Abstract
Regional hyperthermia is described as a targeted therapy and the definitions of six hallmarks of hyperthermia are proposed, representing the pleiotropic effect of this therapeutic modality to counteract tumour growth and progression. We recommend the considerations of these hallmarks in the design of clinical trials involving regional hyperthermia as targeted therapy. Randomised clinical studies using loco-regional hyperthermia as an adjuvant to radiotherapy or to chemotherapy for locally advanced tumours demonstrate the benefit of the combination compared to either of the standard treatments alone for tumour response, disease control, and patient survival outcome. These impressive results were obtained from proof-of-concept trials for superficial or deep-seated malignancies in unselected patients. None of these trials was designed as tailored approaches for the treatment of specified targets or to select potentially more sensitive subpopulations of patients using eligibility criteria. Based upon clinical examples of targeted chemotherapy, some guidelines are described for the successful development of targeted therapeutic combinations. We also retrospectively analyse the stepwise process of generating an ongoing new clinical trial using hyperthermia as targeted therapy to evade DNA repair in combination with a DNA damaging anticancer agent to implement this new vision.
Introduction
Well-designed clinical trials taking into account the hallmark capabilities of malignant cells and tissues are fundamental in the field of modern oncology to gain the therapeutic benefit for cancer patients. The identification of multiple signalling pathways as key drivers of oncogenesis, the discovery of the phenomenon of oncogene addiction, and the resolution of relevant genetic profiles are only examples that provided guidelines for the development of targeted therapies and became the backbone of successful clinical applications for some tumour entities. Here we first describe regional hyperthermia as a targeted therapy and we propose the definition of six hallmarks of hyperthermia, which represent the pleiotropic effect of this therapeutic modality to counteract tumour growth and progression (see Ref. Citation3–7). Randomised clinical studies using loco-regional hyperthermia as an adjuvant to radiotherapy or to chemotherapy for locally advanced tumours demonstrated the benefit of the combination compared to either of the standard treatments alone for tumour response, disease control, and patient survival outcome (see Ref. Citation3–7). These impressive results were obtained from proof-of-concept trials for superficial or deep-seated malignancies in unselected patients. None of these trials were designed as tailored approaches for the treatment of specified targets or to select potentially more sensitive subpopulations of patients using eligibility criteria (see Ref. Citation21–27). Based upon clinical examples of targeted chemotherapy, we describe some guidelines for the successful development of targeted therapeutic combinations. We also retrospectively analysed the stepwise process of generating an ongoing new clinical trial using hyperthermia as targeted therapy to evade DNA repair in combination with a DNA damaging anticancer agent to implement this new vision.
Regional hyperthermia as targeted therapy
Targeting the heating field to the imaged area of the localised tumour, including the immediate surrounding tissue, might be an appropriate definition of ‘regional hyperthermia’ as a clinical intervention within the field of cancer thermal therapy ().
Figure 1. Regional hyperthermia as targeted therapy. Schematic illustration of regional hyperthermia (39–43 °C) targeting the heating field to the area of an intra-abdominal, deep-seated malignant tumour. The external heating device is represented by an open applicator surrounding the body axis of the patient. The increased understanding to control energy deposition in vivo has led to the design of commercially available multi-antenna applicators and the implementation of systems for monitoring temperature distribution within the heated tumour and the tumour-associated stroma.
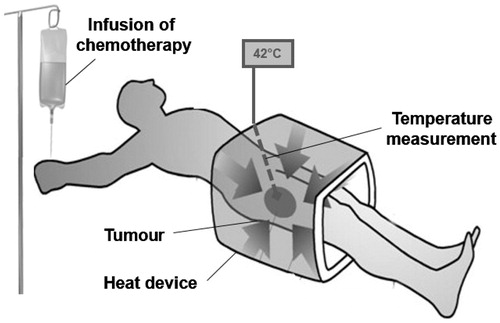
The spatial temperature distribution is heterogeneous (range 39–43 °C) because of energy deposition and conversion into temperature elevation. The site, size, depth, and blood supply of each individual tumour are the major determinants of the temperature distribution. Attempts to achieve more homogeneous high temperature profiles (e.g. application of higher electromagnetic field energy) failed primarily because of the anatomic location, physiological barriers, or increased toxicity [Citation1,Citation2]. However, the application of regional hyperthermia in randomised clinical trials as an adjuvant intervention to standard radiotherapy and chemotherapy regimens demonstrated the benefit in higher response rates, local control, and survival [Citation3–7]. Loco-regional hyperthermia using commercially available equipment to heat deep-seated malignancies is feasible, safe and effective if the application follows treatment guidelines such as the European technical guidelines for quality assurance [Citation8]. The complementary effect of standard treatment is likely due to the enhanced efficacy of either ionising radiation or chemotherapeutic agents at the temperatures achieved in the different areas of heated tumours. Poorly vascularised areas are hypoxic and may reach higher cytotoxic temperatures (42.5–43 °C) because of the less efficient thermal convection by the blood flow, and malignant cells within these areas may be killed by heat alone. These hypoxic areas are more resistant to radiotherapy and chemotherapy. Thermal enhancement of both standard treatments within the entire range of heterogeneous tumour temperatures (39–43 °C) was documented in vitro and in vivo [Citation9].
