Abstract
In utero hyperthermia can cause a variety of developmental issues, but how it alters mammalian body temperature during adolescence is not well-understood. Study objectives were to determine the extent to which in utero hyperthermia affects future phenotypic responses to a heat load. Pregnant first parity pigs were exposed to thermal neutral (TN) or heat stress (HS) conditions during the entire gestation. Of the resultant offspring, 12 were housed in TN conditions, and 12 were maintained in HS conditions for 15 days. Adolescent pigs in HS conditions had increased rectal temperature and respiration rate (RR) compared to TN pigs, regardless of gestational treatment. Within the HS environment, no gestational difference in RR was detected; however, GHS pigs had increased rectal temperature compared to GTN pigs. As rectal temperature increased, GTN pigs had a more rapid increase in RR compared to the GHS pigs. Adolescent HS decreased nutrient intake, and body weight gain, but neither variable was statistically influenced by gestational treatments. In summary, in utero HS compromises the future thermoregulatory response to a thermal insult.
Introduction
Environmentally induced hyperthermia or heat stress (HS), results from the imbalance between thermal energy flowing into and out of an animal [Citation1], and negatively impacts health and development in all mammalian species studied. Typical HS responses include slower growth rates, altered metabolism, mortality and altered body composition characterised primarily by increased adipose tissue and reduced skeletal muscle mass [Citation2–4]. Moreover, swine genetic selection for lean tissue accretion rates further compromises HS tolerance as synthesising and maintaining enhanced muscle mass generates increased metabolic heat [Citation5,Citation6]. Consequently, HS is likely one of the primary factors limiting animal protein production and if the frequency of severe hot weather increases as predicted [Citation7] food security will continue to be compromised during the warm summer months, especially in developing countries [Citation4].
In utero hyperthermia negatively impacts a variety of fetal development parameters and can be teratogenic [Citation8]. Furthermore, maternal hyperthermia can have a lasting imprint on offspring growth, behaviour [Citation9], and metabolism [Citation10]. Heat stress during embryonic development either increases survivability to a future heat load in Drosophila buzzatii [Citation11] or improves the future ability to remain euthermic during a thermal insult in birds [Citation12,Citation13]. Further, thermal conditioning immediately following birth can imprint long-term thermotolerance in rodents [Citation14]. In the aforementioned reports, thermotolerance is defined as the ability to maintain a lower or safe body temperature in response to a future heat load. However, the extent to which in utero HS affects future body temperature indices in mammals lacks thorough exploration. Study objectives were to characterise HS-induced body temperature indices in adolescent pigs exposed to differing in utero thermal environments. We hypothesised that mammalian gestational HS would imprint future thermotolerance to a heat load.
Materials and methods
Animal use in this study was approved by the University of Missouri, Columbia, Animal Care and Use Committee and the Iowa State University Institutional Animal Care and Use Committee.
Gestational environments
Fifteen first parity cross-bred females (Large White × Landrace) were exposed to thermal neutral (TN) (cyclical 15 °C nighttime and 22 °C daytime, 55% relative humidity (RH), n = 8), or HS (cyclical 27 °C night-time and 37 °C daytime, 67.5% RH, n = 7) conditions in the Brody environmental chambers at the University of Missouri, Columbia as previously described by Lucy and colleagues [Citation15]. The environmental treatments began six days after insemination and continued until birth (114 days gestation) and resulted in a sustained increase (0.30 °C) in body temperature in pregnant first parity pigs [Citation15]. After parturition, all piglets were exposed to the same environmental conditions (26 to 32 °C) as recommended for neonatal pigs [Citation16]. At weaning, offspring from both TN- and HS-exposed pregnant first parity pigs were transported in an environmentally controlled trailer to Iowa State University for postnatal experiments.
