Abstract
Purpose: Infrared heat, a transient receptor potential vanilloid type-3 (TRPV3) sensitive stimulus, may have potential physiological effects beneficial to treating metabolic syndrome.
Materials and methods: Obesity prone (OP) and obesity resistant (OR) rats were fed for seven days on a high-fat diet. Heat treated OP rats were exposed twice daily to infrared light for 20 min each, separated by 80 min of rest. Food intake, blood pressure, blood glucose, and body weight measurements were taken daily and compared between treated OP rats, untreated OP rats, and OR controls. The animals were perfused with 4% paraformaldehyde, and immunohistochemistry was performed on the coronal brainstem sections with polyclonal antibodies against TRPV3 and pro-opiomelanocortin (POMC). The positive-staining cells in the medulla nuclei were quantified using a microscope with reticule grid.
Results: Food intake, body weight, and mean arterial blood pressure (MAP) were higher in OP rats, a diet-induced metabolic syndrome model, accompanied by a reduced expression of POMC, an anorectic agent, in the hypoglossal nucleus (HN) and medial nucleus tractus solitarius (mNTS). Food intake in heat-treated OP rats was significantly decreased. POMC positive neuron count was increased in the HN and mNTS of OP rats following treatment. TRPV3 positive staining neurons were increased in the HN and mNTS of OP control rats and decreased following the heat treatments.
Conclusion: Lowered POMC and heightened TRPV3 expressions in the HN and mNTS are involved in development of hyperphagia and obesity in OP rats. Exposure to infrared heat modifies TRPV3 and POMC expression in the brainstem, reducing food intake.
Introduction
Metabolic syndrome, consisting of multiple risk factors for cardiovascular disease, results from the increasing prevalence of obesity and is a major health problem in modern times Citation[1], Citation[2]. Hyperphagia, hypertension, obesity, and insulin resistance define metabolic syndrome, and have been developed in obesity prone (OP) rats [Citation3–5]. Recent studies have demonstrated that whole body heating using far infrared rays improved insulin sensitivity by decreasing obesity-induced insulin resistance in diabetic mice Citation[6]. However, the therapeutic effects and signal transduction of heat treatment is unknown. The transient receptor potential vanilloid type-3 (TRPV3) is a vanilloid receptor primarily responsible for thermal perception [Citation7–9]. TRPV3 is activated by increasing temperature from 33°C to 40°C and has been shown to be expressed in skin, tongue, dorsal root ganglion, trigeminal ganglion, spinal cord and brain in humans [Citation10–13]. TRPV3 shares 40% homology with the transient receptor potential vanilloid type-1 (TRPV1) Citation[14], an ion channel that plays an important role in the detection of noxious physical, chemical, and temperature-related stimuli Citation[14], Citation[15]. TRPV1 is responsible for the heat and pain sensations derived from the chemical capsaicin Citation[16], and may play a role in feeding behaviour and body weight signalling: administration of capsaicin and other agonists can reduce food intake and increase energy expenditure, possibly through TRPV1 receptor desensitisation [Citation17–19]. Recent studies also show that TRPV1 null mice are protected from diet-induced obesity Citation[17].
Pro-opiomelanocortin (POMC), a precursor of hormones influencing feeding behavior such as ?-melanocyte stimulating hormone (α-MSH), plays a central role in homeostatic regulation of body weight Citation[20], Citation[21]. It is known to be expressed in the arcuate nucleus of the hypothalamus [Citation22–24] and the solitary tract in the brainstem Citation[25]. Activation of POMC in the medial nucleus tractus solitarius (mNTS) has been shown to result in a sustained reduction of food intake and improved insulin sensitivity Citation[19], Citation[20], Citation[25]. The mNTS is the principal sensory nucleus entering the CNS and plays an important role in sensory, cardiovascular, and feeding regulation Citation[23], Citation[25–28]]. The hypoglossal nucleus (HN) is the centre of motor afferent control for lower jaw and tongue movements Citation[29], and is involved in taste and gustatory responses in the medulla Citation[30], Citation[31]. Ingestion and anticipation of a meal also created increased Fos expression in the HN Citation[32].
The purpose of this study is to determine the effects of full body thermal stimulation by far-infrared rays on food intake, body weight, arterial blood pressure, and blood glucose levels in obesity prone rats on a high fat diet. TRPV3 and POMC expressions in the brainstem nuclei were examined by immunohistochemistry in control OP, heat-treated OP, and control OR rats after eight days of in vivo experimentation. The influence of infrared heat on expression of POMC and TRPV3 in the brainstem, particularly in the mNTS and HN, was compared between groups and matched with its corresponding physiological data.
