Abstract
Background. The high interstitial fluid pressure (IFP) in solid tumors restricts the access to nutrients, oxygen and drugs. Material and methods. We investigated the ability of the peptide AF-16, involved in water and ion transfer through cell membranes, to lower the IFP in two different solid rat mammary tumors, one chemically induced, slowly growing, and the other transplantable, and rapidly progressing having high cellularity. AF-16 was administered either in the tumor capsule, intranasally or intravenously. The IFP was measured by a miniature fiber optic device. Results. AF-16 significantly lowered the IFP in both the slowly and the rapidly progressing tumors, whether administrated locally or systemically. The AF-16 induced IFP reduction was maximal after 90 min, lasted at least 3 h, and returned to pretreatment levels in less than 24 h. Topical AF-16 transiently reduced the IFP in the DMBA tumors from 17.7 ± 4.2 mmHg to 8.6 ± 2.1 mmHg. Conclusion. We conclude that AF-16 transiently and reversibly lowered the high IFP in solid tumors during a few hours, which might translate into improved therapeutic efficacy.
High interstitial fluid pressure (IFP) is a characteristic feature of most solid, malignant tumors [Citation1]. The increased IFP disturbs the blood flow and the distribution of immune competent cells, drugs, nutrients, and oxygen in the tumor [Citation1–4]. The pathogenesis of the increased IFP in solid tumor is complex, but immunogenic inflammation with leaky immature vessels within a frequently fibrous, dense tissue lacking sufficient lymph drainage is at least part of the background. The high IFP causes a “compartment syndrome” preventing access of oxygen and cancer chemotherapy. A lowering of the tumor IFP is likely to facilitate the availability and distribution of drugs in tumor tissue [Citation1,Citation3–9].
Antisecretory Factor (AF) is a ubiquitously expressed endogenous protein with potent antisecretory and anti-inflammatory actions [Citation10,Citation11]. AF, also named S5a, is a component of the regulatory part of the proteasome, and in most cells there is a free cytoplasmatic pool of the protein exerting effects not related to the proteasome. The antisecretory and anti-inflammatory active site of AF is an eight amino acids long sequence in the N-terminal part of the protein [Citation10]. A somewhat longer peptide, AF-16, is more stable and therefore suitable for experimental work [Citation12]. In a recent study, in which herpes simplex encephalitis was induced in rats, we reported that treatment with AF-16 significantly reduced the mortality [Citation12]. No effect of the treatment on virus replication was demonstrated. The main finding was that AF-16 normalized the raised intracranial pressure caused by the infection. Most likely this effect was due to the peptide's ability to reduce inflammation and subsequently edema. Solid tumors, often surrounded by a capsule, constitute a closed compartment where tumor growth is the major cause of the raised IFP [Citation9], but inflammation probably also contributes to the increase in IFP. The conditions in solid tumors thus show certain similarities with those during infection induced brain edema. Consequently it was of interest to investigate if AF-16 could affect the raised IFP also in solid tumors.
The aim of the present study was to investigate if AF-16 could modulate the high IFP in two different solid rat mammary tumors.
Material and methods
Animals and tumors
Female Sprague-Dawley rats (52 days old, 150–165 g body weight (bw); B & K AB, Stockholm, Sweden) had a single oral dose of 16 mg 9,10-dimethyl-1, 2-benzanthracene (DMBA; Sigma-Aldrich) under isoflurane anesthesia, and developed tumors in 3–4 months [Citation2]. The tumors were discoid, and localized along the mammary ridges, occasionally bilaterally. The DMBA tumors displayed the general appearance of an adenocarcinoma, growing in lobules, divided by strands of connective tissue (). The DMBA tumors were delimited by a distinct connective tissue capsule.
Figure 1. Histopathology of DMBA and MAT B III tumors. Paraffin sections stained with hematoxylin/eosin. Bars: A and B 100 μm; C and D 50 μm. A. Light micrograph showing a DMBA tumor. Cords of tumor cells form gland-like structures, which are separated by strands of dense connective tissue. Note the distinct, thick capsule. Htx/E. B. Light micrograph of a MAT B III tumor, showing low differentiation of the densely packed tumor cells, absence of organized structures, scanty extracellular matrix and irregular infiltration by tumor cells in the surrounding connective tissue. C. Higher magnification of a DMBA tumor, showing the differentiated glandular appearance of the tumor. D. Higher magnification of a MAT B III tumor, showing the densely packed pleomorphic tumor cells. Occasional mitotic figures are seen.