Fundamental mechanistic research in the field of molecular cell biology, physiology, and immunology led to fundamental discoveries of the pleiotropic effect of heat on malignant cells and tumour tissue. Time–temperature-dependent and cell-cycle-dependent clonogenic cell killing was documented as the predominant effect of heat exposure on cells nearly 50 years ago [Citation10]. This discovery was followed by the discovery of thermal sensitisation of ionising radiation as a selective thermal effect on chronically hypoxic tumour cells in vivo [Citation11].
The outstanding contributions from scientists and clinicians to the field of hyperthermia research (see Ref. Citation12–18), especially the past two decades, led us to propose six important features as ‘hallmarks of hyperthermia’: (1) blocking cell survival, (2) inducing cellular stress response, (3) modulating immune response, (4) evading DNA repair, (5) changing tumour microenvironment, and (6) sensitisation to radiation and chemotherapy ().
Figure 2. The hallmarks of hyperthermia. This illustration encompasses six hallmark capabilities induced by heat. The past two decades have witnessed fundamental discoveries toward understanding the mechanistic insights of each hallmark and the linkage to each other.
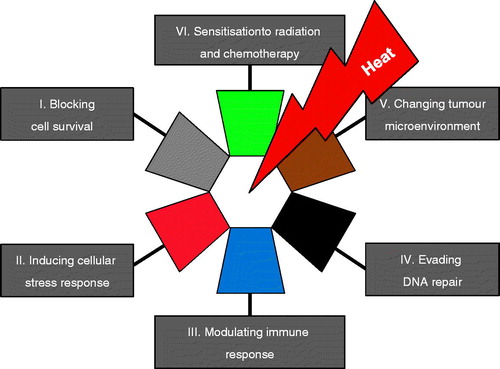
In this report, without going into details, a short description of the hallmarks will be given to illustrate the most distinct features of hyperthermia, although they are in many ways part of an interrelated continuum. The first hallmark ‘blocking cell survival’ is primarily based on the unique capability of heat to kill cells in a time–temperature dose relationship and to induce thermotolerance in the fraction of surviving cells at the same time. The hallmark also refers to promoting cellular death pathways such as apoptosis or necrosis dependent on the magnitude of the applied heat dose. The capability ‘inducing cellular stress response’ by hyperthermia refers to the intracellular expression of inducible heat shock proteins that function as molecular chaperones or proteases within the cell. Consequently, heat shock proteins assist in the recovery from stress either by repairing damaged proteins (protein refolding) or by degrading them. The events of heat-induced cellular stress and cell death are linked such that molecular chaperones induced in response to stress appear to function at key regulatory points in the control of apoptosis. The hallmark ‘modulating immune response’ comprises the expression and up-regulation of surface molecules on heated cells, the extracellular release of exosomes or of heat shock proteins carrying potential tumour antigens, the cross-presentation of chaperoned tumour antigens to T-cells by antigen presenting cells, the thermal activation of immunocompetent cells, and the heat effects on lymphocytes trafficking into the tumour. The hallmark capability of hyperthermia ‘changing tumour microenvironment’ reflects the heat-dependent physiological changes, the effects on tumour vasculature, the unbalanced production of reactive oxygen species, and metabolic alterations. The other two hallmarks ‘sensitisation to radiation and chemotherapy’ and ‘evading DNA repair’ are addressed in this manuscript and are exemplified by the hyperthermia interaction with trabectedin. These unique features are interactive and in some way synergistic if induced at the time of treatment. The understanding of the precise mechanisms of their interaction is far from being complete and it is beyond the scope of this review to explain this issue in a comprehensive way and should be addressed elsewhere. However, several reviews on each of these individual hallmarks of hyperthermia are available [Citation12–17]. Dewhirst et al. [Citation18] focussed on altered metabolism, evasion of immune destruction, and changes in the cellular microenvironment to present emerging fields of study in hyperthermia biology over the next decade and beyond. We address the concept of synthetic lethality and focus on the hallmark capability to inhibit DNA repair in the context of adding regional hyperthermia as a targeted therapy combined with the anticancer agent trabectedin. However, we should remember that the effect of hyperthermia is pleiotropic, and it will be also linked to other capabilities.