Adolescent environments
Between weaning and 15 weeks of age all animals were co-mingled and fed ad libitum in thermal neutral conditions (based upon body weight) [Citation16]. At 15 weeks of age, adolescent pigs from gestational TN (GTN) (n = 12, 67.9 ± 5.3 kg body weight (BW)), and gestational HS (GHS) (n = 12, 64.9 ± 3.7 kg BW) exposed pregnant first parity pigs were housed in individual pens (0.61 × 2.43 m, no wallowing access) in one of two environmentally controlled rooms (TN and HS). Castrated males were selected to reduce the body temperature variability associated with gonadal steroids. In each room, relative humidity (%RH) and ambient temperature (Ta) were recorded every 30 min using two mounted data loggers (El-WIN-USB, Lascar Electronics, Salisbury, UK) for the length of the experiment. Data loggers were positioned in opposite ends of the rooms to confirm that temperature was uniform throughout each room.
Twelve adolescent males (n = 6 GTN, n = 6 GHS) were housed in constant TN conditions, and 12 adolescent males (n = 6 GTN, n = 6 GHS) were maintained in constant HS conditions for 15 days. The TN room was maintained at 22.7 ± 2.5 °C and 71.1 ± 10.0% RH, while the HS room was maintained at a constant 34.7 ± 2.3 °C and 50.4 ± 9.7% RH. All pigs were fed a standard commercial diet ad libitum consisting primarily of corn and soybean meal formulated to meet or exceed nutritional requirements [Citation17]. Nutrient intake was determined on days 7 and 15, while BW was determined on days −1, 7, and 15. Body weights were used to calculated average daily gain (ADG). Unrestrained respiration rate (RR), skin temperature, and rectal temperature (Tre), were measured four times daily (08:00, 12:00, 16:00, 20:00 h) on all animals. Respiration rate in breaths per min (bpm) was determined by counting flank movement over a 1-min interval. Skin temperature (Tskin) was determined by calculating the mean temperature of four sites measured at the ear (Tear), shoulder (Tshoulder), rump (Trump) and tail (Ttail) using a calibrated infrared thermometer (Model 42505, Extech Instruments, Waltham, MA). Rectal temperature was determined with a calibrated and lubricated thermistor thermometer (Welch Allyn SureTemp®Plus, Skaneateles Falls, NY) inserted approximately 10 cm into the rectum of unrestrained pigs. Due to the constant heat load, and lack of statistical hourly differences the hourly body temperature indices (RR, Tskin, Tre) were condensed into daily averages. A thermal circulation index (TCI) was calculated using Tre, temperature at one of the skin sites or Tskin, and Ta, and was used as an indicator of blood and heat transfer to a particular area of the skin under steady state thermal conditions described for agricultural species [Citation18]:
Statistics
All data were analysed using the PROC MIXED procedure in SAS 9.3 (SAS Institute, Cary, NC). Statistical model components included gestational environment (GTN, GHS), adolescent environment (TN, HS), day (1–15), and all possible interactions. All interactions, regardless of significance level, were included in the model and pregnant first parity pig was used as a random effect. Initial body weight was used as a covariate for analysis of final body weight and body weight gain. For repeated analyses, each pig’s respective parameter was analysed using repeated measures with an auto-regressive covariance structure with day as the repeated effect.
Linear (y = mx + b) and quadratic models were created using JMP (SAS Institute) to determine the relationship between RR (y – axis) and Tre (x – axis). Slope and correlation coefficient values from each model were similar, so the linear regression was used for analysis. Slope was obtained for individual animals using all collected data points and analysed using the mixed procedure in SAS 9.3, with slope as the dependent variable, and gestational environment (GTN, GHS), adolescent environment (TN, HS) and the interaction of gestational environment and adolescent environment as fixed effects. Pregnant first parity pig was used as a random effect. All interactions, regardless of significance level were kept in the model. Data are presented as least squares means and statistical significance was defined as p ≤ 0.05, and a tendency was defined as 0.05 < p ≤ 0.10.