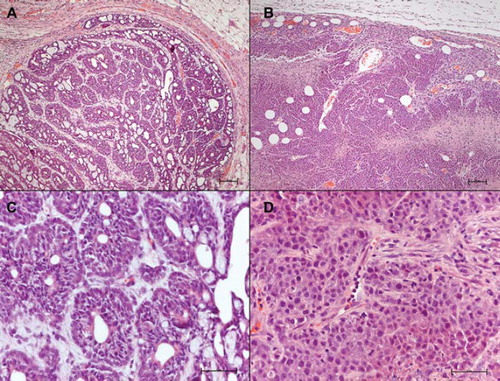
Female Fisher 344 rats (60 days old, 160–170 g bw; B & K) had a subcutaneous injection in the neck, between the scapulae, of cultured suspended MAT B III cells (106 cells in 0.25 mL/ rat; ATCC; CRL-1666; # 13762). A spindle-shaped, rapidly growing tumor mass appeared in a week or two (). Light microscopy demonstrated that the MAT B III tumors initially were solid with scanty extracellular matrix, and with increasing size necrotic areas appeared, delimited by vital cells (). In larger tumors the necrotic parts fused and formed cysts. The connective tissue surrounding the MAT B III tumors formed no distinct capsule.
Figure 2. IFP measurement setup. A. An anaesthetized Fisher rat with two bulging ellipsoid subcutaneous MAT B III tumors. A FOPT is inserted in each tumor, guided by a Venflon™ tube. Plastic clay applied to prevent leakage. B. A FOPT with a sensor in its distal (left) end is shown resting on a finger tip. Note the small dimensions. C. A 0.5 mm space, filled with fluid in situ, is located between the orifice of the PTFE tube and the sensor (dark), enclosed by a protective PTFE tube. D. A side view of a FOPT showing to the left the sensor as a dark spot, inserted in a protecting PTFE tube. This construction prevents direct load on the sensor by the tissue, which may cause faulty recordings.
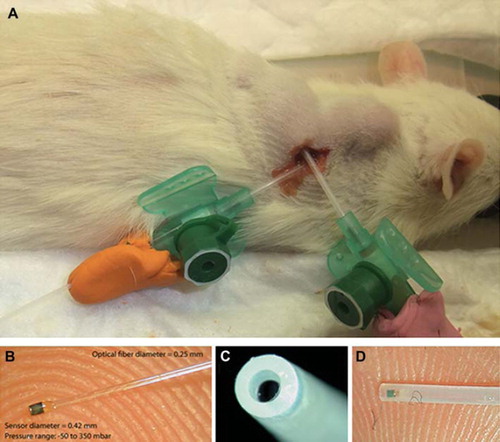
The animals were housed with a 12 h light cycle, at standardized humidity and temperature, and had access to pelleted feed and water ad libitum. The number of animals per treatment group is specified in . At the end of the experiments the animals were killed by opening up of the thorax cavity and sectioning of the heart.
Table I. Effects of AF-16 on IFP in DMBA tumors and in MAT BIII tumor.
Histology
Representative samples from both types of tumors were processed for histology. The tumors were fixed in 4% buffered formaldehyde, dehydrated and embedded in paraffin. Sections, cut 4 μm, were stained with hemaoxylin and eosin. A Zeiss Axio Image M1 microscope was used for documentation.
Ethics
Permits were granted by the Regional Animal Experiments Ethical Committee, and local and federal guidelines (EU 86/609/EEC) were followed.
Chemicals
The peptide AF-16 was synthesized and characterized by Ross Pedersen A/S, Copenhagen, Denmark. DMBA were purchased from Sigma-Aldrich. The cell culture medium RPMI 1640, supplemented with L-glutamine, was purchased from Flow Laboratories.
Administration of AF-16
The full sequence of the peptide AF-16 is: H-Val-Ser-His-Ser-Lys-Thr-Arg-Ser-Asn-Pro-Glu-Asn-Asn-Val-Gly-Leu-OH, and the molecular weight is 1738.89 D. AF-16 was either injected in the tumor capsule (ic; 500 μg/kg bw in 50 μL PBS), instilled intranasally (in; 500 μg/kg bw in 20 μL PBS) or injected intravenously (iv; 500 μg/kg bw in 500 μL PBS). Blood pressure was measured by cuffing the tail vessels (LE 5002, Panlab, Harvard Apparatus).
