Abstract
Purpose: Expression profile alterations of nine breast cancer (BC)-associated secreted microRNAs (miRs) were determined under microenvironmental alterations occurring in tumour progression, metastasis or specific oncological treatment modalities. Thereto, the potential influence of the exogenic stimuli hypoxia, acidosis and hyperthermia was investigated in vitro.
Material and methods: Four established BC cell lines were applied as in vitro BC model systems. Quantitative analyses of secreted microRNA specimens were performed by RNA isolation from cell culture supernatant and subsequent real-time PCR in cells under physiological versus hypoxic, acidic or hyperthermia conditions.
Results: The in vitro application of exogenic stimuli hypoxia, extracellular acidosis and hyperthermia caused heterogeneous expression alterations for the investigated secreted miRNA phenotypes. The majority of relevant exogenic stimuli-dependent microRNA expression alterations were restricted to single events displaying distinct cell type and stimulus dependent correlations only. Most remarkably, hyperthermia triggered a uniform significant down-regulatory effect on the expression levels of the three secreted microRNAs miR-10b, miR-15b and miR-139, respectively. The marked decrease in miR-10b and miR-15b levels was detectable in all four, while miR-139 was found significantly reduced in three out of four BC cell lines.
Conclusion: Hyperthermia-dependent down-regulatory influence on three distinct BC-related microRNAs in vitro generates translational aspects for clinical BC treatment, since the identified microRNAs miR-10b, miR-15b and miR-139 are known to have oncogenic as well as tumour suppressor functions in BC. However, an evaluation regarding the potential impact of microRNA-related hyperthermia-dependent alterations for innovative BC treatment approaches demands further analysis including in vivo data.
Introduction
MicroRNAs (miRNAs, miRs) are short, non-coding RNA molecules exerting specific functions in post-transcriptional gene regulation in physiological, but also in pathological cellular processes, e.g. carcinogenesis [Citation1]. Multiple studies identified miRNAs as important regulators in breast cancer (BC) tumourigenesis and progression due to their ability to participate in the regulation of oncogenic signalling pathways [Citation2,Citation3]. MiRNA molecules are not restricted to intracellular localisation but are frequently subjected to secretion and subsequent extracellular circulation [Citation4]. Cellular pathways of miRNA export include active secretion by microvesicles, exosomes, high-density-lipoproteins, apoptotic bodies and protein complexes, but also passive leakage from cells [Citation5–7]. MiRNA release via protein complexes was found predominately mediated by Argonaute complexes [Citation8], as regular components of the RNA silencing machinery, as well as by nucleophosmin 1 that acts as protector from RNA degradation and mediates miRNA packaging and extracellular export [Citation5,Citation9]. Recent investigations could even reveal mechanisms of exosomal sorting and trafficking of specific miRNAs with supposed signalling function in cell–cell communication processes [Citation10]. A growing number of studies revealed that BC can frequently be characterised by deregulated expression levels of specific miRNA types, allowing early detection of disease or stage-dependent classification of BC progression [Citation11,Citation12]. Thus, in addition to other secreted tumour-derived nucleic acids (e.g. cell-free DNA), circulating miRNA expression levels in blood serum and plasma of BC patients bear a high potential to act as promising non-invasive innovative biomarkers in BC diagnosis and therapy monitoring [Citation13–15]. Alterations in miRNA expression pattern were found also in cells exposed to stressors such as extracellular acidosis, hypoxia, or hyperthermia. However, very little data exists with regard to miRNA expression aberrations under the influence of hyperthermia [Citation16,Citation17]. Wilmink and colleagues could identify >100 different miRNA types with hyperthermia-driven changes in intracellular expression levels in dermal fibroblasts, at which most miRNAs could be identified as typical cellular stress response miRNA types [Citation17]. However miR-452, miR-382 and miR-378 were exclusively found expressed in response to hyperthermia, but not to other cellular stressors in this setting [Citation17]. According to current knowledge, miR-218 is the only circulating miRNA specimen that was found with altered expression under clinical hyperthermia treatment conditions in cancer therapy [Citation5,Citation18].
The heat-induced activation of heat shock proteins (HSP) [Citation19] was shown to be accompanied by HSP regulatory relevant miRNA expression profile alterations [Citation16,Citation20,Citation21].
The combination of hyperthermia and radiotherapy represents a treatment modality in locally recurrent inoperable BC patients in clinical routine [Citation22–27]. A meta-analysis revealed improved local response rates for combinatory hyperthermia/radiotherapy treatment compared to radiotherapy alone (59% versus 41%), suggesting a synergistic effect of both therapy options, without improving overall survival rates however [Citation28]. Another study showed for high-risk BC patients with histopathological R1 situation after mastectomy or with resected early local recurrence with close margins or R1-resection a three-year local control rate of 81% [Citation29]. However, another report revealed a correlation with the tumour type (bulky nodes/nodular tumours or diffuse/multiple tumours) with lower 6-month control rate ranging from 38–67% [Citation30]. Linthorst and colleagues conducted a study focusing on combination therapy of re-irradiation with hyperthermia in recurrent breast cancer patients (N = 248, median follow-up period: 32 months) resulting in a complete response rate of 70% with local control after 1, 3, and 5 years with 53%, 40% and 39%, and overall survival of 66%, 32%, and 18%, respectively; 10% of recurrent breast cancer patients in this study survived within the 10 year period under this treatment regime [Citation31]. The efficacy and safety of hyperthermia treatment in combination with radiation was shown for BC reconstruction patients [Citation32].
Based on a targeted pre-selection process focusing on BC-associated circulating miRNA specimens with diagnostic and therapeutic relevance, a set of different miRNA types was pre-selected. By initial screening expression analysis, a stable, detectable panel of nine secreted miRNA types (miR-let7-a, miR-10a, miR-10b, miR-15b, miR-19b, miR-100, miR-139, miR-205, miR-298) was verified for the applied four BC cell lines in this study (see ‘Materials and methods’ for details).
Let-7-a characterises as one of the most important miRNA types in tumourigenesis and malignant progression [Citation33]. Since oestrogen receptor alpha (ORα) mRNA is a target of let-7a, this miRNA exhibits an inhibitory function of ORα signalling in cell proliferation, as observed in let-7a overexpressing MCF-7 BC cells [Citation34]. Contrariwise, let-7a acts as a tumour suppressor by inhibiting ORα-mediated cellular malignant growth in ORα-positive BC stem cells [Citation35]. Low expression levels of let-7a are likely to induce chemotherapy resistance in BC [Citation36]. Decreased levels of circulating let-7a were identified in cancer patients post-operatively, to levels comparable with control subjects, following curative tumour resection [Citation37]. Among other miRNA types, miR-10a was found down-regulated in tumours from patients with early recurrence, thus defining a high-risk group of patients with shorter relapse-free survival [Citation38]. Higher expression levels of miR-10a in oRα-positive, post-menopausal patients undergoing tamoxifen treatment correlate with increased relapse-free periods, suggesting miR-10a as an independent predictor for tumour relapse in this clinical setting [Citation39]. Elevated expression levels of miR-10b were identified as a major driver of BC metastasis in vitro and in vivo [Citation3,Citation40]. Serum expression levels of circulating miR-10b were found specifically altered in BC patients compared to healthy controls [Citation41]. MiR-10b may also be a useful biomarker for the identification of bone metastatic BC [Citation12]. The expression levels of circulating miR-15b were found dysregulated in cancers [Citation42]. BC of aggressive basal subtype characterise by miR-15b overexpression that correlates with poor prognosis [Citation43]. Tumour suppressor gene p53 is a direct and critical target of and is inhibited by miR-19b. Thus, miR-19b overexpression triggers increased rates of cell cycle, cell migration and invasion, and apoptosis repression in vitro, as well as tumour growth and metastasis in vivo [Citation44]. MiR-19b also targets and down-regulates ORα [Citation45]. Most interestingly, secreted miR-100 was identified as a highly suspect mediator of drug-resistance spread via cell–cell-communications between BC cells partly attributed to the intercellular transfer of specific miRNAs [Citation46]. MiR-100 was also described as a context-dependent master regulator of the IGF/mTOR pathway and as a potential therapeutic target [Citation47]. MiR-139 exhibits crucial impact in the pathogenesis of primary BC [Citation48]. BC cell motility and invasion were shown to be significantly regulated by miR-139, implying a potential application as a prognostic marker for aggressive BC types [Citation49]. Several studies demonstrated the diagnostic value of circulating miR-205 levels in BC patient serum and point out its importance as a non-invasive biomarker in BC screening. Significantly reduced serum levels of miR-205 were found in BC patients compared to healthy controls [Citation50,Citation51]. MiR-298 bears a diagnostic and therapeutic potential for predicting doxorubicin chemo-resistance in human BC. Bao and colleagues [Citation52] identified reduced levels of miR-298 in doxorubicin-resistant BC cells, thus decreasing the inhibitory effect on chemoresistance mediating P-glycoprotein. summarises current data on the tumour-biological relevance of the miRNA types investigated in this study.
