Abstract
Cancer stem-like cells (CSCs)/tumour-initiating cells (TICs) are defined as the small population of cancer cells that have stem cell-like phenotypes and high capacity for tumour initiation. These cells may have a huge impact in the field of cancer therapy since they are extremely resistant to standard chemoradiotherapy and thus are likely to be responsible for disease recurrence after therapy. Therefore, extensive efforts are being made to elucidate the pathological and molecular properties of CSCs/TICs and, with this information, to establish efficient anti-CSC/TIC targeting therapies. This review considers recent findings on stress response genes that are preferentially expressed in CSCs/TICs and their roles in tumour-promoting properties. Implications for a novel therapeutic strategy targeting CSCs/TICs are also discussed.
Characteristics of cancer stem-like cells
Tumours are composed of morphologically and functionally heterogeneous cells. In the cancer stem cell hypothesis, it is proposed that the heterogeneity occurs as a result of differentiation of a small stem cell-like population of cancer cells (cancer stem-like cells). They are presumed to have properties similar to normal stem cells such as self-renewal capacity, pluripotency and longevity. It is known that they use the same signalling pathways as those found in normal stem cells and embryonic stem cells such as Wnt, Notch and Hedgehog [Citation1,Citation2]. However, in addition to these stem-like features, they have two major malignant characteristics that are responsible for tumour recurrence and metastasis.
The first is their high tumour-initiating capacity. As described in a number of reports, only 10 ∼ 100 CSCs/TICs can form a tumour within several weeks after transplantation into immunodeficient mice such as NOD/SCID mice. The resulting tumour recomposes phenotypically heterogeneous tumour cells resembling the original tumour tissue. Therefore, CSCs are designated as TICs in terms of tumour-forming capacity. One valuable technique in which the tumour-initiating capacity seen in vivo can be modelled in vitro is use of the sphere-forming assay. Multicellular spheres that are formed by breast CSCs and prostate CSCs are termed “mammospheres” and “prostaspheres”, respectively [Citation2,Citation3], when they are cultured under certain conditions. The sphere formation assay is currently used to enrich CSCs/TICs in vitro.
A second malignant property of CSCs is their high resistance to stress. CSCs/TICs are resistant to chemotherapeutic drugs, irradiation and other stresses such as hypoxia and glucose starvation [Citation4,Citation5]. The mechanisms by which CSCs/TICs acquire the stress resistance have been explained in terms of expression of drug transporters, G0 arrest of the cell cycle, and resistance to apoptosis [Citation6]. These properties are derived, in part, from parental normal stem/progenitor cells from which cancer cells originate. However, it is likely that CSCs/TICs might gain a specific anti-stress programme, which is observed only in the testis among normal adult tissues, as described in the following sections. The stress resistance is used for isolation of CSCs/TICs in vitro from several kinds of cancer, including breast cancer, lung cancer and ovarian cancer [Citation7,Citation8].
Genes preferentially expressed in CSCs/TICs
To better understand the molecular basis for these malignant characteristics of CSCs/TICs, we isolated them from various human cancer cell lines by side population (SP) assay () and ALDEFLUOR (Stem Cell Technologies, Vancouver, Canada) assay (), which are based on the high activity of ATP-binding cassette (ABC) transporters [Citation9] and aldehyde dehydrogenase 1 (ALDH1), respectively, in CSCs/TICs. The specific methods are described in the figure legends and our previous reports [Citation10,Citation11–14]. CSCs/TICs were enriched in the SP and/or ALDH1-high population and subjected to comparative transcriptome analysis using a DNA microarray and quantitative RT-PCR.
Figure 1. Hoechst33342 staining assay that shows CSC-enriched side population. Ovarian carcinoma MCAS cells were stained with 5.0 µg/mL Hoechst 33342 and analysed by flow cytometry. (A) SP, side population (2.2%); MP, main population. (B) SP was decreased in the presence ABCG2 inhibitor verapamil.