Materials and methods
Animal preparation
All experiments were performed on 7-week-old OP-CD (obesity prone) rats and their age-matched control strain, OR-CD (obesity resistant), acquired from Charles River (Wilmington, MA). This outbred rat strain was previously called DIO, or diet-induced obese rats [Citation3–5]. The rats were fed a high fat diet (Purina Formulab Diet 5008) ad libitum. The OP strain rapidly gained weight and developed metabolic syndrome, while the OR strain would develop other facets (hypertension, hyperglycaemia) of the syndrome without becoming overweight. The protocol was approved by the Harbor-UCLA Animal Care and Use Review Committee, and was in accord with Association for Assessment and Accreditation of Laboratory Animal Care (AAALAC) and National Institutes of Health (NIH) guidelines. The animals were maintained on a 12-hour light–dark cycle in temperature and humidity controlled rooms.
Arterial blood pressure was monitored with a tail-cuff using Model 179 Blood Pressure Analyzer (IITC Life Science, Woodland Hills, CA) and measured in conscious rats daily. Food pellets were weighed and body weight was recorded each day for 8 days with and without treatment. Concentration of blood glucose was measured with a Glucometer Elite (Bayer, Elkhart, IN) on the first and last days after the treatments. Blood was obtained by performing a small incision at the tail end.
Heat treatment
Infrared heating was done by a far-infrared emitting lamp (Prime Herbs, San Jose, CA), as shown in . Temperature was monitored with a digital temperature controller (Harvard Apparatus, Holliston, MA), and kept between 36–40°C (). Heat was applied for 20 min, after which the rats were allowed to rest for 80 min, and then exposed for another 20 min. This treatment was performed each day for 6 days. Food intake and body weight were recorded daily post treatment, starting 2 days before treatment. Arterial blood pressure was recorded in conscious rats every day, beginning 2 days before heating treatment. Systolic, diastolic, and mean arterial blood pressure (MAP) were obtained in conscious rats daily before and 10 min after each treatment. No animals showed any adverse signs or reactions to the treatments.
Figure 1. Photograph illustrating the experimental set-up used in infrared heat application. The infrared heating device, TDP CQ-27 (Lhasa OMS, Weymouth, MA), was utilised as a heat source. The infrared heat lamp is placed above an open-filtered mouse cage, and temperature is maintained by adjusting the proximity of the lamp to the chamber. Temperature is monitored and kept between 36–40°C by a digital temperature controller (Harvard Bioscience, Holliston, MA), with the sensor placed inside the heating chamber, as labelled.
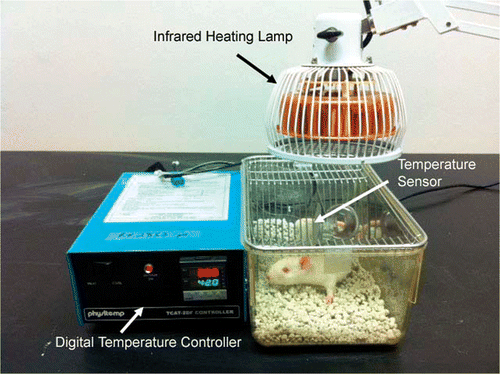
Histological method
After one week of study the animals were sacrificed by transcardial perfusion. A catheter was inserted into the left ventricle, and the right atrium was cut. The animal was perfused with 100–150 mL of 0.9% NaCl, then 4% paraformaldehyde in sodium phosphate buffer for 45 min Citation[33], Citation[34]. The brain was rapidly removed and post-fixed with 4% paraformaldehyde in 0.1 M phosphate buffer at 4°C overnight, and then placed in 30% sucrose for 24 h. The lower brainstem was frozen sectioned coronally at a thickness of 30 µm with a microtome knife (−18°C).
According to the topography of the important nuclei located along the rostrocaudal axis Citation[33], Citation[34], the medulla oblongata was conventionally divided into four parts as follows: the first part extending from the medullospinal border to the caudal end of the inferior olivary complex (on average five sections per set, 5 × 6 × 30 µm = 0.90 mm); ahead of the first part, the second part extending to the caudal end of the area postrema (on average eight sections, ∼1.2 mm); the third part extending to the obex (on average five sections, ∼0.90 mm), and then the fourth part extending to the rostral end of the inferior olivary complex (on average 7 sections, ∼1.26 mm). The first and second parts belong to the caudal region of the gracile nucleus, while the third and fourth parts belong to the rostral region of the gracile nucleus. The cross sections containing the four parts were compared in immunohistochemistry.