Results
Thermal indices
There were no gestational differences detected in overall RR (p > 0.14), but there was a large RR increase (∼2 fold, p < 0.01) for adolescent pigs in HS conditions compared to TN controls (, ). Regardless of gestational treatment, adolescent pigs in HS conditions had increased Tre (p < 0.01, 0.33 °C, ) compared to TN-housed pigs (). An overall gestational by adolescent interaction (p < 0.01) indicated that GHS pigs had a larger increase in Tre during adolescent HS compared to GTN pigs (0.50 versus 0.16 °C, , ). A gestational environment by adolescent environment by day interaction was not observed for Tre ().
Figure 1. Effects of gestational and adolescent thermal environments on the temporal changes in (A) respiration rate (RR), (B) rectal temperature (Tre), and (C) Tre averaged by gestational and adolescent environment in growing pigs. Gestational heat stress (GHS), gestational thermal neutral (GTN), adolescent thermal neutral conditions (TN), adolescent heat stress conditions (HS). Error bars on day 1 and day 15 of the line indicate ±1 SEM. Different letters (a,b,c) above vertical bars in 1C indicate significant differences (p < 0.05).
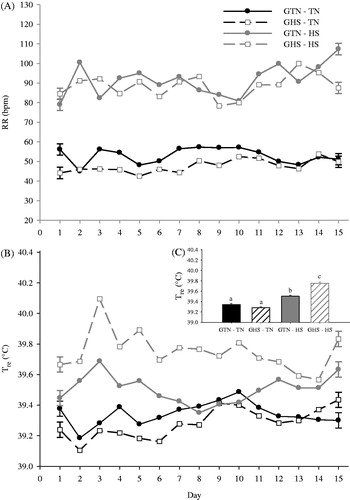
Table I. Effects of gestational and adolescent thermal environments on body temperature indices in growing pigs.
No overall differences were detected at any specific skin temperature site or Tskin when comparing gestational treatments; however, all were increased for adolescent pigs housed in HS conditions compared to TN conditions by 17 to 19% (, ). There were also no gestational by adolescent interactions detected for Tear, Tshoulder, Trump, Ttail or Tskin. Thermal circulation index was similar in adolescent pigs from differing environments when measured at the ear and tail. However, TCI was increased 50 to 52% at the rump and Tskin in heat-stressed pigs compared to pigs in TN conditions. Overall, pigs from GHS had increased TCItail (p = 0.03, 16%) compared to GTN pigs, but this was primarily due to the gestational by adolescent interaction (p = 0.02) indicating that the GHS pigs had a larger increase (150%) during HS compared to GTN pigs (). Adolescent HS tended (p < 0.06) to increase TCIshoulder (32%, ). There was no gestational effect or gestational by adolescent environment interaction detected for TCIshoulder, TCIrump, TCIear, or TCIskin.
Figure 2. Effects of gestational and adolescent thermal environments on the temporal changes in (A) ear skin temperature (Tear), (B) shoulder skin temperature (Tshoulder), (C) rump skin temperature (Trump), and (D) tail skin temperature (Ttail) in growing pigs. Gestational heat stress (GHS), gestational thermal neutral (GTN), adolescent thermal neutral conditions (TN), adolescent heat stress conditions (HS), adolescent conditions (AC). Error bars on day 1 and day 15 of the line indicate ±1 SEM. p Values in each figure represent Tskin differences comparing adolescent TN and HS exposed animals regardless of gestational treatment.
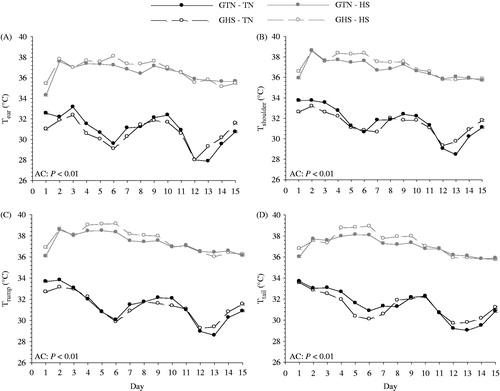
Regression analysis
With regard to the relationship between Tre and RR, GTN pigs had a steeper slope (12 bpm/1 °C) compared to GHS pigs (p < 0.01, ). No gestational environment by adolescent environment interaction differences were detected for this relationship (p = 0.15).
