Abstract
Purpose: We investigated the use of hyperthermia to improve the anti-cancer efficacy of doxorubicin (DOX)-loaded mesoporous silica nanocontainer Si-SS-CD-PEG. The hypothesis was that heat stimulates glutathione-mediated degradation of cyclodextrin gatekeeper, thereby causing the release of DOX from the carrier and DOX-induced cell death.
Materials and methods: The release of DOX from DOX-loaded Si-SS-CD-PEG suspended in PBS containing glutathione (GSH) was studied by assessing the changes in DOX fluorescence intensity. The effect of heating at 42°C on the release of DOX from the intracellular carriers was determined with confocal microscopy. The extents of clonogenic and apoptotic cell death caused by DOX-loaded Si-SS-CD-PEG were determined.
Results: The release of DOX from DOX-loaded Si-SS-CD-PEG in PBS occurred only when GSH presented in the suspension, and heating at 42°C slightly increased the release of DOX from the carriers. Heating significantly elevated the GSH content in A549 cells and increased the release of DOX from the internalised carriers. Heating the cancer cells treated with the carriers at 42°C markedly increased the clonogenic death and apoptosis. The GSH content in A549 cells was greater than that in L-132 cells, and A549 cells were far more sensitive than L-132 cells to DOX-loaded Si-SS-CD-PEG at both 37°C and 42°C.
Conclusions: Hyperthermia increased the GSH-mediated release of DOX from DOX-loaded Si-SS-CD-PEG. Furthermore, hyperthermia markedly elevated the GSH content in cancer cells, thereby increasing the release of DOX from the internalised carriers and potentiating the DOX-induced clonogenic and apoptotic cell death.
Introduction
Numerous nanosized drug-carriers have been developed in recent years to improve delivery of anti-cancer drugs to target sites. Such drugs, either attached to the surface of nanocarriers or encapsulated in the carriers, are usually released at target sites by activation of triggering motifs including redox change Citation[1], pH Citation[2], temperature Citation[3] or enzyme availability Citation[4]. Stimulus-responsive silica nanoparticles featuring porous channels offer great potential for drug delivery owing to their high stability and unique responsiveness to diverse external stimuli [Citation5–7]. Mesoporous silica nanoparticles (Si-MPs) have been shown to be excellent hosts and carriers of molecules of various sizes, shapes, and functionalities. Guest molecules are retained inside Si-MPs using various pore gatekeepers including rotaxanes, polypseudorotaxanes, magnetic nanoparticles, gold nanoparticles, cucurbiturils, antibodies, dendrimers, or anions Citation[2], Citation[8–15]]. Controlled release of guest molecules from pores can be achieved using appropriate external triggering motifs, depending on the nature of the gatekeeper. Cyclodextrins (CDs) of various forms have been demonstrated to be effective pore gatekeepers for Si-MPs Citation[2], Citation[4], Citation[16]. We previously reported that such gatekeepers could be covalently connected using disulphide units, to surface particles of Si-MPs; the disulphide units are easily cleaved by glutathione (GSH) Citation[17]. Importantly, Si-MPs loaded with doxorubicin (DOX) are internalised by cancer cells and DOX can be released upon cleavage of the disulphide unit by intracellular GSH, in turn killing the target cells Citation[17].
The therapeutic potential of hyperthermia used alone or in combination with either radiotherapy or chemotherapy for the control of cancer has been demonstrated in recent years [Citation18–21]. Heat-induced enhancement of chemotherapeutic efficacy may be attributable to: (i) an increased direct cytotoxicity of a drug; (ii) an increased cellular uptake of a drug; and/or (iii) an enhanced drug delivery via a heat-induced increase in blood flow and vascular permeability. In addition, hyperthermia has been shown to be an effective means to improve drug delivery with heat-labile liposomes containing cytotoxic compounds Citation[22].
The purpose of the present study was to investigate the feasibility of using hyperthermia to improve the release of DOX from pores of DOX-loaded Si-SS-CD-PEG, in turn increasing DOX-induced cell death. We observed that heating increased the intracellular levels of GSH and enhanced the GSH-mediated dissociation of CD gatekeepers from the carriers, thereby increasing the release of DOX from the carrier. Consequently, heating markedly increased the efficacy of DOX-loaded Si-SS-CD-PEG against A549 human lung cancer cells.
Materials and methods
Synthesis of doxorubicin-loaded Si-SS-CD-PEG
We previously described the synthesis of DOX-loaded Si-SS-CD-PEG Citation[17]. We first prepared mesoporous silica spheres (Si-MP-O) featuring hexagonally ordered porous channels of average diameter of 60 nm. Pores with an average inner diameter of 2.5 nm serve as nanocontainers for the DOX guest molecule. As shown in , CD gatekeepers were attached to Si-MP-O using disulphide stalk moieties (Si-SS-CD), employing a series of surface functionalisation processes. Finally, Si-SS-CD was PEGylated to produce Si-SS-CD-PEG, using isocyanate-terminated polyethylene glycol (NCO-PEG), to improve the solubility of the silica nanocontainers in aqueous solution. DOX was loaded into the carrier by co-incubation of 5 mg DOX and 20 mg Si-SS-alkyne in 2 mL PBS for 24 h prior to attachment of the gatekeeper CD (B, step 4).
