Abstract
Purpose: To investigate whether mild heat stress at 39.5°C altered Dicer protein and miRNA expression patterns in several cell types.
Methods: Multiple human and mouse cell types were cultured during the course of 9 h at temperatures from 37°C to 39.5°C. Dicer mRNA levels and microRNAs were quantified by TaqMan RT-qPCR assays and Dicer protein by western blotting.
Results: Dicer protein was substantially elevated on western analysis in response to heat stress at 39.5°C in the absence of significant changes in Dicer mRNA by RT-qPCR.
Conclusions: Heat-induced regulation of Dicer expression occurs primarily post- transcriptionally, and the expression levels of Dicer protein are increased and often oscillate in response to fever-range hyperthermia in multiple mouse and human cells. Our studies suggest a potential role for Dicer and microRNAs in the response to mild thermal stress. Additional studies on the mechanisms involved in the stress-induced oscillations of Dicer protein and microRNAs will be of interest.
Introduction
The functionality of a protein is dependent on its ability to fold into a native state. While the appropriate tertiary structure of a given peptide chain is difficult to predict mathematically, it is known that maintaining proper conformation is dependent on the intracellular environment Citation[1], Citation[2]. For example, an increase in temperature, even if small, can denature proteins causing a loss of activity and/or solubility Citation[3]. To maintain homeostasis during periods of thermal stress, the cell undergoes a proteomic paradigm shift known as the heat shock response (HSR) Citation[3–9]. The HSR is classically reported at 42°C but altered heat shock proteins can be observed at lower temperatures, including those achieved during fever range hyperthermia at 39.5°C. The molecular mechanism regulating heat shock and its physiological consequences originally studied in Drosophila was recently introduced into clinical practice Citation[10], Citation[11]. The cellular response to hyperthermia is now known to be regulated at multiple levels Citation[12] although the role of the microRNA (miRNA, miR) system in hyperthermia remains uncertain. More specifically, the link between the function of the RNA endoribonuclease III Dicer shown to respond to several stresses and the cellular responses to mild hyperthermic stress has not been explored.
The HSR is a highly coordinated set of events that enhances cellular survival in a hot environment Citation[13–15]. The HSR is triggered when the added kinetic energy causes cellular proteins to deviate from their native tertiary structure Citation[3]. Protein unfolding, if left unchecked, leads to a loss of protein solubility, functionality and cell death. To protect cells, misfolded proteins are shuttled by HSPs to thermodynamically more stable cellular compartments while targeting irreparably damaged peptide chains for destruction Citation[2], Citation[9], Citation[14–16]. The role played by HSPs is primarily cytoprotective, and this can provide cancer cells a survival advantage, thereby making HSPs attractive therapeutic targets Citation[17]. The activation of HSPs results in the release of transcription factor HSFl, which binds to heat responsive promoter elements (HREs) and induces transcription of multiple heat shock proteins Citation[18]. After translocation to the nucleus, HSFs alter the transcription of additional HSPs, enhancing the cell's capacity to chaperone unfolded proteins Citation[4].
The HSR is also characterised by a sharp decline in the synthesis of non-HSPs, and although the mechanisms of this inhibition are not yet completely understood, heat stress has been shown to lead to cap-dependent translational inhibition Citation[8], Citation[19], Citation[20]. This effect is attributed to the loss of functionality of crucial eukaryotic initiation factors Citation[21]. The Hsp27 chaperone has also been shown to bind to the eukaryotic translation initiation factor 4G (eIF4G) thereby sequestering a protein vital to the translation of multiple genes Citation[21]. However, recent research suggests that Hsp27-eIF4G binding may not be sufficient to halt cap-dependent translation to the degree observed in heat-shocked cells, and therefore additional factors likely contribute to the observed decrease in protein synthesis Citation[8]. At the transcriptional level, HSFl is thought to have the potential to coordinate an extensive chromatin deacetylation event Citation[22], which alters gene methylation and re-prioritises expression of a large segment of the genome.
Several microRNAs have been shown to be thermally responsive Citation[23], Citation[24], suggesting that protein regulation may also be mediated post-transcriptionally by microRNAs. This is an important issue since there is evidence that hyperthermia may have a therapeutic benefit in cancer, especially if combined with chemotherapy and/or radiation Citation[25]. In future studies of hyperthermia in cancer, analysis of the role of microRNAs will be important. Hyperthermic microRNAs may be mediators of some of the biological and/or therapeutic properties of hyperthermia. This remains an important area for future research, especially since anti-microRNAs are becoming available for specific neutralisation of microRNA targets in therapeutic protocols Citation[26].
Previously, we showed that Dicer could be regulated by cellular stresses and interferons as well as by modifications of genomic acetylation Citation[27], factors known to contribute to the HSR Citation[22], Citation[28]. Moreover, enzymes downstream of Dicer, such as Argonaute 2 and other components of the RNA-induced silencing complex (RISC) were recently shown to be responsive to thermal stress Citation[29] and dependent on the heat shock 70/90 machinery for their function Citation[30]. In this report we show that Dicer, the RNase III endoribonuclease responsible for the production of microRNAs from RNA precursors, is also regulated during mild thermal stress, further implicating the Dicer/microRNA system in the HSR. Additionally, we observed that under thermal stress at 39.5°C, the Dicer protein expression levels (but not Dicer mRNA) may oscillate during the initial 9 h of heating.