Immunohistochemistry
Tissue sections were washed in 0.1 M PBS for 15 min, blocked for non-specific binding in 10% normal goat serum in 0.1 M PBS and 0.05% Triton X-100 for 1 h, and probed with a 1:800 dilution of rabbit polyclonal TRPV3 or POMC antibody (Santa Cruz Biotechnology, Santa Cruz CA) overnight at 4°C [Citation33–36]. The antigen-antibody complex was visualised using the Vectastain Avidin-Biotin Complex DAB method (Vector Laboratories, Burlingame, CA). The sections were mounted on Superfrost II slides and counterstained with haematoxylin (Fluka Mayer's haematoxylin solution). Following counterstaining, dehydration was done using ethyl alcohol and Histoclear clearing media (National Diagnostics, Atlanta, GA).
Data presentation and statistical analysis
The TRPV3 and POMC immunoreactivity in the brainstem nuclei were expressed as the numbers of positive staining cells in a microscopic area (200 × 200 µm) as described Citation[37]. The micrographs were quantified using a microscope with a reticule grid to count the number of positive colour stained cells in 8–10 non-overlapping tissue sections in each nucleus. As these brainstem nuclei are symmetrical, the left and right sides were counted individually and averaged. The quantitation for all slides was determined in a blinded fashion before comparison between different groups. Images for figures were acquired and processed using a Zeiss Axioskop40 microscope and digital camera and the corresponding Axiovision 2.0 software (Carl Zeiss USA, Thornwood, NY).
Results were expressed as mean + SEM. Five to seven rats were used for each defined group. Changes in POMC and TRPV3 immunoreactivity in rats receiving infrared heat treatment were compared with unheated control rats. Analysis of variance (ANOVA) and post hoc tests and Student's t-test were used to determine the significance of differences. P values less than 0.05 were considered significant.
Results
Physiological data
Food intake, arterial blood pressure, blood glucose, and body weight were compared between the heated OP (n = 7), unheated OP (n = 5), and OR (n = 5) rats over one week. As shown in , baseline MAP progressively increased over seven-days in OR and OP rats on high fat diet. This value is higher than normal rat blood pressure, defined as lower than 100 mm Hg MAP Citation[38]. All rats exhibited either hypertension or pre-hypertension, regardless of being OP or OR; there was no noticeable difference in mean arterial blood pressure. As shown in Table I, the baseline measurements of MAP in days 1 and 2 were similar among OR, OP and OP heated groups. Following six days of heating treatment, the averaged MAP in OP heated, OP non-heated and OR control rats were 109 ± 2.7, 107 ± 4, and 110 ± 6 mm Hg, respectively. Differences in arterial blood pressure between any of the groups were not statistically significant. The average blood glucose was 119 ± 3.9, 114 ± 2, and 118 ± 2.5 mg/dL for OP heated, OP control, and OR rats, respectively. Likewise there is no difference between groups. This is to be expected as the rats were fed ad libitum and not fasted.
compares food intake of all rats across the 8 days of experimentation. Daily food intake was significantly less in OR rats than in OP rats during the 8 days. Animals given heat treatment showed significantly decreased food intake across the 4 days of treatment, a trend that continued for the rest of the 8-day period. Heated OP rats averaged 24.3 ± 0.5 g of food per day across the 6 days of heat treatment, compared to OP control rats, which averaged 27.9 ± 0.8 g. OR control rats averaged 22.1 ± 0.4 g of food per day across these 6 days. Thus, food intake in heated OP was reduced to levels similar to but still slightly higher than OR control rats ().
Figure 2. Bar graph representing food intake of all rats across 8 days of experimentation. Infrared heat treatment started on day 3. OR rats showed significantly lowered food intake compared to OP controls throughout the experiment. OP heated rats showed significantly reduced food intake after 4 days of daily heat treatment. Each bar represents the mean values and vertical lines represent SEM (n = 5–7). *P < 0.05 (analysis of variance) compared with OR control; #P < 0.05 compared with OP control.
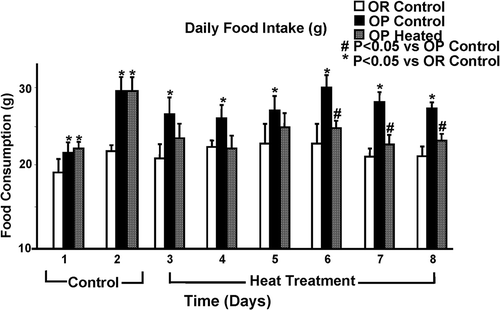
As shown in Table I, all rats exhibited a steady increase in body weight through the week of study. This is to be expected as 7-week-old rats are adolescent and undergoing development. At the end of the study, OP heated rats weighed on average 314.8 ± 10.9 g and OP control rats weighed 336.4 ± 11.2 g on average. OR rats, as expected, weighed significantly less than OP rats, at 260.1 ± 3.6 g. Heat treated OP rats weighed 7% less than OP control rats on average, but this difference fell short of statistical significance (Table I).
Table I. Mean arterial blood pressure and body weight of OP (Obese Prone) control rats compared to age matched OP heated and OR (Obese Resistant) control rats. Heating began on day 3 for OP Heated Rats.
