Abstract
Environmental stress induces damage that activates an adaptive response in any organism. The cellular stress response is based on the induction of cytoprotective proteins, the so-called stress or heat shock proteins (HSPs). HSPs are known to function as molecular chaperones which are involved in the therapeutic approach of many diseases. Therefore in the current study we searched nontoxic chaperone inducers in chemical compounds isolated from medicinal plants. Screening of 80 compounds for their Hsp70-inducing activity in human lymphoma U937 cells was performed by western blotting. Five compounds showed significant Hsp70 up-regulation among them shikonin was most potent. Shikonin was able to induce Hsp70 at 0.1 µM after 3 h without activation of heat shock transcription factor 1 (HSF-1). It also induces significant reactive oxygen species generation. The expression level of genes responsive to shikonin was studied using global-scale microarrays and computational gene expression analysis tools. Significant increase in the nuclear factor erythroid 2-related factor 2 (Nrf2, NFEL2L2) -mediated oxidative stress response was observed that leads to the activation of HSP. The results of gene chip analysis were further confirmed by real-time qPCR assay. In short, the detailed mechanisms of Hsp70 induction by shikonin is not fully understood, Nrf2 and its target genes might be involved in the Hsp70 up-regulation in U937 cells.
Introduction
Human exposure to environmental toxicants has been associated with etiology of many diseases including inflammation, cancer, cardiovascular and neurodegenerative disorders. To counteract the detrimental effects of environmental insults, mammalian cells have evolved a hierarchy of sophisticated sensing and signalling mechanisms to turn on or off endogenous antioxidant responses accordingly Citation1. The ability of cells to counteract stressful conditions, known as cellular adaptive response, requires the activation of pro-survival pathways and the production of molecules with antioxidant, antiapoptotic and proapoptotic activities Citation2. Among the cellular pathways conferring protection against oxidative stress, a key role is played by vitagenes, which include heat shock proteins (HSPs) such as heme oxygenase-1 (HMOX1) and Hsp70, as well as thioredoxin/thioredoxin reductase system Citation3. Heat shock or stress response is a cellular adaptive response, which contributes to establishing a cytoprotective state in a wide variety of human diseases. When appropriately activated, cellular stress response has the capability to restore cellular homeostasis and rebalance redox equilibrium Citation4.
Among the many changes in cellular activity and physiology, the most remarkable event in stressed cells is the production of a highly conserved set of proteins, the heat shock or stress proteins (HSPs) Citation5. HSPs are also expressed constitutively at normal growth temperatures and play an essential role as molecular chaperones by assisting the correct folding of nascent and stressed accumulated misfolded proteins, preventing their aggregation Citation6, assembly/disassembly of multi-subunit oligomers, translocation of proteins across intracellular membranes, process of endocytosis, regulation of apoptosis and cytoskeletal organisation Citation7. A number of studies have reported that molecular chaperones can confer cellular and tissue stress tolerance and provide beneficial effects on various pathological states, such as stress ulcers and ischaemia-induced injuries, as well as on diseases associated with protein misfolding and aggregation Citation8–10.
Given the broad cytoprotective properties of the heat shock response, there is now strong interest in discovering and developing pharmacological agents capable of inducing stress responses. Therefore, in the current study we screened some phyto-medicinal compounds for their HSP-inducing activity.
Materials and methods
Chemical compounds
Chemical compounds used in this study were obtained from the Institute of Natural Medicine, University of Toyama, Japan.
Cell culture
A human lymphoma cell line, U937 was obtained from the Human Sciences Research Resource Bank (Japan Human Sciences Foundation, Tokyo, Japan). The cells were maintained in Roswell Park Memorial Intitute (RPMI) 1640 medium (Wako Pure Chemicals Ltd., Osaka, Japan) with 10% heat-inactivated fetal bovine serum (FBS) (JRH Biosciences Lenexa, KS, USA) and incubated in a CO2 incubator with 5% CO2 and 95% air at 37°C.
Hyperthermia treatment
Hyperthermia (HT) treatment was used as a positive control for induction of HSPs. Cells were collected and suspended in 2 mL fresh medium in plastic culture tubes, and were exposed to 44°C (±0.05°C) for 15 min in a water bath (NTT-1200, Eyela, Tokyo, Japan). After HT treatment, cells were incubated for desired time at 37°C in humidified air with 5% CO2.
