Abstract
Purpose: The response of tissues to radiation with mild temperature hyperthermia is dependent on the interval between the two modalities. This study investigated the effect that the vascular disrupting agent OXi4503 had on this time-interval interaction.
Methods: The normal right rear foot of female CDF1 mice or foot-implanted C3H mammary carcinomas were locally irradiated (230 kV X-rays) and heated (41.5 °C for 60 min) by foot immersion in a water bath. OXi4503 (50 mg/kg) was injected intraperitoneally 1.5 h before irradiating. Irradiation was performed either in the middle of the heating period (simultaneous treatment) or at 1 or 4 h prior to starting the heating (sequential treatments). Response was the percentage of mice showing local tumour control at 90 days or skin moist desquamation between days 11–23. From the radiation dose response curves the dose producing tumour control (TCD50) or moist desquamation (MDD50) in 50% of mice was calculated.
Results: The TCD50 and MDD50 values for radiation alone were 54 Gy and 29 Gy, respectively. Simultaneously heating the tissues enhanced radiation response, the respective TCD50 and MDD50 values being significantly (chi-square test, p < 0.05) reduced to 33 Gy and 14 Gy. A smaller enhancement was obtained with a sequential treatment in both tissues. OXi4503 enhanced the radiation response of tumour and skin. Combined with radiation and heat, the only effect was in tumours where OXi4503 prevented the decrease in sensitisation seen with the sequential treatment.
Conclusion: Combining OXi4503 with a sequential radiation and heat treatment resulted in a 1.4-fold therapeutic gain.
Introduction
Hyperthermia is probably one of the most effective agents for enhancing the response of tumours to radiation. This has clearly been demonstrated in numerous pre-clinical studies [Citation1]. The enhancement observed is dependent on several factors that include the sequence and timing between hyperthermia and radiation, the greatest effect being observed with a simultaneous administration of both modalities [Citation1]. It also depends on the heating temperature and the time of heating [Citation1]. Based on the successful pre-clinical studies, a large number of clinical trials were initiated, but few trials actually demonstrated a clear benefit [Citation1–3]. This lack of success in many clinical studies may partly be attributed to the fact that a truly simultaneous heat and radiation treatment is not yet technically possible. But it has also been suggested to result from a failure to adequately heat tumours [Citation4]. Considerable effort has therefore been made to find additional treatments that could be applied to improve tumour heating.
One area that has received considerable attention in this context is the tumour vascular supply [Citation5] since the blood supply of tissues and the effect of heat are closely interrelated. In tumours, hyperthermia exerts some of its effects by inducing damage to vasculature [Citation6,Citation7]. However, the vascular supply also influences tumour response to heat treatment, and here blood flow is probably one of the most critical factors. It is a major vehicle by which heat is dissipated, thus affects the ability to heat the tumour [Citation8,Citation9]. The blood supply also plays an important role in determining the type of microenvironment that exists within tumours, and cells that exist under poor microenvironmental conditions are known to be more sensitive to killing by heat [Citation10,Citation11]. Attempts to decrease tumour blood flow and so improve the efficacy of heat have thus been applied. These include physical clamping [Citation12–14] and physiological modifiers [Citation15–17]. Alternatively, attempts have been made to use agents that specifically target tumour vasculature using drug-based approaches that either inhibit the angiogenesis process [Citation18–21], or that damage the already established tumour vessels [Citation22–27]. This latter group of so-called vascular disrupting agents (VDAs) have also been applied to enhance tumour response to radiation [Citation28–30], thus the concept of combining VDAs with thermoradiation treatments would appear to be a logical next step. Indeed, the potential benefit of such an approach has been demonstrated using flavonoid compounds such as flavone acetic acid (FAA) [Citation31] and 5,6-dimethylxanthenone-4-acetic acid (DMXAA) [Citation32], tubulin binding agents such as combretastatin [Citation33] and its derivative OXi4503 [Citation34], and anti-cancer agents such as arsenic trioxide [Citation35].