TRPV3 Immunohistochemistry
The brainstem sections of heated OP rats (n = 7) were stained for TRPV3 immunoreactivity and compared with the brainstem tissue of OP control rats (n = 5) and OR control rats (n = 5). As illustrated in , TRPV3 is robustly localised in the HN of the dorsal medulla. The number of positive-staining neurons in the HN is reduced in OR control and OP heated rats (, top and bottom, respectively), and is highest in OP control rats (, middle). In other brainstem nuclei there was either no TRPV3 expression, or the difference in positive staining neuron count between OP/OR and OP/OP heated rats was indistinguishable.
Figure 3. These low (left) and high (right) magnification micrographs from medulla sections show the structures and distributions of TRPV3 staining in response to infrared heat treatment in an OR control rat (top), OP control (middle), and OP heated (bottom) rat. Full body infrared heat treatment resulted in a decrease of TRPV3 staining neurons (indicated by arrows) in the hypoglossal nucleus of the medulla (HgN) in an OP heated rat (bottom) compared to an OP control animal (middle).
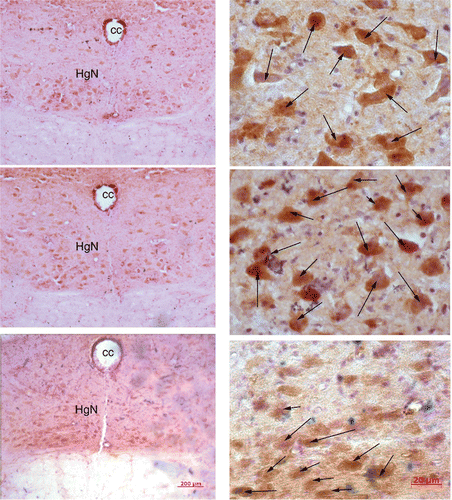
Infrared heating of OP rats resulted in a statistically significant reduction of the number of TRPV3 positive staining neurons in the HN section of the brainstem, to levels similar to OR rats, as shown in , left panel. The average number of TRPV3 positive cells per section area (200 × 200 µm) of the HN in heated OP rats was 17.0 ± 0.37, compared to 21.6 ± 0.47 in unheated control OP rats (, left panel). The average number of TRPV3 positive cells in the HN was 16.7 ± 0.8 in control OR rats. In the medial portion NTS (mNTS), there was also a statistically significant decrease of TRPV3 positive neurons following heat treatment. In heat treated OP rats the number of positive staining neurons in the mNTS was 17.1 ± 1.3 compared to 20.4 ± 0.78 in unheated OP rats, as shown in , right panel. The average number of TRPV3 positive cells in the mNTS was 17.7 ± 0.81 in control OR rats, which was less than those in control OP rats (, right panel). In both cases, the OP rats given heat treatment had their TRPV3 expression decreased to match those of their OR counterparts.
Figure 4. Quantitation of TRPV3 immunoreactivity in the hypoglossal nucleus and medial nucleus tractus solitarius (mNTS) in infrared heat-treated OP rats compared with OP control and OR control rats. TRPV3 immunostaining cells in the hypoglossal nucleus (left side) are significantly reduced in heat-treated OP rats and OR control rats, in comparison to OP control rats. In the mNTS (right side), OP heated and OR rats also displayed significantly lowered positive staining neuron counts, but the absolute difference was less. Each bar represents the mean values and the vertical lines represent SEM (n = 5–7). *P < 0.05 (analysis of variance), compared with OR control; #P < 0.05 compared with OP control.
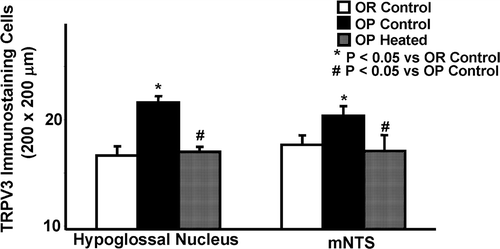
POMC Immunohistochemistry
Immunohistochemistry for POMC was performed in the dorsal medulla sections obtained from OP heated (n = 7), OP control (n = 5), and OR rats (n = 5). POMC localised in the same areas as TRPV3, namely the mNTS and HN. illustrates POMC immunoreactivity in the mNTS. The density of positive-staining neurons is higher in OR control and OP heated rats (, top and bottom, respectively) than in OP control rats (, middle). There was either no expression or no detectable difference of POMC positive neurons in the other brainstem nuclei between OR and OP groups, as well as OP and OP heated groups.
Figure 5. Micrographs, low (left) and high (right) magnification, showing number of POMC positive staining neurons in the medial NTS (mNTS) of an OR control (top), OP control (middle), and OP heated rat (bottom). POMC staining cells (indicated by arrows) were increased in the mNTS in the heat-treated OP rat (bottom) and OR control rat (top) when compared to the OP control (middle).