Lessons learned from the success of targeted therapy in medical oncology
Efforts over the past two decades to characterise the hallmarks of cancer have led to a better understanding of biological capabilities acquired during the multistep development of human tumours. The original hallmarks, as proposed by Hanahan and Weinberg [Citation19], include the sustaining of proliferative signalling, evading growth suppressors, resisting cell death, enabling replicative immortality, inducing angiogenesis, and activating invasion and metastases. Normal cells evolve progressively to a neoplastic state and acquire a succession of these hallmark capabilities that enable the cells to become malignant. Revisiting these hallmarks 11 years later resulted in the addition of two emerging capabilities, reprogramming of energy metabolism and evading immune destruction [Citation20]. The notion that tumours are more than insular masses of proliferating cells was extended during this period of time. Tumours are composed of multiple distinct cells types, which form the tumour-associated stroma. Neoplastic cells and the stroma cells around them interact via microenvironmental signalling. The primary tumour microenvironment changes as tumour cells invade normal tissue and seed and colonise distant tissues with the formation of a metastatic tumour environment.
This knowledge and the identification of the key drivers in the pathway of malignant cells rapidly expanded the era of targeted therapy in medical oncology. Significant improvements in the treatment of some cancer entities was achieved clinically by the use of target-designed new compounds. The notion of some of the key factors in their success may be informative and provide guidelines for the development of therapeutic combinations, including hyperthermia, as targeted therapy.
First example of a target-designed compound is trastuzumab, a monoclonal antibody directed against the human epidermal growth factor receptor 2 (HER2/neu receptor) that is currently used in combination with chemotherapy for the treatment of breast cancer [Citation21]. Retrospectively, robust early data on the HER2/neu pathway in tumorigenesis and the amplification of the HER2/neu oncogene in tumour cell proliferation prompted the clinical observation that the overall survival of breast cancer patients inversely correlated with the amplification of the HER2/neu receptor in these women. Therefore, preclinical findings in vitro and retrospective analyses in vivo that addressed the relevance of the specific target were responsible for the success of the trastuzumab development programme.
Second, imatinib is the quintessential example of a targeted anticancer agent that suppresses the activation of oncogenic tyrosine kinases [Citation22]. Imatinib directly induces apoptosis of malignant cells via inhibition of the tyrosine kinase encoded by the BCR-ABL oncogene or the c-kit and PDGFR oncogenes, which constitutes the main action of this drug [Citation23,Citation24]. This direct anti-proliferative effect is coupled with an additional ‘off-target’ effect that induces dendritic cell (DC)-mediated natural killer (NK) cell activation [Citation25]. Notably, the therapeutic effect of imatinib in the context of gastrointestinal stromal tumour (GIST) crucially depends on the reactivation of an anticancer immune response [Citation26,Citation27].
Taken together, the success of these therapeutic approaches is primarily based on the structural elements listed below as proposed guidelines on the development of therapeutic combinations targeting major signalling pathways [Citation28]:
Robust scientific hypothesis.
Molecular defined target or pathway.
Target drugged or treatable by the intervention.
Supportive preclinical data from contextually appropriate preclinical model systems.
Clinical evidence for the target relevance in patients or subgroup of patients with defined disease.
Consideration of unexpected ‘off-target’ effects.
Consideration of potential immune-modulating effects on the microenvironment.