Materials and methods
Cell culture
Human cervical carcinoma HeLa, lung fibroblast MRC-5, placental choriocarcinoma JAR, colorectal carcinoma HCT116, adrenal carcinoma SW13, breast carcinoma BT-20, and Burkett's lymphoma Raji, as well as murine melanoma B16 were purchased from ATCC (Manassas, VA, USA). Human cell line 2fTGH was a gift from George R. Stark (Lerner Research Institute, Cleveland, OH, USA) Citation[31] and murine trophoblast SM-9 was a gift from Joan Hunt (KU Medical Center, Kansas City, KS, USA) Citation[32]. Mouse kidney cells were dissociated physically and enzymatically from freshly excised kidneys of C57BL/6 J mice. HeLa and MRC-5 cells were cultured in 100 mm2 plates in water-jacketed 37°C, 7% CO2 incubators, all other cells were cultured in 75 cm2 vented-cap flasks at 37°C and 5% CO2. Cells were cultured in DMEM (HCT116, SW13, 2fTGH), MEM (HeLa, MRC-5, BT-20), or RPMI 1640 (JAR, Raji, SM-9, Bl6) and supplemented with 10% heat-inactivated FBS from Atlanta Biologicals (Atlanta, GA, USA). HeLa, MRC-5, JAR, Raji, SM-9 and Bl6 cells were supplemented with sodium pyruvate; non-essential amino acids (NEAA) were added to HeLa, MRC-5 and BT-20 media, and HEPES was used in JAR and Raji media. SM-9 cells were supplemented with 2-mercaptoethanol, 2fTGH with 250 ug/mL hygromycin B, and JAR cells with 4.5 g/L sterile glucose solution. Primary mouse cells were cultured in RPMI 1640 at 5% C02 with sodium pyruvate, NEAA, HEPES, 2- mercaptoethanol, 4 mg/L human recombinant insulin, and an antibiotic/antimycotic solution. Cells were selectively enriched by adherence characteristics into predominantly fibroblast and epithelial cultures Citation[33]. Unless noted, all media and additives were purchased from Invitrogen (Carlsbad, CA, USA).
Heat treatment
Heat treatments were applied by transferring cell culture vessels from a 37°C incubator to an incubator held at 39.5°C for up to 9 h. All time points had equal time of growth and all samples for a particular experiment were harvested together at the experimental end point.
Western blotting
Pelleted cells were lysed with RIPA lysis buffer (Sigma, St Louis, MO, USA) supplemented with a protease inhibitor cocktail (Sigma), HALT phosphatase inhibitor cocktail (Pierce, Rockford, IL, USA), and l mmol dithiothreitol (DTT). SDS sample buffer, supplemented with 0.13 mol DTT (Sigma), was added to protein aliquots of 40 µg (for Dicer), 20 µg (for Hsp90), or 10 µg (for glyceraldehyde 3-phosphate dehydrogenase (GAPDH), Hsp70) and separated on 7% (Dicer), 10% (Hsp90) or 12% (GAPDH, Hsp70) SDS-PAGE gels. Samples were probed with anti-Hsp70, anti-GAPDH (Abcam, Cambridge, MA, USA), anti- human Dicer (Cell Signaling Technology, Boston, MA), anti- moDicer (Bethyl Laboratories, Montgomery, TX, USA), anti-Hsp90 (Enzo Life Sciences, Plymouth Meeting, PA, USA), rabbit anti-rat IgG-HRP (Enzo), goat anti-rabbit IgG-HRP, and goat anti-mouse IgG -HRP (Promega, Madison, WI, USA). Blots were developed with West Pico chemiluminescent substrate (Pierce) and visualised on X-ray film. Western blots were analysed using the Image J image analysis tool (http://rsbweb.nih.gov/ij/).
Quantitative real-time RT-PCR
Total RNA was isolated using the mirVANA kit from Ambion (Austin, TX). Reverse- transcription of 2 µg RNA per sample was performed with Superscript II (Invitrogen). Real-time qPCR was performed on an ABI7900 HT according to manufacturer instructions and relative quantification was calculated using the ΔΔCt method (Perkin-Elmer Taqman User Bulletin 2) with GAPDH as the housekeeping gene. Amplification of cDNA was performed with Taqman PCR Master Mix or SYBR Green Master Mix (Eurogentec, San Diego, CA) depending on primer type. Primers used were GAPDH 5′-GAAGGTGAAGGTCGGAGTC-3′ [fwd], 5′-GAAGATGGTGATGGGATTTC-3′ [rev], 5′-FAM-CAAGCTTCCCGTTCTCAGCC-TAMRA-3′ [probe]; Dicer 5′-GTACGACTACCACAAGTACTTC-3′ [fwd], 5′-ATAGTACACCTGCCAGACTGT-3′ [rev] Citation[34]; Hsp90 5′-AGCTCAAGCCCTAAGAGACAACT-3′ [fwd], 5′-AAGATGACCAGATCCTTCACAGA-3′ [rev]; Hsp70 5′-GCCGAGAAGGAGTTTGA-3′ [fwd], 5′-TCCGCTGATGATGGGGTTAC-3′ [rev]. MicroRNAs were quantified using 10 ng of total RNA in individual Taqman RT-qPCR assays for miR-125b, miR-138, miR-154, miR-196b, miR-378, miR-382, miR-452, and miR-606 (Applied Biosystems), and run on an ABI7900 HT using U6B as a reference transcript.