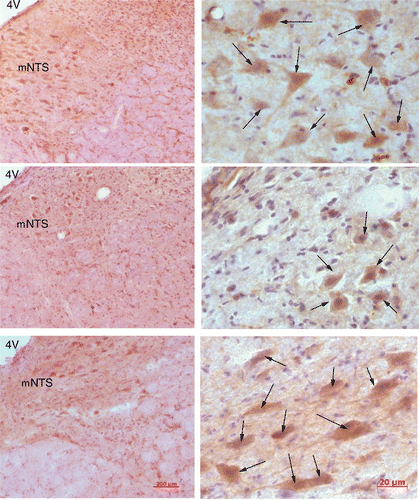
shows changes in POMC expression in the HN and mNTS of OR control, OP control rats, and OP heated rats. The average number of POMC positive cells per section area (200 × 200 µm) of the HN in heat-treated OP rats was 18.9 ± 1.1 compared to 15.4 ± 1.9 in untreated OP rats (, left panel). The average number of POMC positive cells in the HN was 19.9 ± 0.8 in control OR rats. This difference is short of statistical significance. In the mNTS, number of POMC positive neurons was significantly increased following heating treatment, as shown in , right panel. OP heated rats averaged 16.9 ± 0.84 positive-staining neurons, OP controls averaged 14.0 ± 0.25, and OR controls averaged 16.8 ± 0.56. As in the case of TRPV3, the OP rats given the infrared treatment had their POMC levels changed to levels similar to their OR counterparts.
Figure 6. Quantitation of POMC immunoreactivity in the hypoglossal nucleus and medial nucleus tractus solitarius (mNTS) in infrared heat-treated OP rats compared with OP control and OR control rats. POMC immunostaining cells in the hypoglossal nucleus (left side) are increased in heat-treated OP rats and OR control rats, in comparison to OP control rats, but this change was short of statistical significance. In the mNTS (right side), OP heated and OR rats displayed significantly increased POMC positive staining neuron counts. Each bar represents the mean values and the vertical lines represent SEM (n = 5–7). *P < 0.05 (analysis of variance), compared with OR control; #P < 0.05 compared with OP control.
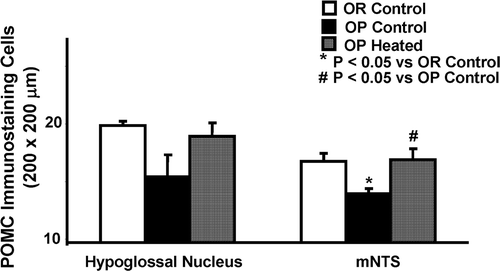
Discussion
We examined the influence of exposure to far-infrared radiation on expression of POMC and TRPV3 in the brainstem, particularly in the mNTS and HN in OP rats on a high fat diet. The effects of infrared heat on physiological feeding behaviour and arterial blood pressure were also investigated. The baseline difference in daily food intake, body weight, arterial blood pressure, as well as molecular expressions in the brainstem nuclei, were compared between OP rats and OR rats. The major new findings from this study are: (1) Food intake and body weight are higher in OP rats compared to OR strain, but MAP was similar between OP and OR rats and higher than normal in both strains; (2) TRPV3 expression is higher and POMC expression is lower in both the HN and mNTS of OP rats compared to OR rats; (3) Heat treatment with far-infrared radiation significantly lowers food intake but did not alter MAP and blood glucose in OP rats; and (4) Levels of TRPV3 and POMC in both the mNTS and HN of heat-treated OP rats decrease and increase, respectively, to match the levels expressed in the leaner OR strain. This is the first evidence showing that the increased food intake and body weight of OP rats are accompanied by a reduced expression of POMC, an anorectic agent, in the HN and the mNTS. It is also consistent with previous reports that OP rats have increased food intake and body weight, and that both strains exhibit high blood pressure compared to normal rats [Citation3–5].
There has been an increasing interest in the use of infrared radiation or elevated temperature to induce beneficial physiological effects over the last few decades. Kokura et al [6]. have demonstrated that treatment with far-infrared waves alleviates insulin resistance in rats with Type II diabetes Citation[3], while Capitano et al. have found that fever-range hyperthermia increases Natural killer cell activity in Type I diabetes model rats Citation[39]. The findings in the current study show that the predominant effect of far-infrared heat is a reduction of food intake in OP rats, a diet-induced metabolic syndrome model. Other symptoms of metabolic syndrome such as MAP and high blood glucose are not detectably altered by the treatment. Consistently, the number of POMC positive neurons was decreased in the HN and mNTS of OP rats and restored in the nuclei following heat treatment. TRPV3 positive staining neurons were higher in the HN and mNTS in OP rats compared to OR rats and also decreased by the treatment. These results suggest that lower POMC and higher TRPV3 expressions in the HN and mNTS play a role in central regulation of food intake, which contributes to development of hyperphagia and obesity in OP rats.