Figure 1. A schematic diagram showing the functionalisation steps from Si-MP-O to DOX-loaded Si-SS-CD-PEG. (A) The concept of GSH-induced release of DOX from the pores of Si-SS-CD-PEG. (B) The synthetic steps from Si-MP-O to the Si-SS-CD-PEG nanocontainer Citation[17]: (1) addition of 3-mercaptopropyltrimethoxysilane; (2) use of S-(2-aminoethylthio)-2-thiopyridine hydrochloride; (3) addition of propargyl bromide; (4) removal of CTAB; followed by DOX loading and treatment with CuSO4, sodium ascorbate, and mono-6-azido-β-CD; (5) Addition of NCO-PEG5000 and dibutyltin dilaurate.
![Figure 1. A schematic diagram showing the functionalisation steps from Si-MP-O to DOX-loaded Si-SS-CD-PEG. (A) The concept of GSH-induced release of DOX from the pores of Si-SS-CD-PEG. (B) The synthetic steps from Si-MP-O to the Si-SS-CD-PEG nanocontainer Citation[17]: (1) addition of 3-mercaptopropyltrimethoxysilane; (2) use of S-(2-aminoethylthio)-2-thiopyridine hydrochloride; (3) addition of propargyl bromide; (4) removal of CTAB; followed by DOX loading and treatment with CuSO4, sodium ascorbate, and mono-6-azido-β-CD; (5) Addition of NCO-PEG5000 and dibutyltin dilaurate.](/cms/asset/63aea719-1bd9-4e30-b749-744b6f9f3a11/ihyt_a_608217_f0001_b.gif)
Estimation of doxorubicin levels released from DOX-loaded Si-SS-CD-PEG
DOX-loaded Si-SS-CD-PEG was placed in a quartz cuvette filled with PBS with or without 1 mM GSH and maintained at 37°C or 42°C. The release of doxorubicin from DOX-loaded Si-SS-CD-PEG was determined by measuring the fluorescence intensity in the PBS with fluorescence emission spectrometry at 558 nm with excitation at 485 nm.
Cell culture
A549 human lung cancer cells, L-132 human lung epithelial cells, RKO human colon cancer cells, HeLa human cervical cancer cells and MIA PaCa-2 human pancreatic cancer cells were maintained in DMEM (Gibco, Gaithersburg, MD) supplemented with 10% (v/v) fetal bovine serum (FBS, Gibco) and 1% (w/v) penicillin/streptomycin (Gibco) in a humidified 5% CO2 incubator at 37°C.
Hyperthermia
Cells were seeded into 25-cm2 flasks and incubated overnight at 37°C in a humidified 5% CO2 incubator. The flasks were tightly sealed and immersed in a water bath preset to 42°C. After heating for 1 h, cells were returned to the incubator at 37°C.
Confocal laser scanning microscopy to assess DOX release
To microscopically assess intracellular release of DOX from drug-loaded Si-SS-CD-PEG, cells grown on Lap-Tek II coverglass were incubated with free Si-SS-CD-PEG or DOX-loaded Si-SS-CD-PEG in 10% (v/v) FBS/PBS at 37°C or 42°C for 1 h. Cells were then maintained at 37°C for 7 h, and fluorescence imaging of DOX was assessed using a Nikon C1-Plus laser-scanning TE2000-E confocal microscope (Nikon, Tokyo, Japan). Control cells were maintained at 37°C for 8 h. Quantitative analysis of fluorescent signal of image captured from the confocal microscope was performed by using the Image J software (NIH) program. Briefly, three fields including 60–70 cells per field captured from the confocal microscope were measured with the ‘analyse particles’ functions of Image J. Total intensity of DOX fluorescence in the field was determined by using the Image J's thresholding tool and then the relative fluorescence intensity of DOX per cell was re-calculated.
Clonogenic cell survival
A549 cells were seeded into 6-well plates and incubated overnight in a humidified 5% CO2 incubator at 37°C. Cells were next treated with free Si-SS-CD-PEG or DOX-loaded Si-SS-CD-PEG at 37°C or 42°C for 1 h, and further incubated with the carriers at 37°C for 23 h. Cells were then gently washed three times with PBS and cultured for a further 10–14 days. After fixing and staining with 1% (w/v) crystal violet in ethanol, colonies containing more than 50 cells were counted, and the surviving fractions of cells were calculated.