Figure 3. Linear regression (y = mx + b) of respiration rate (RR) as a function of rectal temperature (Tre) for gestational thermal neutral (GTN) and gestational heat stress (GHS) exposed pigs, regardless of adolescent environment. Coefficient of determination (R2), and slope (m) is presented for each regression line.
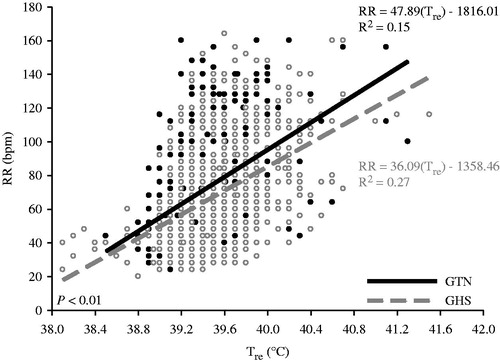
Growth parameters
Overall nutrient intake did not differ between gestational treatments (2.52 kg/day), but it was reduced by 25% (p < 0.01) in the adolescent HS conditions compared to the TN controls (). Animal growth was not affected by gestational thermal treatments (0.70 kg/day), but decreased 34% (p = 0.04) in HS pigs compared to those in TN conditions (). There were no gestational or adolescent treatment effects (p > 0.16) detected for feed efficiency (0.27 kg gain/kg feed, ).
Table II. Effects of gestational and adolescent thermal environments on production parameters in growing pigs.
Discussion
Although HS is a serious economic burden and negatively impacts human health and animal welfare, it is also a primary constraint to efficient livestock growth and development. While this is the case in most geographical areas of North America, it is particularly true in tropical and sub-tropical regions of the world where many developing countries are located [Citation19,Citation20]. As a result, these regions experience extended periods of HS compared to temperate climates (i.e. pigs could be exposed to HS during both gestation and the growing phase), making HS not only an economic issue, but also a serious food security and humanitarian concern [Citation19]. Despite the fact that HS constitutes a well-documented physiological insult with global implications, understanding the epigenetic impact of HS during gestation on future progeny is not well-defined in mammalian species.
A variety of prenatal insults can have permanent effects on postnatal phenotypes and has been evaluated with regard to metabolic dysfunction [Citation10] and teratogenicity [Citation8]. Experimental models such as intrauterine growth retardation indicate that future growth parameters [Citation21,Citation22], body composition [Citation23], and post-absorptive metabolism can be markedly altered by in utero insults [Citation10]. Hales and Barker [Citation24] first introduced the idea of a ‘thrifty phenotype’, whereby fetal malnutrition causes maladaptive programming which results in glucose-sparing metabolism that persists in the offspring. Similar effects can be observed during thermal stress where exposure of unicellular organisms [Citation25], insects [Citation11] and birds [Citation12,Citation13,Citation26,Citation27] to elevated temperatures in previous generations or in ovo alters postnatal survivability and/or body temperature indices. This ‘thermal conditioning’ has also been demonstrated in rodents exposed to HS conditions shortly after birth [Citation14]. This is particularly true for imprinting thermotolerance; defined as the enhanced ability to maintain a lower or safer body temperature in response to a future heat load. However, the degree to which in utero HS affects thermotolerance in mammals during adolescence is not well understood. Herein, we demonstrate that in utero HS impairs the future HS response in a mammalian model, which was contrary to our hypothesis that thermotolerance would have been imprinted.