Those studies that combined VDAs with heat and radiation involved injecting the VDA some 30–60 min after irradiating and then heating at some later time point. The aim of the current study was to extend those investigations to determine the effect VDAs could have on the time interval between radiation and heat when using a mild temperature heat treatment of 41.5 °C for 60 min. This heat treatment was selected and defined as mild because it was the lowest temperature and heating time that produced a measurable enhancement of radiation response in the same tumour model as used in this study [Citation1]. The VDA selected to investigate this interaction was OXi4503. This drug is a second generation analogue of combretastatin A-4 phosphate (CA4P), the leading VDA in clinical development [Citation36]. Both CA4P and OXi4503 are tubulin binding agents that can induce vascular damage in tumours [Citation37]. However, unlike CA4P, OXi4503 also undergoes oxidative activation to a quinone intermediate, and this gives OXi4503 additional direct cell-killing properties [Citation38]. OXi4503 is now also under preliminary clinical evaluation [Citation36]. As in previous studies the tumour model used was our well-established C3H mammary carcinoma with the end point being a clinically relevant one of local tumour control. Furthermore, since the timing between radiation and heat can also influence normal tissue reactions [Citation1], we monitored the effect of the combinations on the development of moist desquamation in normal skin and compared the tumour and normal tissue effects to establish the greatest therapeutic benefit.
Methods
Animal and tumour model
Experiments were performed using 10–14-week-old female CDF1 mice. These were either normal mice or animals that had a C3H mammary carcinoma implanted in the right rear foot. This tumour model is an anaplastic adenocarcinoma that arose spontaneously in a C3H mouse at our institute and was originally designated as HB [Citation39]; the name was changed to C3H mammary carcinoma when it was grown in the more stable CDF1 mouse variant [Citation40]. C3H mammary carcinomas do not grow in culture, thus experimental tumours were produced following sterile dissection of large flank tumours as previously described [Citation41]. Basically, macroscopically viable tumour tissue was minced with scissors and 5–10 µL of this material was implanted into the foot. Treatments were started when foot tumours reached a volume of 200 mm3, which generally occurred within 3 weeks after inoculation, tumour volume being determined by the formula D1 × D2 × D3 × π/6 (where the D values represent three orthogonal diameters). All animal studies were conducted according to the animal welfare policy of Aarhus University (http://dyrefaciliteter.au.dk), with the Danish Animal Experiments Inspectorate’s approval.
Drug preparation
OXi4503 was supplied by Oxigene (South San Francisco, CA, USA). It was freshly prepared before each experiment by dissolving in a sterile saline solution (0.9% NaCl). Stock solutions were kept cold and protected from light. OXi4503 was injected intraperitoneally (i.p.) in a volume of 0.02 mL/g mouse body weight.
Radiation treatments
Irradiations were given with a conventional therapeutic Philips X-ray machine (230 kV, 15 mA, 2-mm Al filter, 1.1-mm Cu half-value layer, dose rate of 2.3 Gy/min) as previously described [Citation42]. Dosimetry was accomplished by use of an integrating chamber. All treatments to mice feet were administered to non-anaesthetised animals placed in a specially constructed jig that restrained them, but allowed their right rear legs to be exposed for irradiation. This jig consisted of a 6.5-cm tube of clear Lucite that had a diameter slightly larger than the animal’s body and was attached to a flat base. The head end of the tube was sealed but had holes in it to allow air circulation, while the opposite end was open thus allowing for the right rear leg to be pulled to the side and exposed. By attaching the mouse tail to the tube with tape and applying additional tape across the rear end of the mouse the animal remained in the jig. For tumour irradiations, the right leg of the mouse was attached to part of the jig base that extended approximately 2 cm to the right; this part was around 1 cm wide. The upper region of the right leg was then attached to this extension with tape. This was loosely applied so that the mouse could move its foot a few millimetres to the left or right, but the physical presence of the tumour prevented the animal from withdrawing its leg during irradiation. During irradiation, homogeneity of the radiation dose was achieved by immersing the mouse foot in a water bath with about 5 cm of water between the X-ray source and the tumour. This was possible because the water bath was covered with a Lucite plate with holes in it; by turning the jig on its side the foot could be immersed approximately 1 cm below the water surface. Only the mouse foot was irradiated, the remainder of the animal being shielded by lead. With the normal foot skin irradiations, the absence of a tumour meant that it was not possible to loosely attach the leg to the jig with tape. To fix the leg in the correct position for irradiation, histoacrylic glue was used [Citation33]. A small drop of glue was placed on the jig in the region of the uppermost part of the leg and the leg firmly compressed against the jig with tape for 5 min. After this time the tape was loosened and the mouse left for a further 10 min before any further treatment was started. Using this procedure irradiations could be performed without the need for anaesthetics. The leg was easily detached from the jig after treatment.