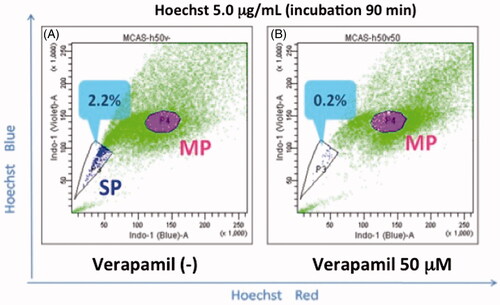
Figure 2. ALDEFLUOR assay revealed CSC-enriched ALDH1-positive population. ALDEFLUOR assay kit was purchased from Stem Cell Technologies (Vancouver, BC, Canada). MCAS cells were suspended in 1 mL assay buffer containing 1.5 mM of an ALDH1 substrate, BODIPY®-aminoacetaldehyde and analysed by flow cytometry. (A) ALDH1-high population (8.3%). (B) ALDH1-high population disappeared in the presence of 10-fold molar excess of diethylamino-benzaldehyde (DEAB).
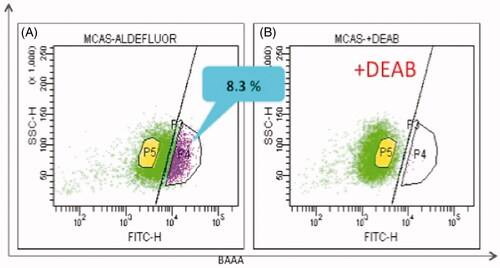
CSC/TIC-preferential genes that were identified in colon, lung, kidney, prostate, uterine and ovarian cancer cells were classified into four categories (). It was found that CSCs/TICs expressed higher levels of 1) stemness-associated genes, 2) stress-resistance genes, and 3) testis-specific genes. Stemness-associated genes are well-known genes that are expressed in embryonic stem cells and normal stem/progenitor cells, and have crucial roles for the maintenance of stemness properties such as self-renewal capacity and pluripotency [Citation10]. Most of the stress-resistance genes are also expressed in normal stem/progenitor cells. For example, ABC transporters are expressed in normal haematopoietic stem cells as well [Citation15]. It is likely that the stress-resistance properties of CSCs/TICs might, in part, originate from normal parental stem/progenitor cells. However, it is interesting that certain stress response genes are exclusively expressed in CSCs/TICs and the testis, which belong to the third group, testis-specific genes [Citation16]. Some of the cancer-expressing testis-specific gene products are termed cancer/testis antigens and have significant roles in the field of tumour immunology since they can serve as immunodominant tumour-rejection antigens [Citation17]. We have reported recently that certain cancer/testis antigens are preferentially expressed in SP cells; therefore designating them cancer/testis/stem (CTS) antigens [Citation16]. The CTS antigens are potent molecular targets for CSC-targeted immunotherapy. It is enigmatic why a number of testis-specific genes are preferentially expressed in non-testicular CSCs/TICs. We speculate that there should be a close similarity between CSCs/TICs and spermatogonial cells. It is likely that CSCs/TICs utilise a specific machinery of spermatogonial stem cells for their unlimited proliferation capacity, longevity and stress resistance.
Table I. A list of genes preferentially expressed in CSCs/TICs of solid cancers.
Stress-resistance genes expressed in CSCs/TICs
Stress-resistance genes expressed in CSCs/TICs can be categorised into the following groups.
ABC transporter genes
ABC transporters are located in the plasma membrane and export certain chemicals and metabolites out of cells, thus protecting the cells from their toxic effects [Citation9]. In addition, they are induced upon stress such as heat shock through their promoter activation and associated with thermosensitivity of tumour cells [Citation18]. Among the ABC transporters, ABCG2 (breast cancer resistance protein, BCRP) and ABCB1 (multidrug resistance 1, MDR1) are preferentially expressed in CSCs/TICs [Citation19]. They are also expressed in normal haematopoietic progenitors and neural stem cells as well as the placenta and blood–testis barrier, protecting them from harmful toxins [Citation9,Citation15,Citation20,Citation21]. Since fluorescent dyes such as Hoechst 33342, dye cycle violet (DCV) and CDy1 can also become substrates of the transporters, they are used as probes for isolation of CSCs/TICs by flow cytometry (side population assay, ) [Citation9,Citation15,Citation20–22]. The ABCC-type subfamily and ABCA-type subfamily are also overexpressed in CSCs/TICs of certain tumour cells [Citation23]. Overexpression of these ABC transporters is the major cause of the chemotherapeutic resistance of CSCs/TICs.
