Abstract
Purpose: This study was to evaluate the efficacy of subcutaneous administration of 5% dextrose in water (D5W), to prevent skin injury during radiofrequency (RF) ablation. Materials and methods: Twenty-four rabbits were divided into three groups: a pre-injection group, a perfusion group, and a control group. Ablative zones were created in the superficial part of the thigh muscle for 6 min. A needle was placed subcutaneously for injection of D5W, and a thermal sensor was positioned nearby for real-time temperature monitoring. The sizes of the ablative zones were measured by contrast-enhanced ultrasonography, and severity of the observed skin injury were scored semi-quantitatively and compared. Results: The highest temperature, the duration of the temperature above 50 °C, and the rise time of the post-procedure temperature were all highest in the control group (p < 0.001), while these values were lower in the perfusion group than those in the pre-injection group (p < 0.001). Post-procedure skin injury was most severe in the control group (p < 0.001). On post-procedure day 1, no significant difference was found between the skin injury of the pre-injection group and the perfusion group (p = 0.091), while the skin injury of the perfusion group was less severe than that of the pre-injection group on post-procedure day 14 (p = 0.004). No significant difference was found in the sizes of the ablative zones among the groups (p = 0.720). Conclusion: Subcutaneous perfusion with D5W is effective in protecting the skin against burns during RF ablation without compromising the effect of ablation.
Introduction
In recent years, image-guided percutaneous procedures have become viable treatment options for solid focal tumours in superficial organ sites, and thermal ablation has been proven safe and effective with minimal morbidity and mortality [Citation1–6]. Complications related to the procedure include skin burns in 0.27–7.00% of cases, and burns were considered a minor complication in several large series [Citation7–9]. However, from a clinical perspective, skin burns should not be overlooked, especially in patients with immunosuppression, diabetes mellitus, or peripheral vascular disease [Citation10].
To avoid unpredictable thermal injury to adjacent tissues, separation of the ablative zone from the skin using pre-injection of solutions of 0.9% NaCl (saline) and 5% dextrose in water (D5W) has been proposed for fluid insulation [Citation1–3]. However, saline is ionic and encourages radiofrequency (RF) current flow, which would increase the risk of skin burn [Citation11–12]. D5W has a substantially lower electrical conductivity than saline, which creates a high-impedance path between the RF electrode and protected tissue; therefore, D5W should be a better choice for the protection of adjacent structures during RF ablation [Citation12–14]. Moreover, Baek et al. reported that rapid dispersal of fluid occurred between the expected lesion and the skin, and they questioned the protective efficacy of this approach [Citation1]. Additionally, to our knowledge, subcutaneous temperature has not been investigated in prior studies as a critical element for skin burns. The purpose of the present study is to evaluate the efficacy of subcutaneous administration of D5W to prevent thermal skin injury during a radiofrequency (RF) ablative procedure in an in vivo rabbit model.
Materials and methods
Animals
The experimental protocols were approved by the animal care and use committee of the Fifth Affiliated Hospital of Sun-Yat Sen University. All the animals were handled in accordance with the general guidelines for the care of laboratory animals issued by the US National Institutes of Health. Twenty-four New Zealand rabbits (weight range 2.34–2.69 kg, mean weight 2.53 kg, Xinhua Laboratory Animal Centre, Guangzhou, China) were used in this study. All experiments were performed in our animal research facility. The animals were randomly assigned to the following three groups: the pre-injection group (n = 8), the perfusion group (n = 8), and the control group (n = 8).
All experimental procedures were performed with the animals under anaesthesia. Induction of anaesthesia was achieved with 30 mg/kg body weight of pentobarbitalum natricum (Shanghai Chemical Reagent, Shanghai, China) administered through a 20 gauge × 1 inch intravenous (IV) catheter (Becton Dickinson, Suzhou, China) placed in the dorsal auricular vein. After an adequate degree of anaesthesia was achieved, the back of the animal was depilated for placement of a grounding pad (13 × 8 cm). The animal was placed in the supine position, and the inner side of the left thigh was shaved and sterilised. The site chosen for the ablative lesion was the superficial area of the thigh muscle.
