Abstract
Purpose: Intravenous administration of indocyanine green (ICG) dye can effectively convert near-infrared (NIR) laser light into heat and enhance thermal injury of blood vessels; however, there is no selective uptake of ICG by the tumour compared to the other tissues, which impacts the therapeutic ratio of this strategy unless uptake can be selectively increased in tumour tissue. Here we investigated the use of local hyperthermia prior to intravenous ICG administration to enhance ICG uptake in tumour tissue, thereby enhancing laser thermal ablation of solid tumours. Methods: Murine SCK breast or SCCVII head and neck tumours were treated with a 755-nm laser light either alone or with prior intravenous administration of 4 mg/kg ICG and/or local tumour hyperthermia at 42.5 °C for 60 min. Retention of ICG was quantified using a NIR animal imaging system. Treatment effects were assessed by growth delay and histology. Results: ICG accumulation in the heated tumours was 1.23-fold greater on average compared to non-heated tumours, in both models. In SCK tumours, animals receiving either laser irradiation alone or in conjunction with ICG had a 1.86- or 3.91-fold increase in tumour growth delay, respectively. The addition of local hyperthermia before ICG injection resulted in complete regression of SCK tumours. Uptake of ICG increased in SCCVII tumours; however, little change in tumour growth delay was observed. Conclusion: Using local hyperthermia may improve the delivery of ICG to the tumour and thereby increase the extent of laser thermal ablation of smaller superficial malignancies that can be effectively exposed to laser therapy.
Introduction
Minimally invasive laser thermal therapy, in combination with nanoparticles or drugs, is among the most promising of emerging technologies to arrest expansion of cancerous growths with minimal morbidity and reduced toxicity [Citation1,Citation2]. One approach is to use systemic injection of laser absorbing agents or dyes to increase laser-induced thermal damage in the tumour. Indocyanine green (ICG) is a water-soluble tricarbocyanine dye (775 g/mol) that was first approved for clinical use by the US Food and Drug Administration in 1956 [Citation3]. After intravenous injection, ICG binds tightly to plasma proteins and is rapidly cleared by the liver. The absorption spectrum of ICG dissolved in plasma exhibits a strong peak from 750–830 nm with a maximum at 800–810 nm [Citation4,Citation5]. The absorption maximum of ICG at about 800 nm promotes absorption of NIR laser light delivered by a diode laser [Citation6]. In addition, preliminary studies by our group have demonstrated that ICG can induce marked thermal damage to solid tumours after laser irradiation compared to laser alone [Citation7]. The current studies were conducted in one of the same tumour cell lines used in the previous paper, A/J mice with SCK tumours.
High magnification of SCK tumours demonstrates cellular features of necrosis, including loss of cytoplasmic and nuclear detail. Significant damage to the vascular wall in peri-tumoural vessels, including endothelial cell necrosis and loss of endothelial cells and fibrin deposition has also been observed [Citation7].
ICG-enhanced diode laser photocoagulation appears to be superior to diode laser treatment alone in achieving vessel closure, but is limited by clot resolution due to both excessive vascular damage and an accompanying inflammatory response [Citation8]. These results suggest that more durable feeder vessel closure may be achievable by the use of hyperthermia prior to ICG administration, allowing for more ICG to enter the tumour vasculature and interstitium and thereby increasing interstitial cell death upon laser exposure.
However, the damage/thermal ablation was not complete, as evidenced by re-growth of the tumours. In general, there has been no study showing evidence of preferential accumulation of ICG in tumour tissue compared to other tissues which calls into question whether or not the appropriate amount and distribution of ICG is obtained before laser therapy for small tumours. In the current investigation we attempted to increase ICG accumulation at the tumour site by exposing tumours to local hyperthermia. Accumulating experimental data adds support to the idea of using local moderate temperature hyperthermia to improve drug delivery via an increase in tumour blood flow and tumour vessel permeability [Citation9–12]. Our previous studies have suggested that for a short-term perfusion/permeability increase, 42.5 °C may be an optimal temperature. Above this temperature there is vascular damage, while below this temperature, while there may be beneficial effects, they might take hours or even days to be expressed. Therefore, for the current study we selected 42.5 °C [Citation13,Citation14]. We hypothesised that local hyperthermia would enhance delivery of ICG to the tumour site and interstitium, thereby allowing a greater thermal ablation effect of laser therapy on the tumour vasculature and surrounding tumour cells to induce tumour regression.