Results
Multiple methods have been employed to induce the heat shock response in vitro, and the dynamics of the response varies somewhat with the technique employed. To verify that our method was sufficient to induce a HSR, the gene and protein expression levels of two abundant and highly conserved heat responsive proteins, Hsp70 and Hsp90 were determined. We measured mRNA levels by real-time RT-qPCR in multiple cell lines heated at 39.5°C for up to 9 h. In the cell lines tested, elevated levels of Hsp70 mRNA occurred after as little as 1 h of exposure to heat. Peak expression of Hsp70 mRNA occurred after 3–4 h of heat exposure followed by a decrease in subsequent hours (), although the exact patterns varied slightly with the cell type. Time course experiments carried out to 9 h show a plateau of Hsp70 expression at a level higher than in the untreated control ().
Figure 1. Transcriptional response of Hsp70 and Hsp90 to fever-range hyperthermia. Mild hyperthermia at 39.5°C induced Hsp70 and Hsp90 mRNA. Bar graphs depict fold changes in mRNA that were measured by RT-qPCR and normalised to GAPDH. Control sample represents cells maintained at 37°C. Human cell lines JAR (placental choriocarcinoma) and HeLa (cervical carcinoma) display typical in vitro response patterns of cells grown at 39.5°C for 8–9 h. Control Hsp70 mRNA expression is up-regulated quickly, peaking at 3–4 h (a, b). Expression levels then fall, stabilising after 7 h at a level above baseline expression. Expression of the Hsp90 gene rises more slowly than Hsp70, reaching a plateau after 5–7 h (c & d). Error bars were calculated using standard deviations based on technical replicates from one of three independent experiments. Statistically significant change in HSP mRNA was seen in both cell lines based on a two tailed Students t-test (p < 0.05).
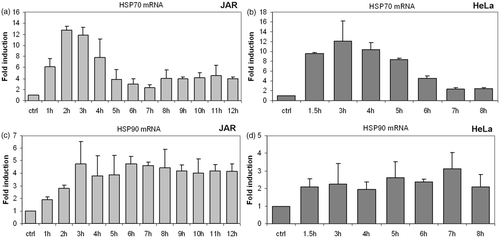
Elevations of Hsp90 mRNA levels were not as pronounced as Hsp70 mRNA. Hsp90 mRNA remained at or near the peak value for the duration of the heat treatment in JAR and HeLa cells ().
To verify that the HSR was affecting post-transcriptional cellular function, protein expression of Hsp70 and Hsp90 was measured by western analysis. Both Hsp70 and Hsp90 responded to 39.5°C hyperthermia ( and ). Unlike the early peak followed by the rapid decline of Hsp70 mRNA levels, Hsp70 protein showed a relatively stable intracellular expression pattern following hyperthermia (). Of the cell types tested, only the human trophoblast line JAR and the mouse trophoblast line SM-9 (data not shown) showed a significant increase in Hsp70 protein following heat treatments at 39.5°C (). It should be noted that Hsp70 protein has a high constitutive presence in many cells, which makes detection of small changes in protein expression difficult by western blots. Hsp70 protein expression was generally up-regulated in the cell types tested, although these levels remained unchanged in certain cell types ( and data not shown). Further, the early effects of the heat shock response rely mainly on a change in the conformation and function of existing Hsp70 molecules rather than, or in addition to, altered expression levels of nascent HSPs Citation[9].
Figure 2. Translational response of Hsp70 to fever range hyperthermia. Western blot analysis was used to determine Hsp70 protein expression following treatment at fever-range hyperthermia. (a) The human JAR trophoblast cell line showed elevations in Hsp70 protein in response to elevated temperatures by 4 h. A similar trend was observed in (b) HeLa cells. However, Hsp70 protein levels were reduced beyond the 6-h time point.
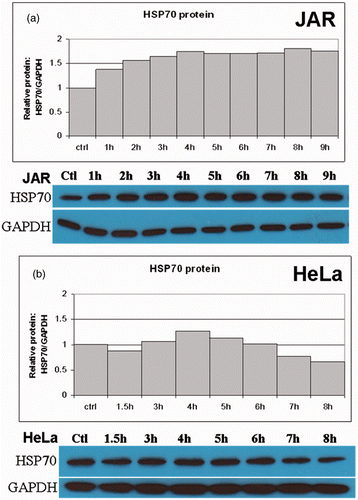
Figure 3. Translational response of Hsp90 to fever range hyperthermia. Western blot analysis was used to analyse Hsp90 protein expression following treatment and fever-range hyperthermia. Hsp90 protein expression varied by cell line, but was enhanced at some point before 9 h of heat treatment. (a) The JAR cell line showed steadily increasing levels of Hsp90 through 7 h. (b) HeLa cells exhibited an interrupted pattern of HSP90 expression, with all time points elevated above baseline.