TUNEL assay
Cells were grown in 8-well chamber slides, treated with free Si-SS-CD-PEG or DOX-loaded Si-SS-CD-PEG at 37°C or 42°C for 1 h, and further incubated at 37°C for 23 h. After fixing with 4% (v/v) paraformaldehyde for 15 min, cells were washed with 1% (w/v) BSA/PBS, permeabilised with 0.1% (v/v) Triton-X100, again washed with PBS, and incubated for 1 h at 37°C in the dark with apoptosis detection solution (Apoptosis Detection System kit, Roche Molecular Biochemicals, Mannheim, Germany). In situ labelled nuclei were observed and photographed using a Nikon C1-Plus laser-scanning TE2000-E confocal microscope.
Immunoblotting
Cell lysates were prepared in lysis buffer (10 mM Tris-HCl (pH 7.6), 150 mM NaCl, 1 mM EDTA, 1% (v/v) NP-40, 0.05% (w/v) sodium dodecyl sulphate (SDS), and 1 mM phenylmethanesulphonylfluoride). Proteins in the lysates were resolved by SDS-polyacrylamide gel electrophoresis (PAGE) and transferred to nitrocellulose membranes (Amersham, Piscataway, NJ). The membranes were blocked with 1% (w/v) non-fat dry milk powder dissolved in Tris-buffered saline containing 0.05% (v/v) Tween 20 (TBST), and subsequently incubated with primary antibodies overnight at 4°C. Reactive bands were detected by incubation with goat anti-rabbit or anti-mouse IgG conjugated with horseradish peroxidase (Amersham), followed by visualisation using an enhanced chemiluminescence technique (Pierce, Rockford, IL).
Determination of total GSH level
The GSH content in cells was determined using a glutathione assay kit (Sigma, St Louis, MO) both before and after heating the cells. Cells suspended in PBS at 1 × 108 cells/mL were centrifuged at 600 × g. After addition of 5% (v/v) 5-sulphosalicylic acid solution, the cell suspensions were frozen and thawed twice, held at 4°C for 5 min, and next centrifuged at 10,000 × g for 10 min. The glutathione concentrations in supernatants were measured spectrophotometrically at 412 nm, as prescribed in the manual of the glutathione assay kit.
Statistical analysis
Differences between groups were analysed using student's t-test, and a P value <0.05 was considered to be statistically significant.
Results
Hyperthermia facilitates GSH-induced DOX release from Si-SS-CD-PEG in vitro
Previously, we showed that incubation of DOX-loaded Si-SS-CD-PEG with GSH in PBS resulted in cleavage of CD gatekeepers via GSH-mediated reduction of the disulphide stalk moiety, causing release of DOX from the silica nanocontainer reservoir Citation[17]. In the present work, we investigated DOX release from DOX-loaded Si-SS-CD-PEG suspended in PBS with or without 1 mM GSH at 37°C or 42°C by measuring the fluorescence intensity of released DOX molecules (). No detectable DOX release was evident in the absence of GSH at either 37°C or 42°C, whereas DOX was released rapidly in the presence of GSH. Although the release of DOX in the presence of GSH at 42°C was noticeably faster than that at 37°C, the lack of DOX release in the absence of GSH even at 42°C indicated that GSH was the dominant factor for DOX release under the cell-free system.
Figure 2. Representative release profile of DOX from DOX-loaded Si-SS-CD-PEG in PBS containing 0 mM or 1 mM GSH at 37°C or 42°C. (1) Without GSH at 37°C; (2) without GSH at 42°C; (3) with 1 mM GSH at 37°C; (4) with 1 mM GSH at 42°C.
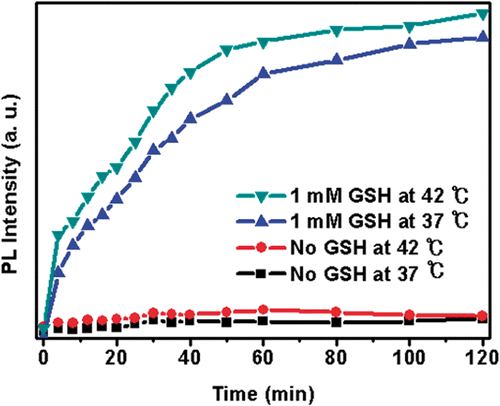
As shown in , considerable DOX fluorescence was evident in cells treated with DOX-loaded Si-SS-CD-PEG at 37°C for 8 h indicating that DOX was released from internalised carriers as a consequence of cleavage of the CD gatekeeper caused by intracellular GSH. The DOX fluorescence intensity within cells treated with DOX-loaded Si-SS-CD-PEG at 42°C for 1 h and further incubated for 7 h at 37°C was about 4-fold greater than that in the cells treated with DOX-loaded Si-SS-CD-PEG at 37°C for 8 h (). When cells were washed after 1 h exposure to DOX-loaded Si-SS-CD-PEG, the DOX intensity was markedly less than that in the cells continually maintained with DOX-loaded Si-SS-CD-PEG (). The GSH level in carrier-free A549 cells slightly increased upon heating at 42°C for 1 h, and it steadily increased thereafter reaching a peak level at 7 h after the heating (). It was thus apparent that the increase in DOX release from pores of Si-SS-CD-PEG by heating at 42°C () was due to a heat-induced increase in GSH level.