Methods
Animal preparation and treatment
SCK mammary or SCCVII squamous cell carcinoma tumour cells (2 x 10e5) were subcutaneously inoculated in the rear limb of A/J or C3H mice, respectively. A/J mice are an inbred albino strain produced by Jackson Laboratories used to grow SCK mammary carcinomas. C3H mice are an inbred agouti strain produced by Jackson Laboratories used for SCCVII squamous cell carcinoma growth. The SCK cell line is a mouse mammary carcinoma which grows rapidly and has significant microvascular dysfunction and the SCCVII is a squamous cell carcinoma that grows slightly slower and more uniformly. Cells were cultured and harvested by an addition of 0.25% trypsin for 5 min, washed in 10% serum containing medium and then suspended in serum free medium. These cells were then injected at 2 × 105 cells per 0.05 mL of serum-free medium into the right rear limb. Tumours were allowed to grow until sizes reached 4–8 mm in diameter, at which time the mice were randomised and divided into a control group (n = 5 for SCK and n = 5 for SCC-VII) and two study groups (n = 5 for ICG/laser and n = 5 for the hyperthermia/ICG/laser for both murine strains). In the two study groups, the mice were treated with NIR laser preceded by an intravenous administration of sterile ICG solution and laser (group 1) or hyperthermia, ICG, and laser (group 2), as described below. No treatment was applied to the mice in the control group.
A single dose of 4 mg/kg of ICG solution was administered 2 min prior to laser irradiation, into the lateral tail vein of each mouse in the first study group and all groups receiving ICG hereafter. In mice we observed a maximum concentration of ICG in the blood and at the tumour site as soon as 2 min and lasting until about 6 min post-injection. However, this window is very dynamic as the ICG is being cleared quickly by the liver. If we were to wait until 6 min, we might have missed this window. We thus chose to start treating at 2 min post-injection. In our earlier studies we had to use multiple laser pulses to cover the tumour area and therefore began at 2 min after inravenous injection as well. For these reasons we chose to keep our studies constant and treat with laser at 2 min after ICG administration. A single pulse from a near-infrared (NIR) laser was applied with a circular beam width of 8 mm diameter. In the second study group, hyperthermia was administered at 42.5 °C for 1 h. A single dose of 4 mg/kg of ICG was injected into the tail vein of each mouse followed by one pulse from a NIR laser.
A NIR laser system (GentleLASE, Candela, Wayland, MA, USA) emitting 755 nm light was used in this study. The laser beam diameter was 8 mm, the pulse time was 3 ms and the laser radiant exposure was 70 J/cm2. Epidermal cooling was accomplished by using cryogen spray cooling with two 30-ms spurts before and 10 ms after the laser pulse. The laser hand-piece was positioned directly above and perpendicular to the heated platform on which the mouse was placed. The platform was attached to a micrometer-driven translation stage to ensure the same orientation was used for each laser exposure. The entire tumour and margins were irradiated using a single pulse while the laser beam was stationary. This pulse resulted in complete coverage of the exposed surface of the tumour.
During laser treatments, the mice were anaesthetised with 1–2% isofluorane by inhalation. The body temperatures of the mice were maintained by individually placing them on a 37 °C heated platform during treatment. Following laser treatment the mice were allowed to recover and returned to the colony. The mice were monitored for behaviour and tumour volumes for up to 10 days post-treatment. Tumour sizes were measured every 1–2 days with a Vernier caliper. Tumour volumes were calculated using the formula a2b/2, where a and b are the shorter and longer diameters of the tumour, respectively.
One mouse from each study group was sacrificed at day 3 after laser treatment for histological analysis. Immediately following euthanasia, the tumours were excised and were processed and embedded in paraffin blocks for routine histological analysis. Tissue sections (5 μm thick) were stained with haematoxylin and eosin (H&E) and examined under a light microscope. Quantitative pathological analysis hardware and software, Aperio Scanscope T2 and ImageScope software (Aperio, Vista, CA, USA), was used to determine the relative necrotic area in each tissue section.
ICG uptake
Indocyanine green was obtained from Pulsion (Pulsion Medical Systems, Munich, Germany) in vials of 25 mg. The ICG powder was dissolved in 5 mL sterile water and further diluted in sterile saline to a concentration of 1.25 mg/mL. A single dose of ICG solution (4 mg/kg body weight) was administered to each mouse, for imaging only. Kodak in vivo imaging system (Carestream Health Molecular Imaging, New Haven, CT, US) was used to excite and monitor the ICG fluorescence intensity within the anaesthetised mouse. The fluorescence intensity was recorded by taking consecutive images of the mice immediately before and up to 15 min post-ICG administration. The change of the fluorescence intensity (FI) was determined by calculating the ratio between the FI at each time point and the FI prior to the ICG administration, as previously described [Citation7].