Detoxification enzyme genes
This group includes the aldehyde dehydrogenases (ALDHs). Class 1 ALDH (ALDH1) is a cytosolic enzyme oxidising harmful aldehydes, converting them into less toxic carboxylic acids. It is also expressed in normal stem/progenitor cells and functions in retinoic acid synthesis as retinal dehydrogenase, which is important in embryonic development and stem cell differentiation [Citation24–26]. Therefore, ALDH1 activity has been extensively studied in normal stem/progenitor cells. There are 19 members of the ALDH-family expressed in cytosol or mitochondria of non-cancerous cells, and some of them are overexpressed in CSCs/TICs [Citation1,Citation27]. ALDH activity can be assessed by flow cytometry using the ALDEFLUOR fluorescent reagent [Citation13,Citation28]. Ginestier et al. first demonstrated that breast cancer cells with increased ALDH activity had stem-like properties and high tumour-initiating capacity, providing a new assay to isolate CSCs/TICs [Citation29]. Using this assay, CSCs/TICs have been isolated successfully from a number of solid tumour cells including those of lung, colon, liver, prostate, uterine and ovarian cancers [Citation11,Citation13,Citation30–32], indicating that high ALDH activity might be a general property of CSCs/TICs of diverse origins. It has been reported that high ALDH1/3 expression is associated with resistance to cyclophosphamide [Citation4,Citation33]. In addition, it was reported recently that ALDH1A1 expression conferred resistance to gemcitabine in human pancreatic cancer cells. Therefore, it is likely that high ALDH activity causes resistance to non-aldehyde toxic stress as well.
Anti-oxidative stress genes
The aldo-keto reductase (AKR) family is a family of enzymes that includes heterogeneous NADPH-dependent oxidoreductases, which are expressed in the normal liver and placenta [Citation34]. These enzymes function to catalyse reactive oxygen species (ROS), thus protecting cells from oxidative stress. There are 12 AKR family proteins, and some of them are preferentially expressed in CSCs/TICs of various solid tumour cells. We and others identified their overexpression (AKR1C1 and AKR1C2) through transcriptome analysis of CSCs/TICs of ovarian and lung cancer cells [Citation35], and Nomura et al. showed overexpression of the proteins (AKR1C1 and AKR1C3) through proteome analysis of CSCs of large cell neuroendocrine carcinoma of the lung [Citation36]. The AKR family is involved in retinoic acid biosynthesis, suppression of cell differentiation and resistance to oxidative stress [Citation37–39]. Expression of the AKR family is regulated by the transcriptional factor NF-E2-related factor 2 (NRF2), a master regulator of antioxidant genes [Citation40,Citation41]. In this context, it is noteworthy that NRF2 is also constitutively activated in CSCs/TICs as well as normal haematopoietic stem cells [Citation42]. Activated NRF2 drives a transcriptional programme to increase oxidative stress resistance and reduce ROS in CSCs/TICs of diverse origins [Citation8].
In addition to the AKR family, certain members of the selenoprotein family are overexpressed in CSCs/TICs as well (). Selenoproteins are proteins that contain the selenium-binding amino acids selenocysteine and selenomethionine, and are known to have antioxidant/redox properties (glutathione peroxidases and thioredoxin reductases) [Citation43,Citation44]. Furthermore, increasing evidence suggests that they have a protective role against genotoxic stress [Citation45].
Figure 3. Semi-quantitative RT-PCR analysis of genes expressed in HEC-1 endometrial cancer cells. Total RNAs of side population (SP) and main population (MP) of HEC-1 endometrial cancer cells were reverse transcribed and amplified by using Taq enzyme (QIAGEN). The thermal cycling conditions were 94 °C for 2 min, followed by 35 cycles of 15 s at 94 °C, 30 s at 60 °C, and 30 s at 72 °C.