The tumor pressure recording system
A LED-based miniature fiber optic pressure transducers (FOPT; Samba 3200; Samba AB, V. Frölunda, Sweden) was used with a sensing element (Ø 0.42 mm) mounted on the tip of an optical glass fiber (Ø 0.25 mm; ) [Citation12–14]. The factory calibrated sensors were checked prior to and after each experiment by immersion in a measuring cylinder filled with water of known height. The software of the Samba equipment calibrated the recordings against the ambient air pressure. The recordings were mostly performed at 1 Hz, but occasionally at up to 1 kHz. The sensors were kept wet in between the recording sessions. Stable steady state recordings could not be observed until approximately 20 minutes post implantation, and any movement of the animals could cause disturbances.
Implantation of pressure sensors
The rats were kept anesthetized by inhalation of isoflurane. They were placed on a heating pad and the body temperature was monitored by a rectal probe. A Panlab Temperature Control Unit HB 101/2 controlled and maintained the body temperature.
VenflonTM infusion tubes guided the FOPT when inserted under ocular supervision (). In most experiments 2 FOPTs were used simultaneously. The sensors were positioned a few mm distal to the VenflonTM needle eye. Great care was taken to ensure that there was no leakage during the recordings.
Statistics
Results are presented as means ± SD. Paired design tests were used, and p < 0.05 considered significant.
Results
Pressure recordings in normal tissue
The IFP in the subcutaneous connective tissue in normal Sprague-Dawley rats ranged from -3 to +1 mmHg (n = 24) with minor variations due to respiration and cardiovascular activities.
Pressure recordings in DMBA-induced tumors
The IFP in the DMBA induced tumors increased with increasing size. Tumors with a diameter of 7–10 mm had an IFP of 8.4 ± 3.1 mmHg (n = 6), increasing to 15.7 ± 4.3 mmHg (n = 15) in tumors with a diameter of 15–25 mm, in agreement with published results [Citation2]. The IFP in the capsule was 4.7 ± 1.4 mmHg (n = 15) at a tumor diameter of at least 15 mm.
The pressure recordings in the DMBA tumors after an injection of 500 μg/kg bw of AF-16 either intracapsularly (ic), intranasally (in) or intravenously (iv) are shown in . After any manipulation of the rats it took up to 30 minutes to achieve stable IFP recordings, as previously reported [Citation3].
A significant reduction in the IFP was achieved after 30 min and lasted at least 3 h, irrespective of the administration route (). A recording from one experiment, at which AF-16 was injected in the tumor capsule, is shown in . The IFP started to decrease after 30 min and approached a low level after 90 min. A single dose of AF-16 kept the pressure lowered for at least 3 h prior to a slowly evolving increase of the IFP. AF-16 administrated intravenously to animals with two tumors of similar dimensions, resulted in a similar IFP reduction in each tumor (). The IFP returned to the pretreatment, elevated level within 24 h. If the AF-16 treatment was repeated after 24 h a similar, transient reduction of the IFP was recorded. When the pressure sensors were implanted with no further manipulations of the animals, the IFP remained stable.
Figure 3. Effects of AF-16 on the IFP in DMBA tumors (A, B), and in MAT B III tumor (C). A. Recording of the IFP in central parts of a DMBA tumor starting 30 min after an intracapsular injection of 500 μg/kg bw of AF-16. The tumor IFP (red) decreased in 3 h to less than 40% of the pretreatment level. The IFP in adjacent normal subcutaneous connective tissue (green) remained unaffected. B. The IFP in two adjacent, but non-communicating tumors (red and yellow) decreased in parallel after intravenous injection of 500 μg/kg bw of AF-16. C. Injection of 500 μg/kg bw of AF-16 once daily in the capsule zone of a MAT B III tumor during two consecutive days reduced the IFP both days.
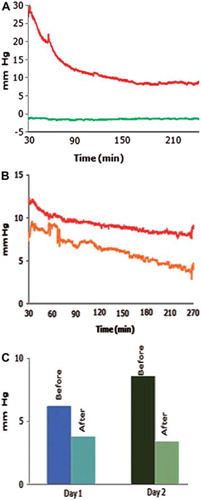
After an ic injection of the vehicle (PBS) a small transient rise in IFP was registered where after the IFP remained stable for at least 1 h.