Hyperthermia treatments
As with the radiation treatments, hyperthermia was given locally to the right rear foot of restrained, non-anaesthetised, tumour or non-tumour bearing, mice. This has been described previously [Citation33]. Briefly, the legs of the animals were attached to the jigs as described for the radiation treatments and hyperthermia delivered by immersing the right rear leg into a circulating water bath (TE 623, Heto, Birkerød, Denmark) as also described previously [Citation33]. Previous measurements of intratumoural temperature have shown stabilisation within 1 to 2 min to approximately 0.2 °C below the water bath temperature [Citation16]. The temperature of the water bath was therefore adjusted to 41.7 °C, 0.2 °C above the desired tumour temperature of 41.5 °C. An identical temperature water bath setting was used for the skin score studies, thus the actual skin temperature may have been slightly higher than that of the tumour, but certainly no greater than 0.2 °C, since the water bath temperature was 41.7 °C.
Response end points and analysis
The response of tumours to treatment was assessed using a local tumour control assay, and for skin damage the end point was the development of moist desquamation, as described previously [Citation43]. For the local tumour control assay this involved observing mice on a weekly basis up to 90 days post-treatment and recording the number of animals in each treatment group (there were an average of 10 mice per group) showing tumour control within this 90-day period. With the skin damage assessment, mice were observed on a daily basis from days 11–23, and the number of animals in each group (average of seven mice per group) that developed moist desquamation was recorded. Both the tumour control and moist desquamation data were subjected to logit analysis, and from the radiation dose–response curves the radiation doses producing tumour control (TCD50) or moist desquamation (MDD50) in 50% of mice were calculated along with the 95% confidence intervals (CI). These TCD50 and MDD50 values were converted into enhancement ratios (ERs) by using the ratio of the response for radiation alone to that for radiation plus OXi4503 and/or heat. The therapeutic gain factor (TGF) for the different treatments was calculated from the ratio of the ERs for the tumour and normal skin. Statistical analysis of all data was achieved using a chi-square test, with a significance level of p < 0.05.
Results
The radiation dose–response curve showing local control in this C3H mammary carcinoma following irradiation alone is shown in . From this data, a TCD50 value (with 95%CI) was calculated to be 54 Gy (52–57). Also shown is the effect of combining radiation with either OXi4503, a simultaneous heat treatment, or when this heat treatment and OXi4503 were combined. A single injection of OXi4503 (50 mg/kg) 1.5 h prior to irradiating moved the dose–response curve to the left and resulted in a statistically significant reduced TCD50 value of 48 Gy (46–51). Irradiating tumours in the middle of mild temperature heating (41.5 °C for 60 min) produced an even greater effect, with the TCD50 reduced to 33 Gy (31–35). No additional effect on radiation response was found when OXI4503 and heating were combined, the TCD50 being 32 Gy (29–35). These TCD50 values, along with those obtained with all the other treatment combinations are summarised in . When an interval was allowed between the time of irradiation and the start of heating there was a substantial decrease in the degree of enhancement of the radiation response. A considerable drop was seen with an interval of 60 min, which then seemed to remain relatively constant up to 4 h. This decrease in effect with increasing interval between radiation and heat was not observed when mice were injected with OXi4503 prior to irradiating, such that the effect of this VDA on the 1- or 4-h radiation–heat interval resulted in TCD50 values that were not significantly different from a truly simultaneous radiation and heat treatment.