Figure 3. Effect of hyperthermia on intracellular release of DOX from DOX-loaded Si-SS-CD-PEG in A549 cells. (A) Representative fluorescence images of A549 cells incubated with DOX-loaded Si-SS-CD-PEG at 37°C or 42°C. Upper panel: cells were first heated at 42°C for 1 h with DOX-loaded Si-SS-CD-PEG, and then further incubated with DOX-loaded Si-SS-CD-PEG at 37°C for 7 h. Lower panel: cells were treated with DOX-loaded Si-SS-CD-PEG at 42°C for 1 h, washed and incubated at 37°C for 7 h. The amount of internalised DOX was detected by using a confocal microscopy. (B) The relative fluorescence intensities of DOX per cell were quantified. Data are expressed as means of three independent experiments ± 1 SE. (C) Relative levels of GSH in A549 cells at 37°C or 42°C. A549 cells were exposed to heat for 1 h, and then further incubated at 37°C for 3 to 9 h. Data are means of thee independent experiments ± 1 SE. *P < 0.05, **P < 0.01, ***P < 0.001. MTH, mild temperature hyperthermia.
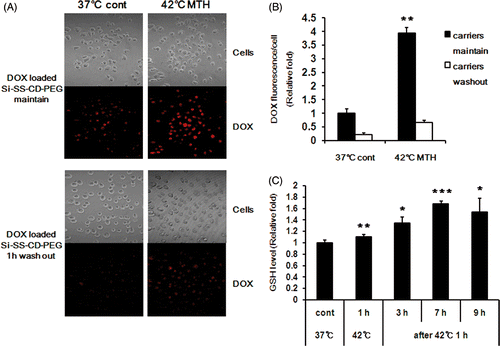
The influence of the intracellular GSH concentration on DOX release was further investigated by comparing the DOX release in A549 cells, which contain high concentration of GSH Citation[23], and human lung epithelial cell L-132, which contain less GSH than A549 cells as shown in . The GSH level in L-132 cells increased only slightly, whereas that in A549 cells significantly increased. The DOX fluorescence intensity in L-132 cells was far less than that in A549 cells after incubating the cells with DOX-loaded Si-SS-CD-PEG at 37°C for 8 h and also after heating at 42°C for 1 h and incubating for 7 h at 37°C ().
Figure 4. Effect of intracellular GSH concentration on DOX release in A549 and L-132 cells. (A) The relative levels of GSH in A549 and L-132 cells at 37°C and 42°C. The cells were heated at 42°C for 1 h, and then further incubated at 37°C for 7 h. Average values obtained from three independent experiments ± 1 SE are shown. (B) Representative fluorescence images of A549 and L-132 cells incubated with DOX-loaded Si-SS-CD-PEG at 37°C for 8 h or incubated with DOX-loaded Si-SS-CD-PEG at 42°C for 1 h and then incubated at 37°C for 7 h. The amount of internalised DOX was detected by using a confocal microscopy. (C) The relative fluorescence intensities of DOX per cell were quantified by pixel intensity using Image J software (NIH), and data was normalised to the cell number. Data are expressed as means of three independent experiments ± 1 SE. **P < 0.01.
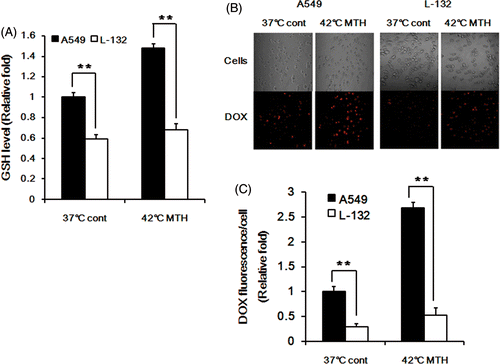
To confirm that increase in intracellular GSH concentration was the cause of the increased release of DOX after heating at 42°C, we conducted further studies with other human cancer cell lines including RKO colon cancer cells, HeLa cervical cancer cells and MIA PaCa-2 pancreatic cancer cells. Heating at 42°C increased the release of DOX from the carriers in all these three cell lines () and heating also increased the GSH content in the cells (). Taken together, these results clearly demonstrated that the release of DOX from the internalised DOX-loaded Si-SS-CD-PEG is dependent on the intracellular level of GSH.
Figure 5. Effect of hyperthermia on intracellular release of DOX from DOX-loaded Si-SS-CD-PEG in RKO, HeLa and MIA PaCa-2 cells. (A) Representative fluorescence images of the cells incubated with DOX-loaded Si-SS-CD-PEG at 37°C for 8 h or heated at 42° for 1 h with DOX-loaded Si-SS-CD-PEG, and then further incubated with the carrier at 37°C for 7 h. The amount of internalised DOX was detected by using confocal microscopy. (B) The relative fluorescence intensities of DOX per cell were quantified. Data are expressed as means of three independent experiments ± 1 SE. (C) The relative levels of GSH in the cells at 37°C or that in the cells heated at 42°C for 1 h and then further incubated at 37°C for 7 h. Data are means of thee independent experiments ± 1 SE. *P < 0.05, **P < 0.01, ***P < 0.001.