Administration of D5W
Under real-time ultrasound (US) guidance using a 7–10-MHz linear-array transducer (LOGIQ E9; GE Healthcare, Wauwatosa, WI, USA), a 22 gauge × 1 inch IV needle (Kangdelai Medical, Wenzhou, China) was inserted into the skin–fat interface for administration of D5W. In animals of the pre-injection group, 3 mL of room temperature (22–24 °C) D5W (Kunming Nanjiang Pharmaceutical, Kunming, Yunnan, China) was pre-injected before RF ablation. In animals belonging to the perfusion group, subcutaneous perfusion of room temperature D5W was administered by an infusion pump (Beyond Medical, Changsha, China), which was connected to an intravenous needle in the skin–fat interface. Immediately at the onset of the ablative procedure, continuous perfusion was initiated at a speed of 0.5 mL/min and continued until 1 min after the ablative procedure was complete. In animals belonging to the control group, RF ablation was performed without the administration of D5W.
Temperature measurement
A 22-gauge fibre-optic thermal sensor (Shandong Micro-Sensor Photonics, Ji’nan, China) was placed under US guidance in the skin–fat interface near the injection needle for real-time temperature monitoring (). The temperature was recorded at 5-s intervals throughout the ablative procedure by means of a temperature display window until 10 min after completion of the procedure. The baseline temperatures and the highest temperatures during the ablative procedure were measured.
Figure 1. Experimental approach. (A) The drawing shows the position of the needle for fluid injection, the thermal sensor, the RF electrode, and the target zone before RF ablation. (B) The drawing shows the distribution of the solution injected subcutaneously, the position of the needle for injection, the thermal sensor, the RF electrode, and the ablative zone during the ablative procedure (35 × 34 mm, 300 × 300 dpi).
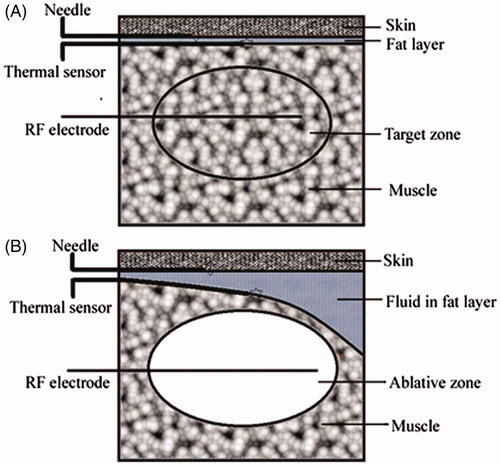
When guinea-pig skin was used as an experimental model, the critical temperature causing permanent and irreversible injury was between 50 °C and 55 °C, and 9 min exposure to 49 °C or 4 min exposure to 51 °C could cause trans-epidermal necrosis [Citation15]. Because there are no available data concerning thermal injury in rabbit skin, and given that the critical temperature for irreversible injury of skin is usually above 50 °C [Citation15–16], the duration of the temperature above 50 °C during the ablative procedure was recorded.
Radiofrequency ablation
An internally cooled RF system (Hokai Medical, Zhuhai, China) was used as the energy source. An 18-gauge single straight tip electrode with a 1-cm exposed tip was used to create the ablative zones in all animals. The electrode was placed in the muscle parallel to the thermal sensor, and a distance of approximately 5 mm between the skin–fat interface and the active tip of the RF electrode was maintained. The RF power was set to 30 W, which was maintained for 6 min. One radiologist (W.Z.L., with 5 years of experience in interventional US) performed all of the procedures under US guidance.
Imaging and data collection
After the ablative procedure, contrast-enhanced ultrasonography (CEUS) was performed to measure the size of the ablative area. A second-generation US contrast agent (sulphur hexafluoride, SonoVue, Bracco, Milan, Italy) was used in this study. During CEUS, the ablative zone was detected as a non-enhancing region [Citation17–18]. The longitudinal dimension (L) was defined as the maximum diameter along the electrode insertion axis, and the transverse dimensions were defined as the two diameters perpendicular to the electrode insertion axis in the transverse plane (i.e. the transverse (T) and antero-posterior dimensions (AP)). The ablative zone was considered as ellipsoid in shape, and the volume of the ablative zones (V) was calculated using the formula, V = L × T × AP × π/6. All CEUS examination results represent the consensus of two radiologists (H.G. and J.Y.L., with 5 years of experience in CEUS) who were blinded to the treatment status.