Figure 1. The effect of OXi4503 (50 mg/kg) and mild temperature hyperthermia (41.5 °C, 60 min) on the radiation response of a C3H mammary carcinoma. (A) Radiation dose response curves for local tumour control in tumours treated with radiation alone (△), OXi4503 injected 1.5 h before irradiating (▴), radiation administered in the middle of heating (○), or the combination of OXi4503, radiation and heat (•). Points are for an average of 10 mice with the lines drawn following logit analysis. (B) The TCD50 doses are a function of time relative to irradiation, and are taken from the curves shown in (A) and for other similar dose–response curves. Results are for radiation alone (
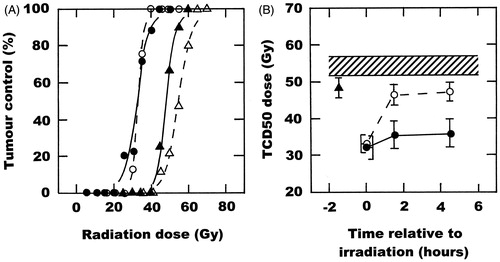
shows the results for similar studies using a skin reaction end point of the development of moist desquamation. The MDD50 value for radiation only obtained from the radiation dose–response curve in was found to be 29 Gy (28–30). When OXi4503 was injected before irradiating there was an extremely small yet statistically significant enhancement of the radiation effect with the MDD50 now being 28 Gy (27–29). Similar to the tumour findings there was a statistically significant effect from performing a simultaneous radiation and heat treatment, with the MDD50 reduced to 14 Gy (12–15). This response was only marginally, yet again statistically significant, decreased to 12 Gy (11–13) by injecting OXi4503 prior to the radiation and heat treatment. The MDD50 values for these curves and all the other treatments are shown in . As with the C3H mammary carcinoma, the enhanced effect of combining radiation and heat decreased as the time interval between irradiation and heating increased, reaching a level equivalent to that seen for radiation alone with a 4-h interval. The inclusion of OXi4503 with both the 1 - and 4-h interval between the radiation and heat treatments had absolutely no additional effect on skin response.
Figure 2. The effect of OXi4503 (50 mg/kg) and mild temperature hyperthermia (41.5 °C, 60 min) on the radiation response of normal foot skin. (A) Radiation dose response curves for moist desquamation in skin treated with radiation alone (△), OXi4503 injected 1.5 h before irradiating (▴), radiation administered in the middle of heating (○), or the combination of OXi4503, radiation and heat (•). Points are for an average of seven mice with the lines drawn following logit analysis. (B) The MDD50 doses are a function of time relative to irradiation, and are taken from the curves shown in (A) and for other similar dose–response curves. Results are for radiation alone (
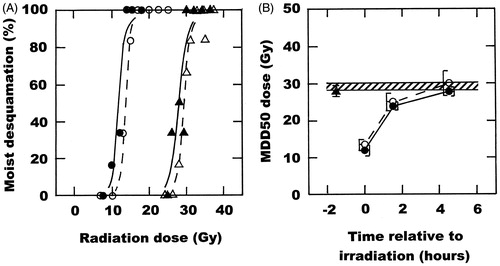
The enhancement ratios obtained when the different treatments were compared to the response for radiation alone in both the C3H mammary carcinoma and normal foot skin are shown in . These values confirm what we have already shown in and regarding the effects of OXi4503 and/or heat on radiation response. From these enhancement ratios it is possible to directly compare the effects in the tumour and normal skin by determining therapeutic gain factors. The results obtained here show no therapeutic benefit with a simultaneous radiation and heat treatment or when these treatments were separated by a 1-h interval; the therapeutic gain factor was never greater than 1.0. Only with the 4-h interval was the tumour effect significantly greater statistically than that seen in normal skin; the therapeutic gain factor was 1.13. When OXi4503 was combined with radiation and heat a similar lack of any therapeutic benefit is seen with the simultaneous radiation and heat treatment, but a statistically significant benefit was observed with both the 1- and 4-h interval, and this increased with time, the therapeutic gain being 1.24 with the 1-h interval and 1.43 at 4 h.