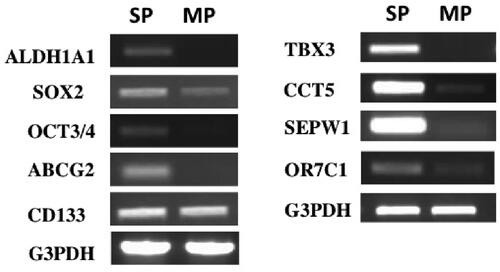
Anti-hypoxic stress genes
Forkhead box O (FOXO) transcription factors are involved in the regulation of the cell cycle, apoptosis and metabolism, and their activity affects the maintenance, pluripotency and longevity of normal stem/progenitor cells [Citation46,Citation47]. FOXO proteins are critical regulators of oxidative stress by modulating the expression of several antioxidant enzyme genes, thus playing a pivotal role in the regulation of the stem/progenitor cell pool in the haematopoietic system [Citation48]. We found that FOXO3A was overexpressed and highly phosphorylated in CSCs/TICs. FOXO3A is known to be activated by hypoxic stress and required for suppression of oxygen consumption and ROS production, thus promoting cell survival in hypoxia [Citation49]. The constitutive expression and activation of FOXO3A in CSCs/TICs might be mediated by the activation of the PI3K/AKT signaling pathway and is likely involved in their high resistance to hypoxic stress and oxidative stress.
Anti-proteotoxic stress genes
Heat shock proteins (HSPs) and heat shock factor 1 (HSF1), key factors in the heat shock response have been implicated in the aetiology of cancer [Citation50]. We and others have found that certain HSPs are preferentially expressed in CSCs/TICs [Citation32]. These include HSPA1B, Hsp70B′, Hsp27, CCT5 and DNAJB8 [Citation3,Citation12]. Expression of HSPs is induced by thermal stress or proteotoxic stressors that change the conformation of the ternary structure of the protein (folding state) or lead to its degradation [Citation13]. Hsp70B′ is an Hsp70 family chaperone that is strictly inducible with little or no basal expression in non-cancerous cells [Citation51]. HSPA1B is also an Hsp70 family chaperone that is expressed during spermatogenesis and early embryogenesis in the absence of stress [Citation52]. DNAJB8 is an Hsp40 family chaperone that is exclusively expressed during spermatogenesis. It remains unknown why these special HSPs are preferentially expressed in CSCs/TICs; however, aberrant expression and activation of heat shock factors (HSFs), master regulators of the heat shock response, might be involved in the constitutive expression of the special HSPs in CSCs/TICs [Citation53]. Notably, we found that HSF1 was constitutively expressed and phosphorylated in the absence of stress in CSC/TIC-derived xenograft tumour tissues of human endometrial cancer cells (). Mendillo et al. reported that HSF1, which is constitutively activated in cancer cells, drives distinct gene expression from heat shock to support a highly malignant cancer phenotype [Citation54]. Although this group did not mention HSF1 activation in CSCs, it is possible that the constitutive activation of the HSF1-driven transcriptional programme in CSCs might cause the aberrant expression of special HSPs and other malignant phenotype-associated genes, thus playing an important role in the stress resistance and tumour-initiation capacity of CSCs/TICs.
Figure 4. Immunostaining of tumour tissues showing highly phosphorylated HSF-1 in ALDH1-high tumour cells. Xenograft tumours were obtained by transplantation of ALDH1-high population (A) and ALDH1-low population (B) of HEC-1 cells into NOD/SCID mice. The formalin-fixed tumour tissue sections were stained with anti-phospho-HSF1 (S326) antibody (abcam, Cambridge, UK). Higher nuclear staining in the ALDH-high tumour tissue (A) indicates that HSF1 could be activated in the cells [Citation60].
