Abstract
Purpose: Heat shock protein 70 (HSPA family) is a multi-functional protein which protects individual cells from proteotoxic shock and the whole organism from microbial, viral and oncogenic pathogens. These diverse functions may depend upon ‘chaperone’ activity that allows Hsp70 to regulate the mechanism of damaged protein recovery or utilisation inside a cell and to be a potent adjuvant, stimulating immune activity against a variety of viral or tumour antigens. The aim of this review is to present recent data on specific roles of intracellular and extracellular Hsp70 in cancerous tissue.
Conclusion: The data presented in this paper show that endogenous Hsp70 protects cancer cells of different origins from a variety of cytotoxic threats including cancer cell therapeutics. In contrast, however, Hsp70 released from stressed cancer cells can serve as a danger signal or may recruit cells responsible for the generation of innate and adaptive immune responses against tumour cells.
Introduction
Protein chaperones belonging to the Hsp70 family mediate a great number of intracellular and extracellular functions. These functions, which include cytoprotection, adjuvant activity, plasma membrane penetration and many others, are thought to be linked to their chaperone activity. The chaperone mechanism of Hsp70 acts to recognise newly synthesised or damaged polypeptides, ‘palpating’ it and deciding what should be done with the protein which could include its reconstitution to the active form or redirecting it to proteolytic machinery. In this function Hsp70 is assisted by ATP and co-chaperones, proteins of the DnaJ family helping in substrate-binding and Bag-1-like proteins regulating nucleotide exchange [Citation1,Citation2]. Using chaperone activity, Hsp70 maintains proteostasis in a given cell and protects it from a variety of cytotoxic agents including, unfortunately, those used in cancer therapy, strongly limiting their effectiveness. However, Hsp70-mediated cell protection can be surmounted by the fact that under the influence of certain factors, Hsp70 may be released into an extracellular milieu and acquire new properties and functions. For instance, it can turn back to the same cells and protect them from cytotoxic stimuli or induce signalling through a variety of receptor-mediated machineries in the manner of a ‘chaperokine’ [Citation3] in order to protect the whole organism. Lastly, the appearance of Hsp70 on a cancer cell surface could sensitise the latter to the cytotoxic action of natural killer (NK) cells, whereas the chaperone accumulated in an extracellular matrix can induce a specific anti-tumour response. Thus some properties of Hsp70 help a cancer cell to evade natural or acquired cytotoxicity while others act to favour immune attack and thus could assist in immunotherapy. This review aims to emphasise some of these diverse properties of Hsp70 that can be regulated in order to control tumour growth and dissemination.
Hsp70 as a survival tool for cancer cells
Cancer cells in a growing tumour show fast and strong metabolism when compared to cells isolated from normal tissue [Citation4]. The process of enhanced protein synthesis requires higher levels of cellular chaperones to ensure correct conformation of nascent polypeptides and their transport to target cellular organelles. This is likely the reason why many types of cancer cells contain high amounts of cellular chaperones, particularly Hsp70 [Citation5]. Another likely reason for extremely high expression of inducible Hsp70 in malignant cells is due to internal stress caused by the accumulation of mutated and incorrectly folded proteins and the inappropriate activity of deregulated signalling pathways [Citation6]. As a result, almost all cell lines derived from clinical tumour samples and employed in cell biology demonstrate elevated amounts of Hsp70. An increased level of this protein was observed at earlier stages of malignancy in hepatocellular carcinoma, a fact that allows us to consider Hsp70 content as a marker of early tumour progression [Citation7]. A positive correlation between hsp70 gene expression and malignancy has been observed in cases of bladder cancer [Citation8], colon cancer [Citation9], endometrium carcinoma, uterus cervix carcinoma [Citation10] and many others.
Hsp70 is known to protect cells from numerous cytotoxic treatments including those able to induce programmed cell death. This was demonstrated by studies in which Hsp70 cellular content was modulated by various means including transfection with the appropriate gene, treatment by a natural factor such as heat shock, liposomal delivery of pure chaperone, treatment with short interfering RNA or others. For instance anti-sense RNA-mediated reduction of Hsp70 synthesis in breast carcinoma MCF-7 cells caused caspase-independent apoptosis [Citation11].
The anti-apoptotic function of Hsp70 has been discussed extensively during last few years and the major conclusion of these reviews is that the chaperone is able to interact with molecules participating at the different levels of apoptotic signalling cascade [Citation12]. Targets of Hsp70 could be upstream and downstream of mitochondria. A key point for Hsp70 action in the apoptotic programme is at the level of Apaf-1. Hsp70 is able to bind to the CARD domain of Apaf-1 to prevent the oligomerisation of the latter and the consequent activation of caspase 9 [Citation13]. Although the oligomerisation takes place in the presence of Hsp70, no binding of pro-caspase 9 to the newly formed complex was found because the CARD domain was already occupied by Hsp70 molecules [Citation14].
Other groups focused on Bax protein, known to facilitate the release of cytochrome c from mitochondria. Over-expression of Hsp70 negatively regulated oligomerisation and translocation of Bax to the mitochondria [Citation15]. The data demonstrated Hsp70-mediated inhibition of stress-induced induction of JNK protein kinase, which in turn resulted in the suppression of Bax transport to mitochondrial membrane. In another study the authors used vectors with the full-length hsp70 gene as well as its deletion mutants lacking either chaperone (hsp70-EEVD) or ATPase functions (hsp70-ATPase). They found as well that the over-expression of the full-size protein inhibited Bax activation and reduced the release of apoptosis initiating factor (AIF), from mitochondria initially caused by a metabolic inhibitor [Citation16]. Interestingly, both mutants lacking either ATPase or EEVD chaperone domains were unable to prevent AIF release, suggesting that for the full-scale protective activity, the whole molecule Hsp70 is necessary [Citation16]. In favour of this notion are data indicating that the full-length Hsp70 molecule can efficiently bind AIF; such types of interaction can be extended to a great number of targets of the chaperone [Citation17].
Effector caspases 3 and 7 and their truncated forms were also shown to be targets for Hsp70 in U-937 human leukaemia cells stimulated to apoptosis with anti-cancer drugs. The delay in proteolysis of the enzymes was associated not only with the inhibition of caspase 9 activity, but also with the trapping of the above enzymes by Hsp70 [Citation18]. Furthermore, Hsp70 was found to prevent the process of cell death even downstream of caspase 3 and 7 activation. Earlier it was shown that phospholipase A2, essential for the last stage of TNF-α-induced apoptosis in WEHI-S mouse fibrosarcoma cells, was inactivated by the over-expressed chaperone [Citation19].
There are several other targets for Hsp70 which are also implicated in apoptosis signalling. First, Hsp70 actively suppresses signal transduction through the cascade of stress-kinases. These proteins, SAPK and JNK, take part in the development of the apoptosis programme, and on the other hand, in the initiation of cell proliferation. Mosser and coauthors showed that Hsp70 prevents JNK activation, and more recently, Hsp70 was proved to directly interact with JNK and to suppress its binding to the substrate, c-Jun [Citation20]. All these activities have been based on chaperone activity of Hsp70, which is supported by experiments with its deletion mutants.
Recent evidence suggests that Hsp70 can also exert its pro-survival function by inhibiting the lysosomal death pathway [Citation21], which involves lysosomal release of cathepsin proteases that can activate both caspase-dependent and caspase-independent cell death [Citation22]. This pathway can also be triggered by classical inducers of apoptosis such as TNF, p53 and ETO, and sensitised by Ras and Src oncogenes.