Previous studies Citation[17] have shown that mice lacking in TRPV1 receptors are protected against diet-induced obesity. It has also been shown that overexposure of the TRPV1 receptor to its agonist, capsaicin, the ingredient responsible for the ‘heat’ component of chilli peppers, can result in desensitisation of the receptor Citation[15],Citation[16], which in turn may be responsible for weight loss and changes in satiety in studies involving capsaicin infusion. Given the 40% homology in protein sequence between the TRPV1 receptor and TRPV3 receptor, we speculate that there may be a similar effect at work. TRPV3 is a receptor mainly for thermal stimuli of around 32–40°C Citation[13]. The present results show that TRPV3 expression in the HN and mNTS is higher in OP rats compared to OR rats. Heat treatment with far-infrared radiation significantly lowers food intake and reduces TRPV3 expression in the mNTS and HN in OP rats. Our results support the previous publications reported that the TRPV family may play a role in feeding behaviour and body weight signalling and further suggest that TRPV3 expression in the mNTS and HN is involved in feeding behaviour. The effects induced by heat treatment in this study may have been caused by exposure and desensitisation of the receptor similar to TRPV1 and capsaicin.
It is well-documented that POMC is a precursor to many behavioural signals, including ACTH, β-endorphin and α-MSH. These molecules downstream to POMC are involved in regulation of metabolism, reducing feeding activity, and lipolysis Citation[20], Citation[24]. Our results show that while POMC expression in the mNTS and HN is reduced in OP rats compared to OR rats, the expression of POMC is restored by whole body thermal therapy in OP rats and accompanied by a reduction of food intake. These results are consistent with that of other investigators, who have demonstrated that activation of POMC in the mNTS results in a sustained reduction of food intake Citation[19], Citation[25]. Physiological and anatomical studies have shown that the mNTS is a region of particularly dense POMC expression and mediates feeding behaviour. However, this region may also serve other autonomic functions, opening up the possibility that our findings might have significance for POMC-mediated autonomic regulation of food intake in the mNTS, modified by temperature and thermotherapy.
With regard to the potential role of POMC and TRPV3 in the HN on regulation of food intake, our results show that TRPV3 and POMC are both clearly expressed in the HN. POMC and TRPV3 levels in the HN rise and fall, respectively, in heated OP rats to levels similar to that of control OR rats. The results suggest that feeding behaviour could possibly be mediated by POMC in the brainstem nuclei, HN and mNTS, through the TRPV receptor pathways in response to heat and infrared radiation. Previous studies have demonstrated that the HN is the centre of motor afferent control for lower jaw and tongue movements Citation[29], and is involved in taste and gustatory responses in the medulla Citation[30], Citation[31]. The somatosensory information travelling along the forelimb nerves also modulates firing neurons in the HN, suggesting that somatosensory signals, together with visual messages, induce tongue reflex responses functionally directed to modulate the postural tone of the tongue and the oral cavity for food reception [Citation40–42]. Our findings would be consistent with this possibility, but we cannot exclude other influences of POMC and TRPV3 in the HN via somatosensory signals, cheek muscle contraction, and taste. Moreover, the regulatory function, pathways, and precise site of the neurons affected by the molecules applied is still unclear. A more sophisticated approach would be required to address these issues. A long-term experiment to observe the effects of chronic treatment over time would also be significant to explore the sustainability of the physiological changes. Despite these limitations, our physiological and immunohistochemical results consistently suggest expression of POMC and TRPV3 in the HN is associated with food intake.
Conclusion
In summary, these results indicate that infrared heating decreases food intake and tends to decrease body weight in OP rats but does not affect other aspects of metabolic syndrome. Consistent with these observations is the lowered TRPV3 and heightened POMC expression in the feeding-related brainstem nuclei of heat treated OP rats compared to control OP rats. Thus, infrared heating may serve to normalise expressions of these molecules in the brain, which in turn leads to reduction of food intake. This is the first evidence in OP rats indicating such a connection, and that thermotherapy may be used to alleviate hyperphagia in metabolic syndrome.
Acknowledgements
These studies were conducted at the biomedical research facilities of the Los Angeles Biomedical Research Institute at Harbor-UCLA Medical Center. The authors would like to thank Xi-yan Li for her technical assistance.
Declaration of interest: This project was made possible by US National Institutes of Health grants (AT004504, and AT004620) from the National Center for Complementary and Alternative Medicine, and a Research Award (ADA 7-07-RA-100) from the American Diabetes Association to Sheng-Xing Ma. The authors alone are responsible for the content and writing of the paper.