Pressure recordings in solid MAT B III tumors
The IFP in the normal subcutaneous tissue of Fisher rats ranged from -4 to +1 mmHg (n = 17), and that in the tumor capsule zone was 2.6 ± 1.7 mmHg (n = 17). Solid MAT B III tumors with a diameter of 15–25 mm had an IFP of 13.8 ± 3.5 mmHg (n = 17). Tumors with a diameter in excess of 20 mm become in parts necrotic and debris-filled cysts appeared, and were excluded.
In the IFP recordings of the MAT B III tumors after treatment with 500 μg/kg bw of AF-16 either intracapsularly, intranasally or intravenously are shown. In this tumor the intracapsular administration was most efficient, resulting in a significant IFP reduction 30 min after the AF-16 injection. The IFP remained reduced for at least 180 min. The intranasal administration of AF-16 significantly lowered the IFP after 90 min and it remained at that level for at least 180 min (). The iv route was less effective and a significant reduction in IFP was not demonstrated until after 120 min. The tumor IFP returned to pretreatment levels in 24 h, and if the AF-16 treatment was repeated the following day, a reduction of the tumor IFP level was recorded once more (). AF-16 did not affect the IFP in the MAT B III capsule zone. The baseline IFP remained at a stable level for a prolonged period.
Effect of AF-16 on systemic blood pressure
Measurement of blood pressure in the IFP experiments revealed no influence of AF-16 compared to vehicle, irrespective of the administration route. The blood pressure remained unaffected during a three hour period in the IFP experiments, and the heart rate was not altered.
Discussion
The main result in the present study was that the peptide AF-16 lowered the raised tumor IFP in two different solid tumor types. The effect of AF-16 on the tumor IFP was rapid, transient, reversible and repeatable. The IFP in the tumor capsule and in the adjacent, subcutaneous connective tissue was not influenced by AF-16. No cardiovascular or systemic effects were recorded in the acute IFP experiments.
The IFP recorded in the present study was of the same magnitude as those previously reported for DMBA tumors [Citation2]. Previous measurements have commonly been based on delicate micropipettes, or the wick-in-needle technique [Citation2,Citation4]. An important advantage of the used miniature FOPT was the robust construction allowing reliable registrations in an environment with blood clots, edema as well as necrotic and tense tissues. The sensor could easily be moved to demanded positions in the tumor and adjacent tissue, enabling mapping of pressure gradients and checking for possible leakage [Citation12,Citation13]. As previously reported [Citation3], manipulation of the animals affected the registrations, but reliable recordings could again be obtained up to 30 min thereafter.
The two tumors used in the present study differed in several aspects. The DMBA induced tumors were slowly growing in a differentiated glandular fashion and were enveloped by a dense connective tissue capsule. In contrast, the pleomorphic MAT B III tumors, evolving in a week or two after subcutaneous deposition of cultured cells, were rapidly growing. They were characterized by high cellularity, scanty extracellular matrix with no distinct collagen network and they lacked a distinct capsule. Thus, two histopathologically divergent types of tumors were utilized in the present study. Despite these differences, AF-16 reduced the raised IFP in both types of tumors, suggesting a common mechanism of action. The different AF-16 administration routes all reduced the tumor IFP (), although with variation in onset times. Surprisingly, in the MAT III B tumors the intranasal route was more effective than the intravenous route. We have no explanation for this phenomenon, but previous experiments have shown that intranasal administration is a reliable and effective way of delivering peptides [Citation12].
Different approaches have been reported to lower the IFP. In tumors with pharmacologically induced apoptosis, which diminish the total tissue volume, the tumor IFP decreases [Citation8,Citation15,Citation16]. Reduction of the IFP may further be achieved by oophorectomy for hormone dependent tumors [Citation17] or by treatment with enzymes [Citation4,Citation7,Citation18,Citation19]. However, the latter approaches may increase the risk for tumor dissemination [Citation20]. Drainage of fluid from the tumor tissue alters the mechanical parameters, and affects the cell proliferation. Treatment with drugs interacting with, for example cytokines, kinases, growth factors and their receptors affect the IFP, and can improve the access of drugs or tracers to tumors [Citation4,Citation6–9,Citation21,Citation22]. Such treatment regimens require up to two weeks or even more before a sufficient lowering of tumor IFP can be demonstrated, and the lowered IFP persists for a long time period. The effects achieved with antibodies to growth factors and kinase inhibitors are associated with rebuilding of the blood vessels and the interstitial tissue [Citation4,Citation22]. Furthermore, such substances are toxic and may also induce adverse reactions and a multitude of side effects, some of which may be life threatening. In contrast, AF-16 was not toxic and did not induce any side effects at used doses. Further, AF-16 transiently decreased the tumor IFP in 30–90 min, and the IFP returned to the pre-treatment, elevated level within 24 h.