Table 1. Comparison of the effects of OXi4503 and/or mild temperature hyperthermia on the radiation response of the C3H mammary carcinoma or normal foot skin.
Discussion
Our results showed that when radiation and heat were combined the greatest enhancement of tumour response was seen with simultaneous treatment (). With the introduction of any interval between radiation and heat the enhancement is reduced, and the longer the interval the greater this reduction. This is a well known finding having been shown previously in numerous pre-clinical studies [Citation1]. The same trend has also been reported by others in normal tissues [Citation1] and is also shown in the current study for skin (). But, unlike tumours, with normal tissues a 4-h interval results in absolutely no enhancement. This is believed to be because a simultaneous effect is the result of sensitisation by heat, and that will be found in any tissue [Citation1]. The exact mechanism by which heat sensitises cells to radiation is not known, but most evidence suggests that heat primarily interferes with the cells’ ability to deal with radiation-induced DNA damage [Citation44,Citation45]. The effect of a sequential 4-h interval is generally considered to be the result of heat simply killing those tumour cells that are radiation resistant. It has been well documented that the radiation resistant population in tumours are the oxygen deficient hypoxic cells [Citation46]. Additional in vitro studies have demonstrated that hypoxic cells are also sensitive to killing by heat [Citation10,Citation11]. Those pre-clinical studies also showed that cells at low pH were also heat sensitive [Citation10,Citation11]. In tumours, the diffusion gradients for oxygen and nutrients are somewhat similar [Citation47], thus regions of hypoxia and acidity are most likely found together. Such cells will only be present in tumours; skin is also known to contain regions of hypoxia [Citation48], but this would not be expected to be associated with acidic conditions. From a therapeutic standpoint it will only be beneficial to give a simultaneous radiation and heat treatment if one can categorically only treat the tumour and not hit normal tissues. If this cannot be avoided then a sequential treatment would be preferred to avoid unwanted treatment-induced side effects.
Numerous pre-clinical studies have shown that VDAs can be used to enhance tumour radiation response [Citation28–30]. In those studies the VDAs were always given after irradiating. This was done to avoid possible radiation protection that could be caused by any induction of tumour hypoxia resulting from the vascular damage. Indeed, one study has recently shown that VDAs do induce hypoxia and that if the drugs are given prior to irradiating the anti-tumour effect of radiation is diminished [Citation49]. The exception to this was OXi4503 [Citation49]. It induces vascular damage in tumours [Citation37] and can increase the level of tumour hypoxia [Citation49]. But it also undergoes oxidative activation to a quinone intermediate, which gives it direct cell-killing properties [Citation38]. As such it probably kills any hypoxic cells that are induced. Such an effect would clearly account for the finding that OXi4503 is equally effective at enhancing radiation damage whether given before or after irradiating [Citation49]. Thus, in the current study we were able to administer OXi4503 prior to irradiating; without administering the drug this way we could not investigate the effect on simultaneous radiation and heat treatment. Our results confirmed the ability of OXi4503 to enhance radiation response. In the limited studies undertaken in normal tissues, no increase in radiation response has ever been reported by VDAs [Citation29], presumably because VDAs are unlikely to damage normal tissue vessels. However, in our current study there was a small, albeit statistically significant, enhancement of skin damage. Whether this was related to any cytotoxic effect of OXi4503 in this normal tissue is not clear and certainly needs further investigation, but the effect in skin did appear to be lower than that seen in tumours (), although the difference was not significant.
