Abstract
Background: Diode laser-based skin heating has been shown to minimise scars by interfering with wound healing responses through the induction of heat shock proteins (HSP). HSP are also induced after ablative fractional laser (AFXL) wound healing. AFXL itself is highly recommended for scar treatment. Therefore, the sequential combination of both modalities may produce superior outcomes.
Objectives: The aim of this study was to examine the pretreatment effects of a diode laser before AFXL on wound healing responses in terms of HSP up-regulation in an in vitro model.
Materials and methods: Immediate responses and responses on days 1, 3 or 6 post-procedure were studied in an in vitro porcine skin model (n = 240). Untreated samples served as control. Immunohistochemical investigation (Hsp70) was performed in all untreated controls, diode laser-, AFXL-, and in diode laser + AFXL-treated samples.
Results: Hsp70 was shown to be up-regulated by all interventions between days 1 and 6 after interventions. The largest effect was caused by the combination of a diode laser and an AFXL procedure.
Conclusion: Diode laser exposure induces a skin HSP response that can be further enhanced by sequential AFXL treatment. Clinical studies are necessary to investigate the dose response of HSP on scar formation and refine suitable laser exposure settings.
Introduction
The human skin becomes marked by scars over a lifetime as a result of injuries, diseases and surgeries. There are currently a wide range of options available to treat scars including lasers [Citation1], and scar prevention is possible if used prior to surgery [Citation2]. Thermal lasers can effectively promote skin wound healing [Citation3]. The main mechanism is attributed to 810–815 nm diode laser-driven temperature elevation, which modifies the wound-healing process by inducing heat shock proteins (HSPs) for up to 7 days [Citation4]. During this period, cell repair and wound healing are initiated and collagen remodelling begins [Citation5]. HSPs also play important roles in mediating death signalling [Citation6], inflammation and cell proliferation during wound healing and suggest there are alternative functions during scarring [Citation7].
HSPs belong to a large family of protein chaperones involved in assisting protein folding and unfolding in cells. In addition to the induction by hyperthermia, HSPs are activated by a wide range of physical, chemical and biological stresses including various laser therapies or light irradiation such as PDT [Citation8]. The most important HSPs are the family members of Hsp70 (72 kDa = Hsp72 and 73 kDa = Hsp73) and Hsp47. Both Hsp72 and Hsp73 are present in the cytoplasm and nucleus of keratinocytes, fibroblasts and adipocytes. Hsp73 is synthesised constitutively in all mammalian cells and is often referred to as the ‘constitutive Hsp70’. The synthesis of Hsp72 is usually restricted to cells experiencing stress and is referred to as the ‘inducible Hsp70’. The two proteins exhibit extremely high sequence homology (95%) and have similar biochemical properties [Citation9].
Fractional laser treatments with photodynamic therapy (PDT) and thermal lasers cause a strong induction of Hsp70 within the epidermal layer [Citation10–12]. Fractionated laser therapies are routinely used in the clinic to treat scars and many other conditions [Citation13]. By treating the skin with fractions, a response is initiated that involves the skin replacing itself in up to 50% of the surface if the individual piece of skin removed is smaller than ∼0.3 mm in diameter. These columns of treated skin can reach as deep as the dermal compartment and are called microscopic treatment zones (MTZ) or microscopic ablation zones (MAZ). On a cellular level, post-fractional wound healing is defined by a stereotypical sequence of cellular responses that are also triggered by the activation of HSPs within the remaining keratinocytes [Citation14]. The response culminates in an active expulsion of pigmented irreparable tissue parts (microscopic epidermal necrotic debris (MEND)) 3 days later [Citation15]. Dermal remodelling then begins to remove the scar tissue [Citation16]. However, the dose response of HSP to scar formation is not known.
The aims of this in vitro study were (1) to prove the ability of an 808 nm linear scanning diode laser treatment to induce Hsp70 expression in skin and (2) to evaluate whether this effect may be more pronounced if a secondary ablative fractional laser treatment (AFXL) is added. If this hypothesis can be proven, an early intervention that induces Hsp70 might improve technologies to reduce scarring and improve wound healing in the future. It is possible that early and intensified induction of Hsp70 may improve wound healing.