The cytoprotective activity of Hsp70 extends to rescuing cancer cells from senescence. Sherman and colleagues found that the down-regulation of Hsp72 in different cancer lines triggered cell senescence associated with activation and stabilisation of p53 and induction of the cell cycle inhibitor p21 [Citation23]. Accordingly, knockout of Hsp70 in the Her2/neu-expressing transgenic mice caused almost complete suppression of tumorigenesis. Instead of mammary tissue hyperplasia due to Her2 expression, the authors observed massive cell senescence in this tissue thus suggesting the pivotal role of the chaperone in protection from senescence signalling pathways [Citation24].
Summarising the research presented thus far, we conclude that in cancer cells Hsp70 functions as a multi-purpose protective mechanism safeguarding cells from the multiple threats to survival. Certainly, to arrange for the most appropriate anti-cancer therapy, one should keep in mind the protective potency of the Hsp70 chaperone. Below, we present some of the advances in strategies to inhibit Hsp70 expression or activity.
Factors regulating Hsp70 synthesis/activity
The biosynthesis of Hsps is regulated by specific transcription factors (HSF), which recognise heat shock elements (HSE) (5′-CNNGAANNTTCNNG-3′). Upon the binding to HSE, HSF summons RNA polymerase II complex to the appropriate HSP gene. Activation of HSF includes several steps of conformational transitions, trimerisation, phosphorylation and other regulatory events and requires the correct function of controlling machinery [Citation25].
Quercetin (3,3′,4′,5,7-pentahydroxyflavone) affects two stages of HSF-1 activation, phosphorylation and DNA-binding [Citation26]. However, later it was found to inhibit the activity of several protein kinases [Citation27]. Another HSF inhibitor, benzylidene lactam compound KNK437, reduces the expression of Hsp40, Hsp70 and Hsp105/110 [Citation28], probably by interfering with the activity of the C-terminal transactivation domain of HSF-1 and blocking its transcriptional activity [Citation29]. KNK437 was found to sensitise prostate cancer cells to hyperthermia [Citation30] and glioma cells to arsenic trioxide [Citation31].
The list of specific inhibitors of the ATPase activity of Hsp70 includes 3′-sulfogalactolipid that binds to the ATP-binding domain of the chaperone and reduces its function by an unknown mechanism [Citation32]. Another compound from the dihydropyrimidine group, MAL2-101 is also able to interact with the ATPase domain of Hsp70 molecule and disrupt Hsp70 function [Citation33]; this substance causes cell cycle arrest and caspase-mediated apoptosis in myeloma cells in vitro and in vivo [Citation34]. Several candidate hits have been received from high-throughput screens aimed at analysing molecules that inhibit interactions between Hsp70 and its co-chaperones, Hsp40 [Citation35] and Bag1 [Citation36]. Some of these compounds such as 2-phenylethynesulfonamide gave promising results in a mouse model of Myc-induced lymphomagenesis [Citation36]
There are a few substances which interact with the peptide-binding domain of Hsp70. Pifithrin-µ or 2-phenylethylensulphonamide can interfere with binding of Hsp70 to its client proteins, such as Apaf-1 [Citation37]. An anti-cancer effect of pifithrin-µ was shown in several tumour models [Citation38]. More specific binding to the Hsp70 C-terminal domain was generated based on the sequence of the binding site of AIF, an Hsp70 client protein. This peptide, ADD70, was shown to bind and inhibit Hsp70 activity and to sensitise mouse melanoma cells to cisplatin [Citation39].
In conclusion, the strong protective potential of intracellular Hsp70 has encouraged researchers to create new chemicals able to inhibit the biosynthesis of the protein or to block its chaperone activity. However, as we will see below, in addition to these efforts, nature may have provided us with another way to exploit the multiple facets of chaperone activity, particularly its function after being released from cancer cells, to better control tumour growth.
Release of Hsp70 from cancer cells and the mechanisms underlying this process
It has been shown previously that intracellular Hsp70 can move to the cell surface and even to the extracellular matrix. First, Hsp70 was found to leave glia and migrate to adjacent neurons where it may help in their protection [Citation40]; later, export of Hsp70 was demonstrated for rat embryo cells [Citation41] and human glioblastoma T-98 G cells [Citation42]. It may be quite meaningful that in the above cases the source of Hsp70 was living cells.
Compared to that seen in patients with non-cancerous pathologies, an elevated level of Hsp70 has been detected in the blood of humans with chronic myeloid leukaemia; moreover, a particularly high level of circulating chaperone was seen in two cases of acute leukaemia, suggesting this parameter to be an indicator of a poor prognosis for this type of cancer [Citation43,Citation44]. Investigating the levels of intracellular, cell surface expressed, and extracellular Hsp70 in patients with chronic lymphoma, Dempsey and colleagues found that the level of chaperone circulating in plasma correlated well with internal levels of Hsp70 [Citation45]. Generally, human leukaemia is one of the cancers that are closely linked to the enhanced export of Hsp70 chaperone into blood circulation [Citation46]. An increased level of Hsp70 was also observed in sera of patients with colorectal cancer; this value was a significant predictor of mortality during the follow-up period [Citation47]. When the levels of soluble Hsp70 were analysed in patients with non-resected primary tumours compared with ones subjected to tumour resection 4–6 weeks before the study, Hsp70 serum content was found to be significantly higher in the former group. The value of serum Hsp70 level was also observed to be an important indicator in patients with glioblastoma [Citation48]. Recent data indicate that pancreatic cancer is accompanied by the enhanced secretion of the chaperone into serum [Citation49]. On the basis of earlier data and data presented above, it appears likely that an increased level of circulating Hsp70 is a common feature of serum proteome of cancers explored to date [Citation50,Citation51]. In this connection it is noteworthy that the potentially dangerous role ascribed to the exogenous Hsp70 is in contradiction with findings of numerous groups that demonstrated significant anti-tumour immune potential of the extracellular chaperone [Citation52]. We suggest that high levels of extracellular Hsp70 in sera of cancer patients are related to progressive cell death in tumour tissue; consequently, a part of the exogenous chaperone pool may become a danger signal for living tumour cells, increasing their capacity to maintain proliferation so another part can induce immune response. These subjects will be discussed in following sections.
The appearance of Hsp70 in extracellular milieux can be caused by the deleterious effects of inflammatory stimuli or oxidative stress leading to the death of large cell populations [Citation53,Citation54]. Another source of exogenous Hsp70 can be factors or drugs affecting cell physiology mainly in a non-destructive mode. During the last few years the list of agents causing Hsp70 release has grown and some of them are worth mentioning. Firstly, photodynamic therapy using meso-tetrahydroxyphenyl chlorin employed in experiments with EMT-6 cells was found to result in growth control and in a significantly elevated export of Hsp70 [Citation55]. The authors showed that the PDT conditions that induce strong heat shock response do not correspond to successful tumour cure, while doses inducing the elevation of extracellular Hsp70 are likely to give optimal control of tumour cell growth, suggesting that the release of the chaperone is most important for therapeutic efficacy. Secondly, several groups have established a direct tie between radiotherapeutic treatment and the amount of Hsp70 released from cancer cells to their cell surface or to the blood. In patients with prostate cancer subjected to X-ray therapy, the level of circulating serum Hsp72 increased an average of 3.5-fold in concert with an increase in the concentrations of interleukin-6, tumour necrosis factor, cytotoxic CD8+ and NK cells.[Citation56]. The results of in vitro studies with the use of supernatants recovered from irradiated human prostate cancer cells showed that the most probable inducer of the immune response observed could be Hsp70-containing exosomes. In another study, cells of human colorectal tumour cell lines and mouse colon carcinoma tumour cell line were subjected to X-ray irradiation and/or hyperthermia. The cells were found to export Hsp70 to their surface, and after the combined action of irradiation and heat stress, to the medium. This treatment resulted in up-regulation of the co-stimulatory molecule CD80 and the chemokine receptor CCR7 on dendritic cells; the rate of phagocytosis by macrophages and dendritic cells (DC), and levels of pro-inflammatory cytokines interleukin-8 and 12 were also significantly increased [Citation57].