VDAs can also enhance tumour response to hyperthermia [Citation22–27]. This is probably the result of the vascular shut-down leading to a better tumour heating, as well as an increase in the more heat sensitive adverse microenvironmental conditions [Citation27]. VDAs also enhance thermoradiation [Citation31–35]. In fact, the combination of VDAs with thermoradiation using a sequential radiation and heat schedule is so effective that the enhancement obtained with a temperature as low as 41.5 °C is actually greater than that obtained with 43 °C in the absence of a VDA [Citation1]. What is more noteworthy from the current study is that OXi4503 does not really influence the response of normal tissue to the combination of radiation and heat regardless of the time interval between irradiating and heating. The effect of OXi4503 on simultaneous radiation and heating in our C3H mammary carcinoma was also minimal. But this drug did prevent the reduction in the enhancement observed when an interval was introduced between radiation and heat, such that the enhancement ratio of 1.11 seen with a 4-h interval was increased to 1.52 when OXi4503 was added to the combination, and this was not significantly different from the 1.64 value obtained with a truly simultaneous radiation and heat treatment (). Since there was no effect in skin with this 4-h interval a large therapeutic gain factor of 1.43 was obtained. Whether the same benefit is likely with other VDAs is not known, although tumour enhancement ratios in the range of 1.4–1.9 have been reported with both tubulin binding agents and flavonoid compounds [Citation1].
Overall, these results clearly suggest that combining a VDA with a sequential radiation and heat treatment could have potential clinical application for treating primary tumours. Using such local treatments one might not expect any effects on metastatic disease. However, a recent pre-clinical study suggested that treating a primary melanoma tumour with mild temperature hyperthermia (with or without radiation) and then targeting the chronically hypoxic cells in that tumour with the hypoxic cell cytotoxin tirapazamine not only influenced the primary tumour but could actually decrease the number of lung metastases in that mouse model [Citation50]. Since tumour cells that are already oxygen deprived are likely to be those that die first following the vascular shut-down induced by OXi4503, it is interesting to speculate whether combining OXi4503 with thermoradiotherapy could also influence metastatic disease.
Acknowledgements
The author would like to thank Dorthe Grand, Pia Schjerbeck, and Inger Marie Horsman for excellent technical assistance, and Marianne Verner Bjerre and Marianne Kristiansen for animal care. This study was made possible by financial support from the Danish Cancer Society, the Danish Council for Independent Research, Medical Sciences, and the Danish Hyperthermia Fund.
Declaration of interest
The author reports no conflict of interest and is entirely responsible for the content and writing of this paper.
References
- Horsman MR, Overgaard J. Hyperthermia: A potent enhancer of radiotherapy. Clin Oncol 2007;19:418–26
- Wust P, Hildebrandt B, Sreenivasa G, Rau B, Gellermann J, Riess H, et al. Hyperthermia in combined treatment of cancer. Lancet Oncol 2002;3:487–97
- Franckena M. Review of radiotherapy and hyperthermia in primary cervical cancer. Int J Hyperthermia 2012;28:543–8
- Oleson JR. Hyperthermia from the clinic to the laboratory: A hypothesis. Int J Hyperthermia 1995;11:315–22
- Horsman MR. Tissue physiology and the response to heat. Int J Hyperthermia 2006;22:197–203
- Vaupel PW, Kelleher DK. Blood flow and associated pathophysiology of uterine cervix cancers: Characterisation and relevance for localised hyperthermia. Int J Hyperthermia 2012;28:518–27