Assessment of apoptosis
For the detection of early apoptosis and secondary necrosis, Annexin V-FITC kit, purchased from Immunotech (Marseille, France), was utilised according to the manufacturer's recommendations. Briefly, cells were stained simultaneously with propidium iodide (PI) and fluorescein isothiocyanate (FITC)-labelled annexin V and assessed with a flow cytometer (Beckman-Coulter EPICS XL™, Beckman Coulter, Miami, FL).
Determination of cell survival by WST-8 assay
Cell survival was determined using a Cell Counting Kit-8 (Wako Pure Chemicals Ltd.). The cells (2 × 104 /well) in 96-well plates were incubated with various concentrations of shikonin for 6 h. After incubation, WST-8 reagents were added to each well. Absorbance at 450 nm was measured using a microplate reader (BioRad, Hercules, CA) after 2 h of incubation with WST-8 reagents. Absorbance is proportionally related to the number of live cells.
Western blot analyses for proteins
Western blot analysis was performed for Hsp70, HSF-1, NRF2 and β-actin by using specific polyclonal or monoclonal antibodies (Santa Cruz Biotechnology, Santa Cruz, CA) as described in previous papers Citation11, Citation12. For the detection of these specific antibodies the chemiluminescence ECL detection reagents were used following the manufacturer's instructions (Amersham Biosciences, Piscataway, NJ).
RNA isolation
Total RNA was extracted from cells using an RNAeasy Total RNA Extraction kit (Qiagen, Valencia, CA) and treated with Dnase I (RNase-free Dnase kit, Qiagen) for 15 min at room temperature to remove genomic DNA.
Assessment of intracellular reactive oxygen species (ROS)
To measure intracellular superoxide () we used 2 µM hydroethidine (HE) (Molecular Probes, Eugene, OR) a dye that is oxidized within the cell and fluoresces when it intercalates into DNA. To measure intracellular peroxides including H2O2 5 µM dichlorofluorescein diacetate (DCFH-DA) (Molecular Probes) was utilised. DCFH-DA upon entering into the cells is de-esterified and then oxidized to dichlorofluorescein (DCF). The fluorescence emission was analysed by flow cytometry Citation13.
High-density oligonucleotide microarray and computational gene expression analysis
Gene expression was analysed using a GeneChip® system with a Human Genome U133-plus 2.0 array (Affymetrix, Santa Clara, CA) spotted with 54,675 probe sets, more than twice the molecular probes used in our previous studies Citation14, Citation15. Samples for array hybridisation were prepared as described in the Affymetrix GeneChip® Expression Technical Manual. Briefly, 5 µg of total RNA was used to synthesise double-stranded cDNA with a GeneChip® Expression 30-Amplification Reagents One-Cycle cDNA Synthesis kit (Affymetrix). Biotin-labelled cRNA was then synthesised from the cDNA using GeneChip® Expression 3′-Amplification Reagents for IVT labelling (Affymetrix). After fragmentation, the biotinylated cRNA was hybridised to the GeneChip array at 45°C for 16 h. The arrays were washed, stained with streptavidin-phycoerythrin, and scanned using a probe array scanner. The scanned chip was analysed using GeneChip Analysis Suite Software (Affymetrix). The obtained hybridisation intensity data were converted into a presence or an absence call for each gene, and changes in gene expression level between experiments were detected by comparative analysis. The data were further analysed using Gene-Spring software (Silicon Genetics, Redwood City, CA) to extract the significant genes Citation16, Citation17. To examine gene ontology, including biological processes, cellular components, molecular functions, and genetic networks, the obtained data were analysed using Ingenuity Pathways Analysis tools (Ingenuity Systems, Mountain View, CA), a web-delivered application that enables the identification, visualisation and exploration of molecular interaction networks in gene expression data. The gene lists identified by GeneSpring containing Affymetrix gene ID and the natural logarithm of normalised expression signal ratios from GeneChip CEL files were uploaded into the Ingenuity Pathways Analysis system. Each gene identifier was mapped to its corresponding gene object in the Ingenuity Pathways Knowledge Base. These so-called focus genes were then used as a starting point for generating biological networks Citation16, Citation17.