Some anti-cancer drugs have also been shown to stimulate Hsp70 release by tumour cells; this was demonstrated by results of experiments with human hematopoietic tumour lines treated with histone deacetylase (HDAC) inhibitor (FR901228,); this treatment caused apoptosis which was strongly linked to the surface expression of Hsp70. Interestingly, two anti-cancer drugs and known inducers of apoptosis, etoposide and camptothecin also induced the extracellular transport of Hsp70 [Citation58]. Similar data were obtained in studies when HepG2 carcinoma cells were treated with anti-cancer drugs. The latter were shown to induce Hsp60, Hsp70 and Hsp90 and caused the release of exosomes loaded with the above stress proteins [Citation59]. Perhaps acting as a decoy, the HSP-containing exosomes stimulated NK cell cytotoxicity and granzyme B production, up-regulated the expression of inhibitory receptor CD94, and reduced the expression of activating receptors CD69, NKG2D, and NKp44. Importantly, only cancer cells were proved to produce exosomes with Hsp70 exposed on the surface, since these particles isolated from ‘normal’ 3T3 mouse fibroblasts did not contain the chaperone [Citation60].
It is noteworthy that serum Hsp70 has been proposed to be a marker of the efficacy of Hsp90 inhibitors. This assumption is based on numerous observations which have demonstrated the capability of such compounds, geldanamycin, its analogues and many others, to induce the robust synthesis of Hsp70 and its extracellular transport. That the origin of the exogenously occurring chaperone was cancer cells was suspected since this was only found in animals with engrafted tumour and not in non-tumour-bearing mice [Citation61].
The mechanisms which trigger or underlie extracellular transport of Hsp70 remain elusive and it is most probable that there are different strategies used in various cell types and these may depend on a factor stimulating the chaperone export. Some of the ways possibly employed by Hsp70 to leave a living cell are depicted in . It is noteworthy that the release of Hsp70 can occur due to canonical and non-canonical mechanisms of protein transport [Citation62].
Figure 1. Pathways of Hsp70 release from cancer cells and consequences of this exodus for anti-tumour immunity. Hsp70 is able to leave cells using different ‘doors’: inside exosomes and on their membrane (I), with lysosomes (II), using secretory-like pathways (III); Hsp70 could form channels (IV), penetrate through the lipid bilayer (V), or the structure of lipid rafts (VI). When Hsp70 appears outside the cancer cell it modulates anti-tumour immunity in different ways. It triggers (red arrows on the left) anti-tumour immunity through activation of pro-inflammatory cytokine expression (1) and CTL-response. Exogenous Hsp70 can trigger CTL-activity directly (2) or through interaction with APC, mediation of cross-presentation, CTL-programming, DC-licensing and NK-DC cross-talk (3). Immunosuppressive properties of Hsp70 (blue arrows on the right) are associated with enhancement of tolerance of cancer cells (4) and activation of cells with immunosuppressive functions (5).
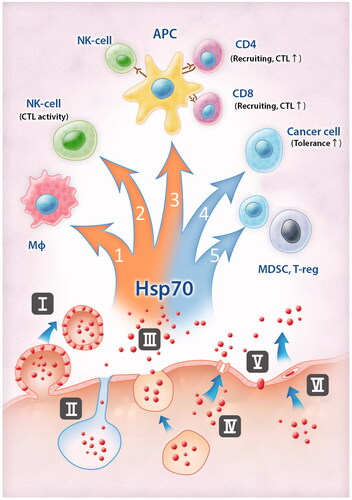
We already mentioned that exosomal transport of Hsp70 can be induced by certain drugs. The fact that exosomes in particular are involved in extracellular transport of Hsp70 was proved by the data of Gastpar and colleagues, who demonstrated that these vesicles possessed all the features of this type of vesicle, floating capacity, acetylcholine esterase activity and specific protein composition [Citation63]. In another study Hsp70 was found to be an obligatory component of exosomes derived from mouse cancer cells, and their release was inhibited by factors suppressing exosomal transport [Citation60]. This route of Hsp70 extracellular transport is essentially employed by tumour cells both in vitro and in vivo [Citation59,Citation64], though exosomes carrying Hsp70 were recently found in endothelial cells [Citation65].
Another mechanism of Hsp70 transport was found to be secretory lysosomes; this was proved by the staining of the surface of cancer cells with antibodies to Hsp70 and to LAMP1 and by inhibitory analysis of lysosomal transport [Citation62]. Very recently, results were published indicating that in B16 mouse melanoma cells with basal level of Hsp70 the transport of Hsp70 to a plasma membrane was mediated by endosomes. In heat stressed cells the content of Hsp70 grew several times and the amount of the chaperone expressed on the cell surface elevated as well. This elevation was due to the accumulation of newly synthesised Hsp70 in lysosomes that were easily released from cells. Since exogenous Hsp70 can efficiently induce innate and/or adaptive immunity, the authors suggested that a simple heat shock (hyperthermia) can be a promising tool to stimulate anti-tumour immune response [Citation66], see .
The ‘classical’ type of Hsp70 export was also found in A-431 human carcinoma cells treated with heat shock or with the specific inhibitor of phospholipase C. The granules carrying Hsp70 were stained with chromogranin A, a marker of secretory granules, and did not carry any markers of lysosomes, lipid bodies or exosomes; the release of Hsp70 was inhibited by brefeldin A [Citation67] . In interleukin-2-treated cells the export of Hsp70 and Tag 7 was shown also blocked by Brefeldin A, suggesting the same mechanism of extracellular transport of the chaperone [Citation68].
During the last decade many groups reported that Hsp70 can be released from cells independently on vesicular structures. First, it was found that the chaperone binds phosphatidylserine suggesting that the interaction can be a prerequisite of the transport of Hsp70 to a cell surface [Citation69]. Similarly, Hsp70 can interact with acidic lipids of the plasma membrane using its ATP- and lipid-binding domains [Citation70]. Interestingly, only acid lipids formed complexes with Hsp70, while neutral glyco- or phospholopids did not. Additionally, pure Hsp70 was found to interact with artificial membranes and form pores, the observation that may be a simple explanation of the protein transport into or from a given cell [Citation71], .
Several groups reported that Hsp70 is associated with cholesterol-rich structures known as lipid rafts and at least in macrophage-like cells this interaction is of particular importance because Hsp70 and some other proteins including Hsp90 and TLR4 take part in the recognition of lipopolysaccharide and in the triggering of NF-κB signalling chain [Citation72]. The extracellular transport of Hsp70 outside a cell via lipid raft was proven by the observation that was inhibited with methyl-β-cyclodextrin destructing raft structures [Citation73], .