Hyperthermia and DNA repair
Enhancement of drug efficacy by heat is well known and the pleiotropic effect of heat in the mechanisms of interaction with specific chemotherapeutic agents at clinical relevant temperatures in vitro and in vivo have been reviewed [Citation29]. Previous studies describing the effects of hyperthermia on DNA repair in the context of radiosensitisation established that sensitisation was observed in cells deficient in repair of DNA double-strand breaks (DSB) through the homologous recombination (HRR) or the non-homologous end-joining (NHEJ) repair pathways. These results suggested that heat was acting more up-stream, e.g. at the single-strand re-ligation step of the base excision repair (BER) pathway [Citation30], because unresolved single-strand breaks in the S-phase (the most thermosensitive phase of the cell cycle) led to the formation of replication- associated DSBs. Experimental approaches using genetic mutants of NHEJ or of HRR-deficient rodent or chicken cells were performed on the hypothesis that if hyperthermia radiosensitised these mutants, then the underlying defective DNA repair pathway would not likely be targeted by heat. Notably, the results of these experiments in different laboratories were inconsistent and contradictory, which suggests that the outcomes of clonogenic survival assays for radiosensitisation are highly dependent on cell type, genotype and temperature applied, and should be interpreted with caution [Citation31]. There is solid evidence that one target of hyperthermia is the HRR pathway via the heat-induced degradation of the breast cancer susceptibility gene 2 (BRCA2) protein [Citation32]. This seminal report opened a new window for the potential use of hyperthermia in the sensitisation of tumours to biologically targeted therapies [Citation33]. BRCA2 is the major player in HRR because it mediates the localisation and functional activation of the key protein in HRR, RAD51, to sites of function in human cells in response to DSBs. BRCA2 binds to the recombinase RAD51 with its carboxyl terminus and controls its DNA binding ability and localisation at the DSBs. RAD51 is recruited to the site of DNA damage and forms helical nucleoprotein filaments that initiate DSB repair via HRR [Citation34]. BRCA2 is downstream of BRCA1 and PALB2 in its engagement at sites of damage [Citation35] but up-stream of RAD51. Earlier data on the heat shock response of human cancer cells to heat (42 °C) reportedly caused a rapid disappearance of the BRCA1 protein [Citation36], but the time scale for BRCA2 was an hour rather than minutes. These results suggest that hyperthermia inhibits the HRR pathway over a sustained period of time after the application of heat because of inhibition at different mechanistic steps. These findings provide a strong rationale for the development of novel anticancer treatment strategies that combine hyperthermia-induced HRR deficiency in tumours with the systemic administration of drugs to sensitise cancer cells locally to the DNA damaging agent [Citation31].
Trabectedin – a cytotoxic and targeting agent
Trabectedin (Ekteinascedin-743) was originally isolated from the Caribbean marine tunicate, Ekteinascidia turbinata, but is now manufactured via a synthetic process. Trabectedin was one of the first agents that was studied specifically for sarcomas. Trabectedin has been marketed as Yondelis (PharmaMar, Madrid, Spain), and is approved in Europe and more recently also by the Food and Drug Administration in the USA for the treatment of advanced soft tissue sarcoma after failure of standard therapy with anthracyclines and ifosfamide or in patients who are unsuited to receive these agents [Citation37]. Its unique mechanism of action prompted claims that trabectedin exhibits the action of a cytotoxic agent and a targeting drug. Two of its three fused tetrahydroisoquinoline rings provide the framework for covalent binding to the minor groove of the DNA double helix, and the third ring allows interactions with adjacent nuclear proteins ().
Figure 3. Trabectedin isolated from the Caribbean marine tunicate - an anticancer agent. The unique mechanisms of action include the initial formation of DNA–trabectedin–XPG ternary complexes. The endonuclease ERRC5 (xeroderma pigmentosum group G; XPG human; Rad13 yeast) is a key-protein of the DNA nucleotide excision repair (NER) pathway. Photos courtesy of PharmaMar.