Real-time quantitative PCR assay
Real-time quantitative PCR (qPCR) assay was performed on a real-time PCR system (Mx3000P, Stratagene, Tokyo, Japan) using SYBR PreMix ExTaq (Takara Bio, Shiga, Japan) or Premix ExTaq (for the use of TaqMan probes; Takara Bio) in accordance with the manufacturer's protocols. Reverse transcriptase reaction (Omniscript Reverse Transcriptase, Qiagen) was carried out with DNase-treated total RNA using an oligo (dT) 16 primer. Real-time qPCR assay was performed using the specific primers listed in , as previously described (Ref. 14-17). Specific primers for human NQO1 were designed using Primer-BLAST and the sequences were as follow: 5′-TGGACCGAGCTGGAAAACCTCCT-3′ and 5′-AACTGAAACACCCAGCCGTCAGC-3′. Each mRNA expression level was normalized to the mRNA expression level of GAPDH.
Table I. List of 80 chemical compounds that were examined for their Hsp70 inducing ability.
Results
Screening of medicinal compounds for Hsp70 up-regulation
Eighty medicinal compounds shown in were examined for their ability to induce Hsp70 up-regulation. The screening procedure was accomplished by treating U937 cells with the compounds 1 to 10 at 0.1 mM for 24 h followed by western blot analysis. Among the tested samples no band of Hsp70 was observed with compounds 3–5 suggesting the toxicity of the dose (data not shown). Therefore, we selected compounds 3–5 and did concentration-dependent screening for Hsp70 up-regulation. U937 cells were treated with 0.1 µM, 1 µM and 10 µM for 24 h followed by western blot analysis. Significant Hsp70 up-regulation was observed with compound 5 at 1 µM (densitometric ratio: control: 1.0 ± 0.08, 0.1 µM: 1.25 ± 0.12, 1 µM: 1.50 ± 0.14, 10 µM: 1.12 ± 0.13, mean ± SD, n = 3) while compounds 3 and 4 did not show any significant increase at all concentrations as compared to control. From these results we selected 1 µM concentration for the screening of medicinal compounds.
Out of the 80 compounds, five (5, 63, 64, 67 and 76) showed significant Hsp70 up-regulation. Among them compound 76 (shikonin) was the most potent (, 1B).
Effects of shikonin on apoptosis induction in U937 cells
To determine the non-toxic concentration of shikonin, cells were exposed to the drug concentration-dependently for 6 h. This was followed by measurement of early apoptosis and secondary necrosis by annexin V FITC/PI staining using flow cytometry. No apoptosis was observed at 0.01 µM and 0.1 µM, significant apoptosis was observed at 1 µM concentration ().
Figure 2. (A) U937 cells were treated with shikonin concentration-dependently for 6 h followed by assessment of early apoptosis (black bar) and secondary necrosis (grey bar) by flow cytometry. Bars indicate standard deviation (n = 3). (B) Cell survival assay was performed with 0.01, 0.1 and 1 µM concentrations of shikonin. After 6 h incubation WST-8 reagent was added to each well. Bars indicate standard deviation (n = 3).
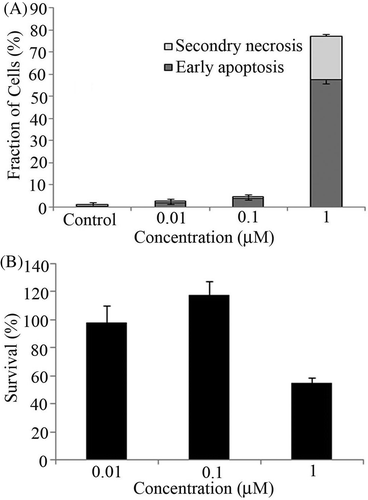
Effects of shikonin on cell survival
To further confirm the non-toxic concentration, cell survival assay was performed with 0.01, 0.1 and 1 µM concentrations after 6 h incubation. The results also showed the toxicity at 1 µM while no toxicity was observed with 0.01 and 0.1 µM concentrations. ().