Results
ICG accumulation
In the ICG uptake assay, hyperthermia was administered at 42.5 °C for 60 min, ICG at 4 mg/kg bodyweight and a single pulse from a laser emitting at a wavelength of 755 nm. Examples of fluorescence intensity measured across the tumour region in a control and heated tumour are shown in .
Figure 1. SCK tumours were treated in vivo with (A) ICG at 4 mg/kg followed by laser thermal therapy or (B) local hyperthermia at 42.5 °C before ICG administration at 4 mg/kg followed by laser thermal therapy. Near-infrared imaging was performed for 30 min following therapy with snapshots taken every 60 s to assess fluorescent intensity of ICG in the tumour.
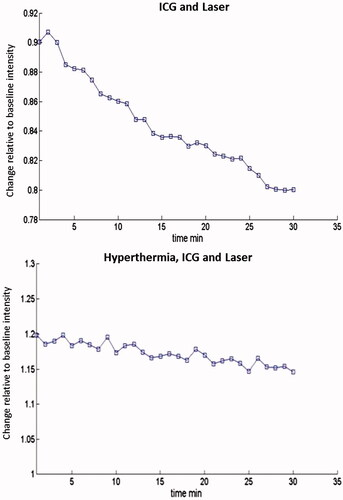
Our results demonstrated an increase in maintained ICG retention over 30 min after hyperthermia as compared to tumours receiving no hyperthermia prior to ICG administration. The area under the curve was determined for each animal in each treatment group. The average of each treatment group is seen in and .
Figure 2. Hyperthermia increases fluorescent intensity of ICG in SCK tumours. Hyperthermia was administered at 42.5 °C for 60 min prior to ICG (4 mg/kg bodyweight) administration and whole body near-infrared imaging. Each treatment group consisted of five animals.
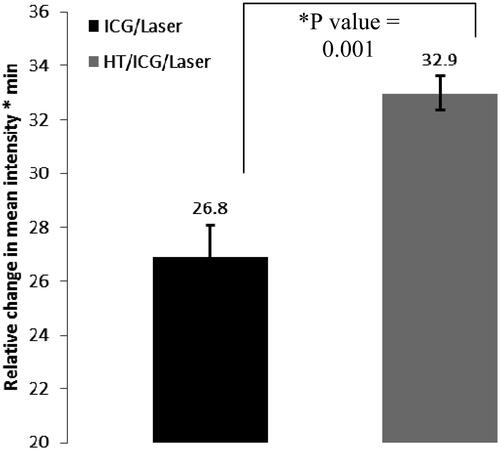
Figure 3. Hyperthermia increases fluorescent intensity of ICG in SCCVII tumours. Hyperthermia was administered at 42.5 °C for 60–90 min prior to ICG (4 mg/kg bodyweight) administration and whole body infrared imaging.
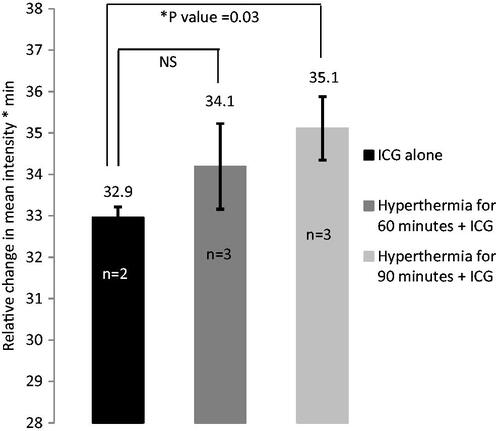
In SCK tumours, ICG retention was assessed when the laser pulse was given 2 min after ICG administration and/or hyperthermia exposure. The retention of ICG was greater over time when hyperthermia was added before ICG and laser (p value = 0.001). In SCCVII tumours, hyperthermia was administered for varying times of 60 or 90 min to determine the optimal duration for this tumour model since our original tumour response was not found to be as substantial as in the SCK model ( and ).
At 90 min, ICG was retained in the tumour at higher concentrations than at 60 min or with no hyperthermia (p value = 0.03). However, when ICG was administered just after 60 min of local hyperthermia, there was an increase in ICG retention but it was not statistically significant (p value = 0.35) compared to animals receiving ICG alone in SCCVII tumours. The statistical significance of the retention assessment was determined by comparing the ratio between the FI at each time point and the FI prior to the ICG administration, followed by an analysis of the area-under-the-curve value that this graph produced, using a two-tailed t-test.