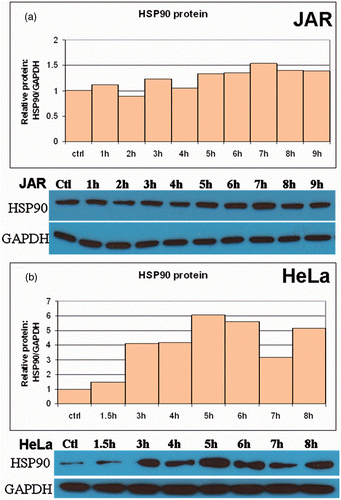
Changes in the protein concentrations of Hsp90 were pronounced when cells were treated at 39.5°C. Basal cellular concentrations of Hsp90 were high, although the pattern of expression varied between JAR and HeLa cells (). In all of the cell lines tested, Hsp90 protein expression increased as the heat treatment time increased. However, this relationship was not always linear and alterations were observed in the first 9 h in Hsp90 levels in HeLa cells (). Collectively, these results describe the kinetics of Hsp70 and Hsp90 transcription and translation in response to fever-range hyperthermia in JAR and HeLa cells.
Having established the heat shock protein response in our system, we initiated experiments to test the hypothesis that the RNase III endoribonuclease Dicer is responsive to fever range hyperthermia. Importantly, we observed changes in the levels of Dicer protein in time course experiments (). Of the 10 cell lines screened, nine showed enhanced expression of Dicer protein at 39.5°C. Analysis of multiple time points during heating showed Dicer protein levels above those at the 37°C control and the expression levels of Dicer fluctuated during the period of heating. These fluctuations (oscillations) of Dicer protein were found to be a consistent feature of the cell's response to mild hyperthermia (). Moreover, unlike the mRNA response of the heat shock proteins, Dicer mRNA expression did not regularly respond to heat treatment (). In the one experiment that showed Dicer mRNA alterations, the magnitude was small and in a repressive direction (). The level of Dicer mRNA in HeLa cells beyond the 7-h time point was not significantly different from Dicer mRNA levels in cells incubated at 37°C based on a two-tailed Student's t-test with a 95% confidence interval. However, Dicer protein alteration beyond the 7-h time point is reflected in the Dicer protein data ( – HeLa). Further, in the JAR cell line, there was no clear concordance between Dicer mRNA ( – JAR) and Dicer protein expression patterns ( – JAR). In sum, significant changes in Dicer mRNA were not detected by real-time RT-qPCR, in contrast to the substantial changes observed in the Dicer protein expression on western analysis.
Figure 4. Translational response of Dicer following treatment at fever range hyperthermia. In all cell lines where the response to thermal stress was confirmed by Hsp70 and Hsp90 mRNA and protein expression changes, levels of Dicer protein were found to fluctuate over the course of exposure to mild hyperthermia at 39.5°C (a). (b–d) Bar graphs in show quantified values obtained from the western blots shown in A. All cells except the breast cancer line BT-20 (b) clearly show fluctuations above the untreated control. Specific patterns of thermal response vary between cell types. Cells where Dicer protein was found to be heat responsive included human cancers (b) Raji, JAR, HeLa and SW13, murine cancer and non-cancer cell lines (c) B16, SM-9 and human lung fibroblast (MRC-5) and (d) primary cells (wild type mouse renal epithelial and fibroblast cells). Western blots and bar graphs are representative of two or more independent experiments.
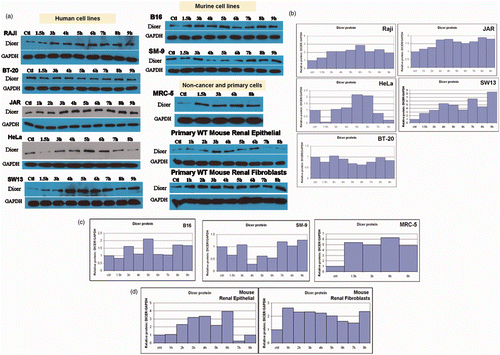
Figure 5. Dicer protein levels fluctuate in response to fever-range hyperthermia, with little change in Dicer mRNA. Bar graphs show Dicer mRNA expression expressed as fold induction relative to GAPDH. Specific patterns of protein fluctuations vary with cell lines, but changes in Dicer protein expression were present in all cells following treatment with fever range hyperthermia. Dicer mRNA levels do not show significant variation (p > 0.05) in the JAR (a) and HeLa (b) cell lines, suggesting that Dicer is regulated post-transcriptionally in these cells. Error bars were calculated using standard deviations based on technical replicates from three independent experiments. Statistical significance was assigned based on a two-tailed Students t-test and found to be significant.