Effects of shikonin on ROS formation
Previously it has been reported that shikonin can induce ROS formation Citation18. Therefore, in the current study we investigated that whether at non-toxic concentration shikonin can induce ROS. Cells were exposed to 0.1 µM shikonin and the levels of DCF and HE fluorescence were monitored time-dependently via flow cytometry. Intracellular peroxides level was increased as early as 30 min after treatment (), while no intracellular was observed (data not shown).
Figure 3. (A) U937 cells were first incubated with DCFH-DA for 30 min and then treated with shikonin in a time-dependent manner. Intracellular peroxides level was measured by flow cytometry. (B) Concentration and time-dependent induction of Hsp70 was measured by western blotting. Hyperthermia (HT) 44°C for 15 min was used as a positive control. (C) Bands were quantified by densitometry and normalised with β-actin. Bars indicate standard deviation (n = 3). (D) HSF1 phosphorylation was measured time-dependently by western blotting. Hyperthermia (HT) 44°C for 15 min was used as a positive control.
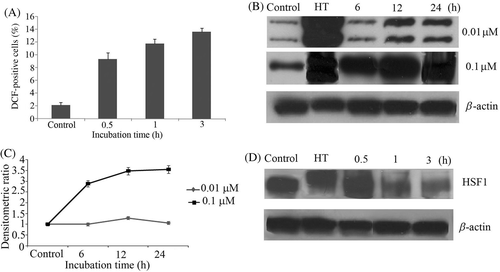
Time-dependent effects of shikonin on Hsp70 induction
U937 cells were treated with 0.01 µM and 0.1 µM shikonin time-dependently, followed by western blot analysis. Up-regulation of Hsp70 was observed with 0.1 µM after 3 h incubation and continued to increase time-dependently, while no up-regulation of Hsp70 was observed at 0.01 µM shikonin (, 3C)
The transcription of HSP genes is regulated by transcription factor HSF, which senses cellular exposure to stress and turns on rapid induction of HSPs Citation19. Therefore we examined the effects of shikonin on the activation of HSF1. U937 cells were treated with 0.1 µM shikonin in a time-dependent manner followed by western blot analysis.
Phosphorylation of HSF1 is usually detected as an upward band shift Citation20. No upward band shift was observed after shikonin treatment till 3 h, while very clear upward shift was observed in HT-treated cells. This result indicates that HSF1 is not playing a role in shikonin-induced HSP up-regulation ().
Identification of genes responsive to shikonin treatment
As shikonin treatment did not show the activation of HSF1, we carried out gene chip analysis of cells treated with or without shikonin after 3 h incubation. Many probe sets were differentially expressed by >2-fold in cells treated with the compound, 277 up-regulated and 262 down-regulated in comparison to control. The complete list of genes from all samples is available on the Gene Expression Omnibus (http://www.ncbi.nlm.nih.gov/geo/query/acc.cgi?acc=GSE24743). The biologically relevant functions and networks of the up-regulated probe sets obtained from the gene chip analysis were identified using Ingenuity Pathways Knowledge Base. Of 277 up-regulated probe sets analysed, 77 genes were functionally annotated. On the basis of significance, the top three molecular functions were cellular compromise (p value: 3.73E-12 to 2.68E-02), cellular function and maintenance (3.73E-12 to 4.65E-02), and post-transcriptional modification (1.36E-7 to 3.34E-02). As shown in , a significant gene network contained HSP-related genes such as HSPA1A (heat shock 70 kDa protein 1A), HSPA6 (heat shock 70 kDa protein 6) and DNAJA1 (DnaJ (Hsp40) homologue, subfamily A, member 1) and Nrf2(NFE2L2)-target genes such as HMOX1 (heme oxygenase (decycling) 1), NQO1 (NAD(P)H dehydrogenase, quinone 1) and SQSTM1 (sequestosome 1). To confirm the results of gene chip analysis, a real-time qPCR assay was performed for four selected genes in the network. As we expected, the expression levels of these genes were significantly increased by the treatment ().
Figure 4. A gene network including up-regulated genes. Cells were treated with the compound and cultured at 37°C for 3 h. Gene chip analysis was performed. Genes that were up-regulated were analysed using Ingenuity Pathways Analysis tools. The network is displayed graphically as nodes (genes or protein group) and edges (the biological relationships between the nodes). The node colour of genes indicated the expression level of genes. Nodes and edges are displayed in various shapes and labels that present the functional class of genes and the nature of the relationship between the nodes, respectively.