![Figure 4. Immunostaining of tumour tissues showing highly phosphorylated HSF-1 in ALDH1-high tumour cells. Xenograft tumours were obtained by transplantation of ALDH1-high population (A) and ALDH1-low population (B) of HEC-1 cells into NOD/SCID mice. The formalin-fixed tumour tissue sections were stained with anti-phospho-HSF1 (S326) antibody (abcam, Cambridge, UK). Higher nuclear staining in the ALDH-high tumour tissue (A) indicates that HSF1 could be activated in the cells [Citation60].](/cms/asset/a828e17c-067b-4753-a3c7-2090357426bb/ihyt_a_814809_f0004_b.jpg)
Stress-resistance proteins as potent therapeutic targets for cancer stem cell therapy
DNAJB8 is an Hsp40-family heat shock protein that is overexpressed in CSCs/TICs of renal cell cancer [Citation12]. Interestingly, siRNA-mediated knockdown of DNAJB8 suppresses the tumour initiation capacity of CSCs/TICs. Wei et al. showed that knockdown of Hsp27 in breast cancer cells decreased some characteristics of CSCs/TICs such as sphere formation and ALDH1 activity [Citation32]. Furthermore, we and others found that siRNA-mediated attenuation of HSF1, a master regulator of heat shock proteins, could lead to decreased sphere-forming activity of ovarian CSCs/TICs and haematopoietic CSCs/TICs [Citation55]. These data strongly suggest that CSCs/TICs of diverse origins might show much greater dependence on the function of HSF1 or specific HSPs to maintain their cancer-initiating capacity and anti-stress properties than their non-CSC counterparts. Therefore, HSF1 or certain HSPs might serve as potential therapeutic targets for CSC-targeted therapy [Citation32,Citation50,Citation56]. For example, Newman et al. reported that low concentrations of 17-AAG, an inhibitor of Hsp90, successfully eliminated lymphoma CSCs whereas it failed to eliminate non-CSCs [Citation55]. Screening for inhibitors of HSF1 is being conducted by many researchers and future studies may reveal the potency of HSF1 as a target in CSC-targeted therapy [Citation50,Citation56]. However, it has to be taken into consideration that HSF1 and most HSPs also have crucial roles for the maintenance of stemness and survival in normal stem/progenitor cells. To develop an effective CSC-targeted therapy with minimal toxicity against normal tissues, CSC/TIC-specific stress response genes should be targeted [Citation57]. In this context, cancer/testis gene products such as DNAJB8 and HSPA1B might serve as ideal targets for cancer therapy. They can serve as ideal target antigens for cancer immunotherapy as well, since they have less tolerance against immunisation [Citation58]. Actually, we reported that immunisation with a DNAJB8-expressing DNA vaccine could induce a specific CD8+ T-cell response and tumour suppression [Citation12]. Therefore, DNAJB8 offers a potent therapeutic target for both molecular-targeted therapy using siRNA or a specific inhibitor and for antigen-specific immunotherapy such as a cancer vaccine.
Conclusion and future perspectives
We showed that a number of stress resistance genes were constitutively expressed and activated in CSCs/TICs even in the absence of cellular stress, which could cause therapeutic resistance and tumour recurrence. Most importantly, some of them are testis-specific genes and play crucial roles in the maintenance of the stemness and tumour-initiation capacity of CSCs/TICs as well as spermatogenesis [Citation59]. Therefore, regulation of their expression or inhibition of their activity could serve as a potent therapeutic strategy targeting to CSCs/TICs. Conversely, it is a matter of concern that, under certain stress conditions in which stress resistance genes are up-regulated, stemness characteristics and tumour-initiating capacity might be augmented, thus aggravating the malignant phenotype of the tumour. Actually, recent studies indicate that CSCs and non-CSCs can engage in a bidirectional shift in response to their microenvironment and stressors [Citation5,Citation8]. If this is true, it is likely that incomplete hyperthermic therapy or chemotherapy might induce the dedifferentiation of non-CSCs to CSCs/TICs and augment tumour aggressiveness. Regulation of the expression and function of stress resistance molecules might suppress such a reverse transformation of tumour cells. Further understanding of aberrantly expressed stress resistance molecules in CSCs/TICs and their molecular mechanisms should lead to novel therapeutic strategies for cancer.
Declaration of interest
The authors report no conflicts of interest. The authors alone are responsible for the content and data in the paper.