The mechanism for the transient decrease in the tumor IFP after AF-16 is not clear, but is assumed to be related to an anti-inflammatory effect translating into reduced cellular edema and less vascular leakage. AF was originally identified by its capacity to normalize cholera toxin induced hyper secretion in the small intestine [Citation11], and experimental and clinical studies have shown that AF is also a potent anti-inflammatory agent [Citation23–25]. Furthermore, we have shown that AF significantly influences the transport of GABA and chloride ions across isolated neuronal membranes of Deiter's cells [Citation26], indicating that AF-16 could affect the cellular transport of ions and water also in vivo. AF is an evolutionary ancient protein which exerts its effects in many different tissues [Citation11]. It is likely that AF acts through a basal signal pathway and/or by affecting a ubiquitous cell population. Ongoing tissue culture studies indicate that AF-16 can affect cytokine release in microglia, indicating that monocyte-derived cell populations may be one target for AF-16.
In conclusion, we have demonstrated that the peptide AF-16 transiently and reversibly lowered the raised IFP in solid tumors during several hours. Local as well as systemic AF-16 treatment reduced the tumor IFP in both investigated types of solid tumors. AF-16 did not influence the IFP in normal, subcutaneous tissue, nor were there any systemic side effects registered. We suggest that AF-16, as an adjuvant, can be of interest for further investigations in experimental tumor therapy models.
Acknowledgments
We thank Dr. Svante Höjer, Samba Sensors AB, for advices, support and help; Professor Ingvar Bosaeus for expert statistical advice; Ingela Jonsson and Margareta Rosenkvist for invaluable technical assistance. This work was in parts presented 2010 at an AACR conference on Tumor Immunology: basic and clinical advances, Miami, Florida, USA (# B9). Grant support from the Swedish federal government under the LUA/ALF agreement (grant # 71570), Sahlgrenska University Hospital (grant # 83030), the Ministry of High Education UAE, Magnus Bergvall Foundation, W. and M. Lundgren Foundation, Frimurare- Barnhus direktionen, Göteborg Medical Society, AB Nectin, and Lantmännen AS-Faktor AB.
Declaration of interest: The authors report no conflicts of interest. The authors alone are responsible for the content and writing of the paper.
References
- Heldin CH, Rubin K, Pietras K, Östman A. High interstitial fluid pressure – an obstacle in cancer therapy. Nature Rev Cancer 2004;4:806–13.
- Wiig H, Tveit E, Hultborn R, Reed RK, Weiss L. Interstitial fluid pressures in DMBA-induced rat mammary tumors. Scand J Clin Lab Invest 1982;42:159–64.
- Flessner MF, Choi J, Credit K, Deverkadra R, Henderson K. Resistance of tumor interstitial pressure to the penetration of intraperitoneally delivered antibodies into metastatic ovarian tumors. Clin Cancer Res 2005;11:3117–25.
- Jain RK, Duda DG. Vascular and interstitial biology of tumors. Abeloff's Clinical Oncology, 4th. London: Churchill Livingstone; 2008. 105–124.
- Rubin K, Sjöquist M, Gustafsson AM, Isaksson B, Salvesson G, Reed RK. Lowering of tumoral interstitial fluid pressure by prostaglandin E1 is paralleled by an increased uptake of 51Cr-EDTA. Int J Cancer 2000;86:636–43.
- Salnikov AV, Iversen VV, Koisti M, Sundberg C, Johansson L, Stuhr LB, . Lowering of tumor interstitial fluid pressure specifically augments efficacy of chemotherapy. FASEB J 2003;17:1756–68.
- Magzoub M, Jin S, Verkman AS. Enhanced macromolecule diffusion deep in tumors after enzymatic digestion of extracellular matrix collagen and its associated proteoglycan decorin. FASEB J 2008;22:276–84.