Skin injury evaluation
Visual inspection of skin changes was performed on post-procedure day 1 and day 14. In contrast to traditional skin burns, which involve heat transfer from the outer to inner skin, the thermal energy in RF ablation is transferred from the inside. Because any thermal skin injury extending beyond the epidermis (deep second- and third-degree) will involve the cutaneous appendages and ultimately result in ulceration and scar formation [Citation19], any ulceration or scar present on the skin was considered total skin damage in this study. The degree of thermal injury on post-procedure day 1 was graded according to a 4-point scoring system (0, none; 1, erythema or vesicle; 2, scar or ulceration <10 mm; and 3, scar or ulceration >10 mm) based on the extent and intensity of the skin damage. Similarly, the degree of thermal injury on post-procedure day 14 was graded according to a 4-point scoring system (0, none; 1, pigmentation; 2, ulceration or scar <10 mm; and 3, ulceration or scar >10 mm). All results of physical examination of the skin represent the consensus of two observers (Y.L.W. and J.Z.X.) who were blinded to the treatment status.
Statistical analysis
Statistical analysis was performed with commercially available software (SPSS version 13.0 for Windows, Chicago, IL, USA). The temperature parameters of the ablative procedure and the measurements of the ablative zones are presented as the means ± standard deviation (M ± SD). One-way analysis of variance was used to determine the differences in these parameters among the groups. In assessing the degree of skin injury on post-procedure day 1 and day 14, the Kruskal-Wallis H test was used to identify the significant difference among the groups. The least significant difference t test was performed for multiple comparisons to determine the differences between individual groups. A difference with a p value <0.05 was considered significant for analysis of variance, and a difference with a p value <0.017 was considered significant for comparisons between individual groups.
Results
RF ablation zones were created in the superficial part of the left thigh muscle abutting the skin in 24 rabbits. Employment of the thermal sensor and subcutaneous injection of D5W were successful in the animals. Due to the longitudinal arrangement of the muscles and fasciae under the skin, subcutaneously injected D5W was observed to disperse to the surrounding tissue. The D5W spread more rapidly in the pre-injection group than in the perfusion group, and more solution was deposited on one side of the ablative zone in the pre-injection group, even at early stages of the ablative procedure. In the perfusion group, the fluid dispersed more slowly and the insulation between the ablative zone and the adjacent skin was thicker than in the pre-injection group during the ablative procedure (). However, after 6 min the spread of the solution in the perfusion group was the same as that observed in the pre-injection group.
Figure 2. Ultrasonography during the RF ablative procedure. Representative images of grey-scale ultrasonography during the RF ablative procedure. (A) The position of the ablative zone and the thermal sensor. (B) The position of the ablative zone and the needle for injection. (C) Distribution of the solution injected in the pre-injection group. (D) Distribution of the solution injected in the perfusion group. The thermal sensor and the needle for injection are viewed as an echogenic line (red arrow). The ablative zone is viewed as an echogenic area (white arrow). The solution injected subcutaneously is viewed as a no-echo region (yellow arrow) with a significantly uneven distribution (54 × 50 mm, 300 × 300 dpi).
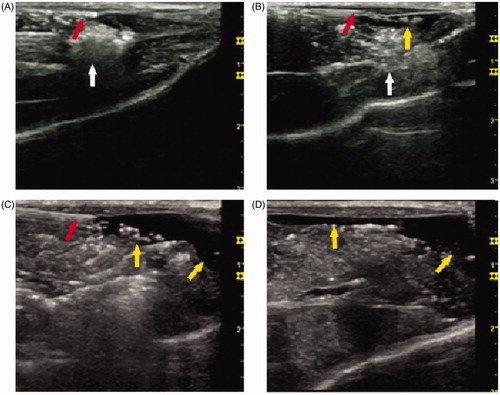
Evaluation of temperature effects
At the onset of RF ablation, the baseline temperature in the pre-injection group was significantly lower than that of the control group (p < 0.001) and the perfusion group (p < 0.001). Following the ablative procedure, the highest temperature in the control group was significantly higher than the corresponding values in the pre-injection group (p < 0.001) and the perfusion group (p < 0.001). The duration of the temperature above 50 °C was longer in the control group than in the pre-injection group (p < 0.001). For animals in the perfusion group, the highest temperature never exceeded 50 °C ().
Table I. Temperature parameters during the RF ablative procedure.
Additionally, we found that the post-procedure subcutaneous temperature continued to rise even after the end of the ablative procedure. In the control and pre-injection groups, the post-procedure temperature exhibited a clear increasing trend followed by a reduction, and the rise time of the post-procedure temperature in the control group was longer than that in the pre-injection group (p < 0.001). For animals in the perfusion group, which had a shorter rise time than the control group (p < 0.001) and the pre-injection group (p < 0.001), the post-procedure temperature dropped quickly after RF ablation (, ).