Table 1. Tumor-biological relevance of pre-selected miRNA types.
Our study targeted the quantitative expression analysis of nine specific miRNA types secreted by BC cells in vitro in response to exogenic stimuli. In addition to hypoxia and extracellular acidosis, as typical epiphenomena of solid tumours, the effect of hyperthermia on miRNA expression and secretion levels was investigated. The introduction of circulating miRNAs as non-invasive biomarkers for BC diagnosis and therapy monitoring is current subject of several studies. In particular, the clinical implementation of circulating miRNA levels as indicators for treatment response in adjuvant or neoadjuvant chemo/radiotherapy with or without accompanying hyperthermia offers auspicious advantages to improve and adapt tailored therapy approaches at an early stage and to better understand and conquer chemoresistance [Citation53,Citation54]. This in vitro model could be used as an initial step for the analysis of therapy-driven alterations in the expression profiles of circulating microRNAs in BC.
Materials and methods
Cell culture conditions and treatments
Established BC lines MCF-7 (1), BT474 (2), SK-BR-3 (2) and MDA-MB-231 (2) () were incubated in a humidified atmosphere at 37 °C and 5% CO2 in 1) RPMI 1640, or 2) DMEM/F12 medium supplemented with 10% newborn calf serum (Gibco™, Thermo Fisher Scientific, Karlsruhe, Germany), 1% HEPES buffer (Gibco) and 100 U/mL penicillin/streptomycin (Sigma-Aldrich®, Taufkirchen, Germany). For the induction of the stress conditions acidosis, hypoxia and hyperthermia, the cells were subcultured in 25 cm2 cell culture flasks and were grown overnight. Acidosis was induced by the addition of 2.2 μL of 80% lactic acid/mL medium. The cells were incubated at 37 °C overnight at acidic conditions. For hypoxia exposure, the cells were incubated in a hypoxic chamber (<1% CO2) for 18 h overnight. For the induction of hyperthermia, the cells were incubated at 42 °C for 2 h followed by a rescue incubation phase at 37 °C for 4 h. The hyperthermia treatment regime was designed with regard to clinically relevant application protocols and transferred to a proven in vitro translational approach, reflecting well-established knowledge [Citation17,Citation74–83]. Cells incubated under standard conditions in parallel served as controls. Following treatment, 2 mL of culture medium (supernatant) were harvested and immediately processed. Experiments were performed in triplicate.
Table 2. Molecular classification of investigated breast cancer cell lines [Citation40].
MicroRNA isolation
MiRNAs from tissue culture supernatant were isolated by using the “Urine microRNA Purification Kit” (Norgen Biotek Corp., Thorold, Canada) according to the manufacturer’s instructions. Isolated RNA was quantitatively determined using the NanoDrop ND1000 (PEQLAB, Erlangen, Germany). RNA samples were stored at −20 °C until further processing.
Reverse transcription and quantitative PCR
50 ng of isolated RNA per sample were used for reverse transcription (RT). The RT reaction mix contained 5 μL RT-buffer (5×), 1 μL stem-loop primer (APARA BIOSCIENCE GmbH, Denzlingen, Germany), 1 μL dNTPs (desoxy-nucleoside triphosphates, EURx®, Gdansk, Poland), 0.25 μL Maxima reverse transcriptase (Thermo), 0.25 μL SUPERase In RNase inhibitor (Thermo Fisher), 10 μL of the RNA sample diluted in nuclease-free water (Qiagen, Hilden, Germany). The reaction was carried out on a thermal cycler (Eppendorf, Hamburg, Germany) at 16 °C for 30 min, 42 °C for 30 min and stopped by 85 °C for 10 min. Processed cDNA was stored at 4 °C.
Relative expression levels of specific microRNAs were assessed by quantitative PCR applying TaqMan assay in duplicate analysis. Per sample, 1 μL cDNA with a concentration of 100 pg/μL was mixed with 19 μL master mix, containing 2 μL buffer (10×), 1 μL dNTPs (EURx), 1 μL primer (Apara), 1 μL TaqMan Universal Probe Library Probe no. 21 (Roche®, Basel, Switzerland), 1 μL Perpetual Taq (EURx) and 13.9 μL nuclease-free water (Qiagen). Primer pairs consist of a universal probe reverse miRNA primer [Citation84,Citation85] and specific miRNA specimen sense primers: let-7a 5p S: 5′-CGGTGAGGTAGTAGGTTGTA-TAGTT-3′; miR-10a 5p S: 5′-GGTACCCTGTAGATCCGAATTT-3′; miR-10b 5p S: 5′-GGTACCCTGTAGAACCGAATTT-3′; miR-15b 5p S: 5′-GTAGCAGCACATCATGGTTTAC-3′; miR-19b 3p S: 5′-GTGTGCAAATCCATGCAAAACT-3′; miR-100 5p S: 5′-CCCGTAGATCCGAACTTGT-3′; miR-139 5p S: 5′-CTACAGTGCACGTGTCTCC-3′; miR-205 5p S: 5′-CTTCATTCCACCGGAGTCT-3′; miR-298 5p S: 5′-CAGAAGCAGGGAGGTTCT-3′. The quantitative PCR was performed on a Mastercycler ep Gradient S (Eppendorf) at 95 °C for 5 min, followed by 40 cycles at 95 °C for 10 s and 60 °C for 30 s. Data were analysed with Realplex software (Eppendorf). The relative expression of single microRNAs was determined via the ΔCt method based on reference value (geometric mean of housekeeping miRs: miR-26b and miR-1225, defined via BestKeeper software tool [Citation86]. Specific sense primers used for housekeeping genes were miR-26 5p S: 5′-GGCGGCGTTCAAGTAATTCAGG-3′, miR-1225 5p S: 5′- GGTGGGCGGGGGCAG-3′.
Pre-selection of miRNA specimen
Potential study-relevant miRNA types were determined by a combined comprehensive survey applying the PubMed interface for original research (http://www.ncbi.nlm.nih.gov/) and the miRTarBase platform (http://mirtarbase.mbc.nctu.edu.tw/) [Citation87]. This specific miRNA information registry was screened for the relevant specimen with regard to ‘breast neoplasm’, typical breast cancer target genes, and pathways as well as literature. Based on the summarised information on potential miRNA candidates, a methodological pre-screening of >20 miRNA types was performed with regard to the experimental in vivo setting focusing on secreted miRNA specimens in cell culture media. Consequently, the pre-set of miRNAs was filtered to identify the final set of miRNAs that were found reliably detectable in culture media. summarises the thereby determined miRNA specimen and provides information on miRNA functional implications based on MiRTarBase.
Table 3. Pre-selected miRNA specimen based on comprehensive survey (PubMed (http://www.ncbi.nlm.nih.gov/), miRTarBase platform (http://mirtarbase.mbc.nctu.edu.tw/) [Citation1]) and expression analysis in experimental setting. BC = verified functional impact in breast cancer; source: Chou et al. [Citation87].
Statistical analysis
Observed miRNA expression levels were graphically visualised in box plots, showing median, minimum and maximum value and quartiles in cell lines under specific treatment conditions. The influence of treatment-dependent alterations in miRNA expression levels of the different BC cell lines was investigated using a linear model with factors treatment and cell line.
Results
In the present study we investigated the effect of hypoxia, acidosis and hyperthermia on the expression levels of nine different BC-associated microRNA types (let-7a, miR-10a, -10b, -15b, -19b, -100, -139, -205, -298) that are actively secreted into the tissue culture supernatant by BC cells. The characteristics of the four BC cell lines investigated, BT-474, MCF-7, MDA-MB-231 and SK-BR-3, are summarised in .
All nine microRNAs examined were stably detectable in the supernatants of all four cell lines. Hypoxia and acidosis were induced to mimic typical microenvironmental alterations which are detectable as peritumoural epiphenomena of solid tumours during tumour growth, progression and metastasis [Citation88–91]. In contrast, hyperthermia served as the model for a therapeutic treatment option. In regard to each of the nine miRNA types investigated, a two-way analysis of variance was performed. Thus, potential regulatory effects triggered by hypoxia, acidosis or hyperthermia in each cell-line, but also relevant differences of potential interaction of these effects amongst the different cell lines, were examined. Thereto the expression levels of all BC-associated miRNA types were determined as mean ΔCt values of the distinct miRNA specimen normalised against the geometric mean of the two housekeepers miR-1225 and miR-26b, respectively.