Collectively, the data show that the amplitude and mechanism of extracellular transport of Hsp70 may depend upon the type of cancer cell studied, and more importantly on the physiological situation in which the cell may exist. Some of targets of the exogenous Hsp70 are depicted in , and among the most important is surface Hsp70. Such an unusual location of Hsp70 and Hsp90 was first observed on the outer membrane of human melanoma cells by Ferrarini and coauthors [Citation74]. The fact that the surface localisation of Hsp70 is a typical feature of tumour cells was demonstrated by Multfoff with colleagues [Citation75]. Using peptide scanning methods they demonstrated that a fragment of Hsp70 exposed on a cell surface was a 14-mer peptide TKDNNLLGRFELSG (TKD, aa450–463), and this peptide as well as a full-size Hsp70 molecule can efficiently induce cytotoxic response of NK cells. Later, it was found that treated with TKD−CD3−CD56 + CD94 + NK cells were selectively attracted by Hsp70 membrane-positive tumour cells. Interestingly, TKD, mimicking surface-bound Hsp70, initiated migration of NK cells in a concentration-dependent and chemokine-independent manner [Citation76]. A similar 14-mer peptide, TRD, was found in the Hsp70 located on the surface of murine tumour cells [Citation77].
TKD peptide was employed for the generation of cmHsp70.1 monoclonal antibody and the latter was used for the screening of the surface-Hsp70-positive tumours. On the other hand the administration of the antibody was found to trigger antibody-dependent cellular cytotoxicity of membrane Hsp70-positive mouse tumour cells by unstimulated spleen cells [Citation78]. The anti-tumour effect was even more pronounced when interleukin-2 and TKD peptide were added to the antibody. Importantly, the membrane expression of TKD peptide revealed with the aid of cmHsp70.1 antibody was dependent on the cancer cell type and on whether a tumour had been subjected to anti-cancer treatment or not. Stangl and colleagues used a recombinant Fab fragment of cmHsp70.1 antibody to trace the surface-attached Hsp70 in CT26 mouse tumour subjected to ionising irradiation in vivo [Citation79]. It was found that Fab Hsp70 labelled with Cy5.5 dye and intravenously injected was accumulated in tumour and not in healthy tissues (surface Hsp70-negative), suggesting a great potential for Fab Hsp70 as a diagnostic tool in oncology.
Extracellular Hsp70 as a trigger of anti-tumour immunity
Hsp70 released from cancer cells can carry peptide antigens including ones specific for a tumour. This notion is supported by a number of reports stemming from the initial discovery by Srivastava [Citation80]. Generally there are several mechanisms by which the chaperone can contribute to the development of anti-cancer immune response. Firstly, Hsp70 was shown to mediate cross presentation starting from the moment of the delivery of tumour antigens to antigen-presenting cells, such as DC using the mechanism of receptor-mediated endocytosis; at the end of this process the peptides become cross-presented to CD8-positive cytotoxic T cells on major histocompatibility complex (MHC) class I molecules [Citation81], . Although many receptors have been identified to be potential mediators of binding and uptake of Hsp70, [Citation82] it is not yet well understood how Hsp70 interacts with cell-surface receptors on a molecular basis. It also remains elusive whether uptake of HSP-peptide complex and activation of immune/inflammatory signalling are carried out by one receptor or multiple receptors specialised in endocytosis and signalling.
Most immunogenic functions of Hsp70 are related to its C-terminal substrate-binding domain; this part is necessary for interaction with antigen-presenting cells leading to cross-presentation of tumour antigens [Citation83]. It was shown that cytokine production and maturation of DC is also activated by the C-terminal domain (peptide 407–426) of the chaperone molecule [Citation84]. The substrate-binding domain of Hsp70 resembles the peptide-binding domain of MHC class I molecules by the criteria of substrate selection [Citation85], thus suggesting that HSPs can be a proper molecular transporter of antigens for binding to MHC class I proteins.
The discovery of Asea and coauthors that Hsp70 is able to interact with pattern recognition receptors such as Toll-like receptors 2 and 4 (TLR2 and TLR4) and therefore to play a pro-inflammatory role convinced the authors to propose a new term for the chaperone, chaperokine [Citation86,Citation87]. From this work it may also be justified to include Hsp70 released from a tumour as a damage-associated molecular pattern, DAMP. From such activity, Hsp70 can additionally activate the secretion of pro-inflammatory cytokines and expression of co-stimulating molecules on the cell types mentioned above, which is necessary for enhanced programming of cytotoxic lymphocytes [Citation88].
In addition to interaction with DCs and stimulation of CD4+ and CD8+ T lymphocytes in a process of antigen presentation, exposure to Hsp70 modulates immune functions of cells belonging to other types. For example, extracellular Hsp70 was found to activate NK cells using a mechanism similar to that described above. The chaperone can activate NK cells to kill tumour cells expressing MHC class I chain-related molecule A (MICA) in a natural killer group 2 member D (NKG2D) ligand-dependent manner. This NK-activating power was attributed also to the TKD peptide part of Hsp70, and both were shown to induce the expression of the cytotoxic protease granzyme B by NK cells [Citation89]. The combination of Hsp70 and HDAC inhibitor-inducing MICA/B activation enhanced cytotoxicity of NK cells against tumour cells. Further, it was shown that Hsp70-mediated activation of NK cells requires DC-NK cross-talk enabled by NKG2D-MICA interaction. Neutralising antibody against NK activating receptor NKG2D as well as antibody against MICA expressed on DC abrogated the HSP-augmented interferon-γ release. Thus extracellular Hsp70, released from dying cells can be a critical link between NK and DCs in developing immune response against tumours [Citation90].
Recent data evidence that exogenously occurring Hsp70 can also promote the production of anti-inflammatory cytokines and activate cells with immunosuppressive function such as FoxP+ regulatory cells (Tregs) and myeloid-derived suppressor cells (MDSC). MDSCs were identified as a population of immature myeloid cells with the ability to suppress T cell activation. Mouse MDSCs are established to express cell-surface antigens Ly-6 C/G and CD11b, and in humans they are typically CD11b+/CD33+/HLA−/DR− [Citation91]. Chalmin and coauthors showed that Hsp70 released from mouse tumour cells in exosomes triggered STAT3 activation in a TLR2/MyD88-dependent manner [Citation60]. Similarly, Hsp70-containing exosomes from human cancer patients were also found to activate MDSCs in the same regulatory mode. Suppressive potential of Hsp70-containing exosomes was strongly reduced when cancer cells from mouse models or from patients were treated with amiloride known to inhibit exosomal transport. Another study was performed in order to reveal the direct effect of pure Hsp70 on CD4+CD25+FoxP3+ regulatory cells (Tregs). Tregs were shown to suppress T-cell proliferation in vitro, they may also inhibit immune responses to autoantigens, alloantigens, tumour antigens and infectious agents in vivo [Citation92]. It was found that the Tregs treated with pure recombinant Hsp70 inhibited proliferation of target cells and reduced the secretion of interferon-gamma and of tumour necrosis factor. At the same time the chaperone increased the secretion of suppressive interleukin-10 and TGF-beta and the ability of Tregs to neutralise CD4+ cell-mediated activity [Citation93]. These results correlate well with data of other authors who have demonstrated Treg-activating function exhibited by extracellular Hsp70 in the process of inflammation. Notably, incubation with microbial Hsp70 was found to induce tolerogenic DC which could induce Tregs, irrespective of the antigen they presented [Citation94].