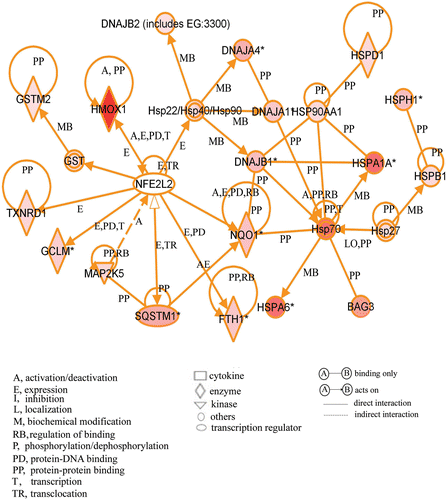
Figure 5. Verification of microarray results by qPCR assay. Cells were treated with 0.1 µM shikonin time-dependently and then real-time qPCR assay was performed. (A) HMOX1 (heme oxygenase (decycling) 1) (B) NQO1 (NAD(P)H dehydrogenase, quinone 1) (C) HSPA6 (heat shock 70 kDa protein 6) (D) DNAJA1(DnaJ (Hsp40) homologue, subfamily A, member 1). Each mRNA expression level was normalised with GADPH. Data are presented as mean ± SD (n = 3).
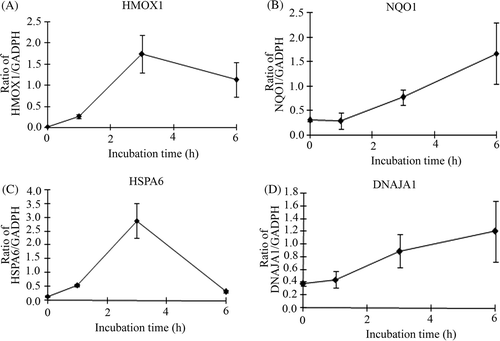
Discussion
Toward the goal of developing novel cytoprotective agents we did screening of 80 chemical compounds isolated from medicinal plants for their HSP-inducing activities. Among the five active Hsp70 inducers, shikonin was most potent. Shikonin is a chemical compound isolated from the root of a plant Lithospermum erythrorhizon. It is known to have antibacterial Citation21, antifungal Citation21, anti-human immunodeficiency virus Citation22, anticancer Citation23 and anti-inflammatory activity Citation23. But to our knowledge there is no report on its ability to induce HSP up-regulation.
Several chemical compounds are known to induce HSP through the activation of HSF1 Citation11. In this study, after shikonin treatment significant Hsp70 up-regulation was observed without the activation of HSF1. With the high-density oligonucleotide microarrays and computational gene expression tools we identified a unique gene network containing HSPs and Nrf2-target genes. Nrf2 is an antioxidant transcription master regulator and belongs to the cap ‘n’ collar family of transcription factors. Small Maf proteins (MafF, MafG and MafK) possess the region-leucine zipper (L-Zip) domain that is required for homodimer or heterodimer complex formation with other basic L-Zip transcription factors such as Nrf2 Citation24. Nrf2 is sequestered in cytoplasm by its repressor Keap1, released and translocated into the nucleus under oxidative stress Citation25. In the nucleus, the heterodimer complex of Nrf2 and small Maf proteins bind to the antioxidant-responsive element (ARE) sequence leading to transcriptional activation of downstream genes encoding phase II detoxifying enzymes, antioxidants Citation26 and chaperone proteins Citation27.
Almeida et al. Citation28 recently indicated that Hsp70 expression is regulated by an ARE/EpRE sequence in a Zebrafish model. In many studies, Nrf2 have been reported to induce different Hsps Citation27, Citation29–31 and in one study, Hsp70 was specifically up-regulated in mouse liver in wild-type but not knockout mice Citation29. Furthermore, it has also been reported that activation of Nrf2 increases expression of Hsp40 Citation27. In addition, several studies reported a concomitant induction of Hsp70 and Nrf2-regulated gene HMOX1 by electrophiles Citation32.