Our analysis clearly identified a uniform down-regulatory effect on miR-10b and miR-15b driven by hyperthermia in all BC cell lines investigated. In detail, the expression levels of miR-10b were decreased by a mean value of 0.253 in BT-474 (CI 0.151–0.355, p = 0.000), 0.277 in MCF-7 (CI 0.175–0.379, p = 0.000), 0.268 in MDA-MB-231 (CI 0.166–0.370, p = 0.000) and 0.235 in SK-BR-3 (CI 0.133–0.337, p = 0.000) cells (, ). A comparable hyperthermia-dependent effect was also determined for miR-15b. The expression levels of miR-15b secreted in hyperthermia-conditioned cells were decreased by a mean value of 0.012, in BT-474 cells (CI 0.005–0.019, p = 0.002), 0.018, in MCF-7 cells (CI 0.011–0.025, p = 0.000), 0.012 in MDA-MB-231 (CI 0.005–0.019, p = 0.003) and 0.015 in SK-BR-3 cells (CI 0.008–0.022, p = 0.000, , ).
Figure 1. Box plot diagram of expression levels of secreted miR-10b in breast cancer cell lines under varying treatment conditions. Levels of secreted miR-10b expression were determined in MCF-7, BT-474, SK-BR-3 and MDA-MB-231 cells under control conditions, extracellular acidosis, hypoxia or hyperthermia. Box plots demonstrate median (thick black line), lower and upper quantile range (box lines), and standard deviation range (dashed lines bounded by horizontal lines). *Significant expression level alterations (p ≤ 0.05) of control conditions versus treatment options. Based on triplicate experiments, real-time quantitative PCR.
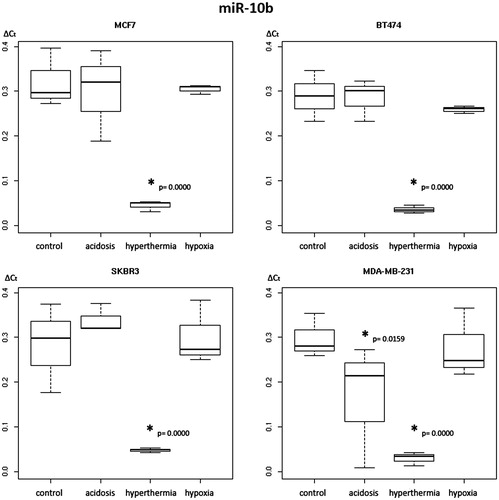
Figure 2. Box plot diagram of expression levels of secreted miR-15b in breast cancer cell lines under varying treatment conditions. Levels of secreted miR-15b expression were determined in MCF-7, BT-474, SK-BR-3 and MDA-MB-231 cells under control conditions, extracellular acidosis, hypoxia or hyperthermia. Box plots demonstrate median (thick black line), lower and upper quantile range (box lines), and standard deviation range (dashed lines bounded by horizontal lines). *Significant expression level alterations (p ≤ 0.05) of control conditions versus treatment options. Based on triplicate experiments, real-time quantitative PCR.
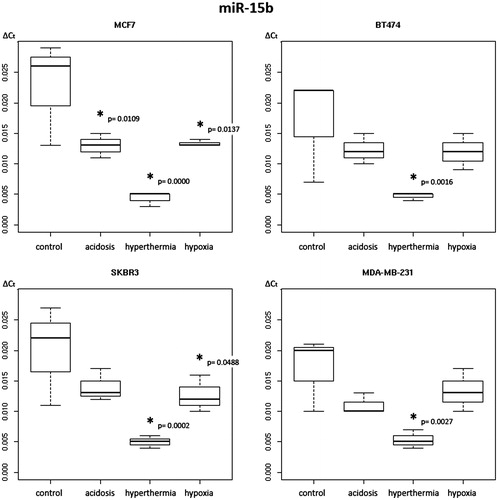
Table 4. Two-way analysis of variance for levels of secreted miR-10b under control, hyperthermic, acidotic and hypoxic conditions in four different breast cancer cell lines
Table 5. Two-way analysis of variance for levels of secreted miR-15b under control, hyperthermal, acidotic and hypoxic conditions in four different breast cancer cell lines.
Furthermore, the two-way analysis of variance revealed a hyperthermia-induced down-regulatory effect on secreted miR-139 levels in three of four cell lines, with the exception of the triple-negative cell line MDA-MB-231. ΔCt expression levels of miR-139 were found decreased by a mean value of 4.291 in BT-474 (CI 0.497–8.085, p = 0.034), 4.827 in MCF-7 (CI 1.033–8.621, p = 0.018) and of 4.117 in SK-BR-3 (CI 0.323–7.911, p = 0.041) cells (, ). Single change hyperthermia-dependent effects were observed for secreted miR-let-7a and miR-100 levels in MCF-7 cells. The expression level of miR-let-7a and miR-100 was decreased by a mean value of 1.030 (CI 0.122–1.939, p = 0.033) and 0.3480 (CI 0.038–0.658, p = 0.035) in this cell line (data not shown).
Figure 3. Box plot diagram of expression levels of secreted miR-139 in breast cancer cell lines under varying treatment conditions. Levels of secreted miR-139 expression were determined in MCF-7, BT-474, SK-BR-3 and MDA-MB-231 cells under control conditions, extracellular acidosis, hypoxia or hyperthermia. Box plots demonstrate median (thick black line), lower and upper quantile range (box lines), and standard deviation range (dashed lines bounded by horizontal lines). *Significant expression level alterations (p ≤ 0.05) of control conditions versus treatment options. Based on triplicate experiments, real-time quantitative PCR.
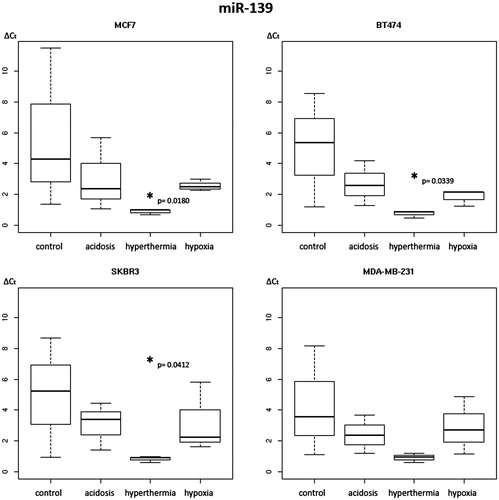
Table 6. Two-way analysis of variance for levels of secreted miR-139 under control, hyperthermic, acidotic and hypoxic conditions in four different breast cancer cell lines
In contrast, hyperthermia induced a specific up-regulation of secreted miR-10a levels with a mean increase of 0.233 in MDA-MB-231 cells (CI 0.059–0.407, p = 0.013) (data not shown). Our analysis could not reveal any significant differences in the expression levels of miR-19b, miR-205 and miR-298 under hyperthermic conditions.
In response to acidosis, single marked alterations in secreted miR-10b and miR-15b expression levels were identified in one cell line only. A mean decrease of secreted miR-10b ΔCt level by 0.132 in MDA-MB-231 cells (CI 0.030–0.234, p = 0.016) and a reduction of miR-15b expression by a mean value of 0.010 in MCF-7 cells (CI 0.003–0.017, p = 0.011) became evident (). Marked effects triggered by hypoxia were found restricted solely to MCF-7 cells in respect of reduced expression levels of secreted miR-15b by a mean value of 0.009 (CI 0.002–0.016, p = 0.014). Oxygen deprivation created a weak effect only on reduced secreted miR-15b expression levels of 0.007 in SK-BR-3 cells (CI 0.000–0.014, p = 0.049).
No significant hyperthermia-dependent miRNA expression level alterations were detected for miRNAs let-7a, miR-10a, -19b, -100, -205 and -298 (, Supplementary ).
Figure 4. Box plot diagrams of expression levels of secreted miRNAs let-7a (A), miR-10a (B), miR-19b (C), miR-100 (D), miR-205 (E) and miR-298 (F) in breast cancer cell lines under varying treatment conditions. Levels of secreted miRNA expression were determined in MCF-7, BT-474, SK-BR-3 and MDA-MB-231 cells under control conditions, extracellular acidosis, hypoxia or hyperthermia. Box plots demonstrate median (thick black line), lower and upper quantile range (box lines), and standard deviation range (dashed lines bounded by horizontal lines). No significant expression level alterations were detected in these miRNA types. Based on triplicate experiments, real-time quantitative PCR.