Materials and methods
A prospective, single-centre in vitro porcine study was designed to examine HSP expression from single and combined laser exposures [Citation17,Citation18]. The study protocol used a previously described model and conformed to the ethical guidelines of the 1975 Declaration of Helsinki. This study was approved by the institutional animal research review committee.
Skin explants and laser systems
Ten raw porcine (Yorkshire, 3 month of age) flank low pigmented skin samples approximately 0.8 cm in thickness were used immediately after surgery and fat removal to create a total of 240 aliquots (). Each sample was kept 37 °C on a heating plate and subjected to six treatment regimens and subdivided into four parts post intervention: (1) untreated control, (2) diode laser treatment, (3) AFXL fractional CO2 laser, (4) AFXL fractional Er:YAG laser, (5) diode laser treatment prior to AFXL CO2 laser and (6) diode laser treatment prior to AFXL Er: YAG laser.
Table 1. Expression levels of Hsp70 given as median–maximum (rating of staining intensity as follows: 0 = undetected, 1 = low density, 2 = medium density, 3 = dense to 4 = very dense). Ten repetitions investigated on day 3 result in 40 samples per intervention.
The laser diode settings were chosen based on previous studies [Citation4]. The diode laser exposure was performed in two passes with a linear scanning 808 nm device (LEDA EPI, Quantel-Derma, now Alma Lasers, Nümberg, Germany). The treatment area was 10 × 12 mm, and the pulse duration was 18 ms. A fluence of 51 J/cm2 was used to heat the skin per pass.
AFXL was performed with a 10,600 nm CO2 laser (EXELO2, formerly Quantel-Derma now Alma Lasers) and a 2,940 nm Er:YAG laser (Burane FXL, formerly Quantel-Derma now Alma Lasers, optic lens array FX12).
The fractionated CO2 laser was operated with a scanner, using a spot diameter of 250 μm. The pulse duration (exposure time) was 1 ms, and the pulse energy of 40 mJ was delivered by 1 stack and 1 pass at a density of 250 MAZ/cm2. The average fluence in each MAZ generated by a scanner was 81.6 J/cm2 (spot area 0.049 mm2; 40 mJ/0.049 mm2 = 0.04 J/0.00049 cm2 = 81.6 J/cm2). The average fluence with the treatment area is calculated as 40 mJ × 250 MAZ/cm2 = 10,000 mJ/cm2 = 10 J/cm2.
The fractionated Er:YAG laser was operated with an FX12 optic a lens array, density of 270 MAZ/cm2, providing a spot diameter of 150 μm. The laser was set to 31.8 mJ pulse energy with a 300 ms pulse duration (exposure time), consisting of 10 stacked subpulses of 3.18 mJ each. The average fluence with the treatment area is calculated as 31.8 mJ × 270 MAZ/cm2 = 8,586 mJ/cm2 = 8.6 J/cm2. Therefore the average fluencies in each treatment area are comparable, despite differences in absorption characteristics.
Routine pathology work-up
Each skin sample was further subdivided into four parts post intervention. The skin samples were then incubated with cell culture medium (DMEM, enriched with streptavidin and 10% fetal calf serum in Petri dishes) for 1, 3 or 6 days at constant temperatures of 31–32 °C, which corresponds to the average skin surface temperature. Day 0 samples were evaluated immediately without culturing.
Immunohistochemistry
The tissue sections were rehydrated and then incubated with 0.5% BSA/PBS (phosphate-buffered saline/bovine serum albumin: 0.5% BSA prepared in PBS) for 5–10 min at 41–43 °C. The sections were washed twice in PBS for 10 min per wash and air-dried. The sections were then incubated in a wet chamber for another 30 min at room temperature. The reaction was stopped with 25–50 μL of normal sheep serum, and the slides were washed twice in PBS for 10 min per wash. The primary antibody was anti-Hsp70 (catalogue no: AM289-5 M, specific for Hsp70), which recognises both the constitutive (Hsp73) and inducible (Hsp72) forms of Hsp70 (BioGenex, San Ramon, CA; 1:1 PBS/0.1% Tween). Anti-Hsp70 was added to the sections and incubated in a wet chamber for 45 min at 37 °C. To visualise mAb binding, the Dako Real Detection System (K5005, Dako, Hamburg, Germany, Alkaline Phosphatase/Red, Rabbit/Mouse) was used according to the manufacturer’s protocol. Non-specific Hsp70 antibody binding was ruled out by omitting the primary antibody.