Evaluation of lesion size
The ablative area was detected as a non-enhancing region by CEUS, and all the ablative zones were located in the superficial area of the muscle abutting the skin (). There was no significant difference in the parameters of the ablative zones among the groups, including the longitudinal (p = 0.632), transverse (p = 0.082), and anteroposterior dimensions (p = 0.840). Similarly, there were no significant differences in the volumes of the ablative zones among the groups (p = 0.720, ).
Figure 4. RF ablative zone detected by contrast-enhanced ultrasonography (CEUS). The representative image shows the ablative zone as a non-enhanced region (white arrow) during the post-procedure examination by CEUS. The ablative zone was located in the muscle abutting the skin (35 × 34 mm, 300 × 300 dpi).
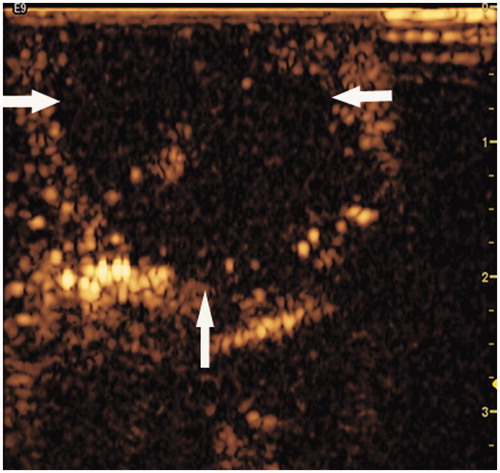
Table II. RF ablation area measurements.
Evaluation of skin injury
On post-procedure day 1, skin changes were evaluated by means of visual inspection in all cases. The most severe skin injury was found in the animals of the control group and included erythema (1/8), vesicles (2/8), and ulceration (5/8). These results were significantly different from those of the pre-injection group (p < 0.001) and the perfusion group (p < 0.001). In the pre-injection and perfusion groups, skin changes were modest, and there was no significant difference between the groups (p = 0.091) (, ).
Figure 5. Visual inspection of skin injuries on post-procedure day 1. Representative photographs of the gross skin injuries on post-procedure day 1. (A) Erythema in the perfusion group. (B) A vesicle in the pre-injection group. (C, D) Ulceration in the control group (54 × 50 mm, 300 × 300 dpi).
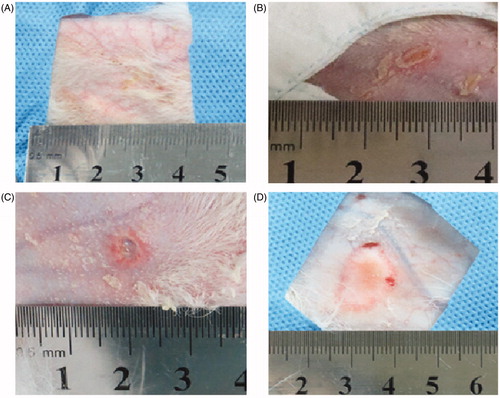
Table III. Visual inspection of skin injuries on post-procedure day 1.
All animals survived for two weeks. The most severe injuries of the skin found in the animals of the control group were scars or ulceration (8/8), which was significantly different from the results in the pre-injection group (p = 0.003) and the perfusion group (p < 0.001). Although administration of D5W was performed in both the pre-injection group and the perfusion group, more skin lesions were found in the pre-injection group (7/8) (p = 0.004). No obvious skin lesion was found in the perfusion group (1/8) except for 1 case of pigmentation (, ).
Figure 6. Visual inspection of skin injuries on post-procedure day 14. Representative photographs of gross skin injuries on post-procedure day 14. (A) Pigmentation in the perfusion group. (B) Ulceration in the pre-injection group. (C, D) Ulceration in the control group (54 × 50 mm, 300 × 300 dpi).
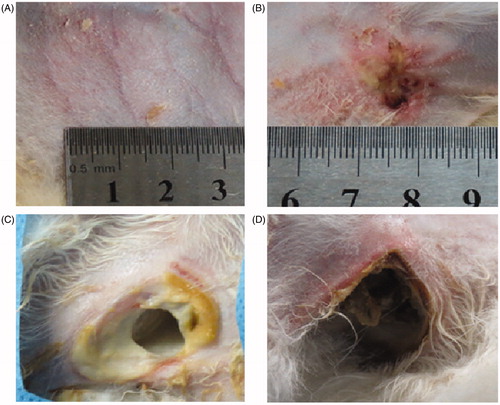
Table IV. Visual inspection of skin injuries on post-procedure day 14.