References
- Zhang F, Song C, Ma Y, Tang L, Xu Y, Wang H. Effect of fibroblasts on breast cancer cell mammosphere formation and regulation of stem cell-related gene expression. Int J Mol Med 2011;28:365–71
- Shahi P, Seethammagari MR, Valdez JM, Xin L, Spencer DM. Wnt and Notch pathways have interrelated opposing roles on prostate progenitor cell proliferation and differentiation. Stem Cells 2011;29:678–88
- Li G, Zhao F, Cui Y. Proteomics using mammospheres as a model system to identify proteins deregulated in breast cancer stem cells. Curr Mol Med 2013;13:459–63
- Dylla SJ, Beviglia L, Park IK, Chartier C, Raval J, Ngan L, et al. Colorectal cancer stem cells are enriched in xenogeneic tumors following chemotherapy. PloS One 2008;3:e2428
- Hsu CS, Tung CY, Yang CY, Lin CH. Response to stress in early tumor colonization modulates switching of CD133-positive and CD133-negative subpopulations in a human metastatic colon cancer cell line, SW620. PloS One 2013;8:e61133
- Abdullah LN, Chow EK. Mechanisms of chemoresistance in cancer stem cells. Clin Transl Med 2013;2:3
- Calcagno AM, Salcido CD, Gillet JP, Wu CP, Fostel JM, Mumau MD, et al. Prolonged drug selection of breast cancer cells and enrichment of cancer stem cell characteristics. J Natl Cancer Inst 2010;102:1637–52
- Achuthan S, Santhoshkumar TR, Prabhakar J, Nair SA, Pillai MR. Drug-induced senescence generates chemoresistant stemlike cells with low reactive oxygen species. J Biol Chem 2011;286:37813–29
- Foster BA, Gangavarapu KJ, Mathew G, Azabdaftari G, Morrison CD, Miller A, et al. Human prostate side population cells demonstrate stem cell properties in recombination with urogenital sinus mesenchyme. PloS One 2013;8:e55062
- Nakatsugawa M, Takahashi A, Hirohashi Y, Torigoe T, Inoda S, Murase M, et al. SOX2 is overexpressed in stem-like cells of human lung adenocarcinoma and augments the tumorigenicity. Lab Invest 2011;91:1796–804
- Nishida S, Hirohashi Y, Torigoe T, Kitamura H, Takahashi A, Masumori N, et al. Gene expression profiles of prostate cancer stem cells isolated by aldehyde dehydrogenase activity assay. J Urol 2012;188:294–9
- Nishizawa S, Hirohashi Y, Torigoe T, Takahashi A, Tamura Y, Mori T, et al. HSP DNAJB8 controls tumor-initiating ability in renal cancer stem-like cells. Cancer Res 2012;72:2844–54
- Nishida S, Hirohashi Y, Torigoe T, Inoue R, Kitamura H, Tanaka T, et al. Prostate cancer stem-like cells/cancer-initiating cells have an autocrine system of hepatocyte growth factor. Cancer Sci 2013;104:431–6
- Morita R, Hirohashi Y, Suzuki H, Takahashi A, Tamura Y, Kanaseki T, et al. DNA methyltransferase 1 is essential for initiation of the colon cancers. Exp Mol Pathol 2013;94:322–9
- Scharenberg CW, Harkey MA, Torok-Storb B. The ABCG2 transporter is an efficient Hoechst 33342 efflux pump and is preferentially expressed by immature human hematopoietic progenitors. Blood 2002;99:507–12
- Yamada R, Takahashi A, Torigoe T, Morita R, Tamura Y, Tsukahara T, et al. Preferential expression of cancer/testis genes in cancer stem-like cells: Proposal of a novel sub-category, cancer/testis/stem gene. Tissue Antigens 2013;81:428–34
- Caballero OL, Chen YT. Cancer/testis (CT) antigens: Potential targets for immunotherapy. Cancer Sci 2009;100:2014–21
- Lage H, Jordan A, Scholz R, Dietel M. Thermosensitivity of multidrug-resistant human gastric and pancreatic carcinoma cells. Int J Hyperthermia 2000;16:291–303
- Caldera V, Mellai M, Annovazzi L, Monzeglio O, Piazzi A, Schiffer D. MGMT hypermethylation and MDR system in glioblastoma cancer stem cells. Cancer Genomics Proteomics 2012;9:171–8