Although the detailed mechanisms by which shikonin induces protein expression of Hsp70 are not fully understood, Nrf2 and its target genes may have participated in the up-regulation of Hsp70 in U937 cells. The elucidation of the molecular mechanism to induce Hsp70 by shikonin remains for further investigation in the future.
Declaration of interest: This work was supported by the Expansion Program, Regional Innovation Cluster Program, Global Type (II) from the Ministry of Education, Culture, Sports, Science and Technology, Japan, and by research grants from the Smoking Research Foundation (TK, TY) and the International Association of Sensitization for Cancer Treatment (IASCT)(KA). The authors alone are responsible for the content and writing of the paper.
References
- Giudice A, Arra C, Turco MC. Review of molecular mechanisms involved in the activation of the Nrf2-ARE signaling pathway by chemopreventive agents. Methods Mol Biol 2010; 647: 37–74
- Calabrese V, Mancuso C, Sapienza M, Puleo E, Calafato S, Cornelius C, et al. Oxidative stress and cellular stress response in diabetic nephropathy. Cell Stress Chaperones 2007; 12: 299–306
- Calabrese V, Mancuso C, Ravagan A, Perluigi M, Cini C, De Macro C, et al. Oxidative stress redox hemostasis and cellular stress response in Ménière's disease: Role of vitagenes. J Neurochem 2007; 10: 709–717
- Calabrese V, Cornelius C, Maiolino L, Luca M, Chiaramonte R, Toscano MA, Serra A. Neurochem Res 2010; 35: 2208–2217
- Schlesinger MJ, Ashburner M, Tissieres A. Heat Shock from Bacteria to Man. Cold Spring Harbor, New York 1982
- Lanneau D, Brunet M, Frisan E, Solary E, Fontenay M, Garrido C. Heat shock proteins: Essential proteins for apoptosis regulation. J Cell Mol Med 2008; 12: 743–761
- Ohtsuka K, Kawashima D, Asai M. Dual functions of heat shock proteins: Molecular chaperones inside of cells and danger signals outside of cells. Thermal Med 2007; 23: 11–22
- Otani S, Otaka M, Jin M, Okuyama A, Itoh S, Iwabuchi A, et al. Effect of preincubation of heat shock proteins on acetic acid-induced colitis in rats. Dig Dis Sci 1997; 42: 833–846
- Rokutan K, Hirakawa T, Teshima S, Nakano Y, Miyoshi M, Kawai T, et al. Implications of heat shock/stress proteins for medicine and disease. J Med Invest 1998; 44: 137–147
- Kobayashi Y, Kume A, Li M, Doyu M, Hata M, Ohtsuka K, et al. Chaperones Hsp70 and Hsp40 suppress aggregate formation and apoptosis in cultured neuronal cells expressing truncated androgen receptor protein with expended polyglutamine tract. J Biol Chem 2000; 275: 8772–8778
- Yan D, Saito K, Ohmi Y, Fujie N, Ohtsuka K. Paeoniflorin, a novel heat shock protein-inducing compound. Cell Stress Chaperones 2004; 9: 378–389
- Genc K, Egrilmez YM, Genc S. Erythropoietin induces nuclear translocation of Nrf2 and heme oxygenase-1 expression in SH-SY5Y cells. Cell Biochem Funct 2010; 28: 197–201
- Ahmed K, Matsuya Y, Nemoto H, Zaidi SF, Sugiyama T, Yoshihisa Y, et al. Mechanism of apoptosis induced by a newly synthesized derivative of macrosphelides with a thiazole side chain. Chem Biol Interact 2008; 117: 218–226
- Tabuchi Y, Takasaki I, Zhao QL, Wada S, Hori T, Feril LB, et al. Genetic networks responsive to low-intensity pulsed ultrasound in human lymphoma U937 cells. Cancer Lett 2008; 270: 286–294
- Hori T, Kondo T, Tabuchi Y, Takasaki I, Zhao QL, Kanamori M, et al. Molecular mechanism of apoptosis and gene expressions in human lymphoma U937 cells treated with anisomycin. Chem Biol Interact 2007; 172: 125–140