Discussion
To prevent thermal skin injury during superficial applications of RF ablation, subcutaneous pre-injection of saline and D5W has been widely used to reduce skin burns [Citation1–3]. Subcutaneously pre-injected solutions can cool the skin directly and act as insulation to defend the skin from burns during ablative procedures [Citation1–3]. Additionally, due to the distance-dependent characteristics of thermal energy during RF application [Citation20], enlarging the distance between the ablative zone and the adjacent skin via subcutaneous injection of solution could minimise the thermal skin injury, with complete ablation of the expected lesions. In our study, D5W reduced the thermal skin injury significantly in the pre-injection and perfusion groups, and the efficacy of subcutaneous administration of D5W to minimise thermal skin injury during RF ablation was again demonstrated.
However, in our study, pre-injection of D5W clearly had disadvantages. The pre-injected D5W dispersed rapidly even at the early stage of the ablative procedure, while additional solution deposited on one side of the ablative zone and was not efficacious in defending the skin from thermal injury. Therefore, isolation of the solution between the ablative zone and the skin is not stable enough to defend against thermal skin injury during the ablative procedure when the RF application has a long duration. Similarly, Baek et al. reported the rapid dispersal of fluid when using pre-injection during treatment of thyroid cancer with RF ablation and questioned the protective efficacy of this approach [Citation1]. However, in the animals of the perfusion group, D5W was injected into the subcutaneous tissue constantly and dispersed slowly, which provided more stable subcutaneous insulation to defend the skin from thermal injury, thereby reducing the likelihood of excessive heating of the skin. Additionally, although the fluid does not seem to flow as actively as blood in the vessels to cause the ‘heat sink’ effect, injected D5W could continue moving and result in more heat radiation and more effective thermal buffering to protect the skin against excessive heat. Similarly, the protective effect of perfusion against thermal injury to the hepatic bile ducts and the renal collecting system via simulation of the ‘heat sink’ effect has been demonstrated by many investigators over the last decade [Citation21–23].
In addition, the increase in the post-procedure temperature observed in our study indicates that the risk of thermal skin injury might still exist even after the ablative procedure is complete. This is because heat transfer from the heat source continues even after RF ablation. Meanwhile, persistent heat congestion underlying the skin leads to an accumulation of the oedematous fluid in the dermis [Citation24]. Because oedematous dermis is an effective heat barrier to the subcutaneous tissues, subcutaneous accumulation of heat will be more obvious. To prevent this post-procedural thermal injury of the skin, protective measures were suggested [Citation25]. In our study, the perfusion group had the shortest rise time of the post-procedure temperature of the groups. The rise time was no more than 15 s for animals in this group, which implies that this approach minimises the risk of intra- or post-procedure skin burns more effectively when superficial lesions are treated with RF ablation.
The ‘heat sink’ effect is also a drawback of RF ablation in the treatment of tumours and reduces the size of the ablative zone by vascular dispersal of the deposited heat energy via a rapid stream of low-temperature blood [Citation26–27]. Consequently, we hypothesised that subcutaneous perfusion with D5W, which simulates the ‘heat sink effect’ during RF ablation, might have a role in depriving the ablative area. However, in our study, the sizes of the ablative zones were similar among the groups, as assessed by CEUS examination. This result indicates that RF ablation with subcutaneous perfusion may prevent skin burns effectively, providing equivalent therapeutic efficacy in superficial RF ablation therapy.
To prevent unpredictable thermal injury to adjacent tissues, D5W has been proposed for local fluid insulation in the treatment of breast cancer with RF ablation. In these studies, the volume of D5W injected exceeded 10 mL, and no additional pain or potential toxicity was associated with the administration of D5W [Citation2–3]. D5W has also been proposed for local insulation in the treatment of other diseases with RF ablation, such as renal and hepatic tumours and retroperitoneal metastatic disease [Citation21,Citation22,Citation28–30]. In addition, intra-peritoneal D5W helped decrease post-procedural pain after treatment of peripheral liver tumours with RF ablation [Citation28].