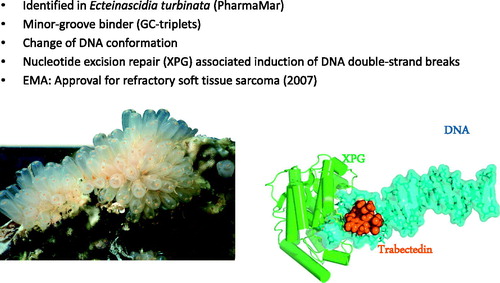
These chemical interactions within the tumour cell trigger a cascade of events that interfere with DNA repair pathways and ultimately lead to the generation of DSBs, G2/M cell cycle arrest, and apoptosis [Citation38]. The observed DSBs occur after the formation of DNA-trabectedin-ERCC5 ternary complexes. The endonuclease ERCC5 (xeroderma pigmentosum group G; XPG) is a key protein of the DNA nucleotide excision repair (NER) pathway. The in vitro efficacy of trabectedin depends on an intact NER system but it is remarkably enhanced in tumour cells with deficiency in the DNA homologous recombination repair (HRR) pathway which is the main repair pathway for DSBs in late S and G2 phases of the cell cycle [Citation39]. The expression of genes and single nucleotide polymorphisms (SNPs) linked to the NER and HRR pathways are predictive for tumour response rate and survival outcome in patients treated with trabectedin [Citation40,Citation41]. Retrospective analysis revealed that soft tissue sarcoma patients treated with trabectedin could be segregated into a high NER/low BRCA1 subpopulation that exhibited better survival outcome compared to the subgroup that expressed an inverse profile in their primary tumour biopsies [Citation42]. Trabectedin also has anti-inflammatory and immunomodulatory ‘off-target’ properties. It selectively targets mononuclear cells, including monocytes and tumour-associated macrophages, and down-regulates the production of pro-inflammatory cytokines and chemokines by tumour and stroma cells. These effects suggest that changes induced within the tumour microenvironment also play a role in trabectedin antitumour activity [Citation43–45].
Hyperthermia targeting the homologous recombination repair pathway combined with trabectedin in human sarcoma
Overall, the results and conclusions outlined above suggest that regional hyperthermia may enhance the anti-proliferative effects of trabectedin at clinically relevant temperatures (41.8–43.0 C) in human sarcomas via the underlying mechanism of targeted chemosensitisation based on the principle of induced synthetic lethality [Citation46]. The targeting of the heating field to the tumour area should transiently impair the HRR pathway and increase the amount of DNA damage due to the genotoxic agent ().
Figure 4. Regional hyperthermia targeting the homologous recombination repair (HRR) pathway. In combination with trabectedin, the formation of the DNA–trabectedin–XPG complex triggers a cascade of events, which ultimately lead to the generation of DSBs. The HRR pathway is inhibited over a sustained period of time after the application of regional hyperthermia due to an immediate degradation of BRCA2 and – later on – of BRCA1 resulting in an increase in unresolved DSBs and cell death.
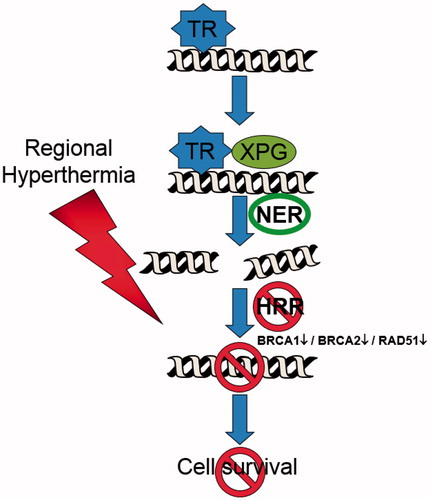
We first investigated the postulated enhancement of trabectedin effectiveness in different human sarcoma cell lines and characterised the cellular events and molecular mechanisms related to heat-induced effects for proof of principle in vitro. Preliminary results demonstrated that the heat combination increased the accumulation of γH2AX foci as a key marker of DSBs. Heat significantly decreased the expression of BRCA2 protein, and consequently the recruitment of downstream RAD51 to the γH2AX positive repair foci was almost abolished. These results indicate the relevant impairment of HRR by heat. The enhancement of trabectedin effectiveness was augmented in BRCA2-proficient cells and alleviated in BRCA2 knock-out or si-RNA transfected BRCA2 knockdown cells [Citation47].
These preclinical findings and previous clinical observations of trabectedin in soft tissue sarcomas were used to design a clinical randomised study entitled ‘Trabectedin combined with regional hyperthermia as second-line treatment for adult patients with soft tissue sarcomas’ (Hyper-TET – hyperthermia enhanced trabectedin, clinicaltrials.gov: NCT02359474). The hyperthermia-enhanced antitumour efficacy of trabectedin is required only within the heat-targeted region of the tumour and the tumour-bearing tissue. Therefore heat has to be applied regionally.