- Tabuchi Y, Takasaki I, Doi T, Ishii Y, Sakai H, Kondo T. Genetic networks responsive to sodium butyrate in colonic epithelial cells. FEBS Lett 2006; 580: 3035–3041
- Salunga TL, Tabuchi Y, Takasaki I, Feril LB, Jr, Zhao QL, Ohtsuka K, et al. Identification of genes responsive to paeoniflorin, a heat shock protein-inducing compound, in human leukaemia U937 cells. Int J Hyperthermia 2007; 23: 529–537
- Mao X, Yu CR, Li WH, Li WX. Induction of apoptosis by shikonin through a ROS/JNK-mediated process in Bcr/Abl-positive chronic myelogenous leukemia (CML) cells. Cell Res 2008; 18: 879–88
- Calderwood SK. Heat shock proteins in breast cancer progression – A suitable case for treatment?. Int J Hyperthermia 2010; 26: 681–685
- Sarge KD, Murphy SP, Morimoto RI. Activation of heat shock gene transcription by heat shock factor 1 involves oligomerization, acquistion of DNA binding activity, and nuclear localization and can occur in the absence of stress. Mol Cell Biol 1993; 13: 1392–1407
- Brigham LA, Michaels PJ, Flores HE. Cell-specific production and antimicrobial activity of naphthoquinones in roots of Lithospermum erythrorhizon. Plant Physiol 1999; 119: 417–428
- Chen X, Yang L, Zhang N, Turpin JA, Buckheit RW, Osterling C, et al. Shikonin, a component of Chinese herbal medicine, inhibits chemokine receptor function and suppresses human immunodeficiency virus type 1. Antimicrob Agents Chemother 2003; 47: 2810–2816
- Chen X, Yang L, Oppenheim JJ, Howard MZ. Cellular pharmacological study of shikonin derivatives. Phytother Res 2002; 16: 199–209
- Motohashi H, O’Connor T, Katsuoka F, Engel JD, Yamamoto M. Integration and diversity of the regulatory network composed of Maf and CNC families of transcription factors. Gene 2002; 294: 1–12
- Itoh K, Chiba T, Takahashi S, Ishii T, Igarashi K, Katoh Y, et al. An Nrf2/small Maf heterodimer mediates the induction of phase II detoxifying enzyme genes through antioxidant response elements. Biochem Biophys Res Commun 1997; 236: 313–322
- Itoh K, Wakabayashi N, Katoh Y, Ishii T, Igarashi K, Engel JD, et al. Keap1 represses nuclear activation of antioxidant responsive elements by Nrf2 through binding to the amino-terminal Nef2 domain. Genes Dev 1999; 13: 76–86
- Kwak MK, Wakabayashi N, Itoh K, Motohashi H, Yamamoto M, Kensler TW. Modulation of gene expression by cancer chemopreventive dithiolethiones through Keap1-Nrf2 pathway. J Biol Chem 2003; 278: 8135–8145
- Almeida DV, Nornberg BF, Geracitano LA, Barros DM, Monserrat JM, Marins LF. Induction of phase II enzymes and hsp70 genes by copper sulfate through the electrophile-responsive element (EpRE): Insights obtained from a transgenic zebrafish model carrying an orthologous EpRE sequence of mammalian origin. Fish Physiol Biochem 2009; 36: 347–353
- Hu R, Xu C, Shen G, Jain MR, Khor TO, Gopalkrishnan A, et al. Identification of Nrf2-regulated genes induced by chemopreventive isothiocyanate PEITC by oligonucleotide microarray. Life Sci 2006; 79: 1944–1955
- Nair S, Li W, Kong AN. Natural dietary anti-cancer chemopreventive compounds: Redox-mediated differential signaling mechanisms in cytoprotection of normal cells versus cytotoxicity in tumor cells. Acta Pharmacol Sin 2007; 28: 459–472
- Thimmulappa RK, Mai KH, Srisuma S, Kensler TW, Yamamoto M, Biswal S. Identification of Nrf2-regulated genes induced by the chemopreventive agent sulforaphane by oligonucleotide microarray. Cancer Res 2002; 62: 5196–5203
- Thompson CA, Burcham PC. Genome-wide transcriptional responses to acrolein. Chem Res Toxicol 2008; 12: 2245–2256