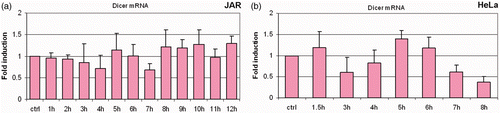
Wilmink Citation[7] identified a subset of microRNAs that were thermally regulated, albeit by high temperature stress (44°C) and for a shorter time (40 min) than in our experiments. Eight microRNAs in concentrations shown to be thermally responsive by Wilmink Citation[23] were assayed by RT-qPCR in time-course experiments during fever-range hyperthermia in JAR and HeLa cells (). In HeLa, miR-125 b and miR-154 were up-regulated, and in JAR, the expression of miR-382 was enhanced.
Figure 6. Effects of fever range hyperthermia on selected microRNA. Expression levels of eight microRNAs previously identified to be thermally regulated (see text) were analysed by RT-qPCR. (a) miR-125 b and miR-154 were found to be thermally up-regulated in HeLa cells. (b) In JAR, miR-382 was found to be enhanced by thermal stress.
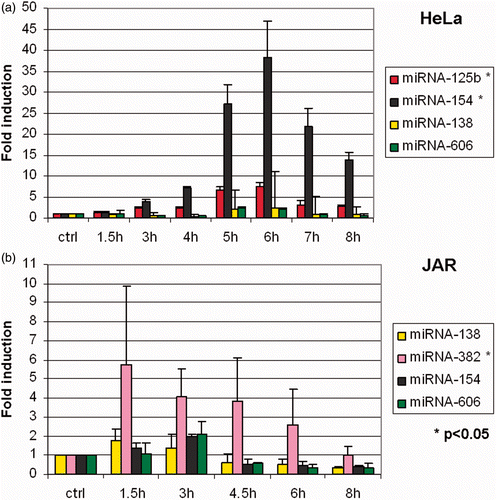
Discussion
Presented in this study is the response of Dicer protein to 39.5°C heat treatment in 10 cancer and non-cancer cell types. Experiments in which the heat treatments induced both a transcriptional and a translational HSP response were examined for Dicer expression. The cells studied included human cancers of the cervix (HeLa), trophoblast (JAR), adrenal gland (SW13), breast (BT-20), and lymph (Raji), as well as mouse trophoblast (SM-9) and skin (B16) cancers. Non-cancer cells were tested as well; both human transformed lung fibroblasts (MRC-5) and primary cells cultured from freshly excised mouse kidneys. The specific patterns of regulation varied with cell type. Variations among repeats of the same cell type were consistent, although small differences occurred in the timing of the highs and lows of Dicer expression ( and ). All cells except BT-20 had at least one time point above baseline (37°C) Dicer expression. Half of the cells had time points where Dicer expression fell below baseline (Bl6, SM-9, HeLa, BT-20, and primary renal epithelial) while the other half (JAR, SWl3, Raji, MRC-5, and primary renal fibroblasts) exhibited up-regulation only. Importantly, examination of Dicer mRNA levels in these samples suggests that regulation is occurring after Dicer mRNA is transcribed. We have confirmed statistically significant differences in Dicer protein levels in normal human kidney cells in culture based on three independent experiments. Furthermore, fever range hyperthermia has also been shown to increase significantly the activity of both types of interferons Citation[28], which we previously demonstrated to have post-transcriptional regulatory effects on Dicer Citation[27]. However, whether Dicer variations have a role in the hyperthermia-induced augmentation of interferon antiviral activity has yet to be established. Important unanswered questions include whether Dicer is modulated at the initiation of cell death pathways, and the role of NF-κB in Dicer regulation.
Dicer protein expression analysed by western blot was found to fluctuate (oscillate) over the course of a 9-h, 39.5°C treatment in multiple cell types ( and ). Oscillations in signalling molecules, such as NF-κB, have recently been identified Citation[35–38]. The finding in our studies that oscillations in Dicer protein expression are induced by mild heat stress (39.5°C) is therefore of interest since oscillations in Dicer could potentially be pivotal in cellular stress responses. Ongoing studies are addressing the effects of heat stress on oscillations of NF-κB, Dicer and microRNAs and their characteristics and impact on cellular function. We are particularly interested in whether NF-κB oscillations occur in these cells and whether this is responsible for the Dicer/microRNA oscillations. Two studies have examined the cellular profiles of microRNAs in response to heat shock Citation[7], Citation[24] although Dicer protein levels were not reported. The methods of heating and experimental set-ups are different between the two studies, and there is an unexpected disparity in the microRNAs found to be altered by the heat shock treatments. We tested several of the microRNAs found by Wilmink Citation[23] and verified that two of the microRNAs identified as thermally regulated (miR-125b, miR-154) were enhanced by our heat treatments in HeLa, and one miR-382, in JAR. This suggests that the intensity and duration of heat stress, as well as the cell type, may affect which microRNAs are transcribed.