Evaluation of the immunohistochemistry staining
All tissue samples were stained at the same time using identical procedures to provide comparable results, and the slides were evaluated by blinded reviewers. Hsp70 expression was analysed microscopically by two investigators using different magnifications (Olympus BX41, Germany, magnification: 1.25, 4, 10, 20, 40, 60×) and documented using a calibrated digital camera system (Olympus DP72) together with the software evaluation package (Olympus Cell F). The expression densities of Hsp70 in skin explants were scored using the following scale: 0 = undetected, 1 = low density, 2 = medium density, 3 = dense or 4 = very dense as described previously by Souil et al. [Citation4]. Any disagreement between assessors was resolved by re-evaluation of samples until an agreement was reached.
Results
A total of 240 porcine skin aliquots were generated by immunohistochemistry for qualitative and semi-quantitative assessment of HSP expression from individual and combined laser treatment with diode laser and ablative fractional lasers ().
In general, no specific macroscopic changes in the tissue samples were visible despite the stereotypical ablation zones post AFXL. On a microscopic level, despite the MAZ including the coagulation zones, there were no specific changes detectable.
The overall qualitative assessments by immunohistochemistry revealed the highest expression of Hsp70 in the epidermal compartment and minor expression was found in the dermal papillary layer around the sebaceous glands, hair follicles, and blood vessels. Hsp70 expression was weak or absent in the corneal layer and in adipose tissue. The laser interventions induced a steady temporal increase of Hsp70 in the epidermis and Hsp70 expression intensified from days 0, 1, 3 to 6 ().
Figure 1. Hsp70 immunoreactivity in response to various treatment modalities (1) control, no treatment, (2) diode laser treatment only, (3) AFXL using the fractional CO2 laser (AFXL CO2, EXELO2, spot size: 250 μm, 10.600 nm), (4) AFXL using the fractional Er:YAG laser (AFXL Er:YAG, Burane FXL, optic array FX12, 2.940 nm), (5) diode laser treatment prior to AFXL with either CO2 or (6) the Er:YAG laser at day 0 (directly after intervention), day 1 (1 day after intervention), day 3 (3 days after intervention), and day 7 (7 days after intervention). The expression densities of Hsp70 in skin explants were scored using the following scale: 0 = undetected, 1 = low density, 2 = medium density, 3 = dense or 4 = very dense (e.g. day 6 diode + AFXL Er:YAG).
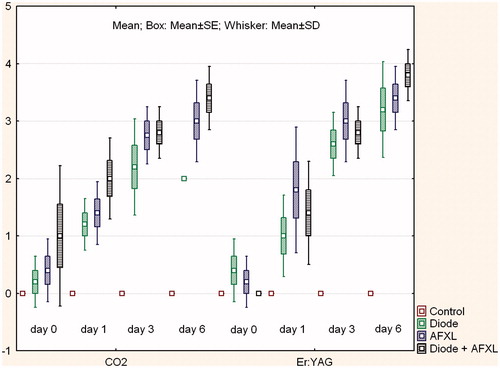
Figure 2. Box-plot (mean (middle point) ± SE (Box)/SD (Whisker)) of Hsp70 immunoreactivity (rating of staining intensity as follows: 0 = undetected, 1 = low density, 2 = medium density, 3 = dense to 4 = very dense) in response to various treatment modalities (control = no treatment, diode = diode laser treatment, AFXL = ablative fractional laser treatment using either the fractional CO2 laser (AFXL CO2, EXELO2, spot size: 250 μm, 10.600 nm), or the fractional Er:YAG laser (AFXL Er:YAG, Burane FXL, optic array FX12, 2.940 nm).