Exogenous Hsp70 and cancer cell tolerance
Extracellular Hsp70 may have some other functions outside the regulation of immune response since it was found to penetrate inside living cells or to activate various receptors on its own or using transactivation mechanisms. Clearly, when the chaperone enters a cell it can substituted for an intracellular analogue and contribute to that ongoing activity. For example, such penetration was shown earlier to rescue neuroblastoma cells from the cytotoxic effects of hyperthermia and apoptosis-inducing factor staurosporine [Citation42]. Indeed, the protective power of circulating Hsp70 is low since its concentration in serum was reported not to exceed hundreds of nanograms; however in case of brain or articular fluid the value can be higher and reach tens of micrograms, a level that can be effective in the protection of adjacent cells.
Another mechanism that can be employed by extracellular Hsp70 is the triggering of receptor-mediated signalling. Several examples of such activity are worthy of mention. First, Hsp70 released from A-431 human carcinoma cells as a consequence of heat stress was found to act as a ligand for TLR2/4, the event followed by transactivation of EGF receptor and subsequent activation of MAP kinase signal cascade [Citation95]. The data of another study show that Hsp70 introduced in the culture of H22 hepatocarcinoma cells elevated their proliferation via activation of TLR2/4 and NF-κB-regulated transcription [Citation96]. These results as well as other data contribute to the hypothesis that exogenous Hsp70 may serve as a non-specific danger signal for a population of cancer cells subjected to a certain stressor [Citation97].
Hsp70-based anti-tumour vaccine
The main conclusion from earlier sections of this review supports the idea that tumour cell Hsp70 can occur in an extracellular space where it can stimulate an anti-tumour immune response. The application of HSPs for stimulation of adaptive and innate components of immune response has led researchers to develop different methods of increasing membrane-bound Hsp70 expression and its secretion into the extracellular space thus rendering cancer cells more susceptible to immune cells. In general, two approaches were studied: 1) application of certain pharmacological agents, including cytostatic drugs, alkyl-lysophospholipids, cyclo-oxigenase 1/2 inhibitors, acetylsalicylic acid and insulin-sensitiser pioglitazone [Citation71]; 2) use of physical factors, including hyperthermia, photodynamic therapy (PDT), ionising irradiation [Citation78]. Recent data indicate that PDT possesses several advantages since it results in long-term tumour cure when choosing the appropriate dose of the treatment to cause the release of a high level of Hsp70 [Citation55,Citation98]. An up-to-date approach in the treatment by physical factors is the combination of different procedures, for instance radiation and hyperthermia [Citation57] or PDT and hyperthermia [Citation99]. It is worth mentioning that all physical methods of anti-cancer therapy have limitations because of the great variation in the individual dose of treatment required to induce Hsp70’s immunostimulatory potential, and of the great difference in immune response between animal models and humans. It appears that the development of more reliable sources of exogenous Hsp70 for the stimulation of the immune system is needed. An interesting example of such continuous supply was demonstrated by Massa and coworkers employing tumour cells engineered to secrete Hsp70 [Citation100]. In several studies DNA vaccines were shown to generate significant immune responses with efficient tumour regression [Citation101,Citation102]. Clinically relevant data were obtained by several groups working on the individual anti-cancer vaccine constituting Hsp70 or GP96 isolated from a tumour and subjected to purification in order to preserve the complex of a chaperone with tumour peptides [Citation80]. The vaccine based on Hsp70 isolated from a tumour combined with Gleevec (also known as Glivec) is on the phase II clinical trials (www.clinicaltrials.gov). Calderwood and coworkers proposed another elegant design of individual anti-cancer vaccine comprising the hybrid of tumour and DC (Hsp70.PC-F); this construct could induce potent anti-tumour immunity [Citation103]. Several studies in which intratumorally or intravenously injected Hsp70 alone or in combination with other factors showed promising results in tumour regression. Some of them are presented in . Intratumoral delivery of Hsp70 in the model of subcutaneous B16 mouse melanoma led to the delay in tumour progression that was significantly enhanced by hyperthermia using magnetic nanoparticles; inhibition of tumour growth was presumably due to an elevation of specific immune response as follows from the data on interferon-γ production [Citation104]. Geng and colleagues showed that the systemic injection of autologous Hsp70 isolated from B16F10 melanoma coupled with the plasmid encoding protein inhibitor of anti-tumour response led to a two-fold increase of survival accompanied by the emphasised immune response [Citation101]. Using Hsp70 isolated from T cell lymphoma and S-180 mouse sarcoma, Kumar and coauthors found that this vaccine induced more than 100% increase of survival rate and almost 90% reduction of the rate of tumour growth [Citation105]. Lastly, in our study the application of hydrogel with soluble Hsp70 on the surface of a B16 melanoma tumour resulted in the tumour growth reducing by half, which was concomitant with the notable elevation of tumour-specific cytotoxicity and interferon-γ production [Citation106]. In conclusion, these data demonstrate the prospect of therapeutic vaccines based on Hsp70 administration at least for the treatment of difficult to treat oncological patients.
Table I. Immunotherapeutic effect of antitumour therapy based on Hsp70.
Conclusion
Hsp70 is a typical Janus-like protein and plays a dual role in cancer cells: occurring inside a cell it protects it from a variety of anti-tumour factors, while exogenously the chaperone coordinates immune response to cancer cells. This phenomenon was proved by a great number of data obtained using tumour models in vitro and in vivo. The development of new methods for the measuring of circulating Hsp70 concentration resulted in data proving that the high level of exogenous Hsp70 in sera of patients with leukaemia, colorectal cancer, glioblastoma and pancreatic cancer corresponds to a poor prognosis for humans. This statement is in contradiction with Srivastava’s paradigm [80] and numerous papers convinced of the extracellular Hsp70 possesses anti-cancer immunogenic potential. Although the data concerning various functions of the exogenous chaperone are thoroughly discussed in this review, we cannot yet explain the above contradiction on the basis of current knowledge and believe that newer results will clarify the situation and be included in future reviews.
Declaration of interest
This work was supported by a grant from the Molecular and Cellular Biology programme of the Russian Academy of Sciences. The authors alone are responsible for the content and writing of the paper.