No overall gestational differences were detected in any body temperature variable during adolescent TN conditions. However, during adolescent HS, pigs derived from heat-stressed first parity pigs had an increase in a variety of body temperature indices, most notably, core temperature (Tre). That gestational differences were only detected in HS conditions is not surprising, as epigenetic alterations impacting body temperature likely need a thermal insult to be expressed. This sort of HS-induced phenotype is similar to what has been reported in poultry [Citation13]. In stark contrast to the aforementioned literature that suggests thermal ‘imprinting’ increases future temperature tolerance, gestationally heat-stressed pigs actually became more hyperthermic during a future HS compared to GTN pigs (). Reasons for the species difference are not clear but of obvious biological and practical interest. Further, given the physiological and phylogenetic similarities between humans and pigs, these data could predict the human response to in utero HS.
The gestation difference in Tre during adolescent HS averaged 0.26 °C and suggests that pigs originating from chronically heat-stressed pregnant first parity pigs are less able to adapt to a future HS compared to pigs derived from TN dams (). The increase in Tre observed in GHS pigs during adolescent HS is corroborated by numerical, but not statistically significant differences in Tskin (). The primary numerical gestational treatment differences in Tskin occurred between days four and nine of the experiment, which temporally coincides with the largest difference in Tre (). As expected, constant adolescent HS markedly increased RR as pigs (in the absence of opportunity for behavioural wetting) utilise panting as their primary route of latent heat loss [Citation3,Citation28]. Despite GHS pigs having increased Tre during HS conditions, RR was similar between gestational treatments within the adolescent HS group. Regardless of the lack of gestation RR differences, the overall relationship (specifically the slope relationship) between Tre and RR was steeper (33%) for GTN compared to GHS pigs. In other words, the RR response to increasing Tre occurred more rapidly in the GTN pigs than for GHS pigs (). The overall blunted respiratory response in GHS animals may have compromised the effectiveness of heat dissipation and resulted in a higher Tre. This may also be due to epigenetic modification(s) that produce an increase in their body temperature ‘set-point’ where the GHS pigs experience a rise in Tre without a subsequent increase in RR or other heat dissipating mechanisms to mitigate the effect. An alternative explanation may be that GHS animals are more tolerant to a higher body temperature and do not need to initiate respiratory cooling mechanisms as quickly as controls.
Consistent with previous reports [Citation3,Citation5,Citation29,Citation30], adolescent HS in this study caused a reduction in a variety of growth variables compared to TN conditions. Specifically, HS reduced final BW (6%), nutrient intake (25%), and BW gain (34%); however, gestational treatment did not statistically impact these variables. Feed efficiency was not influenced by gestational conditions, and surprisingly not affected by adolescent HS. However, this measure is based upon gross body weight change and does not take into account body composition differences, which are important since HS reorganises nutrient partitioning priorities and the hierarchy of tissue synthesis [Citation4].
The Tre increase in the GHS pigs may imply that gestational hyperthermia results in increased susceptibility to future heat loads. Further, the relationship between Tre and RR () suggests that GHS were less effective at utilising enhanced respiration as a means to dissipate excess heat. Consequently, based upon typical measures of thermoregulation, it could be argued that gestational HS compromises tolerance to a future heat load. Yet, despite the increased Tre in response to adolescent HS, pigs derived from in utero HS had similar nutrient intake compared to GTN pigs, suggesting that GHS pigs may have tolerated higher body temperatures better than controls (potentially programmed prenatally). Although no nutrient intake differences were observed for GHS and GTN pigs, ADG was numerically but not statistically reduced (p > 0.15) in GHS compared to GTN pigs by 215 g/day, regardless of adolescent environment. This reduction combined with a possible increase in core temperature set-point has many bioenergetic implications, which if maintained throughout life could result in significant economic losses for the swine industry. If GHS animals are prenatally programmed to maintain an increased core temperature set-point during a future heat load, this would theoretically increase maintenance costs which would have implications for nutrient partitioning, feed efficiency, feed intake, and barn throughput.