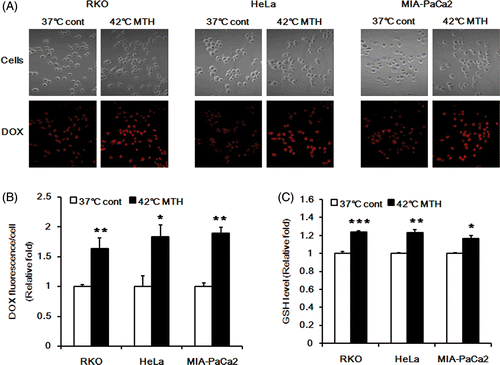
Hyperthermia increases clonogenic cell death caused by DOX-loaded Si-SS-CD-PEG
The clonogenic death of A549 cells treated with free Si-SS-CD-PEG or DOX-loaded Si-SS-CD-PEG for 24 h is shown in . Considerable clonogenic death occurred after treatment with 1 µM DOX-loaded Si-SS-CD-PEG at 37°C or 42°C. The cell death upon treatment with 2 µM or 5 µM DOX at 42°C was markedly greater than that at 37°C. For example, when A549 cells were treated with 2 µM DOX-loaded Si-SS-CD-PEG, the clonogenic death at 42°C was about 10-fold greater than that at 37°C.
Figure 6. Effect of hyperthermia on the clonogenic survival of A549 cells treated with DOX-loaded Si-SS-CD-PEG. (A) A549 cells were heated at 42°C for 1 h with free Si-SS-CD-PEG or DOX-loaded Si-SS-CD-PEG and then further incubated with the carrier at 37°C for 23 h. After the incubation, cells were washed and cultured for a further 10–14 days, and survival proportions were calculated. (B) A549 and L-132 cells were heated at 42°C for 1 h with free DOX, washed, cultured for a further 10–14 days, and the surviving fractions were obtained from the number of colonies. Data are as means of three independent experiments ± 1 SE. *P < 0.05, **P < 0.01, ***P < 0.001.
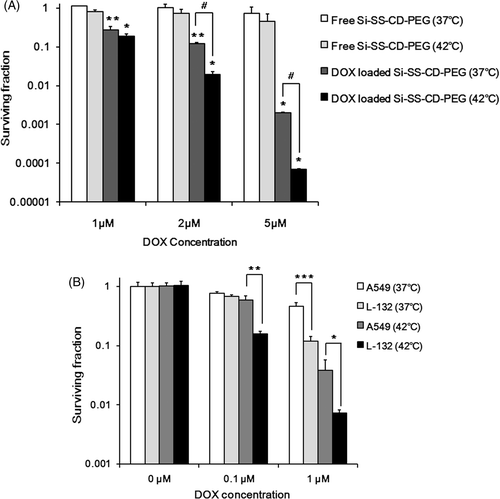
In addition, we investigated the effects of free DOX with or without hyperthermia on the clonogenic survival of A549 and L-132 cells. As shown in , heating at 42°C for 1 h alone caused little change in the clonogenic survival of both cell lines. When treated with 1 µM DOX at 37oC, significantly more cell death occurred in L-132 cells than A549 cells indicating that L-132 cells are more sensitive to DOX. Heating at 42°C for 1 h significantly increased the sensitivity of both A549 and L-132 cells to DOX. It is of note that the clonogenic survival of L-132 cells was lower than that of A549 cells by more than 1 log.
Hyperthermia increases apoptosis of A549 cells caused by DOX-loaded Si-SS-CD-PEG
The levels of apoptotic cell death caused by DOX-loaded Si-SS-CD-PEG at 37°C or 42°C were determined using the TUNEL assay. As shown in , free Si-SS-CD-PEG caused no detectable apoptosis in A549 cells at either 37°C or 42°C, whereas DOX-loaded Si-SS-CD-PEG caused significant apoptotic cell death particularly at 42°C. The apoptotic death 23 h after treatment with DOX-loaded Si-SS-CD-PEG at 42°C for 1 h was about 2.5 times greater than that caused by treating the cells with DOX-loaded Si-SS-CD-PEG at 37°C. The effects of DOX-loaded Si-SS-CD-PEG on the activities of molecular signals associated with apoptosis are shown in . When cells were exposed to DOX-loaded Si-SS-CD-PEG at 42°C for 1 h and maintained at 37°C for 11 h, caspase 3, caspase 8, and caspase 9 were all cleaved (activated), thereby leading to a significant cleavage of poly(ADP-ribose)polymerase (PARP). Interestingly, no measurable changes in caspase activity or PARP cleavage were observed in the cells treated with DOX-loaded Si-SS-CD-PEG at 37°C.