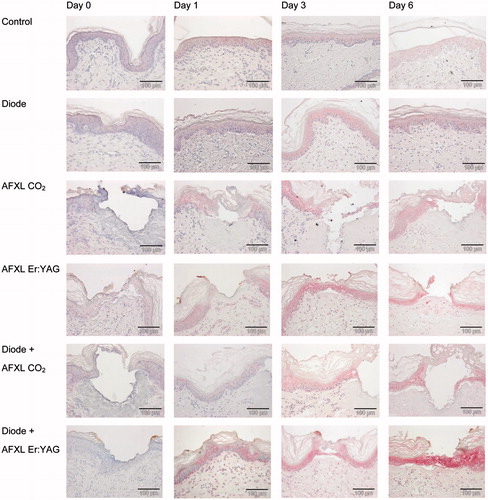
Based on semi-quantitative assessments, the untreated control samples had only minor Hsp70 expression. The diode laser exposure resulted in steady increases of Hsp70 expression, which increased over time and peaked by days 3 (median 2.5) and 6 (median 2) (). AFXL interventions displayed similar trends of time-related Hsp70 up-regulation during the post intervention phase. Thus, both CO2 and Er:YAG AFXL laser interventions reached median scores of 3 on days 3 and 6. The combined application of the diode laser followed by an AFXL procedure revealed the highest expression of Hsp70 on day 6. The median score was 4 for both combinations ().
Discussion
Diode laser-based skin heating has been shown to minimise scars by interfering with wound healing responses through the induction of heat shock proteins [Citation2]. HSPs are also key factors in post-ablative fractional laser (AFXL) wound healing [Citation10–12]. AFXL itself is recommended for scar treatment as early as possible [Citation13]. Thus, the sequential combination of both modalities may induce superior outcomes. The aim of this in vitro study was to prove the ability of linear scanned diode lasers to (1) induce Hsp70 expression and (2) determine whether this effect is more pronounced when the skin is subjected to a secondary ablative fractional laser treatment (AFXL).
Our results demonstrate a similar long-lasting in vitro HSP response of skin explants following a linear scanned diode laser treatment as shown previously with systems of comparable wavelengths and system settings [Citation2,Citation4]. It is unclear whether the extent of HSP induction is further elevated at higher fluences or after repetitive passes. The AFXL treatment using either fractional CO2 or an Er:YAG laser resulted in a strong HSP induction over time and was comparable to previous results. Due to the relative similarity of heat-shock response, the results represent trends rather than being significant and clear-cut.
The skin explant model has been proven to reflect the situation in vivo to some extent [Citation17,Citation18]. The in vivo situation might be significantly different in part due to blood flow and the resulting presence of haemoglobin as a potential chromophore as well as convective cooling by circulation. The diode laser interventions before the AFXL treatment with the CO2 and the Er:YAG laser resulted in clear enhancement of epidermal Hsp70 expression compared to the diode or AFXL treatments alone.
The latter finding might have clinical implications. Diode lasers and fractional lasers have a wide distribution and a great acceptance due to their safety profile and ease of use [Citation13,Citation19]. An early intervention using diode laser might help to increase the results and safety profile of AFXL.
Conclusion
This in vitro study clearly demonstrated that a conventional linear scanning hair removal laser can be adjusted for use in skin heating. Skin heating has been shown to prevent scarring if used prior to surgery in studies of others. This concept can be easily integrated into daily surgical procedures before the actual intervention starts because the effect last for some time. Laser epilation systems often use the wavelengths of interest so it is possible to explore this fascinating field of research and develop better wound healing and scar treatments. Despite this also other source of energy might be suitable for use e.g. intense pulsed light, radio frequency and other modalities.
Using this concept, we were able to show synergistic effects of diode laser in conjunction with AFXL using either a fractional Er:YAG laser or a fractional CO2 laser. A synergistic effect could also be proposed for the treatment of scars of different types because the fractional lasers have been proven to be effective in this field. However, the dose response of HSP to scar formation is not known. Too much HSP or thermal damage might even potentially increase scar formation. Clinical studies are necessary to investigate the dose response of HSP on scar formation and refine suitable laser exposure settings.