Based on the above observations, we conclude that subcutaneous perfusion with D5W is effective in reducing thermal skin injury during the RF ablative procedure due to the following factors. 1) Perfusion of D5W involves constant infusion of low-temperature fluid during the entire ablative procedure to reduce the heat underneath the skin without significant early dispersal of solution into the deeper tissues. Such dispersal would act as a non-coolant, as observed during the pre-injection method. 2) Perfusion with D5W could provide more stable insulation and effective thermal buffering to reduce the likelihood of excessive heating of the adjacent skin. 3) Injected D5W remains moving as a result of homogenous subcutaneous heating and leads to better radiation of subcutaneous heat energy. Although the injected fluid was also heated and would reheat the surrounding tissues as it moves, our results show that the temperature of the heated D5W was not hot enough to injure the adjacent tissues.
In our study, histopathological analysis of the thermal skin injury was not performed because the analysis could be a source of error in the incidence of skin injury if executed during the short period of time after the ablative procedure. Because this study was designed to evaluate gross skin changes that resulted from RF ablation, microscopic observation was beyond its scope. This type of protection must be evaluated in a clinical study investigating appropriate procedures for the long-term follow-up period.
Conclusion
In conclusion, subcutaneous pre-injection of D5W has been widely used to prevent skin burns during RF ablation. D5W has several theoretical and practical advantages, and subcutaneous perfusion with D5W can be more effective in reducing thermal skin injury during RF ablation without impairing the ablative efficacy. Additional clinical studies of the skin protection effectiveness are warranted.
Declaration of interest
The authors report no conflicts of interest. The authors alone are responsible for the content and writing of the paper.
Acknowledgements
We thank Xian-ping Yi from the Department of Pathology of the Fifth Affiliated Hospital of Sun Yat-Sen University (Zhuhai, China) for advice on the manuscript revision.
References
- Baek JH, Kim YS, Sung JY, Choi H, Lee JH. Locoregional control of metastatic well-differentiated thyroid cancer by ultrasound-guided radiofrequency ablation. Am J Roentgenol 2011;197:W331–6
- Kinoshita T, Iwamoto E, Tsuda H, Seki K. Radiofrequency ablation as local therapy for early breast carcinomas. Breast Cancer 2011;18:10–7
- Imoto S, Wada N, Sakemura N, Hasebe T, Murata Y. Feasibility study on radiofrequency ablation followed by partial mastectomy for stage I breast cancer patients. Breast 2009;18:130–4
- Izzo F, Thomas R, Delrio P, Rinaldo M, Vallone P, DeChiara A, et al. Radiofrequency ablation in patients with primary breast carcinoma: A pilot study in 26 patients. Cancer 2001;92:2036–44
- Baek JH, Lee JH, Valcavi R, Pacella CM, Rhim H, Na DG. Thermal ablation for benign thyroid nodules: Radiofrequency and laser. Korean J Radiol 2011;12:525–40
- Alvarado R, Mahon B, Valadez C, Caufield M, Wadhwani S, Hambleton C, et al. Thermal ablation of the goat mammary gland as a model for post-lumpectomy treatment of breast cancer: Preliminary observations. Int J Hyperthermia 2009;25:47–55
- Baek JH, Lee JH, Sung JY, Bae JI, Kim KT, Sim J, et al. Complications encountered in the treatment of benign thyroid nodules with US-guided radiofrequency ablation: A multicenter study. Radiology 2012;262:335–42
- Zhao Z, Wu F. Minimally-invasive thermal ablation of early-stage breast cancer: a systemic review. Eur J Surg Oncol 2010;36:1149–55
- Na DG, Lee JH, Jung SL, Kim JH, Sung JY, Shin JH, et al. Radiofrequency ablation of benign thyroid nodules and recurrent thyroid cancers: Consensus statement and recommendations. Korean J Radiol 2012;13:117–25
- Huffman SD, Huffman NP, Lewandowski RJ, Brown DB. Radiofrequency ablation complicated by skin burn. Semin Intervent Radiol 2011;28:179–82