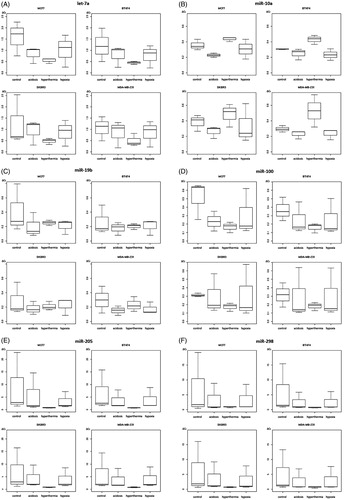
Discussion
In this study we were able to reliably detect a panel of nine secreted BC associated microRNAs, including let-7a, miR-10a, -10b, -15b, -19b, -100, -139, -205, -298 in the supernatants in four BC cell lines (). Subsequently, this in vitro model could be employed for the quantitative examination of circulating (secreted) microRNA expression levels in BC under stress conditions such as hyperthermia, hypoxia and acidosis.
Notably, our data clearly revealed a pronounced hyperthermia-induced regulatory effect in the expression levels of three specific secreted miRNA types among the nine investigated miRNA specimens. Expression levels were determined as median ΔCt values of the distinct miRNA types normalised against the geometric mean of the two housekeeping factors miR-1225 and miR-26b, respectively. While levels of secreted miR-10b and -15b were found significantly down-regulated in all BC cell lines investigated, miR-139 expression levels were found down-regulated in three out of four BC cell types, respectively. This regulatory effect on miRNA expression profiles could preferentially be observed under hyperthermia, but no consistent or comparable trends became apparent under hypoxia or acidosis. Therefore, our data account for a distinct hyperthermia-dependent regulatory process on miRNA expression and secretion with potential impact on cancer cell biology. Our findings in regard to miRNA deregulation via heat shock are in line with several published studies reviewed by Place and Noonan [Citation16]. One study identifies miR-125b, -452, -101, -let-7c, -196a, and -196b as the most significantly regulated miRNA types under thermal treatment in cultured human dermal fibroblast cells [Citation17]. A specific up-regulation of miR-125b, -154 and -382 was reported in human cell lines after induction of mild hyperthermia [Citation92]. Furthermore, another study in small intestine rat tissue showed 18 up-regulated (rno-miR-34b, -137, -154, -672, -219-5p, -375,-7a, -34a, -30a, -500, -185, -203, -200a, -140, -let-7d, -125a-5p, -27b, -210) and 11 down-regulated heat stress-dependent miRNA types (rno-miR-322, -142-5p, -434, -204, -142-3p, -193, -31, -150,-148b-3p, -223, -23a) [Citation93]. One possible underlying mechanism of thermally induced alterations in miRNA expression levels may be the influence on miRNA biogenesis. Oshlag and colleagues identified increased Dicer levels, and hypothesised their potential role as molecular players linking microRNA response with cellular stress [Citation92]. Another suggested explanation is based on the function of heat shock protein complex1 (HSPC1)/heat shock protein 90 (Hsp90) as a major regulator of the RNA-induced silencing complex (RISC) by loading miRNA into this complex. Heat-induced modification in HSPC1/Hsp90 may modulate RISC activity [Citation94]. It is also known that HSPC1/Hsp90 can be secreted into the extracellular compartment.
Functionally miR-10b is a known active promotor of cancer cell migration and invasion [Citation95].
In BC cells, miR-10b could be identified as a target gene of TGF-β1 displaying elevated expression levels during epithelial-mesenchymal transmission processes, thus increasing their metastatic potential. Therefore, miR-10b was recommended as a possible target for gene therapy in BC [Citation96]. In addition, a clinical study observed specifically up-regulated plasma levels of miR-10b in oral cancer patients that clearly discriminate against healthy controls, implying a high potential as an early detection marker in regard to this malignancy [Citation95].
However, the most important functional fact in view of our study is the proven exosome-mediated intercellular transfer of miR-10b in BC cells [Citation97]. Singh and colleagues demonstrated that miRNA-carrying exosomes are subjected to intercellular transfer among different cell lines via a direct uptake. In this process, miR-10b, that was found highly expressed in metastatic BC MDA-MB-231 cells in contrast to non-metastatic BC or non-malignant breast cells, is actively secreted via exosomes. Following the uptake of miR-10b loaded exosomes into non-metastatic BC cells, miR-10b could induce the invasion ability of non-malignant cells [Citation97]. Concerning the expression data obtained in our study (, ), we hypothesise that the application of hyperthermia in the clinical setting bears the potential to promote tumour-suppressive effects via a significant reduction of secreted miR-10b levels subsequently diminishing the metastatic behaviour of BC cells.
Members of the miR-15 gene group are considered as potential cancer prognostic biomarkers, acting either as up-regulated ‘oncomiRs’ or down-regulated tumour-suppressor genes in malignant cells [Citation98]. In HPV-induced cervical cancer, overexpression of oncogenic miR-15b was described as a potential driver of invasion, metastasis and angiogenesis via targeting RECK [Citation99]. MiR-15b is a known inducer of p53 phosphorylation, DNA repair, apoptosis, and cell cycle arrest, possibly via direct targeting of cyclin D1 [Citation100,Citation101]. Contrariwise, overexpression of miR-15b contributes to the chemoresistance mechanism, e.g. cisplatin [Citation102], and promotes epithelial-mesenchymal transition in different malignancies [Citation103]. However, in vitro assays on multi-drug resistance via BCL-2 targeting attested a sensitising effect of miR-15b overexpression in anticancer drug therapy approaches [Citation85]. Rahman and colleagues [Citation81] observed a time- and dose-dependent miR-15b up-regulation under cellular stress caused by irradiation, linking increased miR-15b levels to cell survival pathways including p53.
The observed down-regulatory effect of hyperthermia on miR-15b expression and its putative tumour-biological consequences deserves contemplation concerning the distinct heating and ‘rescue’ protocol applied. Presumably, different protocols could result in variant effects.
Based on these contrary tumour-biological activities and ambivalent effects in regard to varying anticancer treatment regimes, the observed hyperthermia-dependent down-regulation of secreted miR-15b (, ) cannot be considered clearly in regard to a potential therapeutic benefit of hyperthermia in BC management. Further studies are needed to investigate the potential benefit of hyperthermia treatment options in combination with distinct chemo/radiotherapy in regard to the therapeutic influence of miR-15b expression.
The tumour-suppressive regulatory activity of miR-139 could be demonstrated in a variety of different organ malignancies, including breast, lung, colon, liver and oesophagus [Citation67,Citation105–108]. BC cells could be characterised by down-regulated miR-139 expression levels, whereby its positive effects on apoptosis induction or cell cycle arrest and negative impact on cancer cell migration and invasion abilities were found decreased [Citation67]. Most interestingly, miR-139 exerts an opposite function in docetaxel-induced BC cells as a drug-resistance mediator via expression regulation of Notch1 [Citation67]. In colon cancer the miR-139 regulatory influence on target Notch1 results in inhibition of cell proliferation, metastasis, and promotion of apoptosis and cell cycle arrest [Citation108]. Three out of four cell lines investigated in our study exhibited a significant decrease in secreted miR-139 levels in response to hyperthermia (, ). Since secreted levels do not reflect the intracellular levels of this tumour-suppressive miRNA, a hyperthermia-dependent effect in respect to a benefit for BC management remains undefined. However, on the basis of a potential, but so far unknown, signalling function as described for miR-10b, hyperthermia could diminish anti-proliferative effects on BC cells in respect to the known miR-139 activities.
With the focus on secreted miRNA specimen, either determined in cell culture media (in vitro) or liquid patient biopsies (e.g. serum, plasma, urine), their highly potential functional character as intercellular signal vectors requires strict observation. Exosomal miRNAs are discussed as dictators of the microenvironmental niche that influence the benefit of cancer progression via horizontal transfer-enhancing intercellular ‘genetic cargo’ of heterogeneous tumour cell populations [Citation109].
Conclusion
In conclusion, we were able to demonstrate that the expression levels of three microRNAs (miR-10b, -15b and -139) in the supernatants of four BC cell lines were specifically and significantly down-regulated following hyperthermia exposure. These pronounced effects could open the field for miRNAs as novel biomarkers for clinical therapy prediction and monitoring of hyperthermia application in BC management.
IHYT_1161832_Supplemental_data.docx
Download MS Word (647 KB)Disclosure statement
The authors report no conflicts of interest. The authors alone are responsible for the content and writing of the paper.
References
- Romero-Cordoba SL, Salido-Guadarrama I, Rodriguez-Dorantes M, Hidalgo-Miranda A. miRNA biogenesis: biological impact in the development of cancer. Cancer biology & therapy. 2014;15(11):1444–55. doi: 10.4161/15384047.2014.955442.
- Mulrane L, McGee SF, Gallagher WM, O'Connor DP. miRNA dysregulation in breast cancer. Cancer research. 2013;73(22):6554–62. doi: 10.1158/0008-5472.CAN-13-1841.
- Bertoli G, Cava C, Castiglioni I. MicroRNAs: New Biomarkers for Diagnosis, Prognosis, Therapy Prediction and Therapeutic Tools for Breast Cancer. Theranostics. 2015;5(10):1122–43. doi: 10.7150/thno.11543. PubMed PMID: 26199650; PubMed Central PMCID: PMC4508501.