In our experiments, we attempted to provide consistent heating and growth conditions. Nevertheless, some microRNA expression variability was observed between cell types and, to a much lesser degree, between repeated experiments of the same cell type. To evaluate these variables, two independent investigators studied multiple cell types and performed experimental replicates, and the underlying trends in the data were examined and found to be consistent. One goal of these experiments was to mimic clinical hyperthermia with as much accuracy as our in vitro model allowed. Studies currently in progress involve determining whether there are changes of an oscillatory nature in Dicer levels when cells are subjected to varying degrees of thermal stress as compared with other stresses. Recent investigations from other laboratories showed that the expression of important regulatory genes and proteins that respond to interferons, cytokines and growth factors may be governed through oscillatory mechanisms Citation[39–41]. Whether Dicer as well as microRNAs respond to thermal stress in an oscillatory manner is therefore of considerable interest.
Two of the heat shock proteins upregulated by HREs were used to validate heat stress in this study. We characterised the thermal stress reaction in our study as an up-regulation of Hsp70 mRNA and protein paired with up-regulation of Hsp90 mRNA and protein expression. These changes in HSP expression, as well as up-regulation of HSFl mRNA expression, indicate the presence of the heat shock response Citation[6], Citation[19], Citation[42]. No significant changes in HSFl protein expression were detected in our study as the activation and nuclear entry of HSFl occurs rapidly after the application of heat stress Citation[6], Citation[14], Citation[43]. Hence, our experimental window did not capture changes in HSF1 expression. However, HSPs downstream of HSF1, such as Hsp70 and Hsp90 were observed to be significantly up-regulated with our heating protocol and this is emphasised in the discussion.
The scope of our experiments was limited to 9 h at 39.5°C heat treatment. This time frame was selected to study the cellular events occurring during the transition from the basal metabolic state to the heat shock response. The mRNA and protein response of HSPs began to stabilise around 8–9 h. This finding led us to focus on time points earlier than 9 h to observe the transition to the heat shock response. The degree of cell death in the heat-treated samples at 39.5°C was minimal, and consistent with levels seen in controls.
In several aspects of cellular regulation, HSP and microRNA syntheses are interdependent. Hsp90 has been implicated in the formation of RNA-induced silencing complexes in plants Citation[44] and both Hsp70 and Hsp90 have been reported to serve a role in loading the RISC complex with miRNA Citation[30], Citation[45]. Hsp90 also associates with Argonaute 2, an important component in microRNA-induced gene silencing in stress granules Citation[46]. Future studies should also focus on the potential role microRNAs may play in the regulation of non-HSP production during heat stress.
The presence of stress-induced alterations in Dicer suggests that microRNA biogenesis may be stress-responsive. Multiple reports have described how specific microRNA levels are altered in response to heat shock, herpes virus infection and light adaptation among others Citation[23], Citation[47], Citation[48]. Several studies have confirmed that there are alterations in the levels of certain microRNAs that are specific to the stress inducing them Citation[49–52]. These and other observations have led to theories that microRNAs may function to safeguard the genome from environmental fluctuations Citation[53]. The molecular events that regulate microRNA homeostasis are an active research area Citation[54], even though a mechanistic explanation describing how stress-responsive microRNAs are regulated is currently an open question. Although our work focused on Dicer alteration during thermal stress, other studies have described regulatory proteins, such as BRCA1, that act upstream of Dicer in microRNA biogenesis by regulating Drosha Citation[55]. Thus, Dicer is likely one of several molecular players that may link cellular stress with the microRNA response.
Conclusion
In this study we described the repression of human Dicer protein during heat treatment at 39.5°C in 10 unique cell lines, as well as normal kidney cells. We showed that Dicer is regulated post-transcriptionally in JAR and HeLa cells and that Dicer protein levels oscillate following heat stress.
Acknowledgements
We thank Professor Elizabeth Repasky for helpful discussions during this work and for reviewing the manuscript, and Jennifer Wiesen for excellent technical assistance and suggestions. We extend a special note of appreciation to Professor Edwin Mirand for his support of our program.
Declaration of interest: Research reported in this publication was supported by the National Cancer Institute of the US National Institutes of Health under award number R01 CA124971 and utilised core facilities supported in part by an NCI Comprehensive Cancer Center core grant P30 CA016056. The authors report no conflicts of interest. The authors alone are responsible for the content and writing of the paper.