The first clinical evidence in pilot patients with locally advanced soft tissue sarcomas treated with trabectedin (1.5 mg/m2, 24-h infusion) and regional hyperthermia (41–43 °C, 60 min treatment time, at the end of trabectedin infusion) demonstrated feasibility, and no uncommon side effects or increased toxicity was observed [Citation48]. In the ongoing HyperTET study, all patients receive standard trabectedin treatment, but patients are randomised for the addition of regional hyperthermia. The primary end point of the study is progression-free survival. Eligibility criteria of the study protocol allow the inclusion of patients with oligometastases outside of the localised tumour area to be heated in the combined treatment group. Therefore the design of the clinical protocol allows us to determine whether the regional application of hyperthermia may cause off-target effects to metastatic sites by comparison of both treatment groups. We are also planning to analyse further the numbers of nuclear γH2AX foci in peripheral blood mononuclear cells of patients after trabectedin or trabectedin plus regional hyperthermia to define a potential surrogate biomarker.
Concluding remarks
We have introduced six biological and clinical capabilities defined as ‘hallmarks of hyperthermia’ to emphasise the pleiotropic effect induced by heat in malignant cells and tissues. We recommend the considerations of these hallmarks in the design of clinical trials involving regional hyperthermia as targeted therapy. Here, we focus on the hallmark capability of hyperthermia to evade DNA repair. In the HyperTET study design, regional hyperthermia targets the heating field to the tumour and the homologous recombination repair pathway at the molecular level to block the repair of DSBs induced by the anticancer agent trabectedin. The on-demand induction of BRCAness by hyperthermia may open a new therapeutic window in the future study design for patients with BRCA-proficient tumours to become sensitised to platinum-based chemotherapy or poly-ADP ribose polymerase (PARP)-inhibitors (e.g. triple negative breast cancer, ovarian cancer).
Declaration of interest
The authors report no conflicts of interest. The authors alone are responsible for the content and writing of the paper.
References
- Tilly W, Wust P, Rau B, Harder C, Gellermann J, Schlag P, et al. Temperature data and specific absorption rates in pelvic tumours: Predictive factors and correlations. Int J Hyperthermia 2001;17:172–88
- Song CW, Park JH, Lee CK, Griffin R. Implications of increased tumor blood flow and oxygenation caused by mild temperature hyperthermia in tumor treatment. Int J Hyperthermia 2005;21:761–7
- Overgaard J, Gonzalez Gonzalez D, Hulshof MC, Arangeli G, Dahl O, Mella O, et al. Randomised trial of hyperthermia as adjuvant to radiotherapy for recurrent or metastatic malignant melanoma. European Society for Hyperthermic Oncology. Lancet 1995;345:540–3
- van der Zee, Gonzalez Gonzales D, van Rhoon GC, van Dijk JD, van Putten WL, Hart AA. Comparison of radiotherapy alone with radiotherapy plus hyperthermia in locally advanced pelvic tumours: A prospective, randomised, multicentre trial. Dutch Deep Hyperthermia Group. Lancet 2000;344(9210):1119–25
- Vernon CC, Hand JW, Field SB, Machin D, Whaley JB, van der Zee J, et al. Radiotherapy with or without hyperthermia in the treatment of superficial localized breast cancer: Results from five randomized controlled trials. International Collaborative Hyperthermia Group. Int J Radiat Oncol Biol Phys 1996;35:731–44
- Jones EL, Oleson JR, Prosnitz LR, Samulski TV, Vujaskovic Z, Yu D, et al. Randomized trial of hyperthermia and radiation for superficial tumors. J Clin Oncol 2005;23:3079–85