- Nagano S, Perentes JY, Jain RK, Boucher Y. Cancer cell death enhances the penetration and efficacy of oncolytic herpes simplex virus in tumors. Cancer Res 2008;68:3795–802.
- Reed RK, Rubin K. Transcapillary exchange: Role and importance of the interstitial fluid pressure and the extracellular matrix. Cardiovasc Res 2010;87:211–7.
- Johansson E, Lange S, Lönnroth I. Identification of an active site in the Antisecretory Factor protein. Biochim Biophys Acta 1997;1362:177–82.
- Johansson E, Lönnroth I, Lange S, Jonson I, Jennische E, Lönnroth C. Molecular cloning and expression of a pituitary gland protein modulating intestinal fluid secretion. J Biol Chem 1995;270:20615–20.
- Jennische E, Bergström T, Johansson M, Nyström K, Tarkowski A, Hansson HA, . The peptide AF-16 abolishes sickness and death at experimental encephalitis by reducing increase of intracranial pressure. Brain Res 2008; 1227:189–97.
- Krave U, Höjer S, Hansson HA. Transient, powerful pressures are generated in the brain by a rotational acceleration impulse to the head. Eur J Neurosci 2005;21:2876–82.
- Ozerdem U. Measuring interstitial fluid pressure with fiberoptic pressure transducers. Microvasc Res 2009;77: 226–9.
- Griffon-Etienne G, Boucher Y, Brekken C, Suit HD, Jain RK. Taxane-induced apoptosis decompresses blood vessels and lowers interstitial fluid pressure in solid tumors: Clinical implications. Cancer Res 1999;59:3776–82.
- Hofmann M, Schultz M, Bernd A, Bereiter-Hahn J, Kaufmann R, Kippenberger S. Long-term lowering of tumor interstitial fluid pressure reduces Ki-67 expression. J Biomech 2007;40:2324–9.
- Hultborn R, Tveit E, Angerås M, Köpf I, Weiss L. Effects of oophorectomy on interstitial fluid pressure, blood flow and vascularity in rat mammary tumor. Anticancer Res 1997; 17:1877–80.
- Brekken C, Hjelstuen MH, Bruland OS, de Lange Davies C. Hyaluronidase-induced periodic modulation of the interstitial fluid pressure increases selective antibody uptake in human osteosarcoma xenografts. Anticancer Res 2000; 20:3513–9.
- Eikenes L, Bruland OS, Brekken C, de Lange Davies C. Collagenase increases the transcapillary pressure gradient and improves the uptake and distribution of monoclonal antibodies in human osteosarcoma xenografts. Cancer Res 2004;64:4768–73.
- Deryugina EI, Quigley JP. Pleiotropic roles of matrix metalloproteinases in tumor angiogenesis: Contrasting, overlapping and compensatory functions. Bioch Bioph Acta Mol Cell Res 2010;1803:103–20.
- Pietras K, Östman A, Sjöquist M, Buchdunger E, Reed RK, Heldin CH, . Inhibition of PDGF receptors reduces interstitial hypertension and increases transcapillary transport in tumors. Cancer Res 2001;61:2929–34.
- Svendsen ØS, Barczyk MM, Popova SN, Liden Å, Gullberg D, Wiig H. The α11β1 integrin has a mechanistic role in control of interstitial fluid pressure and edema formation in inflammation. Arterioscl Thromb Vasc Biol 2009;29:1864–70.
- Björck S, Bosaeus I, Ek E, Jennische E, Lönnroth I, Johansson E, . Food induced stimulation of the antisecretory factor can improve symptoms in human inflammatory bowel disease: A study of a concept. Gut 2000;46:824–9.
- Eriksson A, Shafazand M, Jennische E, Lange S. Effect of antisecretory factor in ulcerative colitis on histological and laborative outcome: A short period clinical trial. Scand J Gastroenterol 2003;38:1045–9.
- Hanner P, Rask-Andersen H, Lange S, Jennische E. Antisecretory factor-inducing therapy improves the clinical outcome in patients with Meniere's disease. Acta Otolaryngol 2010;130:223–7.
- Rappalino MV, Lange S, Lönnroth I, Cupello A. Antisecretory Factor peptide derivatives specificly inhibits (3H)-GABA/36Cl- out → in transport across the isolated rabbit Deiters´neuronal membrane. Acta Phys Scand 2003; 179:367–71.