- Romero-Mendez R, Tobajas P, Burdio F, Gonzalez A, Navarro A, Grande L, et al. Electrical-thermal performance of a cooled RF applicator for hepatic ablation with additional distant infusion of hypertonic saline: In vivo study and preliminary computer modelling. Int J Hyperthermia 2012;28:653–62
- Laeseke PF, Sampson LA, Winter TC III, Lee FT Jr. Use of dextrose 5% in water instead of saline to protect against inadvertent radiofrequency injuries. Am J Roentgenol 2005;184:1026–7
- Laeseke PF, Sampson LA, Brace CL, Winter TC III, Fine JP, Lee FT Jr. Unintended thermal injuries from radiofrequency ablation: protection with 5% dextrose in water. Am J Roentgenol 2006;186:S249–54
- Brace CL, Laeseke PF, Prasad V, Lee FT. Electrical isolation during radiofrequency ablation: 5% dextrose in water provides better protection than saline. Conf Proc IEEE Eng Med Biol Soc 2006;1:5021–4
- Moritz AR, Henriques FC. Studies of thermal injury: II. The relative importance of time and surface temperature in the causation of cutaneous burns. Am J Pathol 1947;23:695–720
- Moritz AR. Studies of thermal injury: III. The pathology and pathogenesis of cutaneous burns. An experimental study. Am J Pathol 1947;23:915–41
- Lei F, Jing Z, Bo W, Dongmei H, Zhencai L, Xue J, et al. Uterine myomas treated with microwave ablation: The agreement between ablation volumes obtained from contrast-enhanced sonography and enhanced MRI. Int J Hyperthermia 2014;30:11–8
- Liu F, Yu X, Liang P, Cheng Z, Han Z, Dong B. Contrast-enhanced ultrasound-guided microwave ablation for hepatocellular carcinoma inconspicuous on conventional ultrasound. Int J Hyperthermia 2011;27:555–62
- Andrews ML, Robertson I, Weedon D. Cutaneous manifestations of chronic graft-versus-host disease. Australas J Dermatol 1997;38:53–62; quiz 63–4
- Mertyna P, Dewhirst MW, Halpern E, Goldberg W, Goldberg SN. Radiofrequency ablation: The effect of distance and baseline temperature on thermal dose required for coagulation. Int J Hyperthermia 2008;24:550–9
- Hwang SI, Cho JY, Kim SH, Jun SR, Lee HJ, Moon KC, et al. Protection of the renal collecting system during radiofrequency ablation with antegrade cold dextrose infusion. Radiology 2010;256:759–66
- Cantwell CP, Wah TM, Gervais DA, Eisner BH, Arellano R, Uppot RN, et al. Protecting the ureter during radiofrequency ablation of renal cell cancer: A pilot study of retrograde pyeloperfusion with cooled dextrose 5% in water. J Vasc Interv Radiol 2008;19:1034–40
- Raman SS, Aziz D, Chang X, Ye M, Sayre J, Lassman C, et al. Minimizing central bile duct injury during radiofrequency ablation: Use of intraductal chilled saline perfusion – Initial observations from a study in pigs. Radiology 2004;232:154–9
- Henriques FC, Moritz AR. Studies of thermal injury: I. The conduction of heat to and through skin and the temperatures attained therein. A theoretical and an experimental investigation. Am J Pathol 1947;23:530–49
- Marcy PY, Magne N, Castadot P, Bailet C, Namer M. Ultrasound-guided percutaneous radiofrequency ablation in elderly breast cancer patients: Preliminary institutional experience. Br J Radiol 2007;80:267–73
- Nan Q, Zheng W, Fan Z, Liu Y, Zeng Y. Analysis to a critical state of thermal field in microwave ablation of liver cancer influenced by large vessels. Int J Hyperthermia 2010;26:34–8
- Liu Z, Ahmed M, Sabir A, Humphries S, Goldberg SN. Computer modelling of the effect of perfusion on heating patterns in radiofrequency tumour ablation. Int J Hyperthermia 2007;23:49–58
- Hinshaw JL, Laeseke PF, Winter TC 3rd, Kliewer MA, Fine JP, Lee FT Jr. Radiofrequency ablation of peripheral liver tumors: Intraperitoneal 5% dextrose in water decreases postprocedural pain. Am J Roentgenol 2006;186:S306–10
- Chen EA, Neeman Z, Lee FT, Kam A, Wood B. Thermal protection with 5% dextrose solution blanket during radiofrequency ablation. Cardiovasc Intervent Radiol 2006;29:1093–6
- Arellano RS, Flanders VL, Lee SI, Mueller PR, Gervais DA. Imaging-guided percutaneous radiofrequency ablation of retroperitoneal metastatic disease in patients with gynecologic malignancies: clinical experience with eight patients. Am J Roentgenol 2010;194:1635–8