References
- Levinthal C. How to Fold Graciously. Mossbauer Spectroscopy. ‘Biological Systems’ Proc of a meeting held at Allerton house, Monticello, Illinios, JTP DeBrunner, JCM Tsibris, E Miinck. University of Illinois Press, Urbana, IL 1969; 22–24
- Bryngelson JD, Socci ND, Wolynes PG. Funnels, pathways, and the energy landscape of protein folding: A synthesis. Proteins 1995; 21: 167–195
- Dubois MF, Hovanessian AG, Bensaude O. Heat-shock-induced denaturation of proteins. Characterization of the insolubilization of the interferon-induced p68 kinase. J Biol Chem 1991; 266: 9707–9711
- Hendrick JP, Hartl F-U. Molecular Chaperone Functions of Heat-Shock Proteins. Annu Rev Biochem 1993; 62: 349–384
- Mifflin LC, Cohen RE. Characterization of denatured protein inducers of the heat shock (stress) response in Xenopus laevis oocytes. J Biol Chem 1994; 269: 15710–15717
- Wu C. Heat shock transcription factors: Structure and regulation. Annu Rev Cell Dev Biol 1995; 11: 441–469
- Wilmink GJ, Rivest BD, Ibey BL, Cundin LX, Haywood EC, Roach WP. A signature microRNA expression profile for the cellular response to thermal stress. Proc SPIE 2009; 7175: 7175OU1–7175OU-11
- Ma S, Bhattacharjee RB, Bag J. Expression of poly(A)-binding protein is upregulated during recovery from heat shock in HeLa cells. FEBS J 2009; 276: 552–570
- Vabulas RM, Raychaudhuri S, Hayer-Hartl M, Hartl FU. Protein Folding in the Cytoplasm and the Heat Shock Response. Cold Spring Harb Perspect Biol 2010; 2: a004390, doi:10.1101/cshperspect.a004390
- Falk MH, Issels RD. Hyperthermia in oncology. Int J Hyperthermia 2001; 17: 1–18
- Mace TA, Zhong L, Kokolus KM, Repasky EA. Effector CD8+ T cell IFN-γ production and cytotoxicity are enhanced by mild hyperthermia. Int J Hyperthermia 2012; 28: 9–18
- Richter K, Haslbeck M, Buchner J. The heat shock response: Life on the verge of death. Mol Cell 2010; 40: 253–266
- Akerfelt M, Morimoto RI, Sistonen L. Heat shock factors: Integrators of cell stress, development and lifespan. Nat Rev Mol Cell Biol 2010; 11: 545–555
- Pirkkala L, Nykanen P, Sistonen L. Roles of the heat shock transcription factors in regulation of the heat shock response and beyond. FASEB J 2001; 15: 1118–1131
- Westerheide SD, Morimoto RI. Heat shock response modulators as therapeutic tools for diseases of protein conformation. J Biol Chem 2005; 280: 33 097–33100
- Taipale M, Jarosz DF, Lindquist S. Hsp90 at the hub of protein homeostasis: Emerging mechanistic insights. Nat Rev Mol Cell Biol 2010; 11: 515–528
- Ciocca DR, Fanelli MA, Cuello-Carrion FD, Castro GN. Heat shock proteins in prostate cancer: From tumorigenesis to the clinic. Int J Hyperthermia 2010; 26: 737–747
- Zou J, Guo Y, Guettouche T, Smith DF, Voellmy R. Repression of heat shock transcription factor HSFl activation by Hsp90 (Hsp90 complex) that forms a stress-sensitive complex with HSFl. Cell 1998; 94: 471–480
- Morimoto RI. The heat shock response: Systems biology of proteotoxic stress in aging and disease. Cold Spring Harb Symp Quant Biol 2011; 76: 91–99
- Spriggs KA, Bushell M, Willis AE. Translational Regulation of gene expression during conditions of cell stress. Mol Cell 2010; 40: 228–237
- Cuesta R, Laroia G, Schneider RJ. Chaperone Hsp27 inhibits translation during heat shock by bind eiF4G and facilitates dissociation of cap-initiation complexes. Genes Dev 2000; 14: 1460–1470
- Fritah S, Cole E, Boyault C, Govin J, Sadoul K, Chiocca S, et al. Heat-shock factor 1 controls genome-wide acetylation in heat-shocked cells. Mol Biol Cell 2009; 20: 4976–4984
- Wilmink GJ, Roth CL, Ibey BL, Ketchum N, Bernhard J, Cerna CZ, et al. Identification of microRNAs associated with hyperthermia-induced cellular stress response. Cell Stress Chaperones 2010; 15: 1027–1038
- Yu J, Liu F, Yin P, Zhu X, Cheng G, Wang N, et al. Integrating miRNA and mRNA expressions profiles in response to heat stress-induced injury in rat small intestine. Funct Integr Genomics 2011; 11: 203–213
- Kaur P, Hurwitz MD, Krishnan S, Asea A. Combined Hyperthermia and Radiotherapy for the Treatment of Cancer. Cancers 2011; 3: 3799–3823
- Gandellini P, Profumo V, Folini M, Zaffaroni N. MicroRNAs as new therapeutic targets and tools in cancer. Expert Opin Ther Targets 2011; 15: 265–279
- Wiesen JL, Tomasi TB. Dicer is regulated by cellular stresses and interferons. Mol Immunol 2008; 46: 1222–1228
- Payne J, Nair MP, Ambrus JL, Chadha KC. Mild hyperthermia modulates biological activities of interferons. Int J Hyperthermia 2000; 16: 492–507
