L, Halpern EF, Gazelle GS. Percutaneous radio-frequency ablation of hepatic metastases from colorectal cancer: Long-term results in 117 patients. Radiology 2001; 221: 159–166
- Goldberg SN, Gazelle GS. Radiofrequency tissue ablation: Physical principles and techniques for increasing coagulation necrosis. Hepatogastroenterology 2001; 48: 359–367
- Flanders VL, Gervais DA. Ablation of liver metastases: Current status. J Vasc Interv Radiol 2010; 21: S214–222
- Zytoon AA, Ishii H, Murakami K, El-kholy MR, Furuse J, El-Dorry A, El-Malah A. Recurrence-free survival after radiofrequency ablation of hepatocellular carcinoma. A registry report of the impact of risk factors on outcome. Jpn J Clin Oncol 2007; 37: 658–672
- McWilliams JP, Yamamoto S, Raman SS, Loh CT, Lee EW, Liu DM, Kee ST. Percutaneous ablation of hepatocellular carcinoma: Current status. J Vasc Interv Radiol 2010; 21: S204–213
- Bruners P, Lipka J, Gunther RW, Schmitz-Rode T, Mahnken AH. Bipolar radiofrequency ablation: Is the shape of the coagulation volume different in comparison to monopolar RF-ablation using variable active tip lengths?. Minim Invasive Ther Allied Technol 2008; 17: 267–274
- Hong K, Georgiades C. Radiofrequency ablation: Mechanism of action and devices. J Vasc Interv Radiol 2010; 21: S179–186
- Wu H, Exner AA, Krupka TM, Weinberg BD, Haaga JR. Vasomodulation of tumor blood flow: Effect on perfusion and thermal ablation size. Ann Biomed Eng 2009; 37: 552–564
- Krupka TM, Dremann D, Exner AA. Time and dose dependence of Pluronic bioactivity in hyperthermia-induced tumor cell death. Exp Biol Med (Maywood) 2009; 234: 95–104
- Griffin WC. Classification of surface-active agents by ‘HLB’. J Soc Cosmet Chem 1949; 1: 311–326
- Nelson JL, Roeder BL, Carmen JC, RoloH F, Pitt WG. Ultrasonically activated chemotherapeutic drug delivery in a rat model. Cancer Res 2002; 62: 7280–7283
- Batrakova EV, Li S, Li Y, Alakhov VY, Elmquist WF, Kabanov AV. Distribution kinetics of a micelle-forming block copolymer Pluronic P85. J Control Release 2004; 100: 389–397
- Euhus DM, Hudd C, LaRegina MC, Johnson FE. Tumor measurement in the nude mouse. J Surg Oncol 1986; 31: 229–234
- Wei LJLDY, Weissfeld L. Regression analysis of multivariate incomplete failure time data by modeling marginal distributions. J Am Stat Assoc 1989; 84: 1065–1073
- Lin DY. MULCOX: A computer program for the Cox regression analysis of multiple failure time variables. Comput Methods Programs Biomed 1990; 32: 125–135
- Bewick V, Cheek L, Ball J. Statistics review 12: Survival analysis. Crit Care 2004; 8: 389–394
- Danson S, Ferry D, Alakhov V, Margison J, Kerr D, Jowle D, Brampton M, Halbert G, Ranson M. Phase I dose escalation and pharmacokinetic study of Pluronic polymer-bound doxorubicin (SP1049C) in patients with advanced cancer. Br J Cancer 2004; 90: 2085–2091
- Koolman J, Roehm KH. Color Atlas of Biochemistry. Thieme, New York, Stuttgart 2005
- Dynlacht JR, Fox MH. The effect of 45°C hyperthermia on the membrane fluidity of cells of several lines. Radiat Res 1992; 130: 55–60
- Konings AW, Ruifrok AC. Role of membrane lipids and membrane fluidity in thermosensitivity and thermotolerance of mammalian cells. Radiat Res 1985; 102: 86–98
- Kabanov AV. Micelles of amphilphilic block copolymers as vehicles for drug delivery. Elsevier, Masterdam 2000; 347–376
- Batrakova E, Lee S, Li S, Venne A, Alakhov V, Kabanov A. Fundamental relationships between the composition of Pluronic block copolymers and their hypersensitization effect in MDR cancer cells. Pharm Res 1999; 16: 1373–1379