References
- Hartl FU, Hayer-Hartl M. Molecular chaperones in the cytosol: From nascent chain to folded protein. Science 2002;295:1852–8
- Young JC, Barral JM, Ulrich Hartl F. More than folding: Localized functions of cytosolic chaperones. Trends Biochem Sci 2003;28:541–7
- Asea A. Initiation of the Immune Response by Extracellular Hsp72: Chaperokine Activity of Hsp72. Curr Immunol Rev 2006;2:209–15
- Li GC, Mivechi NF, Weitzel G. Heat shock proteins, thermotolerance, and their relevance to clinical hyperthermia. Int J Hyperthermia 1995;11:459–88
- Ciocca DR, Calderwood SK. Heat shock proteins in cancer: Diagnostic, prognostic, predictive, and treatment implications. Cell Stress Chaperones 2005;10:86–103
- Powers MV, Jones K, Barillari C, Westwood I, van Montfort RL, Workman P. Targeting HSP70: The second potentially druggable heat shock protein and molecular chaperone? Cell Cycle 2010;9:1542–50
- Chuma M, Sakamoto M, Yamazaki K, Ohta T, Ohki M, Asaka M, et al. Expression profiling in multistage hepatocarcinogenesis: Identification of Hsp70 as a molecular marker of early hepatocellular carcinoma. Hepatology 2003;37:198–207
- Syrigos KN, Harrington KJ, Karayiannakis AJ, Sekara E, Chatziyianni E, Syrigou EI, et al. Clinical significance of heat shock protein-70 expression in bladder cancer. Urology 2003;61:677–80
- Hwang TS, Han HS, Choi HK, Lee YJ, Kim YJ, Han MY, et al. Differential, stage-dependent expression of Hsp70, Hsp110 and Bcl-2 incolorectal cancer. J Gastroenterol Hepatol 2003;18:690–700
- Novoselov SS, Verbova MV, Vasil'eva EV, Vorob'eva NK, Margulis, BA, Guzhova IV. Expression of Hsp70 and Hdj1 chaperone proteins in human tumor cells. Vopr Onkol 2004;50:174–8
- Nylandsted J, Rohde M, Brand K, Bastholm L, Elling F, Jäättelä M. Selective depletion of heat shock protein 70 (Hsp70) activates a tumor-specific death program that is independent of caspases and bypasses Bcl-2. Proc Natl Acad Sci USA 2000;97:7871–6
- Calderwood SK, Khaleque MA, Sawyer DB, Ciocca DR. Heat shock proteins in cancer: Chaperones of tumorigenesis. Trends Biochem Sci 2006;31:164–72
- Saleh A, Srinivasula SM, Balkir L, Robbins PD, Alnemri ES. Negative regulation of the Apaf-1 apoptosome by Hsp70. Nat Cell Biol 2000;2:476–83
- Beere HM, Wolf BB, Mosser DD, Mahboubi A, Kuwana T, Tailor P, et al. Heat-shock protein 70 inhibits apoptosis by preventing recruitment of procaspase-9 to the Apaf-1 apoptosome. Nat Cell Biol 2000;2:469–75
- Stankiewicz AR, Lachapelle G, Foo CP, Radicioni SM, Mosser DD. Hsp70 inhibits heat-induced apoptosis upstream of mitochondria by preventing Bax translocation. J Biol Chem 2005;280:38729–39
- Ruchalski KL, Mao H, Li Z, Wang Z, Gillers S, Wang Y, et al. Distinct Hsp70 domains mediate apoptosis inducing factor release and nuclear accumulation. J Biol Chem 2006;281:7873–80
- Cande C, Cohen I, Daugas E, Ravagnan L, Larochette N, Zamzami N, et al. Apoptosis-inducing factor (AIF): A novel caspase-independent death effector released from mitochondria. Biochimie 2002;84:215–22
- Komarova EY, Afanasyeva EA, Bulatova MM, Cheetham ME, Margulis BA, Guzhova IV. Downstream caspases are novel targets for the anti-apoptotic activity of the molecular chaperone Hsp70. Cell Stress Chaperones 2004;9:265–75
- Jäättelä M. Overexpression of major heat shock protein hsp70 inhibits tumor necrosis factor-induced activation of phospholipase A2. J Immunol 1993;151:4286–94
- Mosser DD, Caron AW, Bourget L, Meriin AB, Sherman MY, Morimoto RI, et al. The chaperone function of hsp70 is required for protection against stress-induced apoptosis. Mol Cell Biol 2000;20:7146–59
- Nylandsted J, Gyrd-Hansen M, Danielewicz A, Fehrenbacher N, Lademann, U, Hoyer-Hansen M, et al. Heat shock protein 70 promotes cell survival by inhibiting lysosomal membrane permeabilization. J Exp Med 2004;200:425–35
- Kroemer G, Jäättelä M. Lysosomes and autophagy in cell death control. Nat Rev Cancer 2005;5:886–97
- Yaglom JA, Gabai VL, Sherman MY. High levels of heat shock protein Hsp72 in cancer cells suppress default senescence pathways. Cancer Res 2007;67:2373–81
- Meng L, Hunt C, Yaglom JA, Gabai VL, Sherman MY. Heat shock protein Hsp72 plays an essential role in Her2-induced mammary tumorigenesis. Oncogene 2011;30:2836–45
- Akerfelt M, Morimoto RI, Sistonen L. Heat shock factors: Integrators of cell stress, development and lifespan. Nat Rev Mol Cell Biol 2010;11:545–55
- Nagai N, Nakai A, Nagata K. Quercetin suppresses heat shock response by down regulation of HSF1. Biochem Biophys Res Commun 1995;208:1099–105
- Powers MV, Workman P. Inhibitors of the heat shock response: Biology and pharmacology. FEBS Lett 2007;581:3758–69
- Yokota S, Kitahara M, Nagata K. Benzylidene lactam compound, KNK437, a novel inhibitor of acquisition of thermotolerance and heat shock protein induction in human colon carcinoma cells. Cancer Res 2000;60:2942–8
- Westerheide SD, Kawahara TL, Orton K, Morimoto RI. Triptolide, an inhibitor of the human heat shock response that enhances stress-induced cell death. J Biol Chem 2006;281:9616–22
- Sahin E, Sahin M, Sanlioğlu AD, Gümüslü S. KNK437, a benzylidene lactam compound, sensitises prostate cancer cells to the apoptotic effect of hyperthermia. Int J Hyperthermia 2011;27:63–73
- Song X, Chen Z, Wu C, Zhao S. Abrogating HSP response augments cell death induced by As2O3 in glioma cell lines. Can J Neurol Sci 2010;37:504–11
- Mamelak D, Lingwood C. The ATPase domain of hsp70 possesses a unique binding specificity for 3′-sulfogalactolipids. J Biol Chem 2001;276:449–56
- Rodina A, Vilenchik M, Moulick K, Aguirre J, Kim J, Chiang A, et al. Selective compounds define Hsp90 as a major inhibitor of apoptosis in small-cell lung cancer. Nat Chem Biol 2007;3:498–507
- Braunstein MJ, Scott SS, Scott CM, Behrman S, Walter P, Wipf P, et al. Antimyeloma effects of the heat shock protein 70 molecular chaperone inhibitor MAL3-101. J Oncol 2011;2011:232037
- Wisén S, Bertelsen EB, Thompson AD, Patury S, Ung P, Chang L, et al. Binding of a small molecule at a protein–protein interface regulates the chaperone activity of hsp70–hsp40. ACS Chem Biol 2010;5:611–22
- Leu JI, Pimkina J, Frank A, Murphy ME, George DL. A small molecule inhibitor of inducible heat shock protein 70. Mol Cell 2009;36:15–27
- Goloudina AR, Demidov ON, Garrido C. Inhibition of HSP70: A challenging anti-cancer strategy. Cancer Lett 2012;325:117–24
- Leu JI, Pimkina J, Pandey P, Murphy ME, George DL. HSP70 inhibition by the small-molecule 2-phenylethynesulfonamide impairs protein clearance pathways in tumor cells. Mol Cancer Res 2011;9:936–47