References
- Eckel RH, Alberti KG, Grundy SM, Zimmet PZ. The metabolic syndrome. Lancet 2010; 375: 181–183
- Grundy SM, Brewer HB, Jr, Cleeman JI, Smith SC, Jr, Lenfant C. Definition of metabolic syndrome: Report of the National Heart, Lung, and Blood Institute/American Heart Association conference on scientific issues related to definition. Arterioscler Thromb Vasc Biol 2004; 24: e13–18
- Geiger BM, Behr GG, Frank LE, Caldera-Siu AD, Beinfeld MC, Kokkotou EG, Pothos EN. Evidence for defective mesolimbic dopamine exocytosis in obesity-prone rats. FASEB J 2008; 22: 2740–2746
- Li S, Zhang HY, Hu CC, Lawrence F, Gallagher KE, Surapaneni A, Estrem ST, Calley JN, Varga G, Dow ER, Chen Y. Assessment of diet-induced obese rats as an obesity model by comparative functional genomics. Obesity (Silver Spring) 2008; 16: 811–818
- Tschop M, Heiman ML. Rodent obesity models: An overview. Exp Clin Endocrinol Diabetes 2001; 109: 307–319
- Kokura S, Adachi S, Manabe E, Mizushima K, Hattori T, Okuda T, Nakabe N, Handa O, Takagi T, Naito Y, et al. Whole body hyperthermia improves obesity-induced insulin resistance in diabetic mice. Int J Hyperthermia 2007; 23: 259–265
- Chung MK, Guler AD, Caterina MJ. Biphasic currents evoked by chemical or thermal activation of the heat-gated ion channel, TRPV3. J Biol Chem 2005; 280: 15928–15941
- Moqrich A, Hwang SW, Earley TJ, Petrus MJ, Murray AN, Spencer KS, Andahazy M, Story GM, Patapoutian A. Impaired thermosensation in mice lacking TRPV3, a heat and camphor sensor in the skin. Science 2005; 307: 1468–1472
- Zimmermann K, Leffler A, Fischer MM, Messlinger K, Nau C, Reeh PW. The TRPV1/2/3 activator 2-aminoethoxydiphenyl borate sensitizes native nociceptive neurons to heat in wildtype but not TRPV1 deficient mice. Neuroscience 2005; 135: 1277–1284
- Chung MK, Lee H, Mizuno A, Suzuki M, Caterina MJ. 2-aminoethoxydiphenyl borate activates and sensitizes the heat-gated ion channel TRPV3. J Neurosci 2004; 24: 5177–5182
- Smith GD, Gunthorpe MJ, Kelsell RE, Hayes PD, Reilly P, Facer P, Wright JE, Jerman JC, Walhin JP, Ooi L, et al. TRPV3 is a temperature-sensitive vanilloid receptor-like protein. Nature 2002; 418: 186–190
- Vriens J, Appendino G, Nilius B. Pharmacology of vanilloid transient receptor potential cation channels. Mol Pharmacol 2009; 75: 1262–1279
- Xu H, Ramsey IS, Kotecha SA, Moran MM, Chong JA, Lawson D, Ge P, Lilly J, Silos-Santiago I, Xie Y, et al. TRPV3 is a calcium-permeable temperature-sensitive cation channel. Nature 2002; 418: 181–186
- Huang SM, Lee H, Chung MK, Park U, Yu YY, Bradshaw HB, Coulombe PA, Walker JM, Caterina MJ. Overexpressed transient receptor potential vanilloid 3 ion channels in skin keratinocytes modulate pain sensitivity via prostaglandin E2. J Neurosci 2008; 28: 13727–13737
- Cioffi DL. The skinny on TRPV1. Circ Res 2007; 100: 934–936
- Zhang LL, Yan LD, Ma LQ, Luo ZD, Cao TB, Zhong J, Yan ZC, Wang LJ, Zhao ZG, Zhu SJ, et al. Activation of transient receptor potential vanilloid type-1 channel prevents adipogenesis and obesity. Circ Res 2007; 100: 1063–1070
- Motter AL, Ahern GP. TRPV1-null mice are protected from diet-induced obesity. FEBS Lett 2008; 582: 2257–2262
- Suri A, Szallasi A. The emerging role of TRPV1 in diabetes and obesity. Trends Pharmacol Sci 2008; 29: 29–36
- Wang JH, Wang F, Yang MJ, Yu DF, Wu WN, Liu J, Ma LQ, Cai F, Chen JG. Leptin regulated calcium channels of neuropeptide Y and proopiomelanocortin neurons by activation of different signal pathways. Neuroscience 2008; 156: 89–98
- Cone RD. Anatomy and regulation of the central melanocortin system. Nat Neurosci 2005; 8: 571–578
- Fan W, Boston BA, Kesterson RA, Hruby VJ, Cone RD. Role of melanocortinergic neurons in feeding and the agouti obesity syndrome. Nature 1997; 385: 165–168