- Issels RD, Lindner LH, Verweij J, Wust P, Reichardt P, Schem BC, et al. Neo-adjuvant chemotherapy alone or with regional hyperthermia for localised high-risk soft-tissue sarcoma: A randomised phase 3 multicentre study. Lancet Oncol 2010;11:561–70
- Bruggmoser G, Bauchowitz S, Canters R, Crezee H, Ehmann M, Gellermann J, et al. Quality assurance for clinical studies in regional deep hyperthermia. Strahlenther Onkol 2011;187:605–10
- Hildebrandt B, Wust P, Ahlers O, Dieing A, Sreenivasa G, Kerner T, et al. The cellular and molecular basis of hyperthermia. Crit Rev Oncol Hematol 2002;43:33–56
- Westra A, Dewey WC. Variation in sensitivity to heat shock during the cell-cycle of Chinese hamster cells in vitro. Int Radiat Biol Relat Stud Phys Chem Med 1971;19:467–77
- Dewey WC, Thrall DE, Gillette EL. Hyperthermia and radiation – a selective thermal effect on chronically hypoxic tumor cells in vivo. Int J Radiat Oncol Biol Phys 1977;2:99–103
- Dewey WC. Arrhenius relationships from the molecule and cell to the clinic. Int J Hyperthermia 1994;10:457–83
- Thorary-Brown S, Fiering S. Local tumour hyperthermia as immunotherapy for metastatic cancer. Int J Hyperthermia 2014;30:531–9
- Westerheide SD, Morimoto RI. Heat shock response modulators as therapeutic tools for diseases of protein conformation. J Biol Chem 2005;280:33097–100
- Jolly C, Morimoto RI. Role of the heat shock response and molecular chaperones in oncogenesis and cell death. J Natl Cancer Inst 2000;92:1564–72
- Slimen IB, Najar T, Ghram A, Dabbebi A, Mrad MB Abdrabbah M. Reactive oxygen species, heat stress and oxidative-induced mitochondrial damage. A review. Int J Hyperthermia 2014;30:513–23
- Wust P, Hildebrandt B, Sreenivasa G, Rau B, Gellermann J, Riess H, et al. Hyperthermia in combined treatment of cancer. Lancet Oncol 2002;3:487–97
- Dewhirst MW, Lee CT, Ashcraft KA. The future of biology in driving the field of hyperthermia. Int J Hyperthermia 2015. (in press)
- Hanahan D, Weinberg RA. The hallmarks of cancer. Cell 2000;100:57–70
- Hanahan D, Weinberg RA. Hallmarks of cancer: The next generation. Cell 2011;144:646–74
- Slamon DJ, Leyland-Jones B, Shak S, Fuchs H, Paton V, Bajamonde A, et al. Use of chemotherapy plus a monoclonal antibody against HER2 for mestastatic breast cancer the overexpresses HER2. N Engl J Med 2001;344:783–92
- Heinrich MC, Blanke CD, Druker BJ, Corless CL. Inhibition of KIT tyrosine kinase activity: A novel molecular approach to the treatment of KIT-positive malignancies. J Clin Oncol 2002;20:1692–703
- Apperley JF, Gardembas M, Melo JV, Russell-Jones R, Bain BJ, Baxter EJ, et al. Response to imatinib mesylate in patients with chronic myeloproliferative diseases with rearrangements of the platelet-derived growth factor receptor beta. N Eng J Med 2002;347:481–7
- Heinrich MC, Corless CL, Duensing A, McGreevey L, Chen CJ, Joseph N, et al. PDGFRA activating mutations in gastrointestinal stroma tumors. Science 2003;299:708–10
- Borg C, Terme M, Taieb J, Ménard C, Flament C, Robert C, Maruyama K, et al. Novel mode of action of c-kit tyrosine kinase inhibitors leading to NK cell-dependent antitumor effects. J Clin Invest 2004;114:379–88
- Zitvogel L, Kroemer G. Anticancer effects of imatinib via immunostimulation. Nat Med 2011;17:1050–1
- Rusakiewicz S, Semeraro M, Sarabi M, Desbois M, Locher C, Mendez R, et al. Immune infiltrates are prognostic factors in localized gastrointestinal stromal tumors. Cancer Res 2013;73:3499–510
- Yap TA, Omlin A, de Bono JS. Development of therapeutic combinations targeting major cancer signaling pathways. J Clin Oncol 2013;31:1592–602
- Issels RD. Hyperthermia adds to chemotherapy. Eur J Cancer 2008;44:2546–54