Although it is tempting to speculate that GHS animals have an increase in maintenance costs due to greater body temperature (at least during HS), we only measured growth variables to provide a context for the body temperature measurements. Accordingly, the growth data need to be interpreted with care, as determining the effects of gestational HS on future production was not a primary objective and would certainly require more measurements and animals per treatments to make meaningful conclusions. Additionally, whether or not these temperature differences are maintained (or augmented) during a diurnal heat pattern compared to a constant heat load would be of significant interest. Clearly this experiment needs to be repeated with more of an emphasis placed on continuous body temperature indices obtained in both TN and HS conditions. Regardless, the results suggest that the well-documented decrease in nutrient intake and increase in RR associated with increased core body temperature [Citation2,Citation3,Citation5,Citation28,Citation29,Citation31] may be partially influenced by fetal programming. Furthermore, the phenotypic responses to HS are variable [Citation31,Citation32], and it is tempting to hypothesise that a portion of this variation may be due to epigenetic imprinting [Citation28].
Conclusion
Maternal hyperthermia can have lasting effects on developmental patterns. Further, it can alter future growth and behaviour, and we have now demonstrated that it alters the body temperature response to a heat load. Specifically, animals that experienced in utero HS became more hyperthermic in response to a future heat load. This has obvious bioenergetic implications in both human health and animal agriculture. A prerequisite to developing HS mitigation strategies is a better understanding of how HS epigenetically alters physiological responses throughout all stages of the life cycle. Further, how this compromised thermoregulatory response during HS influences metabolic function, future development, and bioenergetics is unknown and will be the focus of upcoming investigations.
Declaration of interest
Results described here within were supported by the Agriculture and Food Research Initiative Competitive Grant no. 2011-67003-30007 from the USDA National Institute of Food and Agriculture. Any opinion, findings, conclusions or recommendations expressed in this publication are those of the authors and do not necessarily reflect the view of the US Department of Agriculture. The authors alone are responsible for the content and writing of the paper.
Acknowledgements
The authors would like to acknowledge the assistance of Levi Long, Emily Ullrich, and Wesley Schweer in collecting temperature and production data, and Anna Gabler for organising animal care and maintenance of facilities.
References
- Kleiber M. The Fire of Life: An Introduction to Animal Energetics. New York: Wiley; 1961. pp 129–74
- Collin A, van Milgen J, Dubois S, Noblet J. Effect of high temperature on feeding behaviour and heat production in group-housed young pigs. Br J Nutr 2001;86:63–70
- Brown-Brandl TM, Nienaber JA, Xin H, Gates RS. A literature review of swine production. Trans Am Soc Ag Eng 2004;47:259–70
- Baumgard LH, Rhoads RP. Effects of heat stress on post-absorptive metabolism and energetics. Annu Rev Anim Bio 2013;1:311–37
- Brown-Brandl TM, Eigenberg RA, Nienaber JA, Kachman SD. Thermoregulatory profile of a newer genetic line of pigs. J Livestock Prod Sci 2001;71:253–60
- Renaudeau D, Giorgi M, Silou F, Weisbecker JL. Effect of breed (lean or fat pigs) and sex on performance and feeding behaviour of group housed growing pigs in a tropical climate. Asian–Aust J Anim Sci 2006;19:593–601
- McGeehin MA, Mirabelli M. The potential impacts of climate variability and change on temperature-related morbidity and mortality in the United States. Environ Health Perspect 2001;109:185–9
- Graham JM, Edwards MJ, Edwards MJ. Teratogen update: Gestational effects of maternal hyperthermia due to febrile illnesses and resultant patterns of defects in humans. Teratology 1998;58:209–21