- Vukovic J, Bedin AS, Bartlett PF, Osborne GW. A novel fluorescent reporter CDy1 enriches for neural stem cells derived from the murine brain. Stem Cells Dev 2013; epub ahead of print. DOI: 10.1089/scd.2012.0660
- Hawley TS, Riz I, Yang W, Wakabayashi Y, Depalma L, Chang YT, et al. Identification of an ABCB1 (P-glycoprotein)-positive carfilzomib-resistant myeloma subpopulation by the pluripotent stem cell fluorescent dye CDy1. Am J Hematol 2013;88:265–72
- Gangavarpu KJ, Huss WJ. Isolation and applications of prostate side population cells based on dye cycle violet efflux. Curr Protoc Toxicol 2011;22:22
- Saini V, Hose CD, Monks A, Nagashima K, Han B, Newton DL, et al. Identification of CBX3 and ABCA5 as putative biomarkers for tumor stem cells in osteosarcoma. PloS One 2012;7:e41401
- Hsu LC, Chang WC, Hoffmann I, Duester G. Molecular analysis of two closely related mouse aldehyde dehydrogenase genes: Identification of a role for Aldh1, but not Aldh-pb, in the biosynthesis of retinoic acid. Biochem J 1999;339:387–95
- Rhinn M, Dolle P. Retinoic acid signalling during development. Development (Cambridge) 2012;139:843–58
- Corti S, Locatelli F, Papadimitriou D, Donadoni C, Salani S, Del Bo R, et al. Identification of a primitive brain-derived neural stem cell population based on aldehyde dehydrogenase activity. Stem Cells 2006;24:975–85
- Ikawa M, Impraim CC, Wang G, Yoshida A. Isolation and characterization of aldehyde dehydrogenase isozymes from usual and atypical human livers. J Biol Chem 1983;258:6282–7
- Hellsten R, Johansson M, Dahlman A, Sterner O, Bjartell A. Galiellalactone inhibits stem cell-like ALDH-positive prostate cancer cells. PloS One 2011;6:e22118
- Ginestier C, Hur MH, Charafe-Jauffret E, Monville F, Dutcher J, Brown M, et al. ALDH1 is a marker of normal and malignant human mammary stem cells and a predictor of poor clinical outcome. Cell Stem Cell 2007;1:555–67
- Lin L, Liu A, Peng Z, Lin HJ, Li PK, Li C, et al. STAT3 is necessary for proliferation and survival in colon cancer-initiating cells. Cancer Res 2011;71:7226–37
- Ucar D, Cogle CR, Zucali JR, Ostmark B, Scott EW, Zori R, et al. Aldehyde dehydrogenase activity as a functional marker for lung cancer. Chem Biol Interact 2009;178:48–55
- Wei L, Liu TT, Wang HH, Hong HM, Yu AL, Feng HP, et al. Hsp27 participates in the maintenance of breast cancer stem cells through regulation of epithelial-mesenchymal transition and nuclear factor-kappaB. Breast Cancer Res 2011;13:R101
- Sreerama L, Sladek NE. Cellular levels of class 1 and class 3 aldehyde dehydrogenases and certain other drug-metabolizing enzymes in human breast malignancies. Clin Cancer Res 1997;3:1901–14
- Bohren KM, Bullock B, Wermuth B, Gabbay KH. The aldo-keto reductase superfamily. cDNAs and deduced amino acid sequences of human aldehyde and aldose reductases. J Biol Chem 1989;264:9547–51
- Seo DC, Sung JM, Cho HJ, Yi H, Seo KH, Choi IS, et al. Gene expression profiling of cancer stem cell in human lung adenocarcinoma A549 cells. Mol Cancer 2007;6:75
- Nomura M, Fukuda T, Fujii K, Kawamura T, Tojo H, Kihara M, et al. Preferential expression of potential markers for cancer stem cells in large cell neuroendocrine carcinoma of the lung. An FFPE proteomic study. J Clin Bioinforma 2011;1:23
- Ahmed MM, Wang T, Luo Y, Ye S, Wu Q, Guo Z, et al. Aldo-keto reductase-7A protects liver cells and tissues from acetaminophen-induced oxidative stress and hepatotoxicity. Hepatology 2011;54:1322–32
- Desmond JC, Mountford JC, Drayson MT, Walker EA, Hewison M, Ride JP, et al. The aldo-keto reductase AKR1C3 is a novel suppressor of cell differentiation that provides a plausible target for the non-cyclooxygenase-dependent antineoplastic actions of nonsteroidal anti-inflammatory drugs. Cancer Res 2003;63:505–12