- Cone RD. The central melanocortin system and energy homeostasis. Trends Endocrinol Metab 1999; 10: 211–216
- Schwartz MW, Seeley RJ, Woods SC, Weigle DS, Campfield LA, Burn P, Baskin DG. Leptin increases hypothalamic pro-opiomelanocortin mRNA expression in the rostral arcuate nucleus. Diabetes 1997; 46: 2119–2123
- Seo YJ, Kwon MS, Choi SS, Han EJ, Jung JS, Choi HW, Park SH, Jang JE, Suh HW. Characterization of the hypothalamic proopiomelanocortin gene and beta-endorphin expression in the hypothalamic arcuate nucleus of mice elicited by inflammatory pain. Neuroscience 2008; 152: 1054–1066
- Li G, Zhang Y, Rodrigues E, Zheng D, Matheny M, Cheng KY, Scarpace PJ. Melanocortin activation of nucleus of the solitary tract avoids anorectic tachyphylaxis and induces prolonged weight loss. Am J Physiol Endocrinol Metab 2007; 293: E252–258
- Abboud FM, Thames MD. Interaction of Cardiovascular Reflexes in Circulatory Controlsecond. American Physiological Society, Bethesda, MD 2011
- Toney GM, Mifflin SW. Sensory modalities conveyed in the hindlimb somatic afferent input to nucleus tractus solitarius. J Appl Physiol 2000; 88: 2062–2073
- Wang X, Miyares RL, Ahern GP. Oleoylethanolamide excites vagal sensory neurones, induces visceral pain and reduces short-term food intake in mice via capsaicin receptor TRPV1. J Physiol 2005; 564: 541–547
- Lorier AR, Funk GD, Greer JJ. Opiate-induced suppression of rat hypoglossal motoneuron activity and its reversal by ampakine therapy. PLoS One 2010; 5: e8766
- Streefland C, Jansen K. Intramedullary projections of the rostral nucleus of the solitary tract in the rat: Gustatory influences on autonomic output. Chem Senses 1999; 24: 655–664
- Swank MW, Bernstein IL. c-Fos induction in response to a conditioned stimulus after single trial taste aversion learning. Brain Res 1994; 636: 202–208
- Emond MH, Weingarten HP. Fos-like immunoreactivity in vagal and hypoglossal nuclei in different feeding states: A quantitative study. Physiol Behav 1995; 58: 459–465
- Ma S, Cornford ME, Vahabnezhad I, Wei S, Li X. Responses of nitric oxide synthase expression in the gracile nucleus to sciatic nerve injury in young and aged rats. Brain Res 2000; 855: 124–131
- Ma SX, Ma J, Moise G, Li XY. Responses of neuronal nitric oxide synthase expression in the brainstem to electroacupuncture Zusanli (ST 36) in rats. Brain Res 2005; 1037: 70–77
- Milanski M, Degasperi G, Coope A, Morari J, Denis R, Cintra DE, Tsukumo DM, Anhe G, Amaral ME, Takahashi HK, et al. Saturated fatty acids produce an inflammatory response predominantly through the activation of TLR4 signaling in hypothalamus: Implications for the pathogenesis of obesity. J Neurosci 2009; 29: 359–370
- Moraes JC, Coope A, Morari J, Cintra DE, Roman EA, Pauli JR, Romanatto T, Carvalheira JB, Oliveira AL, Saad MJ, et al. High-fat diet induces apoptosis of hypothalamic neurons. PLoS One 2009; 4: e5045
- Miura M, Okada J, Takayama K, Jingu H. Barosensitive and chemosensitive neurons in the rat medulla: A double labeling study with c-Fos/glutamate, GAD, PNMT and calbindin. J Auton Nerv Syst 1996; 61: 17–25
- Pass D, Freeth G. The Rat. ANZCCART News 1993; 6: 1–4
- Capitano ML, Ertel BR, Repasky EA, Ostberg JR. Winner of the 2007 Society for Thermal Medicine Young Investigator Award. Fever-range whole body hyperthermia prevents the onset of type 1 diabetes in non-obese diabetic mice. Int J Hyperthermia 2008; 24: 141–149
- Borke RC, Nau ME, Ringler RL, Jr. Brain stem afferents of hypoglossal neurons in the rat. Brain Res 1983; 269: 47–55
- Elmund J, Bowman JP, Morgan RJ. Vestibular influence on tongue activity. Exp Neurol 1983; 81: 126–140
- Mameli O, Tolu E. Somatosensory input from the forelimb nerves to the hypoglossal neurons. Exp Neurol 1986; 94: 757–766