- Shiota K, Kayamura T. Effects of prenatal heat stress on postnatal growth, behavior and learning capacity in mice. Biol Neonate 1989;56:6–14
- Chen X, Fahy AL, Green AS, Anderson MJ, Rhoads RP, Limesand SW. β2-Adrenergic receptor desensitization in perirenal adipose tissue in fetuses and lambs with placental insufficiency-induced intrauterine growth restriction. J Physiol 2010;588:3539–49
- Sorensen JG, Dahlgaard J, Loeschcke V. Genetic variation in thermal tolerance among natural populations of Drosophila buzzatii: Down regulation of Hsp70 expression and variation in heat stress resistance traits. Funct Ecol 2001;15:289–96
- Tzschentke B. Attainment of thermoregulation as affected by environmental factors. Poult Sci 2007;86:1025–36
- Piestun Y, Schinder D, Ruzal M, Halevy O, Brake J, Yahav S. Thermal manipulations during broiler embryogenesis: Effect on the acquisition of thermotolerance. Poult Sci 2008;87:1516–25
- Tetievsky A, Horowitz M. Posttranslational modifications in histones underlie heat acclimation-mediated cytoprotective memory. J Appl Physiol 2010;109:1552–61
- Lucy MC, Safranski TJ, Rhoades JN, Ross JW, Gabler NK, Rhoads RP, et al. Litter characteristics and thermoregulatory behavior of first parity sows exposed to a controlled heat stress (HS) during gestation. J Anim Sci 2012;90:731
- Federation of Animal Science Societies. Guide for the Care and Use of Agricultural Animals in Research and Teaching. 3rd ed. Champaign, IL: Federation of Animal Science Societies; 2010. Chapter 11, pp. 143--156
- National Research Council. Nutrient Requirements of Swine, 11th ed. Washington, DC: National Academy Press; 2012. pp 101–29
- Curtis S. Environmental Management in Animal Agriculture. Ames, IA: Iowa State University Press; 1983. pp 6–96
- Battisti DS, Naylor RL. Historical warnings of future food insecurity with unprecedented seasonal heat. Science 2009;323:240–44
- Muller C, Cramer W, Hare WL, Lotze-Campen H. Climate change risks for African agriculture. Proc Natl Acad Sci USA 2010;108:4313–15
- Foxcroft GR, Dixon WT, Novak S, Putman CT, Town SC, Vinsky MD. The biological basis for prenatal programming of postnatal performance in pigs. J Anim Sci 2006;84:E105–12
- Foxcroft GR, Dixon WT, Dyck MK, Novak S, Harding JC, Almeida FC. Prenatal programming of postnatal development of the pig. Soc Reprod Fertil Suppl 2009;66:213–31
- Pinney SE, Simmons RA. Epigenetic mechanisms in the development of type 2 diabetes. Trends Endocrinol Metab 2010;21:223–9
- Hales CN, and Barker DJ. Type 2 (non-insulin-dependent) diabetes mellitus: The thrifty phenotype hypothesis. Diabetologia 1992;35:595–601
- Estruch F. Stress-controlled transcription factors, stress-induced genes and stress tolerance in budding yeast. FEMS Micro Bio Rev 2006;24:469–86
- Yahav S. Effect of early-age thermal conditioning and food restriction on performance and thermotolerance of male broiler chickens. Br Poult Sci 1999;40:120–26
- Yahav S, McMurtry JP. Thermotolerance acquisition in broiler chickens by temperature conditioning early in life: The effect of timing and ambient temperature. J Poult Sci 2001;80:1662–66
- Baumgard LH, Rhoads RP, Rhoads ML, Gabler NK, Ross JW, Keating AF, et al. Impact of climate change on livestock production. In: Sejian V, Naqvi SMK, Ezeji T, Lakritz J, Lal R, editors. Environmental Stress and Amelioration in Livestock Production. Berlin: Springer; 2012. pp 413–68
- Renaudeau D, Kerdoncuff M, Anais C, Gourdine JL. Effect of temperature on thermal acclimation in Large White growing pigs. Animal 2008;2:1619–26
- Renaudeau D, Anais C, Tel L, Gourdine JL. Effect of temperature on thermal acclimation in growing pigs estimated using a nonlinear function. J Anim Sci 2010;88:3715–24
- Collin A, van Milgen J, Le Dividich J. Modeling the effect of high, constant temperature on food intake in young growing pigs. Anim Sci 2001;72:519–27
- Angilletta MJ. Thermal Adaptation: A Theoretical and Empirical Synthesis. St Joseph, MN: Oxford University Press; 2009. pp 1–6