Declaration of interest
Uwe Paasch received unrestricted research grants from Quantel-Derma. The linear scanning 808 nm diode laser was loaned from Quantel-Derma. Uwe Paasch and Sonja Grunewald served as consultants for Quantel-Derma GmbH, now Alma Lasers GmbH. The authors alone are responsible for the content and writing of the paper.
References
- Oliaei S, Nelson JS, Fitzpatrick R, Wong BJ. Laser treatment of scars. Facial Plast Surg 2012;28:518–24
- Capon A, Iarmarcovai G, Gonnelli D, Degardin N, Magalon G, Mordon S. Scar prevention using laser-assisted skin healing (LASH) in plastic surgery. Aesthetic Plast Surg 2010;34:438–46
- Capon A, Mordon S. Can thermal lasers promote skin wound healing? Am J Clin Dermatol 2003;4:1–12
- Souil E, Capon A, Mordon S, Dinh-Xuan AT, Polla BS, Bachelet M. Treatment with 815-nm diode laser induces long-lasting expression of 72-kDa heat shock protein in normal rat skin. Br J Dermatol 2001;144:260–6
- Laplante AF, Moulin V, Auger FA, Landry J, Li H, Morrow G, et al. Expression of heat shock proteins in mouse skin during wound healing. J Histochem Cytochem 1998;46:1291–301
- Beere HM, Wolf BB, Cain K, Mosser DD, Mahboubi A, Kuwana T, et al. Heat-shock protein 70 inhibits apoptosis by preventing recruitment of procaspase-9 to the Apaf-1 apoptosome. Nat Cell Biol 2000;2:469–75
- Souil E, Capon A, Mordon S, Dinh-Xuan AT, Polla BS, Bachelet M. Treatment with 815-nm diode laser induces long-lasting expression of 72-kDa heat shock protein in normal rat skin. Br J Dermatol 2001;144:260–6
- Kiang JG, Tsokos GC. Heat shock protein 70 kDa: Molecular biology, biochemistry, and physiology. Pharmacol Ther 1998;80:183–201
- Welch WJ. Mammalian stress response: Cell physiology, structure/function of stress proteins, and implications for medicine and disease. Physiol Rev 1992;72:1063–81
- Helbig D, Bodendorf MO, Grunewald S, Kendler M, Simon JC, Paasch U. Immunohistochemical investigation of wound healing in response to fractional photothermolysis. J Biomed Opt 2009;14:064044
- Laubach HJ, Tannous Z, Anderson RR, Manstein D. Skin responses to fractional photothermolysis. Lasers Surg Med 2006;38:142–9
- Helbig D, Simon JC, Paasch U. Photodynamic therapy and the role of heat shock protein 70. Int J Hyperthermia 2011;27:802–10
- Ong MW, Bashir SJ. Fractional laser resurfacing for acne scars: A review. Br J Dermatol 2012;166:1160–9
- Helbig D, Paasch U. Molecular changes during skin aging and wound healing after fractional ablative photothermolysis. Skin Res Technol 2011;17:119–28
- Manstein D, Herron GS, Sink RK, Tanner H, Anderson RR. Fractional photothermolysis: A new concept for cutaneous remodeling using microscopic patterns of thermal injury. Lasers Surg Med 2004;34:426–38
- Ozog DM, Liu A, Chaffins ML, Ormsby AH, Fincher EF, Chipps LK, et al. Evaluation of clinical results, histological architecture, and collagen expression following treatment of mature burn scars with a fractional carbon dioxide laser. JAMA Dermatol 2013;149:50–7
- Helbig D, Moebius A, Simon JC, Paasch U. Nonablative skin rejuvenation devices and the role of heat shock protein 70: Results of a human skin explant model. J Biomed Opt 2010;15:038002
- Helbig D, Bodendorf M, Anderegg U, Simon JC, Paasch U. Human skin explant model to study molecular chances in response to fractional photothermolysis: Spatio-temporal expression of HSP70. Med Laser Application 2010;25:173–80
- Haedersdal M, Wulf HC. Evidence-based review of hair removal using lasers and light sources. J Eur Acad Dermatol Venereol 2006;20:9–20