Tumour growth delay
Treatment groups in the SCK tumour model were untreated control, laser alone, ICG and laser, and hyperthermia with ICG and laser. Animals receiving laser irradiation alone had a tumour growth delay 1.86-fold greater than untreated control tumours, and tumours treated with laser irradiation in conjunction with ICG had a 3.91-fold increase in growth delay. When SCK tumours were treated with hyperthermia for 60 min at 42.5 °C followed by ICG administration and laser thermal therapy, a complete regression was seen in four of four tumours monitored for tumour growth (). Healthy tissue was spared and no major skin damage was observed during or post-treatment.
Figure 4. Mean SCK tumour volume as a function of time (days) after treatment. Tumours were treated with hyperthermia for 60 min prior to ICG administration. Thereafter, one laser pulse was delivered to the tumour region. Tumour diameters were measured every day and tumour volume was calculated by the formula a2b/2, where a and b are the shorter and longer diameters of the tumour, respectively. Measurements were taken every 24 h.
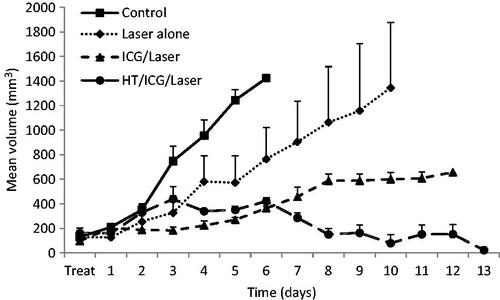
The same treatment groups were used in the SCCVII tumour model () with the same heating and laser parameters. The tumour volume was approximately 1.5-fold greater on average than that in the SCK tumours on the day of treatment. Control animals grew to four-fold the starting volume in approximately 7 days. Tumours exposed to laser alone also grew four-fold in volume in 7 days. When mice were treated with ICG with or without hyperthermia before laser exposure, the mean tumour volume increased 4-fold in approximately 8 days, a tumour growth delay of 1.15 days.
Figure 5. Mean SCCVII tumour volume as a function of time (days) after treatment. Tumours were treated with hyperthermia for 60 min prior to ICG administration. Thereafter, one laser pulse was delivered to the tumour region. Tumour diameters were measured every day and tumour volume was calculated by the formula a2b/2, where a and b are the shorter and longer diameters of the tumour, respectively. Measurements were taken every 48 h.
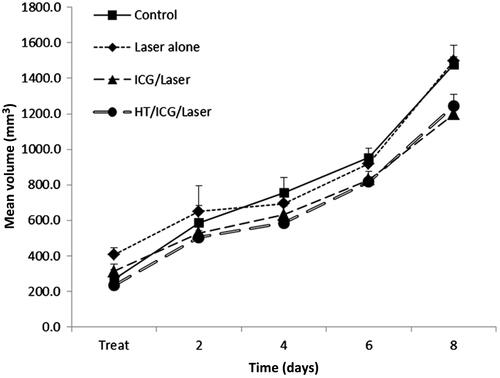
Histological assay
Two SCK tumours were harvested 72 h after treatment, one from the ICG with laser group and one from the hyperthermia, ICG with laser group. Both tumour tissue samples were fixed and stained with H&E (). These sections were converted to greyscale in Image J, and a threshold intensity value was set using the ‘image > adjust > threshold’ menu to exclude background fluorescence of normal epithelial structures in a non-ablated control sample. Using the black and white method of digital mark-up previously developed by our group, normally fluorescent structures (collagen and keratin) remain white, while viable epithelial cells and tumour cells are black [Citation15]. Tumour margins were outlined in red, as illustrated in . When tumours were treated with hyperthermia, ICG and laser, a marked decrease in tumour tissue viability was observed at 72 h post-treatment compared to tumours treated with ICG and laser irradiation.
Figure 6. H&E stain after treatment. Tumours were harvested 72 h post-treatment and subsequently treated with H&E stain, fixed and mounted. (A) SCK tumours were treated with ICG and laser. (B) SCK tumours were treated with hyperthermia at 42.5 °C for 60 min, ICG and laser. (C) SCK tumours were treated with ICG and laser. The portion of this slide that has been outlined in red denotes the tumour tissue. Within this region the white area is dead while the black portion is live tissue. (D) SCK tumours were treated with hyperthermia at 42.5 °C for 60 min, ICG and laser. The portion of this slide that has been outlined in red denotes the tumour tissue. Within this region the white area is dead while the black portion is live tissue.