- Kosaka N, Iguchi H, Ochiya T. Circulating microRNA in body fluid: a new potential biomarker for cancer diagnosis and prognosis. Cancer science. 2010;101(10):2087–92. doi: 10.1111/j.1349-7006.2010.01650.x.
- Huang YK, Yu JC. Circulating microRNAs and long non-coding RNAs in gastric cancer diagnosis: An update and review. World journal of gastroenterology. 2015;21(34):9863–86. doi: 10.3748/wjg.v21.i34.9863. PubMed PMID: 26379393; PubMed Central PMCID: PMC4566381.
- Kosaka N, Ochiya T. Unraveling the Mystery of Cancer by Secretory microRNA: Horizontal microRNA Transfer between Living Cells. Frontiers in genetics. 2011;2(97):1–6. doi: 10.3389/fgene.2011.00097. PubMed PMID: 22303391; PubMed Central PMCID: PMC3262223.
- Turchinovich A, Weiz L, Langheinz A, Burwinkel B. Characterization of extracellular circulating microRNA. Nucleic acids research. 2011;39(16):7223–33. doi: 10.1093/nar/gkr254. PubMed PMID: 21609964; PubMed Central PMCID: PMC3167594.
- Arroyo JD, Chevillet JR, Kroh EM, Ruf IK, Pritchard CC, Gibson DF, et al. Argonaute2 complexes carry a population of circulating microRNAs independent of vesicles in human plasma. Proceedings of the National Academy of Sciences of the United States of America. 2011;108(12):5003–8. doi: 10.1073/pnas.1019055108. PubMed PMID: 21383194; PubMed Central PMCID: PMC3064324.
- Wang K, Zhang S, Weber J, Baxter D, Galas DJ. Export of microRNAs and microRNA-protective protein by mammalian cells. Nucleic acids research. 2010;38(20):7248–59. doi: 10.1093/nar/gkq601. PubMed PMID: 20615901; PubMed Central PMCID: PMC2978372.
- Zhang J, Li S, Li L, Li M, Guo C, Yao J, et al. Exosome and exosomal microRNA: trafficking, sorting, and function. Genomics, proteomics & bioinformatics. 2015;13(1):17–24. doi: 10.1016/j.gpb.2015.02.001. PubMed PMID: 25724326; PubMed Central PMCID: PMC4411500.
- Cuk K, Zucknick M, Heil J, Madhavan D, Schott S, Turchinovich A, et al. Circulating microRNAs in plasma as early detection markers for breast cancer. International journal of cancer Journal international du cancer. 2013;132(7):1602–12. doi: 10.1002/ijc.27799.
- Zhao FL, Hu GD, Wang XF, Zhang XH, Zhang YK, Yu ZS. Serum overexpression of microRNA-10b in patients with bone metastatic primary breast cancer. The Journal of international medical research. 2012;40(3):859–66.
- Cortez MA, Welsh JW, Calin GA. Circulating microRNAs as noninvasive biomarkers in breast cancer. Recent results in cancer research Fortschritte der Krebsforschung Progres dans les recherches sur le cancer. 2012;195:151–61. doi: 10.1007/978-3-642-28160-0_13. PubMed PMID: 22527502; PubMed Central PMCID: PMC3855311.
- Redova M, Sana J, Slaby O. Circulating miRNAs as new blood-based biomarkers for solid cancers. Future oncology. 2013;9(3):387–402. doi: 10.2217/fon.12.192.
- Schwarzenbach H. Circulating nucleic acids as biomarkers in breast cancer. Breast cancer research: BCR. 2013;15(5(211)):1–9. doi: 10.1186/bcr3446. PubMed PMID: 24090167; PubMed Central PMCID: PMC3978696.
- Place RF, Noonan EJ. Non-coding RNAs turn up the heat: an emerging layer of novel regulators in the mammalian heat shock response. Cell stress & chaperones. 2014;19(2):159–72. doi: 10.1007/s12192-013-0456-5. PubMed PMID: 24002685; PubMed Central PMCID: PMC3933615.
- Wilmink GJ, Roth CL, Ibey BL, Ketchum N, Bernhard J, Cerna CZ, et al. Identification of microRNAs associated with hyperthermia-induced cellular stress response. Cell stress & chaperones. 2010;15(6):1027–38. doi: 10.1007/s12192-010-0189-7. PubMed PMID: 20352393; PubMed Central PMCID: PMC3024070.
- Zhang XL, Shi HJ, Wang JP, Tang HS, Wu YB, Fang ZY, et al. MicroRNA-218 is upregulated in gastric cancer after cytoreductive surgery and hyperthermic intraperitoneal chemotherapy and increases chemosensitivity to cisplatin. World journal of gastroenterology. 2014;20(32):11347–55. doi: 10.3748/wjg.v20.i32.11347. PubMed PMID: 25170221; PubMed Central PMCID: PMC4145775.
- Manjili MH, Wang XY, Park J, Macdonald IJ, Li Y, Van Schie RC, et al. Cancer immunotherapy: stress proteins and hyperthermia. International journal of hyperthermia: the official journal of European Society for Hyperthermic Oncology, North American Hyperthermia Group. 2002;18(6):506–20. doi: 10.1080/02656730110116696.
- Morey TM, Roufayel R, Johnston DS, Fletcher AS, Mosser DD. Heat shock inhibition of CDK5 increases NOXA levels through miR-23a repression. The Journal of biological chemistry. 2015;290(18):11443–54. doi: 10.1074/jbc.M114.625988. PubMed PMID: 25829494; PubMed Central PMCID: PMC4416849.
- Ozgur A, Tutar L, Tutar Y. Regulation of Heat Shock Proteins by miRNAs in human breast cancer. MicroRNA. 2014;3(2):118–35.
- Diagnosis and treatment of patients with primary and metastatic breast cancer [Internet]. Comission Mamma, Arbeitsgemeinschaft Gynäkologische Onkologie (AGO) e.V. 2014 [cited March 2014]. Available from: http://www.ago-online.de/fileadmin/downloads/leitlinien/mamma/maerz2014/AGO_Mamma_2014_en.zip.
- Oldenborg S, Van Os RM, Van rij CM, Crezee J, Van de Kamer JB, Rutgers EJ, et al. Elective re-irradiation and hyperthermia following resection of persistent locoregional recurrent breast cancer: A retrospective study. International journal of hyperthermia: the official journal of European Society for Hyperthermic Oncology, North American Hyperthermia Group. 2010;26(2):136–44. doi: 10.3109/02656730903341340.
- Van Der Zee J, De Bruijne M, Mens JW, Ameziane A, Broekmeyer-Reurink MP, Drizdal T, et al. Reirradiation combined with hyperthermia in breast cancer recurrences: overview of experience in Erasmus MC. International journal of hyperthermia: the official journal of European Society for Hyperthermic Oncology, North American Hyperthermia Group. 2010;26(7):638–48. doi: 10.3109/02656736.2010.495104.
- Vujaskovic Z, Kim DW, Jones E, Lan L, McCall L, Dewhirst MW, et al. A phase I/II study of neoadjuvant liposomal doxorubicin, paclitaxel, and hyperthermia in locally advanced breast cancer. International journal of hyperthermia: the official journal of European Society for Hyperthermic Oncology, North American Hyperthermia Group. 2010;26(5):514–21. doi: 10.3109/02656731003639364. PubMed PMID: 20377362; PubMed Central PMCID: PMC2956498.
- Zagar TM, Oleson JR, Vujaskovic Z, Dewhirst MW, Craciunescu OI, Blackwell KL, et al. Hyperthermia for locally advanced breast cancer. International journal of hyperthermia: the official journal of European Society for Hyperthermic Oncology, North American Hyperthermia Group. 2010;26(7):618–24. doi: 10.3109/02656736.2010.501051. PubMed PMID: 20849257; PubMed Central PMCID: PMC2949291.
- Zagar TM, Oleson JR, Vujaskovic Z, Dewhirst MW, Craciunescu OI, Blackwell KL, et al. Hyperthermia combined with radiation therapy for superficial breast cancer and chest wall recurrence: a review of the randomised data. International journal of hyperthermia: the official journal of European Society for Hyperthermic Oncology, North American Hyperthermia Group. 2010;26(7):612–7. doi: 10.3109/02656736.2010.487194. PubMed PMID: 20849256; PubMed Central PMCID: PMC2956421.
- Vernon CC, Hand JW, Field SB, Machin D, Whaley JB, van der Zee J, et al. Radiotherapy with or without hyperthermia in the treatment of superficial localized breast cancer: results from five randomized controlled trials. International Collaborative Hyperthermia Group. International journal of radiation oncology, biology, physics. 1996;35(4):731–44.