- Schmitt E, Maingret L, Puig PE, Rerole AL, Ghiringhelli F, Hammann A, et al. Heat shock protein 70 neutralization exerts potent antitumor effects in animal models of colon cancer and melanoma. Cancer Res 2006;66:4191–7
- Tytell M, Greenberg SG, Lasek RJ. Heat shock-like protein is transferred from glia to axon. Brain Res 1986;363:161–4
- Hightower LE, Guidon PT Jr. Selective release from cultured mammalian cells of heat-shock (stress) proteins that resemble glia-axon transfer proteins. J Cell Physiol 1989;138:257–66
- Guzhova I, Kislyakova K, Moskaliova O, Fridlanskaya I, Tytell M, Cheetham M, et al. In vitro studies show that Hsp70 can be released by glia and that exogenous Hsp70 can enhance neuronal stress tolerance. Brain Res 2001;914:66–73
- Yeh CH, Tseng R, Zhang Z, Cortes J, O'Brien S, Giles F, et al. Circulating heat shock protein 70 and progression in patients with chronic myeloid leukemia. Leuk Res 2009;33:212–7
- Yeh CH, Tseng R, Hannah A, Estrov Z, Estey E, Kantarjian H, et al. Clinical correlation of circulating heat shock protein 70 in acute leukemia. Leuk Res 2010;34:605–9
- Dempsey NC, Leoni F, Ireland HE, Hoyle C, Williams JH. Differential heat shock protein localization in chronic lymphocytic leukemia. J Leukoc Biol 2010;87:467–76
- Nylandsted J. Extracellular heat shock protein 70: A potential prognostic marker for chronic myeloid leukemia. Leuk Res 2009;33:205–6
- Kocsis J, Madaras B, Tóth EK, Füst G, Prohászka Z. Serum level of soluble70-kD heat shock protein is associated with high mortality in patients with colorectal cancer without distant metastasis. Cell Stress Chaperones 2010;15:143–51
- Elstner A, Stockhammer F, Nguyen-Dobinsky TN, Nguyen QL, Pilgermann I, Gill A, et al. Identification of diagnostic serum protein profiles of glioblastoma patients. J Neurooncol 2011;102:71–80
- Dutta SK, Girotra M, Singla M, Dutta A, Otis Stephen F, Nair PP, et al. Serum HSP70: A novel biomarker for early detection of pancreatic cancer. Pancreas 2012;41:530–4
- Sherman M, Multhoff G. Heat shock proteins in cancer. Ann N Y Acad Sci 2007;1113:192–201
- Rérole AL, Jego G, Garrido C. Hsp70: Anti-apoptotic and tumorigenic protein. Methods Mol Biol 2011;787:205–30
- Murshid A, Gong J, Stevenson MA, Calderwood SK. Heat shock proteins and cancer vaccines: Developments in the past decade and chaperoning in the decade to come. Expert Rev Vaccines 2011;10:1553–68
- Satoh M, Shimoda Y, Akatsu T, Ishikawa Y, Minami Y, Nakamura M. Elevated circulating levels of heat shock protein 70 are related to systemic inflammatory reaction through monocyte toll signal in patients with heart failure after acute myocardial infarction. Eur J Heart Fail 2006;8:810–5
- Molvarec A, Rigo J Jr, Lazar L, Balogh K, Mako V, Cervenak L, et al. Increased serum heat-shock protein 70 levels reflect systemic inflammation, oxidative stress and hepatocellular injury in preeclampsia. Cell Stress Chaperones 2009;14:151–9
- Mitra S, Giesselman BR, Jesus-Andrino FJ, Foster TH. Tumor response to mTHPC-mediated photodynamic therapy exhibits strong correlation with extracellular release of Hsp70. Lasers Surg Med 2011;43:632–43
- Hurwitz MD, Kaur P, Nagaraja GM, Bausero MA, Manola J, Asea A. Radiation therapy induces circulating serum Hsp72 in patients with prostate cancer. Radiother Oncol 2010;95:350–8
- Schildkopf P, Frey B, Ott OJ, Rubner Y, Multhoff G, Sauer R, et al. Radiation combined with hyperthermia induces HSP70-dependent maturation of dendritic cells and release of pro-inflammatory cytokines by dendritic cells and macrophages. Radiother Oncol 2011;101:109–15
- Jensen H, Andresen L, Hansen KA, Skov S. Cell-surface expression of Hsp70 on hematopoietic cancer cells after inhibition of HDAC activity. J Leukoc Biol 2009;86:923–32
- Lv LH, Wan YL, Lin Y, Zhang W, Yang M, Li GL, et al. Anticancer drugs cause release of exosomes with heat shock proteins from human hepatocellular carcinoma cells that elicit effective natural killer cell antitumor responses in vitro. J Biol Chem 2012;287:15874–85
- Chalmin F, Ladoire S, Mignot G, Vincent J, Bruchard M, Remy-Martin JP, et al. Membrane-associated Hsp72 from tumor-derived exosomes mediates STAT3-dependent immunosuppressive function of mouse and human myeloid-derived suppressor cells. J Clin Invest 2010;120:457–71
- Dakappagari N, Neely L, Tangri S, Lundgren K, Hipolito L, Estrellado A, et al. An investigation into the potential use of serum Hsp70 as a novel tumour biomarker for Hsp90 inhibitors. Biomarkers 2010;15:31–8
- Mambula SS, Stevenson MA, Ogawa K, Calderwood SK. Mechanisms for Hsp70 secretion: Crossing membranes without a leader. Methods 2007;43:168–75
- Gastpar R, Gehrmann M, Bausero MA, Asea A, Gross C, Schroeder JA, et al. Heat shock protein 70 surface-positive tumor exosomes stimulate migratory and cytolytic activity of natural killer cells. Cancer Res 2005;65:5238–47
- Radons J, Multhoff G. Immunostimulatory functions of membrane-bound and exported heat shock protein 70. Exerc Immunol Rev 2005;11:17–33
- Zhan R, Leng X, Liu X, Wang X, Gong J, Yan L, et al. Heat shock protein 70 is secreted from endothelial cells by a non-classical pathway involving exosomes. Biochem Biophys Res Commun 2009;387:229–33
- Juhász K, Thuenauer R, Spachinger A, Duda E, Horváth I, Vígh L, et al. Lysosomal rerouting of hsp70 trafficking as a potential immune activating tool for targeting melanoma. Curr Pharm Des 2013;19:430–40
- Evdonin AL, Martynova MG, Bystrova OA, Guzhova IV, Margulis BA, Medvedeva ND. The release of Hsp70 from A431 carcinoma cells is mediated by secretory-like granules. Eur J Cell Biol 2006;85:443–5
- Sashchenko LP, Dukhanina EA, Yashin DV, Shatalov YV, Romanova EA, Korobko EV, et al. Peptidoglycan recognition protein Tag7 forms a cytotoxic complex with heat shock protein 70 in solution and in lymphocytes. J Biol Chem 2004;279:2117–24
- Arispe N, Doh M, Simakova O, Kurganov B, De Maio A. Hsc70 and Hsp70 interact with phosphatidylserine on the surface of PC12 cells resulting in a decrease of viability. FASEB J 2004;18:1636–45
- Harada Y, Sato C, Kitajima K. Complex formation of 70-kDa heat shock protein with acidic glycolipids and phospholipids. Biochem Biophys Res Commun 2007;353:655–60
- Arispe N, Doh M, De Maio A. Lipid interaction differentiates the constitutive and stress-induced heat shock proteins Hsc70 and Hsp70. Cell Stress Chaperones 2002;7:330–8