- Meng Q, He C, Shuaib A, Wang CX. Hyperthermia worsens ischaemic brain injury through destruction of microvessels in an embolic model in rats. Int J Hyperthermia 2012;28:24–32
- Jain RK, Grantham FH, Gullino PM. Blood flow and heat transfer in Walker 256 mammary carcinoma. J Natl Cancer Inst 1979;62:927–33
- Patterson J, Strang R. The role of blood flow in hyperthermia. Int J Radiat Oncol Biol Phys 1979;5:235–41
- Overgaard J, Bichel P. The influence of hypoxia and acidity on the hyperthermic response of malignant cells in vitro. Radiol 1977;123:511–14
- Gerweck LE, Nygaard TG, Burlett M. Response of cells to hyperthermia under acute and chronic hypoxic conditions. Cancer Res 1979;39:966–72
- Thrall DE, Gillette EL, Dewey WC. Effect of heat and ionizing radiation on normal and neoplastic tissue of the C3H mouse. Radiat Res 1975;63:363–77
- Dewey WC, Thrall DE, Gillette EL. Hyperthermia and radiation – A selective thermal effect on chronically hypoxic tumor cells in vivo. Int J Radiat Oncol Biol Phys 1977;2:99–103
- Wallen CA, Colby TV, Stewart JR. Cell kill and tumor control after heat treatment with and without vascular occlusion in RIF-1 tumors. Radiat Res 1986;106:215–23
- Urano M, Montoya V, Booth A. Effect of hyperglycemia on the thermal response of murine normal and tumor tissue. Cancer Res 1983;43:453–5
- Horsman MR, Christensen KL, Overgaard J. Hydralazine-induced enhancement of hyperthermic damage in a C3H mammary carcinoma in vivo. Int J Hyperthermia 1989;5:123–36
- Prescott DM, Samulski TV, Dewhirst MW, Page RL, Thrall DE, Dodge RK, et al. Use of nitroprusside to increase tissue temperature during local hyperthermia in normal and tumor-bearing dogs. Int J Radiat Oncol Biol Phys 1992;23:377–85
- Yano T, Tanase M, Watanabe A, Sawada H, Yamada Y, Shino Y, et al. Enhancement effect of an anti-angiogenic agent, TNP-470, on hyperthermia-induced growth suppression of human esophageal and gastric cancers transplantable to nude mice. Anticancer Res 1995;15:1355–8
- Nishimura Y, Murata R, Hiraoka M. Combined effects of an angiogenesis inhibitor (TNP-470) and hyperthermia. Br J Cancer 1996;73:270–4
- Eikesdal HP, Bjorkhaug ST, Dahl O. Hyperthermia exhibits anti-vascular activity in the BT4An rat glioma: Lack of interaction with the angiogenesis inhibitor batimastat. Int J Hyperthermia 2002;18:141–52
- Verhulst J. Effects of bevacizumab and hyperthermia in a rodent model of hyperthermic intraperitoneal chemotherapy (HIPEC). Int J Hyperthermia 2013;29:62–70
- Kallinowski F, Moehle R, Vaupel P. Substantial enhancement of tumor hyperthermic response by tumor necrosis factor. In: Sugahara T, Saito M, editors. Hyperthermic Oncology, Vol. 1. London: Taylor & Francis; 1989. pp 258–9
- Lin JC, Park HJ, Song CW. Combined treatment of IL-α and TNF-α potentiates the antitumour effect of hyperthermia. Int J Hyperthermia 1996;12:335–44
- Griffin RJ, Lee SH, Rood KL, Stewart MJ, Lyons JC, Lew YS, et al. Use of arsenic trioxide as an antivascular and thermosensitizing agent in solid tumors. Neoplasia 2000;2:555–60
- Griffin RJ, Monzen H, Williams BW, Park H, Lee SH, Song CW. Arsenic trioxide induces vascular damage via oxidative stress and increases thermosensitivity of tumours. Int J Hyperthermia 2003;19:575–89
- Horsman MR, Murata R. Combination of vascular targeting agents with thermal and radiation therapy. Int J Radiat Oncol Biol Phys 2002;54:1518–23
- Horsman MR, Murata R. Vascular targeting therapies and hyperthermia. In: Siemann DW, editor. Vascular-Targeted Therapies in Oncology. Chichester: Wiley; 2006. pp 137–57
- Siemann DW, Warrington KH, Horsman MR. Vascular targeting agents: Adjuvants to radiation therapy. Radiother Oncol 2000;57:5–12
- Horsman MR, Siemann DW. Pathophysiological effects of vascular targeting agents and the implications for combination with conventional therapies. Cancer Res 2006;66:11520–39