Figure 7. Effect of hyperthermia on the level of apoptosis caused by DOX-loaded Si-SS-CD-PEG and molecular signals involved in apoptosis (A) Representative photomicrographs of apoptotic cells, i.e. TUNEL-positive cells, after treatment with free Si-SS-CD-PEG or DOX-loaded Si-SS-CD-PEG at 37°C or 42°C. A549 cells were first heated at 42°C for 1 h with free Si-SS-CD-PEG or DOX- Si-SS-CD-PEG and then incubated with the carrier at 37°C for 23 h. TUNEL-positive cells were detected under a confocal microscopy. (B) Relative fluorescence intensities were quantified and normalised to the fluorescence intensity of DAPI. Data are expressed as means of three independent experiments ± 1 SE. (C) Effect of hyperthermia on the caspase activity in cells treated with DOX-loaded Si-SS-CD-PEG. Cells were heated at 42°C for 1 h with free Si-SS-CD-PEG or DOX-loaded Si-SS-CD-PEG, and then further incubated with the carrier at 37°C for 11 h. Protein levels were determined by western blotting. Representative examples from five repeat experiments are shown. *P < 0.05, **P < 0.01.
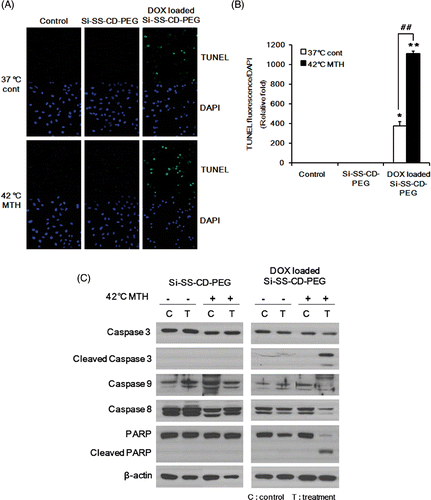
The influence of intracellular GSH levels on the cytotoxicity of DOX-loaded Si-SS-CD-PEG to cause apoptosis was examined by comparing the levels of apoptosis in A549 and L-132 cells. As shown in , at both 37°C and 42°C, DOX-loaded Si-SS-CD-PEG caused significantly more apoptosis in A549 cells than in L-132 cells, which contained less GSH level than A549 cells (). These results provide further evidence that GSH plays a central role in the release of DOX from internalised DOX-loaded Si-SS-CD-PEG and the resultant DOX-induced cell death.
Figure 8. Comparison of apoptosis in A549 and L-132 cells. (A) Representative photomicrographs of apoptotic cells, i.e. TUNEL-positive in A549 and L-132 cells after treatment with DOX-loaded Si-SS-CD-PEG at 37°C or 42°C. Cells were first heated at 42°C for 1 h with DOX-loaded Si-SS-CD-PEG, and then further incubated with the carrier at 37°C for 23 h. TUNEL-positive cells were detected under confocal microscopy. (B) Relative fluorescence intensities were quantified by pixel intensity using Image J software (NIH), and data was normalised to the fluorescence intensity of DAPI. Data are expressed as means of three independent experiments ± 1 SE. *P < 0.05, **P < 0.01.
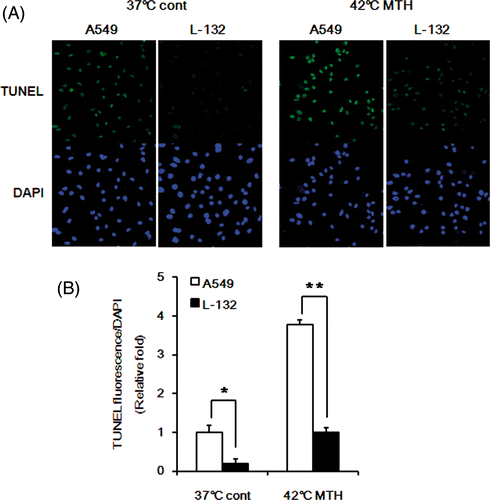
Discussion
In the present study we have confirmed that mesoporous silica nanocontainers bearing CD gatekeepers are potentially valuable for delivery of anti-cancer drugs to target cells. We have shown that heating significantly increases DOX release and DOX-mediated cell death.