- Welz S, Hehr T, Lamprecht U, Scheithauer H, Budach W, Bamberg M. Thermoradiotherapy of the chest wall in locally advanced or recurrent breast cancer with marginal resection. International journal of hyperthermia: the official journal of European Society for Hyperthermic Oncology, North American Hyperthermia Group. 2005;21(2):159–67. doi: 10.1080/02656730400003393.
- Li G, Mitsumori M, Ogura M, Horii N, Kawamura S, Masunaga S, et al. Local hyperthermia combined with external irradiation for regional recurrent breast carcinoma. International journal of clinical oncology. 2004;9(3):179–83. doi: 10.1007/s10147-004-0395-3.
- Linthorst M, Baaijens M, Wiggenraad R, Creutzberg C, Ghidey W, van Rhoon GC, et al. Local control rate after the combination of re-irradiation and hyperthermia for irresectable recurrent breast cancer: Results in 248 patients. Radiotherapy and oncology: journal of the European Society for Therapeutic Radiology and Oncology. 2015;117(2):217–22. doi: 10.1016/j.radonc.2015.04.019.
- Linthorst M, van Rhoon GC, van Geel AN, Baaijens M, Ghidey W, Broekmeyer-Reurink MP, et al. The tolerance of reirradiation and hyperthermia in breast cancer patients with reconstructions. International journal of hyperthermia: the official journal of European Society for Hyperthermic Oncology, North American Hyperthermia Group. 2012;28(3):267–77. doi: 10.3109/02656736.2012.663951.
- Wang X, Cao L, Wang Y, Wang X, Liu N, You Y. Regulation of let-7 and its target oncogenes (Review). Oncology letters. 2012;3(5):955–60. doi: 10.3892/ol.2012.609. PubMed PMID: 22783372; PubMed Central PMCID: PMC3389667.
- Zhao Y, Deng C, Wang J, Xiao J, Gatalica Z, Recker RR, et al. Let-7 family miRNAs regulate estrogen receptor alpha signaling in estrogen receptor positive breast cancer. Breast cancer research and treatment. 2011;127(1):69–80. doi: 10.1007/s10549-010-0972-2.
- Sun X, Qin S, Fan C, Xu C, Du N, Ren H. Let-7: a regulator of the ERalpha signaling pathway in human breast tumors and breast cancer stem cells. Oncology reports. 2013;29(5):2079–87. doi: 10.3892/or.2013.2330.
- Wu J, Li S, Jia W, Deng H, Chen K, Zhu L, et al. Reduced Let-7a Is Associated with Chemoresistance in Primary Breast Cancer. PloS one. 2015;10(7):e0133643. doi: 10.1371/journal.pone.0133643. PubMed PMID: 26218285; PubMed Central PMCID: PMC4517895.
- Heneghan HM, Miller N, Lowery AJ, Sweeney KJ, Newell J, Kerin MJ. Circulating microRNAs as novel minimally invasive biomarkers for breast cancer. Annals of surgery. 2010;251(3):499–505. doi: 10.1097/SLA.0b013e3181cc939f.
- Perez-Rivas LG, Jerez JM, Carmona R, de Luque V, Vicioso L, Claros MG, et al. A microRNA signature associated with early recurrence in breast cancer. PloS one. 2014;9(3):e91884. doi: 10.1371/journal.pone.0091884. PubMed PMID: 24632820; PubMed Central PMCID: PMC3954835.
- Hoppe R, Achinger-Kawecka J, Winter S, Fritz P, Lo WY, Schroth W, et al. Increased expression of miR-126 and miR-10a predict prolonged relapse-free time of primary oestrogen receptor-positive breast cancer following tamoxifen treatment. European journal of cancer. 2013;49(17):3598–608. doi: 10.1016/j.ejca.2013.07.145.
- Ma L, Teruya-Feldstein J, Weinberg RA. Tumour invasion and metastasis initiated by microRNA-10b in breast cancer. Nature. 2007;449(7163):682–8. doi: 10.1038/nature06174.
- Mar-Aguilar F, Mendoza-Ramirez JA, Malagon-Santiago I, Espino-Silva PK, Santuario-Facio SK, Ruiz-Flores P, et al. Serum circulating microRNA profiling for identification of potential breast cancer biomarkers. Disease markers. 2013;34(3):163–9. doi: 10.3233/DMA-120957. PubMed PMID: 23334650; PubMed Central PMCID: PMC3810231.
- Taguchi YH, Murakami Y. Principal component analysis based feature extraction approach to identify circulating microRNA biomarkers. PloS one. 2013;8(6):e66714. doi: 10.1371/journal.pone.0066714. PubMed PMID: 23874370; PubMed Central PMCID: PMC3715582.
- Kedmi M, Ben-Chetrit N, Korner C, Mancini M, Ben-Moshe NB, Lauriola M, et al. EGF induces microRNAs that target suppressors of cell migration: miR-15b targets MTSS1 in breast cancer. Science signaling. 2015;8(368):ra29. doi: 10.1126/scisignal.2005866.
- Fan Y, Yin S, Hao Y, Yang J, Zhang H, Sun C, et al. miR-19b promotes tumor growth and metastasis via targeting TP53. Rna. 2014;20(6):765–72. doi: 10.1261/rna.043026.113. PubMed PMID: 24742936; PubMed Central PMCID: PMC4024631.
- Castellano L, Giamas G, Jacob J, Coombes RC, Lucchesi W, Thiruchelvam P, et al. The estrogen receptor-alpha-induced microRNA signature regulates itself and its transcriptional response. Proceedings of the National Academy of Sciences of the United States of America. 2009;106(37):15732–7. doi: 10.1073/pnas.0906947106. PubMed PMID: 19706389; PubMed Central PMCID: PMC2747188.
- Chen WX, Liu XM, Lv MM, Chen L, Zhao JH, Zhong SL, et al. Exosomes from drug-resistant breast cancer cells transmit chemoresistance by a horizontal transfer of microRNAs. PloS one. 2014;9(4):e95240. doi: 10.1371/journal.pone.0095240. PubMed PMID: 24740415; PubMed Central PMCID: PMC3989268.
- Gebeshuber CA, Martinez J. miR-100 suppresses IGF2 and inhibits breast tumorigenesis by interfering with proliferation and survival signaling. Oncogene. 2013;32(27):3306–10. doi: 10.1038/onc.2012.372.
- Yang Y, Xing Y, Liang C, Hu L, Xu F, Chen Y. Crucial microRNAs and genes of human primary breast cancer explored by microRNA-mRNA integrated analysis. Tumour biology: the journal of the International Society for Oncodevelopmental Biology and Medicine. 2015;36(7):5571–9. doi: 10.1007/s13277-015-3227-3.
- Krishnan K, Steptoe AL, Martin HC, Pattabiraman DR, Nones K, Waddell N, et al. miR-139-5p is a regulator of metastatic pathways in breast cancer. Rna. 2013;19(12):1767–80. doi: 10.1261/rna.042143.113. PubMed PMID: 24158791; PubMed Central PMCID: PMC3884652.
- Zhang H, Li B, Zhao H, Chang J. The expression and clinical significance of serum miR-205 for breast cancer and its role in detection of human cancers. International journal of clinical and experimental medicine. 2015;8(2):3034–43. PubMed PMID: 25932280; PubMed Central PMCID: PMC4402927.
- Shaker O, Maher M, Nassar Y, Morcos G, Gad Z. Role of microRNAs -29b-2, -155, -197 and -205 as diagnostic biomarkers in serum of breast cancer females. Gene. 2015;560(1):77–82. doi: 10.1016/j.gene.2015.01.062.
- Bao L, Hazari S, Mehra S, Kaushal D, Moroz K, Dash S. Increased expression of P-glycoprotein and doxorubicin chemoresistance of metastatic breast cancer is regulated by miR-298. The American journal of pathology. 2012;180(6):2490–503. doi: 10.1016/j.ajpath.2012.02.024. PubMed PMID: 22521303; PubMed Central PMCID: PMC3378910.
- Giovannetti E, Erozenci A, Smit J, Danesi R, Peters GJ. Molecular mechanisms underlying the role of microRNAs (miRNAs) in anticancer drug resistance and implications for clinical practice. Critical reviews in oncology/hematology. 2012;81(2):103–22. doi: 10.1016/j.critrevonc.2011.03.010.
- Magee P, Shi L, Garofalo M. Role of microRNAs in chemoresistance. Annals of translational medicine. 2015;3(21:332):1–9. doi: 10.3978/j.issn.2305-5839.2015.11.32. PubMed PMID: 26734642; PubMed Central PMCID: PMC4690999.