- Detzer A, Engel C, Wiinsche W, Sczakiel G. Cell stress is related to the re-localization of Argonaute 2 and to decreased RNA interference in human cells. Nucl Acids Res 2011; 39: 2727–2741
- Iwasaki S, Kobayashi M, Yoda M, Sakaguchi Y, Katsuma S, Suzuki T, et al. Hsc70/Hsp90 chaperone machinery mediates ATP-dependent RISC loading of small RNA duplexes. Mol Cell 2010; 39: 292–299
- McKendry R, John J, Flavell D, Muller M, Kerr IM, Stark GR. High-frequency mutagenesis of human cells and characterization of a mutant unresponsive to both alpha and gamma interferons. Proc Natl Acad Sci USA 1991; 88: 11455–11459
- Hunt JS, Banerjee S, Pace JL. Differential expression and regulation of a human transgene, HLA-B27, in mouse placental and embryonic cell lines. Mol Human Reprod 1998; 4: 817–825
- Khandrika L, Kim FJ, Campagna A, Koul S, Meacham RB, Koul HK. Primary culture and characterization of human renal inner medullary collecting duct epithelial cells. J Urol 2008; 179: 2057–2063
- Karube Y, Tanaka H, Osada H, Tomida S, Tatematsu Y, Yanagisawa K, et al. Reduced expression of Dicer associated with poor prognosis in lung cancer patients. Cancer Sci 2005; 96: 111–115
- Nelson DE, Ihekwaba AE, Elliott M, Johnson JR, Gibney CA, Foreman BE, et al. Oscillations in NF-kappaB signaling control the dynamics of gene expression. Science 2004; 306: 704–708
- Avraham R, Yarden Y. Regulation of signaling by microRNAs. Biochem Soc Trans 2012; 40: 26–30
- Kadener S, Menet JS, Sugino K, Horwich MD, Weissbein U, Nawathean P, et al. A role for microRNAs in the Drosophila circadian clock. Genes Dev 2009; 23: 2179–2191
- Saini C, Morf J, Stratmann M, Gos P, Schibler U. Simulated body temperature rhythms reveal the phase-shifting behavior and plasticity of mammalian circadian oscillators. Genes Dev 2012; 26: 567–580
- Bartfeld S, Hess S, Bauer B, Machuy N, Ogilvie LA, Schuchhardt J, et al. High-throughput and single-cell imaging of NF-kappaB oscillations using monoclonal cell lines. BMC Cell Biol 2010; 11: 21
- Sun L, Yang G, Zaidi M, Iqbal J. TNF-induced gene expression oscillates in time. Biochem Biophys Res Commun 2008; 371: 900–905
- Shankaran H, Ippolito DL, Chrisler WB, Resat H, Bollinger N, Opresko LK, et al. Rapid and sustained nuclear-cytoplasmic ERK oscillations induced by epidermal growth factor. Mol Syst Biol 2009; 5: 332
- Wang SM, Khandekar JD, Kaul KL, Winchester DJ, Morimoto RI. A method for the quantitative analysis of human heat shock gene expression using a multiplex RT-PCR assay. Cell Stress Chaperones 1999; 4: 153–161
- Zobeck KL, Buckley MS, Zipfel WR, Lis JT. Recruitment timing and dynamics of transcription factors at the Hsp70 loci in living cells. Mol Cell 2010; 40: 965–975
- Iki T, Yoshikawa M, Nishikiori M, Jaudal MC, Matsumoto-Yokoyama E, Mitsuhara I, et al. In vitro assembly of plant RNA-induced silencing complexes facilitated by molecular chaperone Hsp90. Mol Cell 2010; 39: 282–291
- Landthaler M. Chaperones get RISC loaded. Mol Cell 2010; 39: 161–162
- Leung AK, Sharp PA. Quantitative analysis of Argonaute protein reveals microRNA-dependent localization to stress granules. Proc Natl Acad Sci USA 2006; 103: 18125–18130
- Cazalla D, Yario T. Steitz JA. Down-regulation of a host microRNA by a Herpes virus saimiri noncoding RNA. Science 2010; 328: 1563–1566
- Krol J, Busskamp V, Markiewicz I, Stadler MB, Ribi S, Richter J, et al. Characterizing light-regulated retinal microRNAs reveals rapid turnover as a common property of neuronal microRNAs. Cell 2010; 141: 618–631
- Crosby ME, Kulshreshtha R, Ivan M, Glazer PM. MicroRNA regulation of DNA repair gene expression in hypoxic stress. Cancer Res 2009; 69: 1221–1229
- Strum JC, Johnson JH, Ward J, Xie H, Feild J, Hester A, et al. MicroRNA 132 regulates nutritional stress-induced chemokine production through repression of SIRT1. Mol Endocrinol 2009; 23: 1876–1884
- Sangokoya C, Telen MJ, Chi JT. MicroRNA miR-144 modulates oxidative stress tolerance and associates with anemia severity in sickle cell disease. Blood 2010; 116: 4338–4348
- Saleh AD, Savage JE, Cao L, Soule BP, Ly D, DeGraff W, et al. Cellular stress induced alterations in microRNA let-7a and let-7b expression are dependent on p53. PLoS One 2011; 6: e24429
- Leung AK, Sharp PA. MicroRNAs: A safeguard against turmoil?. Cell 2007; 130: 581–585
- Krol J, Loedige I, Filipowicz W. The widespread regulation of microRNA biogenesis, function and decay. Nat Rev Genet 2010; 11: 597–610
- Kawai S, Amano A. BRCA1 regulates microRNA biogenesis via the DROSHA microprocessor complex. J Cell Biol 2012; 197: 201–208