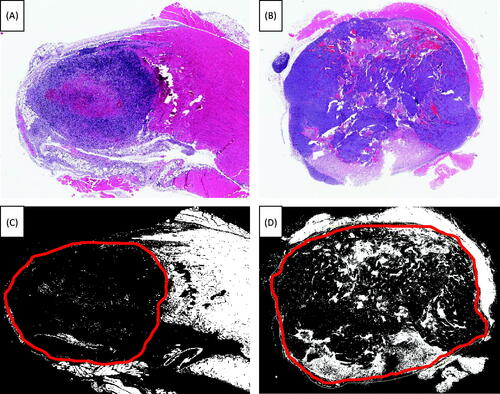
SCCVII tumours were not studied with histology because there was no noticeable difference in tumour response between the treated and control groups.
Discussion
The purpose of this study was to determine the effects of hyperthermia on the anti-tumour effect of ICG in conjunction with laser therapy in two murine tumour types. We hypothesised that the addition of heat via local hyperthermia would create increases in tumour perfusion and vascular permeability which would, in turn, lead to increased ICG concentration within the tumour tissue. We expected that an increase in ICG concentration would result in higher absorption of the laser light and maximise temperature elevation within the target tissue, based on our previous observations with hyperthermia and ICG-induced thermal ablations [Citation7,Citation16].
Tumour vasculature is formed from normal arterioles which become incorporated into the tumour tissue [Citation17]. These vessels dilate upon heating at mild temperatures, probably due to smooth muscle relaxation via stimulation by nitric oxide synthesised by endothelial cells [Citation18]. The increase in tumour blood flow and vascular permeability caused by mild temperature hyperthermia is demonstrated to increase delivery of ICG in our investigation. This result agrees with other studies that have demonstrated that other treatment agents such as drug-containing liposomes, immunotherapeutic agents and genetic constructs can be delivered more effectively when hyperthermia is applied [Citation19].
From our results we can conclude that SCK tumours in A/J mice respond in a therapeutically favourable manner to the addition of local hyperthermia before ICG administration and laser irradiation as evidenced in the full tumour regression observed (). Since this tumour type is a model of a mammary carcinoma, it appears feasible that patients presenting with superficial mammary tumours or chest wall recurrent nodules at a relatively shallow depth or those that could be exposed to laser irradiation interstitially or intra-operatively may have improved tumour control by adding ICG-based laser therapy.
In the other model studied, a head and neck cell line (SCCVII), the tumours did not respond as favourably over time. These tumours were 1.5-fold larger on average than the SCK tumours at the time of treatment and possibly did not receive a homogenous laser dose, which is always a limiting factor for laser-based treatments. The ICG uptake results also indicated a less statistically significant increase in ICG accumulation after heating. Interestingly, we noted an obvious treatment effect in the top layer of the tumours (data not shown), especially after the combined heat, ICG and laser exposure, which suggests that a portion of the tumour responded favourably, but the remainder of the tumour volume continued to grow and therefore overshadowed the damage caused in the top 3 mm of tumour tissue. This result was to be somewhat expected as these tumours generally grew to diameters near the boundary of the treatment area (8 mm) by the day of treatment. This may have accounted for the poor response we saw in this tumour model overall. Regardless, as proof of principle our results suggest that in a clinical application with optimised lasers there may be improved tumour control if superficial head and neck tumours could be locally heated before ICG infusion and laser irradiation.
From a clinical standpoint, in vivo monitoring of ICG accumulation after hyperthermia may be used for determining the optimal time for delivery of laser irradiation and the likelihood of success for that treatment. The fluorescent nature of ICG when excited by laser light offers a means of visualising the peak time for laser exposure as denoted by ICG accumulation and thereby may elicit a more favourable therapeutic response. For example, a photoacoustic flow cytometry method was developed for real-time detection of ICG and other contrast agents to characterise the kinetics of ICG in a mouse ear [Citation12] and there are clinical systems already in use to determine clearance time of ICG in the blood [Citation20]. Therefore, a similar approach to monitor tumour concentration may be useful in the further development of individually specialised laser therapy in conjunction with ICG injection and local hyperthermia to maximise tumour response.
Declaration of interest
This study was supported in part by NIH/NCI 3R01CA044114-21S1and CA44114. R.J.G. and G.S. were co-principal investigators of this study.