Mesoporous silica nanoparticles are known to be excellent hosts of various molecules including anti-cancer drugs, indicating that nanoparticles are potentially valuable in cancer treatment. The major problem in the use of various nanosized drug delivery systems is controlled release of drugs at target sites. In our approach, we have taken advantage of the fact that GSH cleaves a disulphide linker that holds gatekeepers in the vicinity of pores and that cancer cells contain high concentrations of GSH. In the present study, consistent with the results of our previous report Citation[17], DOX was released from internalised Si-SS-CD-PEG () and caused clonogenic cell death () and apoptosis (). Importantly, heating at 42°C for 1 h enhanced the level of DOX release (), which in turn increased DOX-mediated clonogenic death () and apoptosis (). Although the heat-induced enhancement of DOX release from DOX-loaded Si-SS-CD-PEG was due mainly to the heat-induced increase in GSH level in the cells (), heat-induced increase in GSH-mediated cleavage of the disulphide stalk moiety may also play a role, albeit small, in the heat-induced enhancement of DOX release from DOX-loaded Si-SS-CD-PEG (). As shown in , the GSH level in L-132 cells was lower than that in A549 cells. Note that the release of DOX from DOX-loaded Si-SS-CD-PEG in L-132 cells was far less than that in A549 cells () demonstrating that the cellular GSH level plays a central role in the release of DOX from DOX-loaded Si-SS-CD-PEG. The results of the study with other human cancer cell lines () confirmed the above conclusion that the release of DOX from DOX-loaded Si-SS-CD-PEG is dependent on cellular GSH levels. The intracellular GSH content in different types of cells varies considerably Citation[23]; thus, the efficacy of Si-SS-CD-PEG as a drug carrier may vary depending on the types of cancer cells. However, it is probable that hyperthermic treatment may increase GSH content in the cells to a level adequate for cleavage of the disulphide linker, even in cancer cells with intrinsically low GSH levels Citation[24]. The mechanism for the heat-induced increase in GSH level is unclear, but it might be related to up-regulation of Hsp70 in heated cells. It has been demonstrated that Hsp70 increases the activity of glutathione reductase Citation[25], which is responsible for the conversion of oxidised glutathione (GSSG) to reduced glutathione (GSH). It would therefore be reasonable to suggest that the increase in GSH level by heating is due to an increase in glutathione reductase activity by virtue of heat-induced up-regulation of Hsp70.
It is well known that hyperthermia potentiates the cytotoxicity of various anti-cancer drugs both in vitro and in vivo. DOX is a quinone-containing anthracycline antibiotic used for the treatment of various types of cancers, and previous studies have shown that hyperthermia significantly potentiates the anti-cancer effect of DOX Citation[22], Citation[26]. Consistent with these previous reports, heating at 42°C for 1 h markedly increased the effect of free DOX to cause clonogenic death in A549 and L-132 cells in our study (). It is of note that L-132 cells were more sensitive than A549 cells to DOX and that the thermal sensitisation to DOX was also greater for L-132 cells than A549. On the contrary, at both 37°C and 42°C, DOX-loaded Si-SS-CD-PEG caused significantly more apoptosis in A549 cells than in L-132 cell (), which contained less GSH level than A549 cells (). These results provide further evidence that GSH plays a central role in the release of DOX from internalised DOX-loaded Si-SS-CD-PEG and the resultant DOX-induced cell death.
It is notable that heating at 42°C markedly increased the effect of DOX-loaded Si-SS-CD-PEG to cause cleavage of PARP, caspases 3, 8, and 9. Interestingly, the activation of these signals by DOX-loaded Si-SS-CD-PEG was negligible at 37°C. Whether the molecular pathways for the DOX-induced apoptosis at 42°C is different from that at 37°C or heating at 42°C merely enhanced the signal changes remain to be investigated.
Taken together, we concluded that hyperthermia enhances the efficacy of DOX-loaded Si-SS-CD-PEG to kill cancer cells by augmenting the GSH level in the cells, and thus enhancing the release of DOX from the carrier. In addition, heat-mediated sensitisation of cells to DOX may also play a role in the heat-induced increase in the clonogenic and apoptotic cell death by DOX-loaded Si-SS-CD-PEG.
Declaration of interest: This work was supported by grants from the Korea Health 21 R&D Project (A062254), the Nuclear R&D Program of KOSEF (2009-0093747) and the Korea Research Foundation (KRF) grant funded by the Korea government (MEST) (No. 2009-0079739). The authors alone are responsible for the content and writing of the paper.