- Siemann DW, Horsman MR. Vascular targeted therapies in oncology. Cell Tissue Res 2009;335:241–8
- Horsman MR, Murata R, Overgaard J. Improving local tumor control by combining vascular targeting drugs, mild hyperthermia and radiation. Acta Oncol 2001;40:497–503
- Murata R, Horsman MR. Tumour specific enhancement of thermoradiotherapy at mild temperatures by the vascular targeting agent 5,6-dimethylxanthenone-4-acetic acid. Int J Hyperthermia 2004;20:393–404
- Murata R, Overgaard J, Horsman MR. Combretastatin A-4 disodium phosphate: A vascular targeting agent that improves the anti-tumor effects of hyperthermia, radiation and mild thermoradiotherapy. Int J Radiat Oncol Biol Phys 2001;51:1018–24
- Hokland S, Horsman MR. The new vascular disrupting agent combretastatin A-1 disodium phosphate (OXi4503) enhances tumour response to mild hyperthermia and thermoradiosensitisation. Int J Hyperthermia 2007;23:599–606
- Griffin RJ, Williams BW, Koonce NA, Bischof JC, Song CW, Asur R, et al. Vascular disrupting agent arsenic trioxide enhances thermoradiotherapy of solid tumors. J Oncol 2012. doi: 10.1155/2012/934918
- Clémenson C, Chargari C, Deutsch E. Combination of vascular disrupting agents and ionizing radiation. Crit Rev Oncol Hematol 2013;86:143–60
- Salmon HW, Siemann DW. Effect of the second-generation vascular disrupting agent OXi4503 on tumor vascularity. Clin Cancer Res 2006;12:4090–4
- Folkes LK, Christlieb M, Madej E, Stratford MRL, Wardman P. Oxidative metabolism of combretastatin A-1 produces quinine intermediates with the potential to bind to nucleophiles and to enhance oxidative stress via free radicals. Chem Res Toxicol 2007;20:1885–94
- Overgaard K, Overgaard J. Investigations on the possibility of a thermic tumour therapy – 1. Short-wave treatment of a transplanted isologous mouse mammary carcinoma. Eur J Cancer 1972;8:65–78
- Overgaard J. Simultaneous and sequential hyperthermia and radiation treatment of an experimental tumor and its surrounding normal tissue in vivo. Int J Radiat Oncol Biol Phys 1980;6:1507–15
- Horsman MR, Murata R, Breidahl T, Nielsen FU, Maxwell RJ, Stødkilde-Jørgensen H, et al. Combretastatins: Novel vascular targeting drugs for improving anti-cancer therapy. Adv Exp Med Biol 2000;476:311–23
- Horsman MR, Murata R. Vascular targeting effects of ZD6126 in a C3H mouse mammary carcinoma and the enhancement of radiation response. Int J Radiat Oncol Biol Phys 2003;57:1047–55
- Horsman MR, Chaplin DJ, Overgaard J. Combination of nicotinamide and hyperthermia to eliminate radioresistant chronically and acutely hypoxic tumour cells. Cancer Res 1990;50:7430–6
- Kampinga HH, Dikomey E. Hyperthermic radiosensitization: Mode of action and clinical relevance. Int J Radiat Biol 2001;77:399–408
- Roti Roti JL. Introduction: Radiosensitization by hyperthermia. Int J Hyperthermia 2004;20:109–14
- Moulder JE, Rockwell S. Hypoxic fractions of solid tumors. Int J Radiat Biol Oncol Phys 1984;10:695–712
- Vaupel P, Kallinowski F, Okunieff P. Blood flow, oxygen and nutrient supply, and metabolic micro-environment of human tumors: A review. Cancer Res 1989;49:6449–65
- Hendry JH. Quantitation of the radiotherapeutic importance of naturally-hypoxic normal tissues from collated experiments with rodents using single doses. Int J Radiat Oncol Biol Phys 1979;5:971–6
- Iversen AB, Busk M, Horsman MR. Induction of hypoxia by vascular disrupting agents and the significance for their combination with radiation therapy. Acta Oncol 2013;52:1320–6
- Masunaga S-I, Liu Y, Sakurai Y, Tanaka H, Suzuki M, Kondo N, et al. Usefulness of combined treatment with continuous administration of tirapazamine and mild temperature hyperthermia in γ-ray irradiation in terms of local tumour response and lung metastatic potential. Int J Hyperthermia 2012;28:636–44