- Ruiz FX, Porte S, Pares X, Farres J. Biological role of aldo-keto reductases in retinoic acid biosynthesis and signaling. Frontiers Pharmacol 2012;3:58
- Jung KA, Choi BH, Nam CW, Song M, Kim ST, Lee JY, et al. Identification of aldo-keto reductases as NRF2-target marker genes in human cells. Toxicol Lett 2013;218:39–49
- Nguyen T, Nioi P, Pickett CB. The Nrf2-antioxidant response element signaling pathway and its activation by oxidative stress. J Biol Chem 2009;284:13291–5
- Tsai JJ, Dudakov JA, Takahashi K, Shieh JH, Velardi E, Holland AM, et al. Nrf2 regulates haematopoietic stem cell function. Nat Cell Biol 2013;15:309–16
- Kim HY, Gladyshev VN. Methionine sulfoxide reductases: Selenoprotein forms and roles in antioxidant protein repair in mammals. Biochem J 2007;407:321–9
- Lee BC, Dikiy A, Kim HY, Gladyshev VN. Functions and evolution of selenoprotein methionine sulfoxide reductases. Biochim Biophys Acta 2009;1790:1471–7
- Ferguson LR, Karunasinghe N, Zhu S, Wang AH. Selenium and its role in the maintenance of genomic stability. Mutat Res 2012;733:100–10
- Sedding DG. FoxO transcription factors in oxidative stress response and ageing – A new fork on the way to longevity? Biol Chem 2008;389:279–83
- Zhang X, Rielland M, Yalcin S, Ghaffari S. Regulation and function of FoxO transcription factors in normal and cancer stem cells: What have we learned? Curr Drug Targets 2011;12:1267–83
- Eijkelenboom A, Burgering BM. FOXOs: Signalling integrators for homeostasis maintenance. Nat Rev Mol Cell Biol 2013;14:83–97
- Sunayama J, Sato A, Matsuda K, Tachibana K, Watanabe E, Seino S, et al. FoxO3a functions as a key integrator of cellular signals that control glioblastoma stem-like cell differentiation and tumorigenicity. Stem Cells 2011;29:1327–37
- Calderwood SK. Heat shock proteins in breast cancer progression – A suitable case for treatment? Int J Hyperthermia 2010;26:681–5
- Noonan EJ, Place RF, Giardina C, Hightower LE. Hsp70B′ regulation and function. Cell Stress Chaperones 2007;12:219–29
- Le Masson F, Christians E. HSFs and regulation of Hsp70.1 (HSPA1B) in oocytes and preimplantation embryos: New insights brought by transgenic and knockout mouse models. Cell Stress Chaperones 2011;16:275–85
- Wilkerson DC, Murphy LA, Sarge KD. Interaction of HSF1 and HSF2 with the HSPA1B promoter in mouse epididymal spermatozoa. Biol Reprod 2008;79:283–8
- Mendillo ML, Santagata S, Koeva M, Bell GW, Hu R, Tamimi RM, et al. HSF1 drives a transcriptional program distinct from heat shock to support highly malignant human cancers. Cell 2012;150:549–62
- Newman B, Liu Y, Lee HF, Sun D, Wang Y. HSP90 inhibitor 17-AAG selectively eradicates lymphoma stem cells. Cancer Res 2012;72:4551–61
- Whitesell L, Lindquist S. Inhibiting the transcription factor HSF1 as an anticancer strategy. Expert Opin Therapeut Targets 2009;13:469–78
- Wierenga PK, Konings AW. Effect of a hyperthermic treatment on the pluripotent haemopoietic stem cell in normal and anaemic mice. Int J Hyperthermia 1990;6:793–800
- Hirohashi Y, Torigoe T, Inoda S, Takahashi A, Morita R, Nishizawa S, et al. Immune response against tumor antigens expressed on human cancer stem-like cells/tumor-initiating cells. Immunotherapy 2010;2:201–11
- Cappell KM, Sinnott R, Taus P, Maxfield K, Scarbrough M, Whitehurst AW. Multiple cancer testis antigens function to support tumor cell mitotic fidelity. Mol Cell Biol 2012;32:4131–40
- Chou SD, Prince T, Gong J, Calderwood SK. mTOR is essential for the proteotoxic stress response, HSF1 activation and heat shock protein synthesis. PloS One 2012;7:e39679