- Li J, Zhang Y, Zhao Q, Wang J, He X. MicroRNA-10a Influences Osteoblast Differentiation and Angiogenesis by Regulating beta-Catenin Expression. Cellular physiology and biochemistry: international journal of experimental cellular physiology, biochemistry, and pharmacology. 2015;37(6):2194–208. doi: 10.1159/000438576.
- Yu T, Liu L, Li J, Yan M, Lin H, Liu Y, et al. MiRNA-10a is upregulated in NSCLC and may promote cancer by targeting PTEN. Oncotarget. 2015;6(30):30239–50. doi: 10.18632/oncotarget.4972.
- Ahmad A, Ginnebaugh KR, Yin S, Bollig-Fischer A, Reddy KB, Sarkar FH. Functional role of miR-10b in tamoxifen resistance of ER-positive breast cancer cells through down-regulation of HDAC4. BMC cancer. 2015;15:540. doi: 10.1186/s12885-015-1561-x. PubMed PMID: 26206152; PubMed Central PMCID: PMC4512090.
- Fkih M'hamed I, Privat M, Ponelle F, Penault-Llorca F, Kenani A, Bignon YJ. Identification of miR-10b, miR-26a, miR-146a and miR-153 as potential triple-negative breast cancer biomarkers. Cellular oncology. 2015;38(6):433–42. doi: 10.1007/s13402-015-0239-3. PubMed PMID: 26392359; PubMed Central PMCID: PMC4653246.
- Ma Z, Chen Y, Min L, Li L, Huang H, Li J, et al. Augmented miR-10b expression associated with depressed expression of its target gene KLF4 involved in gastric carcinoma. International journal of clinical and experimental pathology. 2015;8(5):5071–9. PubMed PMID: 26191201; PubMed Central PMCID: PMC4503073.
- Yoo B, Kavishwar A, Ross A, Wang P, Tabassum DP, Polyak K, et al. Combining miR-10b-Targeted Nanotherapy with Low-Dose Doxorubicin Elicits Durable Regressions of Metastatic Breast Cancer. Cancer research. 2015;75(20):4407–15. doi: 10.1158/0008-5472.CAN-15-0888. PubMed PMID: 26359455; PubMed Central PMCID: PMC4609288.
- Guo Y, Lang X, Lu Z, Wang J, Li T, Liao Y, et al. MiR-10b Directly Targets ZEB1 and PIK3CA to Curb Adenomyotic Epithelial Cell Invasiveness via Upregulation of E-Cadherin and Inhibition of Akt Phosphorylation. Cellular physiology and biochemistry: international journal of experimental cellular physiology, biochemistry, and pharmacology. 2015;35(6):2169–80. doi: 10.1159/000374022.
- Jia Z, Wang K, Zhang A, Wang G, Kang C, Han L, et al. miR-19a and miR-19b overexpression in gliomas. Pathology oncology research: POR. 2013;19(4):847–53. doi: 10.1007/s12253-013-9653-x.
- Jiang Q, He M, Guan S, Ma M, Wu H, Yu Z, et al. MicroRNA-100 suppresses the migration and invasion of breast cancer cells by targeting FZD-8 and inhibiting Wnt/beta-catenin signaling pathway. Tumour biology: the journal of the International Society for Oncodevelopmental Biology and Medicine. 2015;37:5001–11. doi: 10.1007/s13277-015-4342-x.
- Luan Y, Zhang S, Zuo L, Zhou L. Overexpression of miR-100 inhibits cell proliferation, migration, and chemosensitivity in human glioblastoma through FGFR3. OncoTargets and therapy. 2015;8:3391–400. doi: 10.2147/OTT.S85677. PubMed PMID: 26604796; PubMed Central PMCID: PMC4655956.
- Zhang B, Zhao R, He Y, Fu X, Fu L, Zhu Z, et al. Micro RNA 100 sensitizes luminal A breast cancer cells to paclitaxel treatment in part by targeting mTOR. Oncotarget. 2015;7(5):5702–14. doi: 10.18632/oncotarget.6790.
- Sun C, Sang M, Li S, Sun X, Yang C, Xi Y, et al. Hsa-miR-139-5p inhibits proliferation and causes apoptosis associated with down-regulation of c-Met. Oncotarget. 2015;6(37):39756–92. doi: 10.18632/oncotarget.5476.
- Zhang HD, Sun DW, Mao L, Zhang J, Jiang LH, Li J, et al. MiR-139-5p inhibits the biological function of breast cancer cells by targeting Notch1 and mediates chemosensitivity to docetaxel. Biochemical and biophysical research communications. 2015;465(4):702–13. doi: 10.1016/j.bbrc.2015.08.053.
- Li P, Xue WJ, Feng Y, Mao QS. MicroRNA-205 functions as a tumor suppressor in colorectal cancer by targeting cAMP responsive element binding protein 1 (CREB1). American journal of translational research. 2015;7(10):2053–9. PubMed PMID: 26692949; PubMed Central PMCID: PMC4656782.
- Niu K, Shen W, Zhang Y, Zhao Y, Lu Y. MiR-205 promotes motility of ovarian cancer cells via targeting ZEB1. Gene. 2015;574(2):330–6. doi: 10.1016/j.gene.2015.08.017.
- Yang G, Zhang P, Lv A, Liu Y, Wang G. MiR-205 functions as a tumor suppressor via targeting TGF-alpha in osteosarcoma. Experimental and molecular pathology. 2016;100(1):160–6. doi: 10.1016/j.yexmp.2015.12.010.
- Zhang H, Fan Q. MicroRNA-205 inhibits the proliferation and invasion of breast cancer by regulating AMOT expression. Oncology reports. 2015;34(4):2163–70. doi: 10.3892/or.2015.4148.
- Wu S, Huang S, Ding J, Zhao Y, Liang L, Liu T, et al. Multiple microRNAs modulate p21Cip1/Waf1 expression by directly targeting its 3' untranslated region. Oncogene. 2010;29(15):2302–8. doi: 10.1038/onc.2010.34.
- Zhao H, Zhao D, Tan G, Liu Y, Zhuang L, Liu T. Bufalin promotes apoptosis of gastric cancer by down-regulation of miR-298 targeting bax. International journal of clinical and experimental medicine. 2015;8(3):3420–8. PubMed PMID: 26064232; PubMed Central PMCID: PMC4443066.
- Garcia MP, Cavalheiro JR, Fernandes MH. Acute and long-term effects of hyperthermia in B16-F10 melanoma cells. PloS one. 2012;7(4):e35489: 1–9. doi: 10.1371/journal.pone.0035489. PubMed PMID: 22532856; PubMed Central PMCID: PMC3332049.
- Habash RW, Bansal R, Krewski D, Alhafid HT. Thermal therapy, part 2: hyperthermia techniques. Critical reviews in biomedical engineering. 2006;34(6):491–542.
- Habash RW, Bansal R, Krewski D, Alhafid HT. Thermal therapy, part 1: an introduction to thermal therapy. Critical reviews in biomedical engineering. 2006;34(6):459–89.
- Heese C, Lavagnini P, Mills P, Lewis M, Markman M. Superficial Hyperthermia plus External Beam Radiation in the Palliation of Locally Progressive Chemoradiation-Resistant Breast Cancer. Case reports in oncology. 2012;5(3):520–3. doi: 10.1159/000343041. PubMed PMID: 23139665; PubMed Central PMCID: PMC3492962.
- Kampinga HH. Cell biological effects of hyperthermia alone or combined with radiation or drugs: a short introduction to newcomers in the field. International journal of hyperthermia: the official journal of European Society for Hyperthermic Oncology, North American Hyperthermia Group. 2006;22(3):191–6. doi: 10.1080/02656730500532028.
- Larson N, Gormley A, Frazier N, Ghandehari H. Synergistic enhancement of cancer therapy using a combination of heat shock protein targeted HPMA copolymer-drug conjugates and gold nanorod induced hyperthermia. Journal of controlled release: official journal of the Controlled Release Society. 2013;170(1):41–50. doi: 10.1016/j.jconrel.2013.04.006. PubMed PMID: 23602864; PubMed Central PMCID: PMC3860371.
- Baranzio GF, Hager ED. Hyperthermia In Cancer Treatment: A Primer. 2006;1:110–27. doi: 10.1007/978-0-387-33441-7.
- Toraya-Brown S, Fiering S. Local tumour hyperthermia as immunotherapy for metastatic cancer. International journal of hyperthermia: the official journal of European Society for Hyperthermic Oncology, North American Hyperthermia Group. 2014;30(8):531–9. doi: 10.3109/02656736.2014.968640. PubMed PMID: 25430985; PubMed Central PMCID: PMC4558619.