- Kampinga HH, Dikomey E. Hyperthermic radiosensitization mode of action and clinical relevance. Int J Radiat Biol 2001;77:399–408
- Eppink B, Krawczyk PM, Stap J, Kanaar R. Hyperthermia-induced DNA repair deficiency suggests novel therapeutic anti-cancer strategies. Int J Hyperthermia 2012;28:509–17
- Krawczyk PM, Eppink B, Essers J, Stap J, Rodermond H, Odijk H, et al. Mild hyperthermia inhibits homologous recombination, induces BRACA2 degradation, and sensitizes cancer cells to poly (ADP-ribose) polymerase-1 inhibition. Proc Natl Acad Sci USA 2011;108:9851–6
- Powell SN, Kachnic LA. Homologous recombination is heating up and ready for therapy. Proc Natl Acad Sci USA 2011;108:9731–2
- Park JY, Singh TR, Nassar N, Zhang F, Freund M, Hanenberg H, et al. Breast cancer-associated missense mutants of the PALB2 WD40 domain, which directly binds RAD51C, RAD51 and BRCA2, disrupt DNA repair. Oncogene 2014;2:33:4803–12
- Xia B1, Sheng Q, Nakanishi K, Ohashi A, Wu J, Christ N, et al. Control of BRCA2 cellular and clinical functions by a nuclear partner, PALB2. Mol Cell 2006;23:719–29
- Ma YX, Fan S, Xiong J, Yuan R, Meng Q, Gao M, et al. Role of BRCA1 in heat shock response. Oncogene 2003;22:10–27
- Blay JY, Blomquist C, Bonvalet S, Boukovinas I, Casali PG, De Alava E, et al. Soft tissue and visceral sarcomas: ESMO clinical practice guidelines for diagnosis, treatment and follow-up. Annal Oncol 2012;23(Suppl 7):vii92–9
- D’Incalci M, Galmarini CM. A review of trabectedin (ET-743): A unique mechanism of action. Mol Cancer Ther 2010;9:2157–63
- Herrero AB, Martin-Castellanos C, Marco E, Gago F, Moreno S. Cross-talk between nucleotide excision and homologous recombination DNA repair pathways in the mechanism of action of antitumor trabectedin. Cancer Res 2006;66:8155–62
- Italiano A, Laurand A, Laroch A, Casali P, Sanfilippo R, Le Cesne A, et al. ERCC5/XPG, ERCC1, and BRCA1 gene status and clinical benefit of trabectedin in patients with soft tissue sarcoma. Cancer 2011;117:3445–56
- Laroche-Clary A, Chaire V, Le Morvan V, Neuville A, Bertucci F, Salas S, et al. BRCA1 haplotype and clinical benefit of trabectedin in soft tissue sarcoma patients. Br J Cancer 2015;112:688–92
- Schoeffski P, Taron M, Jimeno J, Grosso F, Sanfilippo R, Casali PG, et al. Predictive impact of DNA repair functionality on clinical outcome of advanced sarcoma patients treated with trabectedin: A retrospective multicentric study. Eur J Cancer 2011;47:1006–12
- Allavena P, Signorelli M, Chieppa M, Erba E, Bianchi G, Marchesi F, et al. Anti-inflammatory properties of the novel antitumor agent Yondelis (trabectedin): Inhibition of macrophage differentiation and cytokine production. Cancer Res 2005;65:2964–71
- Germano G, Frapolli R, Simone M, Tavecchio M, Erba E, Pesce S, et al. Antitumor and anti-inflammatory effects of trabectedin on human myxoid liposarcoma cells. Cancer Res 2010;70:2235–44
- Germano G, Frapolli R, Belgiovine C, Anselmo A, Pesce S, Liguori M, et al. Role of macrophage targeting in the antitumor activity of trabectedin. Cancer Cell 2013;23:249–62
- Kaelin WG. The concept of synthetic lethality in the context of anticancer therapy. Nat Rev Cancer 2005;5:689–98
- Kampmann E, Harnicek D, Cardoso Martins AS, Eppink B, Gallmeier E, Lindner L, et al. Heat-shock (H-S) and trabectedin efficacy in human soft-tissue sarcoma (STS) cells in vitro. J Clin Oncol 2013;31. (suppl; abstr e13540)
- Kampmann E, Harnicek D, Cardoso Martins SA, Tanovic A, Knösel T, Laubender R, et al. Trabectedin with regional hyperthermia. Experiences in patients with high-risk liposarcoma. Connective Tissue Oncology Society, 18th Annual Meeting 2013 (PO 355), New York