References
- Liu R, Zhao X, Wu T, Feng P. Tunable redox-responsive hybrid nanogated ensembles. J Am Chem Soc 2008; 130: 1418–14419
- Park C, Oh K, Lee SC, Kim C. Controlled release of guest molecules from mesoporous silica particles based on a pH-responsive polypseudorotaxane motif. Angew Chem 2007; 46: 1455–1457
- Fu Q, Rao GV, Ward TL, Lu Y, Lopez GP. Thermoresponsive transport through ordered mesoporous silica/PNIPAAm copolymer membranes and microspheres. Langmuir 2007; 23: 170–174
- Park C, Kim H, Kim S, Kim C. Enzyme Responsive nanocontainers with cyclodextrin gatekeepers and synergistic effects in release of guests. J Am Chem Soc 2009; 131: 16614–16615
- Saha S, Leung KC-F, Nguyen TD, Stoddart J, Zink JI. Nanovalves. Adv Funct Mater 2007; 17: 685–693
- Trewyn BG, Slowing II, Giri S, Chen HT, Lin VS. Synthesis and functionalization of a mesoporous silica nanoparticle based on the sol-gel process and applications in controlled release. Acc Chem Res 2007; 40: 846–853
- Cotí KK, Belowich ME, Liong M, Ambrogio MW, Lau YA, Khatib HA, Zink JI, Khashab NM, Stoddart JF. Mechanised nanoparticles for drug delivery. Nanoscale 2009; 1: 16–39
- Nguyen TD, Liu Y, Saha S, Leung KC, Stoddart JF, Zink JI. Design and optimization of molecular nanovalves based on redox-switchable bistable rotaxanes. J Am Chem Soc 2007; 129: 626–634
- Giri S, Trewyn BG, Stellmaker MP, Lin VS. Stimuli-responsive controlled-release delivery system based on mesoporous silica nanorods capped with magnetic nanoparticles. Angew Chem 2005; 44: 5038–5044
- Vivero-Escoto JL, Slowing II, Wu CW, Lin VS. Photoinduced intracellular controlled release drug delivery in human cells by gold-capped mesoporous silica nanosphere. J Am Chem Soc 2009; 131: 3462–3463
- Angelos S, Yang YW, Patel K, Stoddart JF, Zink JI. pH-responsive supramolecular nanovalves based on cucurbit [6] uril pseudorotaxanes. Angew Chem Int Ed 2008; 47: 2222–2226
- E Climent E, Bernardos A, Martínez-Máñez R, Maquieira A, Marcos MD, Pastor-Navarro N, Puchades R, Sancenón F, Soto J, Amorós P. Controlled delivery systems using antibody-capped mesoporous nanocontainers. J Am Chem Soc 2009; 131: 14075–14080
- Radu DR, Lai CY, Jeftinija K, Rowe EW, Jeftinija S, Lin VS. A polyamidoamine dendrimer-capped mesoporous silica nanosphere-based gene transfection reagent. J Am Chem Soc 2004; 126: 13216–13217
- Casasús R, Climent E, Marcos MD, Martínez-Mañez R, Sancenón F, Soto J, Amorós P, Cano J, Ruiz E. Dual aperture control on pH- and anion-driven supramolecular nanoscopic hybrid gate-like ensembles. J Am Chem Soc 2008; 130: 1903–1917
- Casasús R, Aznar E, Marcos MD, Martínez-Máñez R, Sancenón F, Soto J, Amorós P. New methods for anion recognition and signaling using nanoscopic gatelike scaffoldings. Angew Chem Int Ed 2006; 45: 6661–6664
- Park C, Lee K, Kim C. Photoresponsive cyclodextrin-covered nanocontainers and their sol-gel transition induced by molecular recognition. Angew Chem Int Ed 2009; 48: 1275–1278
- Kim H, Kim S, Park C, Lee H, Park HJ, Kim C. Glutathione-induced intracellular release of guests from mesoporous silica nanocontainers with cyclodextrin gatekeepers. Adv Mater 2010; 22: 4280–4283
- Li GC, Evans RG, Hahn GM. Modification and inhibition of repair of potentially lethal X-ray damage by hyperthermia. Radiat Res 1976; 67: 491–501
- Mills MD, Meyn RE. Hyperthermic potentiation of unrejoined DNA strand breaks following irradiation. Radiat Res 1983; 95: 327–338
- Herman TS. Effect of temperature on the cytotoxicity of vindesine, amsacrine, and mitoxantrone. Cancer Treat Rep 1983; 67: 1019–1022
- Herman TS. Temperature dependence of adriamycin, cis-diamminedichloroplatinum, bleomycin, and 1,3-bis(2-chloroethyl)-1-nitrosourea cytotoxicity in vitro. Cancer Res 1983; 43: 517–520
- Yarmolenko PS, Zhao Y, Landon C, Spasojevic I, Yuan F, Needham D, Viglianti BL, Dewhirst MW. Comparative effects of thermosensitive doxorubicin-containing liposomes and hyperthermia in human and murine tumours. Int J Hyperthermia 2010; 26: 485–498
- Park C, Youn H, Kim H, Noh T, Kook YH, Oh ET, Park HJ, Kim C. Cyclodextrin-covered gold nanoparticles for targeted delivery of an anti-cancer drug. J Mater Chem 2009; 19: 2310–2315
- Mirtsch S, Strohmenger U, Streffer C. Glutathione level in melanoma cells and tissue. Rec Results Cancer Res 1988; 107: 22–26
- Guo S, Wharton W, Moseley P, Shi H. Heat shock protein 70 regulates cellular redox status by modulating glutathione-related enzyme activities. Cell Stress Chaperones 2007; 12: 245–254
- Shen J, Zhang W, Wu J, Zhu Y. The synergistic reversal effect of multidrug resistance by quercetin and hyperthermia in doxorubicin-resistant human myelogenous leukaemia cells. Int J Hyperthermia 2008; 24: 151–159