- Triantopoulou S, Efstathopoulos E, Platoni K, Uzunoglou N, Kelekis N, Kouloulias V. Radiotherapy in conjunction with superficial and intracavitary hyperthermia for the treatment of solid tumors: survival and thermal parameters. Clinical & translational oncology: official publication of the Federation of Spanish Oncology Societies and of the National Cancer Institute of Mexico. 2013;15(2):95–105. doi: 10.1007/s12094-012-0947-3.
- van der Zee J, de Bruijne M, van Rhoon GC. Thermal medicine, heat shock proteins and cancer. International journal of hyperthermia: the official journal of European Society for Hyperthermic Oncology, North American Hyperthermia Group. 2006;22(5):433–37; author reply 7-47. doi: 10.1080/02656730600768589.
- Varkonyi-Gasic E, Hellens RP. Quantitative stem-loop RT-PCR for detection of microRNAs. Methods in molecular biology. 2011;744:145–57. doi: 10.1007/978-1-61779-123-9_10.
- Varkonyi-Gasic E, Wu R, Wood M, Walton EF, Hellens RP. Protocol: a highly sensitive RT-PCR method for detection and quantification of microRNAs. Plant methods. 2007;3(12):1–12. doi: 10.1186/1746-4811-3-12. PubMed PMID: 17931426; PubMed Central PMCID: PMC2225395.
- Pfaffl MW, Tichopad A, Prgomet C, Neuvians TP. Determination of stable housekeeping genes, differentially regulated target genes and sample integrity: BestKeeper–Excel-based tool using pair-wise correlations. Biotechnology letters. 2004;26(6):509–15.
- Chou CH, Chang NW, Shrestha S, Hsu SD, Lin YL, Lee WH, et al. miRTarBase 2016: updates to the experimentally validated miRNA-target interactions database. Nucleic acids research. 2016;44(D1):D239–47. doi: 10.1093/nar/gkv1258. PubMed PMID: 26590260; PubMed Central PMCID: PMC4702890.
- Fang JS, Gillies RD, Gatenby RA. Adaptation to hypoxia and acidosis in carcinogenesis and tumor progression. Seminars in cancer biology. 2008;18(5):330–7. doi: 10.1016/j.semcancer.2008.03.011. PubMed PMID: 18455429; PubMed Central PMCID: PMC2953714.
- Gatenby RA, Gillies RJ. Why do cancers have high aerobic glycolysis? Nature reviews Cancer. 2004;4(11):891–9. doi: 10.1038/nrc1478.
- Gatenby RA, Smallbone K, Maini PK, Rose F, Averill J, Nagle RB, et al. Cellular adaptations to hypoxia and acidosis during somatic evolution of breast cancer. British journal of cancer. 2007;97(5):646–53. doi: 10.1038/sj.bjc.6603922. PubMed PMID: 17687336; PubMed Central PMCID: PMC2360372.
- Sameni M, Mullins SR, Moin K, Sloane BF, Osuala K. Importance of the Tumor Microenvironment. In: Ahmad A, editor. Breast Cancer Metastasis and Drug Resistance: Progress and Prospects. 1. 1 ed. New York: Springer; 2013. p. 178–9.
- Oshlag JZ, Devasthanam AS, Tomasi TB. Mild hyperthermia enhances the expression and induces oscillations in the Dicer protein. International journal of hyperthermia: the official journal of European Society for Hyperthermic Oncology, North American Hyperthermia Group. 2013;29(1):51–61. doi: 10.3109/02656736.2012.753471.
- Yu J, Liu F, Yin P, Zhu X, Cheng G, Wang N, et al. Integrating miRNA and mRNA expression profiles in response to heat stress-induced injury in rat small intestine. Functional & integrative genomics. 2011;11(2):203–13. doi: 10.1007/s10142-010-0198-8.
- Iwasaki S, Kobayashi M, Yoda M, Sakaguchi Y, Katsuma S, Suzuki T, et al. Hsc70/Hsp90 chaperone machinery mediates ATP-dependent RISC loading of small RNA duplexes. Molecular cell. 2010;39(2):292–9. doi: 10.1016/j.molcel.2010.05.015.
- Lu YC, Chen YJ, Wang HM, Tsai CY, Chen WH, Huang YC, et al. Oncogenic function and early detection potential of miRNA-10b in oral cancer as identified by microRNA profiling. Cancer prevention research. 2012;5(4):665–74. doi: 10.1158/1940-6207.CAPR-11-0358.
- Han X, Yan S, Weijie Z, Feng W, Liuxing W, Mengquan L, et al. Critical role of miR-10b in transforming growth factor-beta1-induced epithelial-mesenchymal transition in breast cancer. Cancer gene therapy. 2014;21(2):60–7. doi: 10.1038/cgt.2013.82.
- Singh R, Pochampally R, Watabe K, Lu Z, Mo YY. Exosome-mediated transfer of miR-10b promotes cell invasion in breast cancer. Molecular cancer. 2014;13(256):1–11. doi: 10.1186/1476-4598-13-256. PubMed PMID: 25428807; PubMed Central PMCID: PMC4258287.
- Finnerty JR, Wang WX, Hebert SS, Wilfred BR, Mao G, Nelson PT. The miR-15/107 group of microRNA genes: evolutionary biology, cellular functions, and roles in human diseases. Journal of molecular biology. 2010;402(3):491–509. doi: 10.1016/j.jmb.2010.07.051. PubMed PMID: 20678503; PubMed Central PMCID: PMC2978331.
- Gomez-Gomez Y, Organista-Nava J, Gariglio P. Deregulation of the miRNAs expression in cervical cancer: human papillomavirus implications. BioMed research international. 2013;2013:407052. doi: 10.1155/2013/407052. PubMed PMID: 24490161; PubMed Central PMCID: PMC3899709.
- Rahman M, Lovat F, Romano G, Calore F, Acunzo M, Bell EH, et al. miR-15b/16-2 regulates factors that promote p53 phosphorylation and augments the DNA damage response following radiation in the lung. The Journal of biological chemistry. 2014;289(38):26406–16. doi: 10.1074/jbc.M114.573592. PubMed PMID: 25092292; PubMed Central PMCID: PMC4176210.
- Sun G, Shi L, Yan S, Wan Z, Jiang N, Fu L, et al. MiR-15b targets cyclin D1 to regulate proliferation and apoptosis in glioma cells. BioMed research international. 2014;2014:687826. doi: 10.1155/2014/687826. PubMed PMID: 24995320; PubMed Central PMCID: PMC4065679.
- Zhao Z, Zhang L, Yao Q, Tao Z. miR-15b regulates cisplatin resistance and metastasis by targeting PEBP4 in human lung adenocarcinoma cells. Cancer gene therapy. 2015;22(3):108–14. doi: 10.1038/cgt.2014.73.
- Zhang WL, Zhang JH, Wu XZ, Yan T, Lv W. miR-15b promotes epithelial-mesenchymal transition by inhibiting SMURF2 in pancreatic cancer. International journal of oncology. 2015;47(3):1043–53. doi: 10.3892/ijo.2015.3076.
- Xia L, Zhang D, Du R, Pan Y, Zhao L, Sun S, et al. miR-15b and miR-16 modulate multidrug resistance by targeting BCL2 in human gastric cancer cells. International journal of cancer Journal international du cancer. 2008;123(2):372–9. doi: 10.1002/ijc.23501.
- Gu W, Li X, Wang J. miR-139 regulates the proliferation and invasion of hepatocellular carcinoma through the WNT/TCF-4 pathway. Oncology reports. 2014;31(1):397–404. doi: 10.3892/or.2013.2831.
- Liu R, Yang M, Meng Y, Liao J, Sheng J, Pu Y, et al. Tumor-suppressive function of miR-139-5p in esophageal squamous cell carcinoma. PloS one. 2013;8(10):e77068. doi: 10.1371/journal.pone.0077068. PubMed PMID: 24204738; PubMed Central PMCID: PMC3799985.
- Xu W, Hang M, Yuan CY, Wu FL, Chen SB, Xue K. MicroRNA-139-5p inhibits cell proliferation and invasion by targeting insulin-like growth factor 1 receptor in human non-small cell lung cancer. International journal of clinical and experimental pathology. 2015;8(4):3864–70. PubMed PMID: 26097570; PubMed Central PMCID: PMC4466957.
- Zhang L, Dong Y, Zhu N, Tsoi H, Zhao Z, Wu CW, et al. microRNA-139-5p exerts tumor suppressor function by targeting NOTCH1 in colorectal cancer. Molecular cancer. 2014;13(124):1-12. doi: 10.1186/1476-4598-13-124. PubMed PMID: 24885920; PubMed Central PMCID: PMC4065091.
- Falcone G, Felsani A, D'Agnano I. Signaling by exosomal microRNAs in cancer. Journal of experimental & clinical cancer research: CR. 2015;34(32):1–10. doi: 10.1186/s13046-015-0148-3. PubMed PMID: 25886763; PubMed Central PMCID: PMC4391656.