Acknowledgements
We thank Pulsion Medical Systems AG for providing the ICG.
References
- El-Sayed IH, Huang X, El-Sayed MA. Selective laser photo-thermal therapy of epithelial carcinoma using anti-EGFR antibody conjugated gold nanoparticles. Cancer Lett 2006;239:129–35
- Kennedy LC, Bickford LR, Lewinski NA, Coughlin AJ, Hu Y, Day ES, et al. A new era for cancer treatment: Gold-nanoparticle-mediated thermal therapies. Small 2011;7:169–83
- Fox I, Brooker L, Heseltine D, Essex H, Wood E. New dyes for continuous recording of dilution curves in whole blood independent of variations in blood oxygen saturation. Am J Physiol 1956;187:599–612
- Landsman ML, Kwant G, Mook GA, Zijlstra WG. Light-absorbing properties, stability, and spectral stabilization of indocyanine green. J Appl Physiol 1976;40:575–83
- Philip R, Penzkofer, A, Bäumler W, Szeimies R, Abels C. Absorption and fluorescence spectroscopic investigation of indocyanine green. J Photochem Photobiol 1996;96:137–48
- Zafereo ME, Hanasono MM, Rosenthal DI, Sturgis EM, Lewin JS, Roberts DB, et al. The role of salvage surgery in patients with recurrent squamous cell carcinoma of the oropharynx. Cancer 2009;115:5723–33
- Shafirstein G, Bäumler W, Hennings LJ, Siegel ER, Friedman R, Moreno MA, et al. Indocyanine green enhanced near-infrared laser treatment of murine mammary carcinoma. Int J Cancer 2012;130:1208–15
- Wolfe JD, Csaky KG. Indocyanine green enhanced retinal vessel laser closure in rats: Histologic and immunohistochemical observations. Exp Eye Res 2004;79:631–8
- Song CW, Park HJ, Lee CK, Griffin R. Implications of increased tumor blood flow and oxygenation caused by mild temperature hyperthermia in tumor treatment. Int J Hyperthermia 2005;21:761–7
- Griffin RJ, Dings RPM, Jamshidi-Parsian A, Song CW. Mild temperature hyperthermia and radiation therapy: Role of tumour vascular thermotolerance and relevant physiological factors. Int J Hyperthermia 2010;26:256–63
- Dreher MR, Liu W, Michelich CR, Dewhirst MW, Yuan F, Chilkoti A. Tumor vascular permeability, accumulation, and penetration of macromolecular drug carriers. J Natl Cancer Inst 2006;98:335–44
- Li L, ten Hagen TLM, Bolkestein M, Gasselhuber A, Yatvin J, van Rhoon GC, et al. Improved intratumoral nanoparticle extravasation and penetration by mild hyperthermia. J Control Release 2013;167:130–7
- Shakil A, Osborn JL, Song CW. Changes in oxygenation status and blood flow in a rat tumor model by mild temperature hyperthermia. Int J Radiat Oncol Biol Phys 1999;43:859–65
- Song CW, Park HJ, Lee CK, Griffin R. Improvement of tumor oxygenation status by mild temperature hyperthermia alone or in combination with carbogen. Semin Oncol 1997;24:626–32
- Hennings L, Kaufmann Y, Griffin R, Siegel E, Novak P, Corry P, et al. Dead or alive? Autofluorescence distinguishes heat-fixed from viable cells. Int J Hyperthermia 2009;25:355–63
- Babilas P, Shafirstein G, Baier J, Schacht V, Szeimies RM, Landthaler M, et al. Photothermolysis of blood vessels using indocyanine green and pulsed diode laser irradiation in the dorsal skinfold chamber model. Lasers Surg Med 2007;39:341–52
- Folkman J. Tumor angiogenesis: Therapeutic implications. N Engl J Med 1971;285:1182–6
- Song CW, Park H, Griffin RJ. Improvement of tumor oxygenation by mild hyperthermia. Radiat Res 2001;155:515–28
- Peller M, Schwerdt A, Hossann M, Reinl HM, Wang T, Sourbron S, et al. MR characterization of mild hyperthermia-induced gadodiamide release from thermosensitive liposomes in solid tumors. Invest Radiol 2008;43:877–92
- Cuccia DJ, Bevilacqua F, Durkin AJ, Merritt S, Tromberg BJ, Gulsen G, et al. In vivo quantification of optical contrast agent dynamics in rat tumors by use of diffuse optical spectroscopy with magnetic resonance imaging coregistration. Appl Opt 2003;42:2940–50