- Triantafilou M, Triantafilou K. Heat-shock protein 70 and heat-shock protein 90 associate with Toll-like receptor 4 in response to bacterial lipopolysaccharide. Biochem Soc Trans 2004;32:636–9
- Bausero MA, Gastpar R, Multhoff G, Asea A. Alternative mechanism by which IFN-gamma enhances tumor recognition: Active release of heat shock protein 72. J Immunol 2005;175:2900–12
- Ferrarini M, Heltai S, Zocchi MR, Rugarli C. Unusual expression and localization of heat-shock proteins in human tumor cells. Int J Cancer 1992;51:613–9
- Multhoff G, Botzler C, Wiesnet M, Müller E, Meier T, Wilmanns W, et al. A stress-inducible 72-kDa heat-shock protein (HSP72) is expressed on the surface of human tumor cells, but not on normal cells. Int J Cancer 1995;61:272–9
- Gastpar R, Gross C, Rossbacher L, Ellwart J, Riegger J, Multhoff G. The cell surface-localized heat shock protein 70 epitope TKD induces migration and cytolytic activity selectively in human NK cells. J Immunol 2004;172:972–80
- Zhang H, Liu R, Huang W. A 14-mer peptide from HSP70 protein is the critical epitope which enhances NK activity against tumor cells in vivo. Immunol Invest 2007;36:233–46
- Stangl S, Themelis G, Friedrich L, Ntziachristos V, Sarantopoulos A, Molls M, et al. Detection of irradiation-induced, membrane heat shock protein 70 (Hsp70) in mouse tumors using Hsp70 Fab fragment. Radiother Oncol 2011;99:313–6
- Stangl S, Gehrmann M, Riegger J, Kuhs K, Riederer I, Sievert W, et al. Targeting membrane heat-shock protein 70 (Hsp70) on tumors by cmHsp70.1 antibody. Proc Natl Acad Sci USA 2011;108:733–8
- Srivastava PK. Immunotherapy for human cancer using heat shock protein-peptide complexes. Curr Oncol Rep 2005;7:104–8
- Noessner E, Gastpar R, Milani V, Brandl A, Hutzler PJ, Kuppner MC, et al. Tumor-derived heat shock protein 70 peptide complexes are cross-presented by human dendritic cells. J Immunol 2002;169:5424–32
- Murshid A, Theriault J, Gong J, Calderwood SK. Investigating receptors for extracellular heat shock proteins. Methods Mol Biol 2011;787:289–302
- MacAry PA, Javid B, Floto RA, Smith KG, Oehlmann W, Singh M, et al. HSP70 peptide binding mutants separate antigen delivery from dendritic cell stimulation. Immunity 2004;20:95–106
- Wang Y, Whittall T, McGowan E, Younson J, Kelly C, Bergmeier LA, et al. Identification of stimulating and inhibitory epitopes within the heat shock protein 70 molecule that modulate cytokine production and maturation of dendritic cells. J Immunol 2005;174:3306–16
- Grossmann ME, Madden BJ, Gao F, Pang YP, Carpenter JE, McCormick D, et al. Proteomics shows Hsp70 does not bind peptide sequences indiscriminately in vivo. Exp Cell Res 2004;297:108–17
- Asea A, Rehli M, Kabingu E, Boch JA, Bare O, Auron PE, et al. Novel signal transduction pathway utilized by extracellular HSP70: role of toll-like receptor (TLR) 2 and TLR4. J Biol Chem 2002;277:15028–34
- Asea A. Initiation of the immune response by extracellular Hsp72: Chaperokine activity of Hsp72. Curr Immunol Rev 2006;2:209–15
- Calderwood SK, Murshid A, Gong J. Heat shock proteins: Conditional mediators of inflammation in tumor immunity. Front Immunol. 2012;3:75
- Elsner L, Flügge PF, Lozano J, Muppala V, Eiz-Vesper B, Demiroglu SY, et al. The endogenous danger signals HSP70 and MICA cooperate in the activation of cytotoxic effector functions of NK cells. J Cell Mol Med 2010;14:992–1002
- Qiao Y, Liu B, Li Z. Activation of NK cells by extracellular heat shock protein 70 through induction of NKG2D ligands on dendritic cells. Cancer Immun 2008;8:12
- Ostrand-Rosenberg S, Sinha P. Myeloid-derived suppressor cells: Linking inflammation and cancer. J Immunol 2009;182:4499–506
- Geiger TL, Tauro S. Nature and nurture in Foxp3(+) regulatory T cell development, stability, and function. Hum Immunol 2012;73:232–9
- Wachstein J, Tischer S, Figueiredo C, Limbourg A, Falk C, Immenschuh S, et al. HSP70 enhances immunosuppressive function of CD4(+)CD25(+)FoxP3(+) T regulatory cells and cytotoxicity in CD4(+)CD25(−) T cells. PLoS One 2012;7:e51747
- Borges TJ, Wieten L, van Herwijnen MJ, Broere F, van der Zee R, Bonorino C, et al. The anti-inflammatory mechanisms of Hsp70. Front Immunol 2012;3:95
- Evdonin AL, Guzhova IV, Margulis BA, Medvedeva ND. Extracellular heat shock protein 70 mediates heat stress-induced epidermal growth factor receptor transactivation in A431 carcinoma cells. FEBS Lett 2006;580:6674–8
- Wu FH, Yuan Y, Li D, Liao SJ, Yan B, Wei JJ, et al. Extracellular HSPA1A promotes the growth of hepatocarcinoma by augmenting tumor cell proliferation and apoptosis-resistance. Cancer Lett 2012;317:157–64
- Calderwood SK, Mambula SS, Gray PJ Jr. Extracellular heat shock proteins in cell signaling and immunity. Ann N Y Acad Sci 2007;1113:28–39
- Merchant S, Korbelik M. Heats shock protein 70 is acute phase reactant: Response elicited by tumor treatment with photodynamic therapy. Cell Stress Chaperones 2011;16:153–62
- He Y, Ge H, Li S. Haematoporphyrin based photodynamic therapy combined with hyperthermia provided effective therapeutic vaccine effect against colon cancer growth in mice. Int J Med Sci 2012;9:627–33
- Massa C, Guiducci C, Arioli I, Parenza M, Colombo MP, Melani C. Enhanced efficacy of tumor cell vaccines transfected with secretable hsp70. Cancer Res 2004 Feb 15;64:1502–8
- Geng H, Zhang G-M, Xiao H, Yuan Y, Li D, Zhang H, et al. HSP70 vaccine in combination with gene therapy with plasmid DNA encoding sPD-1 overcomes immune resistance and suppresses the progression of pulmonary metastatic melanoma. Int J Cancer 2006;118:2657–64
- Chang C-L, Tsai Y-C, He L, Wu T-C, Hung C-F. Cancer immunotherapy using irradiated tumor cells secreting heat shock protein 70. Cancer Res 2007;67:10047–57
- Gong J, Zhu B, Murshid A, Adachi H, Song B, Lee A, et al. T Cell activation by heat shock protein 70 vaccine requires TLR signaling and scavenger receptor expressed by endothelial cells-1. J Immunol 2009;183:3092–8
- Ito A, Matsuoka F, Honda H, Kobayashi T. Atitumor effects of combined therapy of recombinant heat shock protein 70 and hyperthermia using magnetic nanoparticles in an experimental subcutaneous murine melanoma. Cancer Immunol Immunother 2004;53:26–32
- Kumar S, Deepak P, Acharya A. Autologous Hsp70 immunization induces anti-tumor immunity and increases longevity and survival of tumor-bearing mice. Neoplasma 2009;56:259–68
- Abkin SV, Pankratova KM, Komarova EY, Guzhova IV, Margulis BA. Hsp70 chaperone-based gel composition as a novel immunotherapeutic anti-tumor tool. Cell Stress Chaperones 2012. doi 10.1007/s12192-012-0391-x